- 1Department of Biochemistry and Molecular Biology, School of Basic Medical Science, Southwest Medical University, Luzhou, China
- 2Department of Dermatology, The Affiliated Hospital of Southwest Medical University, Luzhou, China
Ferroptosis is a recently discovered category of programmed cell death. It is much different from other types of cell death such as apoptosis, necrosis and autophagy. The main pathological feature of ferroptosis is the accumulation of iron-dependent lipid peroxidation. The typical changes in the morphological features of ferroptosis include cell volume shrinkage and increased mitochondrial membrane area. The mechanisms of ferroptosis may be mainly related to lipid peroxidation accumulation, imbalance in amino acid antioxidant system, and disturbance of iron metabolism. Besides, hypoxia-inducible factor (HIF), nuclear factor-E2-related factor 2 (Nrf2), and p53 pathway have been demonstrated to be involved in ferroptosis. At present, the molecular mechanisms of ferroptosis pathway are still unmapped. In this review, an outlook has been put forward about the crucial role of apurinic/apyrimidinic endodeoxyribonuclease 1 (APEX1) in the regulation of ferroptosis. APEX1 plays an important role in the regulation of intracellular redox balance and can be used as a potential inhibitor of ferroptotic cell death. Bioinformatics analysis indicated that the mRNA level of APEX1 is decreased in cases of ferroptosis triggered by erastin. Besides, it was found that there was a significant correlation between APEX1 and genes in the ferroptosis pathway. We have discussed the possibility to employ APEX1 inducers or inhibitors in the regulation of ferroptosis as a new strategy for the treatment of various human diseases.
Introduction
Cell death plays an important role in the development, aging, tissue homeostasis, immunity, and stress tolerance of all multicellular organisms. There are several types of cell death, such as apoptosis, necrosis, and autophagy. These different types of cell death play an important role in the progression of various diseases, such as cancer (1), neurodegenerative diseases (2), and cardiovascular diseases (3). A variety of drugs has been used to target these types of cell death in order to treat many human diseases. Ferroptosis is a novel type of cell death discovered by Dixon in 2012 (4). The mechanisms of ferroptosis are generally accompanied by the accumulation of large amount of iron and lipid peroxide in cells. The main characteristics of ferroptosis include a wrinkling of mitochondrial morphology and loss of mitochondrial cristae, while no nuclear sequestration or chromatin border set is found. Also, in contrast to the classical autophagy pathway, ferroptosis does not form any autophagic lysosome structure (4).
Iron is an essential trace element found in all organisms and it plays a key role in the smooth functioning of the body. Cellular iron uptake is mediated mainly by transferrin (Tf) and transferrin receptor1 (TfR1) (5). Under normal physiological conditions, extracellular iron binds to Tf, and in turn Tf binds to TfR1 that is present on the surface of the cell membrane to form iron-Tf-TfR1 complex. Thus formed iron-Tf-TfR1 complex enters the cell through endocytosis. Under the action of divalent metal transporter 1 (DMT1), the extracellular iron enters the cytoplasm forming labile iron pool (LIP) that can be used by the mitochondria or it can also be used by the cytoplasm itself. This intracellular iron also can be stored by ferritin or it can be transported to the exterior of the cell through ferroportin 1 (FPN1) (6). Therefore, under pathological conditions, intracellular iron overload is an important cause of ferroptosis. Zhang et al. have reported that ferroptosis triggered by iron overload plays an important role in Parkinson’s disease (7). Besides, many studies have reported that excess free iron can generate reactive oxygen species (ROS) through Fenton reaction (8, 9). Iron circulates between the reduced and oxidized states, resulting in the formation of free radicals. There is no doubt that the occurrence of ferroptosis is directly related to the presence of iron in the cell. Hence, level of iron present in the cell directly determines the mechanism of ferroptosis.
Cystine/glutamate transporter (system XC-) is an important transporter that mediates the exchange of extracellular cystine (Cys2) and intracellular glutamate (Glu). It is composed of two subunits, namely, solute carrier family 7 member 11 (SLC7A11) and solute carrier family 3 member 2 (SLC3A2). The basic function of system XC- is to absorb Cys2 and excrete Glu. First, Cys2 is absorbed by system XC- and reduced to cysteine (Cys). Next, Cys takes part in the synthesis of glutathione (GSH) (10). Later, GSH can convert toxic lipid hydroperoxides (L-OOH) into non-toxic lipid alcohols (L-OH) by using GSH peroxidase 4 (GPX4) (11). Therefore, inhibition of GSH synthesis will lead to oxidative damage, thereby resulting in cell death. GPX4, (a member of the GPXs family), plays an important role in ferroptosis. The main function of GPX4 is promotion of decomposition of hydroperoxide and protection of the structure and function of the cell membrane from oxidative damage (12). Therefore, inhibition of GPX4 synthesis will lead to oxidative damage or ferroptosis (13). It can be understood that there is increasing evidence stating that the inducer of ferroptosis, such as erastin (14), RSL3 (15), has a promising future in the treatment of cancer. At the same time, the inhibitor of ferroptosis such as ferrostatin-1 (16), liproxstatin-1 (17), etc. can contribute to the treatment of various nervous system diseases and cardiovascular diseases.
Apurinic/Apyrimidinic endodeoxyribonuclease 1 (APEX1), also named as reduction-oxidation factor-1 (Ref1), belongs to the DNA repair enzymes. It is a multifunctional protein and plays a central role in the cellular response to oxidative stress. On the other hand, APEX1 plays an important role in the repair of oxidized and alkylated genomic DNA bases by identifying and cleaving nucleotide chains at 5’ apurinic (AP) sites (18). APEX1 also exerts reversible nuclear redox activity to regulate DNA binding affinity and transcriptional activity of transcriptional factors by controlling the redox status of their DNA-binding domain. In this review, we mainly focus on the function of APEX1 in antioxidant and regulating transcriptions. Previous studies have reported that APEX1 overexpression enhances the ability to resist oxidative stress and reduces the levels of ROS (19, 20). Besides, Daniel et al. have demonstrated that the accumulation of iron inhibits the activity of APEX1 (21). It has been well researched and concluded that the accumulation of iron and ROS is an important cause of ferroptosis (4). APEX1 is originally identified as a DNA repair enzyme and shown to be important for the base excision repair pathway (22, 23). In addition, it was demonstrated that APEX1 reduces a redox-sensitive cysteine residue of the transcription factor activator protein-1 (AP-1) and thereby facilitates its DNA-binding and transcriptional activities (24). There is increasing evidence that APEX1 is widely involved in various diseases caused by oxidative stress, such as Parkinson’s disease (20), ischemic stroke (25), Alzheimer’s disease (26), and cancer (27). Besides, many studies have proven that ferroptosis is involved in the occurrence as well as the development of these diseases. Therefore, we suggest that APEX1 may inhibit ferroptosis. However, further mechanistic details of the APEX1-ferroptosis link remain to be unclear. In this review, we highlighted the potential roles of APEX1 in ferroptosis. We hope to further explore the ferroptosis signaling pathway. It can be suggested that APEX1 is involved in various diseases through ferroptosis pathway. In the future, APEX1 will be an important target for the treatment of various human diseases.
APEX1 and Lipid Peroxidation in the Ferroptosis Pathway
The abnormal metabolism of amino acids is closely related to ferroptosis. Mounting evidences have reported that the absence of cysteine leads to the decrease of GSH synthesis, thereby resulting in the inactivation of GPX4 and the accumulation of lipid peroxidation (28–30). There are two forms of glutathione, namely reduced glutathione (GSH) and oxidized glutathione (GSSG). The antioxidant function of GSH relies on its sulfhydryl group, which is derived from cysteine. Therefore, the levels of intracellular cysteine affect the activity of GPX4, thereby affecting ferroptosis. The accumulation of lipid peroxidation caused by the inactivation of GPX4 is one of the important reasons to induce ferroptosis. Jie et al. have reported that APEX1 overexpression inhibits the accumulation of ROS and the decrease of GSH level (31). The decrease of GSH expression affects the activity of GPX4, indirectly. Studies have demonstrated that the lipid peroxidation and APEX1 expression are significantly higher in the tumor tissue compared to the non-tumor regions (32–36). We suggest that APEX1 overexpression can inhibit the accumulation of lipid peroxidation, thereby promoting the survival of the tumor cells. Due to the function of APEX1, APEX1 may be related to the repair of DNA damage, on the other hand, APEX1 is involved in activation of several antioxidant transcription factors. Thioredoxin-1 (Trx-1) is an important part of antioxidant system in cells (37). Earlier reports have demonstrated that Trx-1 can associate directly with APEX1 in the nucleus (38, 39). Hirota et al. have suggested that AP-1 activation in response to ionizing radiation involves the passage of redox signals through Trx-1 from the cytoplasm to the nucleus, followed by interaction with APEX1 (40). Besides, Ando et al. have found that APEX1, as a redox chaperone, can regulate DNA-binding activities of various transcription factors through promoting the reduction of their critical cysteine residues by other reducing molecules such as GSH and Trx-1 (41). Trx-1 also can inhibit the accumulation of lipid peroxidation (42, 43). Recently, Trx-1 has been proven to be an inhibitor of ferroptosis (44, 45). Therefore, these results suggested that APEX1 can inhibit the accumulation of lipid peroxidation by regulating the expression of antioxidant factors through the interaction with Trx-1.
APEX1 and System XC- in the Ferroptosis Pathway
GPX4 and system XC- are considered to be the main signaling pathways of ferroptosis (4). System XC- belongs to the family of heterodimeric amino acid transporters. The main function of system XC- is to exchange cystine with glutamate (46). It is well known that cisplatin is a very effective and widely used anticancer drug (47). Earlier reports have reported that the mechanism of action of cisplatin is mainly through the production of ROS, leading to apoptosis in osteosarcoma (48). Recent studies have suggested that cisplatin is also an inducer of ferroptosis (49–51). Tetsuro Sasada et al. have discovered the cisplatin-resistant variants of HeLa cells and the cisplatin-resistant cells also showed enhanced cystine uptake, which led to a significant increase in the content of intracellular sulfhydryl as well as GSH (52). Interestingly, overexpression of APEX1 can inhibit the accumulation of ROS induced by cisplatin (48, 53). Therefore, these findings suggested that APEX1 may play a potential role in the inhibition of cisplatin-induced ferroptosis through scavenging ROS. Also, APEX1 may be a key target in the ferroptosis pathway.
APEX1 and Nrf2 in the Ferroptosis Pathway
Nuclear factor-E2-related factor 2 (Nrf2) belongs to a small family of transcription factors, which has the oxidation resistance function. Kelch-like ECH-associated protein 1 (Keap1) binds to Nrf2 and causes rapid ubiquitination and degradation. It then inhibits the transcriptional activity of Nrf2. Under stressful conditions, the cysteine residues of Keap1 get modified, causing it to lose its ability to ubiquitinate Nrf2. This causes Nrf2 to enter the nucleus and activate a series of downstream target genes (54) (Figure 1). Recently, many studies have proven that the Nrf2/Keap1 pathway is closely related to ferroptosis. Fan et al. have suggested that activation of the Nrf2 pathway can upregulate the system XC- and overexpression of Nrf2 or knockdown of Keap1 expression promotes resistance to ferroptosis (55). Besides, there is increasing evidence suggesting that inhibition of the Nrf2/Keap1 pathway by targeting GPX4 could reverse the resistance towards ferroptosis (56–58). In other words, the activation of the Nrf2 pathway can induce the expression of antioxidant genes in the nucleus, thus resisting ferroptosis. In immunoprecipitation reaction experiment, Thakur et al. have found that APEX1 and Nrf2 physically interacts, which suggests that APEX1 mediates Nrf2 activation in lung cancer cells (59). Besides, Sriramajayam et al. have demonstrated that APEX1 is required for the activation of Nrf2 and subsequently activates the expression of antioxidant responsive element (ARE). The relationship between APEX1 and Nrf2 may be involved in the redox function of APEX1, which might be directly regulating the ARE-mediated neuronal survival (60) (Figure 1). To put it in other words, important oxidative stress genes, including Txnrd1, Hmox1, and Gpx4, which are involved in ferroptosis, will be expressed. These results implied that the crucial functions of APEX1 interacting with Nrf2 activates the expression of ARE and then resists ferroptosis induced by ROS. More importantly, many studies have confirmed that the relationship between APEX1 and Nrf2 is crucial for maintaining the homeostasis of ROS (60). It is now known that the APEX1/Nrf2/Keap1 is involved in a variety of human diseases and plays a significant role in Alzheimer’s disease (61) and cancer (59). All of these diseases have been reported to be closely related to ferroptosis. Therefore, APEX1 could possibly play a crucial role in the ferroptosis pathway (Figure 1).
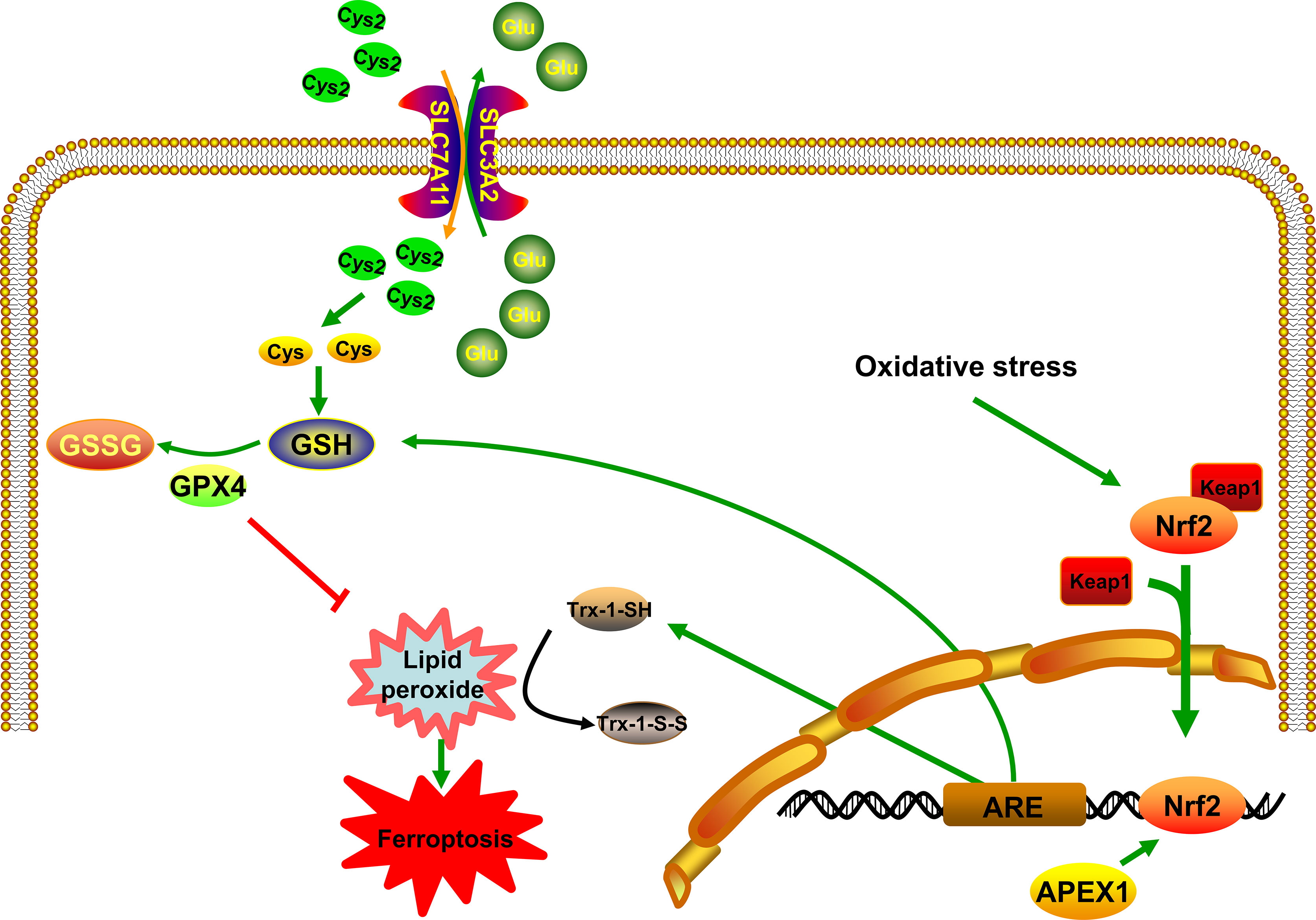
Figure 1 APEX1 inhibits ferroptosis by regulating Nrf2/ARE pathway. APEX1 induces the expression of ARE by regulating the activity of Nrf2. Trx-1 and GSH resist the accumulation of lipid peroxidation through its sulfhydryl group.
APEX1 and p53 in the Ferroptosis Pathway
Tumor suppressor protein p53 (TP53) plays an important role in cellular stress response to DNA damage and hypoxia (62). Low levels of stress can trigger the activation of p53, which induces cell cycle arrest, DNA repair, and survival of the cell. Downregulating intracellular ROS, p53 can protect the cells from oxidative stress-induced DNA damage and cell death. On the contrary, high levels of stress lead to the activation of p53, which induces apoptosis and cell death (62). In the recent past, p53 has been identified as a novel regulator in ferroptosis (63–65) and it has been identified to inhibit SLC7A11 gene transcription in ferroptosis (66) (Figure 2). Celia R. Berkers et al. have proposed that p53 inhibits the fatty acid synthesis and promotes fatty acid oxidation, thus playing a negative regulatory role in lipid synthesis (67). Ou et al. have proven that p53 can induce ferroptotic responses by directly activating its target gene SAT1, which is correlated with the expression level of arachidonate 15-lipoxygenase (ALOX15) (68) (Figure 2). Silencing of ALOX15 significantly decreases both RSL3-induced and erastin-induced ferroptosis in vitro (69). Activation of p53 significantly decreases system XC- expression (70). Upregulated p53 expression by Tanshinone IIA results in the destruction of cysteine import, which reduces glutathione production and promotes ROS mediated ferroptosis (71). Besides, it is known that p53 can enhance ferroptosis by promoting glutaminase 2 (GLS2). Knockdown of GLS2 expression inhibits ferroptosis through control of glutaminolysis (72) (Figure 2). Additionally, a large number of studies have shown that there is an important regulatory relationship between APEX1 and p53. Zhu et al. have found that Genistein stabilizes p53 through targeting interaction between APEX1 and p53. The interaction between APEX1 and p53 can promote p53 degradation. Interestingly, under oxidative stress condition, APEX1 will be oxidized by ROS and dissociate from the p53, and thus to stabilizing p53 (73). According to the above research, the possible role of APEX1 as an oxidative stress sensor may inhibit ferroptosis by interacting p53 protein. Additionally, Cun et al. have proven that downregulation of APEX1 can enhance the sensitivity of p53 mutant tumor cells to radiotherapy in-vitro and in-vivo. In other words, the possible mechanism of APEX1 regulating p53 represented that APEX1 is involved in the development of ferroptosis. Several studies have proven that APEX1 is overexpressed in many human tumors (74). In addition, tumor tissues also exhibit increased levels of ROS than normal tissues (75, 76). However, under sustained oxidative stress, tumor tissues become well-adapted to such stress through a series of mechanisms, and it often has defects in the mechanism of cell death, which is one of the primary reasons for drug resistance. Therefore, we realize that overexpression of APEX1 in tumor cells may be a protective mechanism to resist the accumulation of lipid peroxidation and make tumor cells survival. APEX1 overexpression in tumor cells could be owing to the following reasons: one is to resist the high levels of ROS; the other is to prevent the death of tumor cells by resisting apoptosis and ferroptosis by interacting p53 protein. This may be the reason for the high expression of APEX1 in tumor cells.
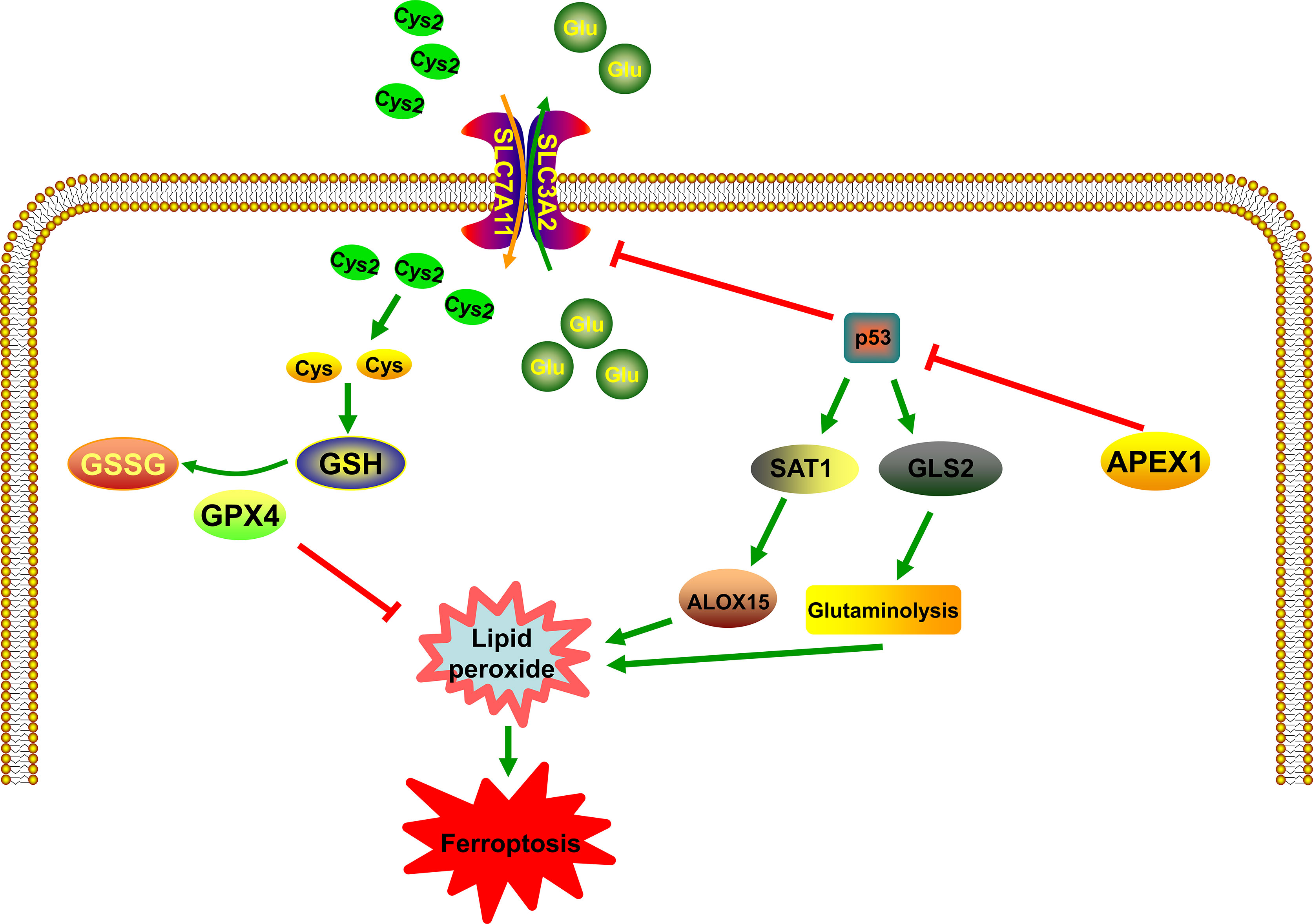
Figure 2 The relationship between APEX1 and p53 in the control of ferroptosis. p53 plays an important role in the regulation of lipid peroxidation in ferroptosis. p53 can promote ferroptosis through the inhibition of system XC- expression or the induction of SAT1 and GLS2 expression. APEX1 is involved in the development of ferroptosis by regulating p53.
APEX1 and Hypoxia-Inducible Factor-1 (HIF-1) in the Ferroptosis Pathway
HIF-1 is a transcriptional complex that plays an important role in the regulation of gene expression by oxygen. It was first identified as a transcriptional activator, binding to the hypoxia response element (HRE) in the promoter region of erythropoietin (77). HIF consists of a constitutively present β subunit and oxygen-regulated α subunit. HIF subunits can be divided into three types: HIF-1α, HIF-2α, and HIF-3α. Among them, HIF-1α is the most widely studied α isoforms and expressed in all human cell types. Recently, studies have demonstrated that HIF-1 is involved in many diseases by regulating ferroptosis pathway, such as stroke (78), cancer (79) and some other neurological diseases (80). The rapid growth of tumor will lead to local hypoxia. HIF-1α can activate the expression of downstream target gene vascular endothelial growth factor (VEGF), induce tumor cells to generate blood vessels, and bring oxygen and nutrients to tumor cells. Inhibitors of HIF-1α can suppress the proliferation, growth, metastasis and invasion of tumor cells. More importantly, current studies have reported that HIF-1α can limit ferroptosis by influencing lipid metabolism and storing lipids in droplets (81), thus attenuating peroxidation-mediated damage (82). Moreover, recent studies have demonstrated that HIF-1α can activate the Nrf2 pathway to protect from ischemia-reperfusion cardiac (83) and skeletal muscle injuries (84). Heme oxygenase-1 (HO-1) is one of the HIF target genes (85). Lately, it has been reported that HO-1 has a dual role in ferroptosis (86, 87) (Figure 3). Feng et al. have reported that ferrostatin-1, a ferroptosis inhibitor, can resist ferroptosis induced by diabetic nephropathy by regulating HIF-1α/HO-1 pathway (88) (Figure 3). Besides, in the brain, M30, as an iron chelator, can stabilize HIF-1α (89). This leads to the ability of iron chelators to stabilize HIF-1α thereby adding to the protective effects, through their ability to prevent the accumulation of lipid peroxide via the Fenton reaction. Interestingly, Bianchi et al. have reported that the iron deprivation can stimulate TfR transcription by regulating HIF-1α (90). These results confirmed that HIF-1α is a key factor in ferroptosis. Stabilizing of HIF can probably inhibit the accumulation of lipid peroxide and promote the expression of VEGF, which contributes to survival of tumor. In other words, it may inhibit ferroptosis, indirectly. Latest researchers have mentioned that ferroptosis is involved in many nervous system diseases and inhibition of HIF can lead to neuronal cell death. Regulating the expression of HIF may be a potential target in ferroptosis. APEX1 can promote DNA binding activity by regulating a variety of transcription factors, including HIF (91). Huang et al. have demonstrated that overexpression of APEX1 significantly potentiates hypoxia-induced expression of a reporter construct containing the wild-type HIF-1 binding site (92). Logsdon et al. have reported that APEX1 interacts with HIF-1α under hypoxia and inhibition of APEX1 will result in decreased HIF-1α–mediated induction of carbonic anhydrase IX, which will inhibit viability of cancer cells (93). At present, no HIF-1-specific inhibitors currently exist, so targeting APEX1 regulate HIF activity is a promising method to modulate ferroptosis signaling in tumor. Interestingly, a previous in-vitro study has proven that an inhibitor of APEX1, E3330, lead to tumor growth inhibition (94). Besides, E3330 exposure promotes endogenous ROS formation in pancreatic cancer cells, thereby inhibiting cancer cell growth and migration (95). Based on the above research findings, it can be mentioned that APEX1 acts as an endogenous antioxidant factor in response to acute and chronic oxidative stress conditions by regulating HIF pathway. The accumulation of ROS and HIF pathway are involved in ferroptosis, however, there has been no reports that whether APEX1 plays a key role in regulating ferroptosis. These results indicated that APEX1 may be involved in ferroptosis by regulating transcription factors through its antioxidant capacity.
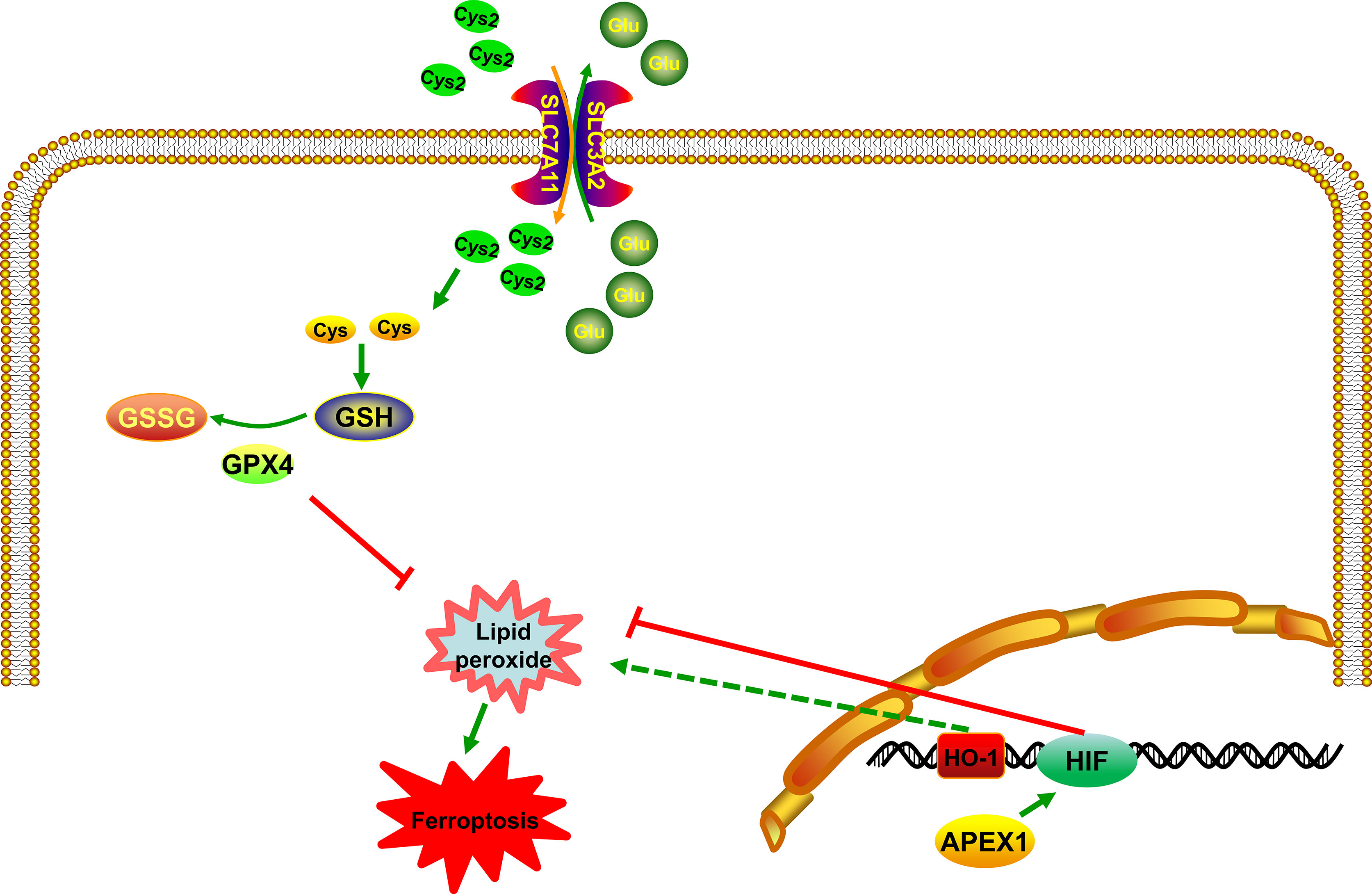
Figure 3 APEX1 inhibits ferroptosis by regulating the HIF pathway. APEX1 regulates regulate ferroptosis by interacting with HIF. HO-1 has a dual role in ferroptosis.
Discussion
Ferroptosis is a new type of cell death found in recent years. It is involved in a variety of molecular expression and signaling pathways. The metabolic imbalance of iron and the accumulation of lipid peroxide are the primary mechanisms of ferroptosis. Since the discovery of ferroptosis, the specific molecular mechanism still remained unmapped. However, many studies have confirmed that ferroptosis pathway is involved in the occurrence and development of many human diseases such as cancer (96), kidney diseases (97), and brain diseases (98). With the development of drug research, we have also identified several highly effective drugs that can be used in the targeting of ferroptosis to treat various diseases such as acetaminophen, sulfasalazine, and so on. Potential mechanisms of drugs used in the treatment of diseases by targeting ferroptosis are shown in Table 1. In this review, the possible molecular mechanisms of ferroptosis signaling pathways have been focused, and it is observed that the expression and activity of APEX1 play a vital role in regulating ferroptosis pathways, including system XC-, lipid peroxidation, Nrf2, p53 and HIF. APEX1 is a dual-function protein containing a redox domain and a DNA repair domain. Besides, APEX1 also can act as an essential transcription factor to regulate gene expression (18). Okazaki et al. have proven that APEX1 functions as a trans-acting factor that binds to negative calcium response elements (nCaRE) complex in the human PTH gene promoter (131). In addition, recent studies have reported that APEX1 plays a key role in the regulation of apoptosis (132) and autophagy (133). In this review, we report the possible mechanisms of APEX1 regulating ferroptosis, and these mechanisms beckon further in-detail studies. At least, we indicate that APEX1 may inhibit ferroptosis by regulating redox balance through regulating transcription factors. A previous study has demonstrated that Trx-1 inhibits 1-methyl-4-phenyl-1,2,3,6-tetrahydropyridine (MPTP)-induced ferroptosis by upregulating the expression of GPX4 (45). Besides, Evijola Llabani et al. have proven that the Trx-1 inhibitor can cause the occurrence of ferroptosis, directly (44). Importantly, we highlight that there is an interaction between APEX1 and Trx-1. In other words, APEX1 may be an important molecule in the ferroptosis pathway by inhibiting the accumulation of lipid peroxidation. In addition, APEX1 may affect ferroptosis by regulating other pathways. Owing to the role of APEX1 in many signaling pathways, the inducer or inhibitor of APEX1 may be a more effective drug for the treatment of various clinical diseases. For example, APEX1 can inhibit both apoptosis and ferroptosis, so it may be more effective than other drugs in the treatment of various diseases. At present, the inhibitor of APEX1, E3330, has been used to inhibit tumor migration in pancreatic cancer and non-small cell lung cancer cells (134). Previous studies have reported that Parkinson’s disease can cause neuronal ferroptosis (135, 136). Importantly, Kang et al. have demonstrated that APEX1 overexpression inhibits the increase of ROS induced by Parkinson’s disease model (20). Therefore, APEX1 may be a promising target to treat cancer and nervous diseases by regulating ferroptosis.
So how APEX1 regulates the transcription factors? Previous study has reported that the redox function of APEX1 depends primarily on a buried cystine residue. Cys65, Cys93, and Cys99 are necessary for its redox activity, which relates to a redox cycle through the formation of intermolecular disulfide bonds. Among them Cys65 functions as the nucleophilic cysteine, while the others are involved in resolving disulfide bonds that are formed in APEX1 (137). Xanthoudakis et al. have given insight that APEX1 can stimulate AP-1 DNA-binding activity through the conserved Cys residues in Fos and Jun, which may regulate eukaryotic gene expression (138). Cardoso et al. have proven that the binding of signal transducer and activator of transcription 3 (STAT3) to DNA is regulated by the redox function of the APEX1, directly (139). APEX1 inhibition decreased the expression of HIF-1α (93). Nishi et al. have demonstrated that APEX1 can reduce Cys p62 of p50, resulting in the activation of NF-κB DNA binding (140). APEX1 redox function was required for GSK-3β-mediated APEX1 regulation of Nrf2 in Barrett’s related esophageal adenocarcinoma cells (60). Therefore, these transcription factors may be activated by APEX1, presumably through the same redox process. Besides, in order to further illustrate the potential molecular mechanism of APEX1 in ferroptosis, we analyzed transcriptome datasets downloaded from NCBI [GSE104462 (141) and GSE154425 (142)]. It was found that under the action of special ferroptosis inducer erastin, the mRNA level of APEX1 was decreased compared to control group in HepG2 cells (GSE104462) (Figure 4A). Also, the mRNA level of APEX1 was decreased compared to control group in HCC38 cells (GSE154425) (Figure 4D). Volcano plots showed visualizing expression of different genes screening and cluster analysis (Figures 4B, E). Spearman’s correlation of any of two genes was calculated. The genes with a significant correlation (P < 0.05) were found to be the coordinated expression. If two genes resulted in a negative correlation then one gene was concluded to be downregulated, while the other to be upregulated. If two genes resulted in a positive correlation, then they are confirmed either to be down-regulated or up-regulated. Figure 4C exhibited that APEX1 had significant Spearman’s correlations with genes in the ferroptosis pathway, including GPX4, SLC7A11, SLC11A2, voltage dependent anion channel (VDAC2), glutamate-cysteine ligase modifier subunit (GCLM), poly (rC) binding protein 1 (PCBP1), PCBP2, STEAP3 metalloreductase (STEAP3), microtubule associated protein 1 light chain 3 beta 2 (MAP1LC3B2), MAP1LC3C, lysophosphatidylcholine acyltransferase 3 (LPCAT3), glutamate-cysteine ligase catalytic subunit (GCLC), prion protein (PRNP), and acyl-CoA synthetase long chain family member 1(ACSL1) (GSE104462). Also, Figure 4F exhibited that APEX1 had significant Spearman’s correlations with genes in the ferroptosis pathway, including SLC7A11, STEAP3, GCLC, heme oxygenase 1 (HMOX1), ACSL4, and MAP1LC3C. The above data indicated that APEX1 expression decreased significantly in ferroptosis induced by erastin. APEX1 correlated with genes in the ferroptosis pathway. APEX1 may be involved in ferroptosis, however, the specific mechanisms need further study. Through this review, further understanding of the possible signaling pathways of ferroptosis are undertaken. APEX1 inhibitors or knockdown of APEX1 expression to promote tumor cell death by regulating the ferroptosis pathway can be utilized in the treatment of cancer. Alternatively, we can use APEX1 inducer or overexpression of APEX1 to resist several neurological diseases by inhibiting the ferroptosis pathway. Therefore, we propose that APEX1 may be a potential target for regulating ferroptosis.
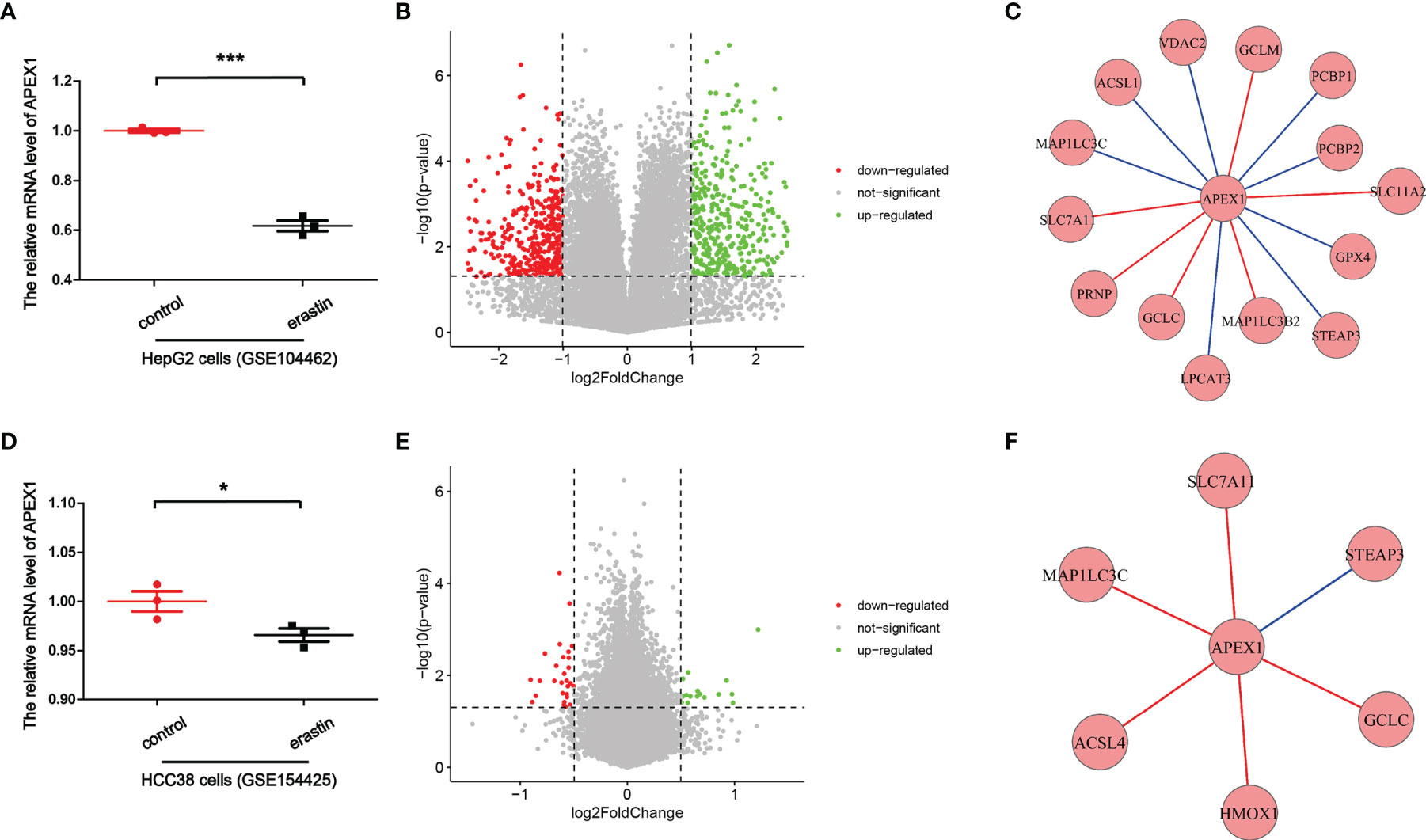
Figure 4 Bioinformatics analysis for APEX1 transcriptional expression. (A) The relative mRNA level of APEX1 in HepG2 cells. (GSE104462) (***P < 0.001, n = 3). (B) Volcano plot showed significantly differentially expressed genes. (GSE104462). (C) APEX1 correlated with genes in the ferroptosis pathway. (GSE104462). (D) The relative mRNA level of APEX1 in HCC38 cells. (GSE154425) (*P < 0.05, n = 3). (E) Volcano plot showed significantly differentially expressed genes. (GSE154425). (F) APEX1 correlated with genes in the ferroptosis pathway. (GSE154425).
Here, we have majorly reviewed the possible regulatory mechanisms of APEX1 in the ferroptosis pathway. So far, there has been no direct evidence that APEX1 can regulate the ferroptosis pathway. The bioinformatics analysis proved that the mRNA level of APEX1 was decreased in HepG2 cells and HCC38 cells in ferroptosis induced by erastin (Figures 4A, B). Through analysis of existing literature, it can be concluded that APEX1 must be involved in ferroptosis through its antioxidant domain. There is an interesting phenomenon: the expression of APEX1 in tumor cells is increased compared to the non-tumor regions, that can resist ROS induced apoptosis. However, the DNA repair function of APEX1 was masked by tumor cells. Therefore, it is worthwhile to explore the role of APEX1 in regulating ferroptosis, especially in the area of cancer. In summary, APEX1 is a multifunctional protein with both important DNA repair and redox capabilities. APEX1 plays key roles in regulation of the ferroptosis pathway, and it is an important potential target for the treatment of various ferroptosis related diseases. We hope that in the future, APEX1 can be used to treat patients and help in improving the condition of the patients suffering from various types of diseases.
Conclusion
In this review, we highlighted the potential roles of APEX1 in the regulation of ferroptosis. We found that APEX1 plays a vital role in regulating the pathways like system XC-, lipid peroxidation, Nrf2, HIF, and p53. In other words, APEX1 has the potential to regulate cancer, nervous system diseases, and other diseases through ferroptosis. Bioinformatics analysis revealed that the mRNA level of APEX1 was decreased in ferroptosis induced by erastin. APEX1 correlated with genes in the ferroptosis pathway. Therefore, we have put forward such a panoramic view of APEX1 acting as an inhibitor of ferroptosis.
Author Contributions
The authors declare no competing financial interests. XL and FZ were responsible for the study concept and design. NG, YZ, YD, and YC drafted the manuscript. XL and FZ provided a critical revision of the manuscript for important intellectual content. All authors contributed to the article and approved the submitted version.
Funding
This study was financially supported by grants from the Science and Technology Strategic Cooperation Project of the Luzhou People’s Government and Southwest Medical University (No. 2019LZXNYDJ34, 2019LZXNYDJ17, and 2020-JYJ-44) and the undergraduate innovation and entrepreneurship training program (S202110632241). This study was financially supported by school level scientific research project of Southwest Medical University (2021ZKQN008).
Conflict of Interest
The authors declare that the research was conducted in the absence of any commercial or financial relationships that could be construed as a potential conflict of interest.
Publisher’s Note
All claims expressed in this article are solely those of the authors and do not necessarily represent those of their affiliated organizations, or those of the publisher, the editors and the reviewers. Any product that may be evaluated in this article, or claim that may be made by its manufacturer, is not guaranteed or endorsed by the publisher.
References
1. D’Arcy MS. Cell Death: A Review of the Major Forms of Apoptosis, Necrosis and Autophagy. Cell Biol Int (2019) 43(6):582–92. doi: 10.1002/cbin.11137
2. Chen Q, Kang J, Fu C. The Independence of and Associations Among Apoptosis, Autophagy, and Necrosis. Signal Transduct Target Ther (2018) 3:18. doi: 10.1038/s41392-018-0018-5
3. Del Re DP, Amgalan D, Linkermann A, Liu Q, Kitsis RN. Fundamental Mechanisms of Regulated Cell Death and Implications for Heart Disease. Physiol Rev (2019) 99(4):1765–817. doi: 10.1152/physrev.00022.2018
4. Dixon SJ, Lemberg KM, Lamprecht MR, Skouta R, Zaitsev EM, Gleason CE, et al. Ferroptosis: An Iron-Dependent Form of Nonapoptotic Cell Death. Cell (2012) 149(5):1060–72. doi: 10.1016/j.cell.2012.03.042
5. Xie Y, Hou W, Song X, Yu Y, Huang J, Sun X, et al. Ferroptosis: Process and Function. Cell Death Differ (2016) 23(3):369–79. doi: 10.1038/cdd.2015.158
6. Kajarabille N, Latunde-Dada GO. Programmed Cell-Death by Ferroptosis: Antioxidants as Mitigators. Int J Mol Sci (2019) 20(19). doi: 10.3390/ijms20194968
7. Zhang P, Chen L, Zhao Q, Du X, Bi M, Li Y, et al. Ferroptosis was More Initial in Cell Death Caused by Iron Overload and Its Underlying Mechanism in Parkinson’s Disease. Free Radic Biol Med (2020) 152:227–34. doi: 10.1016/j.freeradbiomed.2020.03.015
8. Jing X, Du T, Chen K, Guo J, Xiang W, Yao X, et al. Icariin Protects Against Iron Overload-Induced Bone Loss via Suppressing Oxidative Stress. J Cell Physiol (2019) 234(7):10123–37. doi: 10.1002/jcp.27678
9. Gordan R, Wongjaikam S, Gwathmey JK, Chattipakorn N, Chattipakorn SC, Xie LH. Involvement of Cytosolic and Mitochondrial Iron in Iron Overload Cardiomyopathy: An Update. Heart Fail Rev (2018) 23(5):801–16. doi: 10.1007/s10741-018-9700-5
10. Liu N, Lin X, Huang C. Activation of the Reverse Transsulfuration Pathway Through NRF2/CBS Confers Erastin-Induced Ferroptosis Resistance. Br J Cancer (2020) 122(2):279–92. doi: 10.1038/s41416-019-0660-x
11. Forcina GC, Dixon SJ. GPX4 at the Crossroads of Lipid Homeostasis and Ferroptosis. Proteomics (2019) 19(18):e1800311. doi: 10.1002/pmic.201800311
12. Maiorino M, Conrad M, Ursini F. GPx4, Lipid Peroxidation, and Cell Death: Discoveries, Rediscoveries, and Open Issues. Antioxid Redox Signal (2018) 29(1):61–74. doi: 10.1089/ars.2017.7115
13. Hu CL, Nydes M, Shanley KL, Morales Pantoja IE, Howard TA, Bizzozero OA. Reduced Expression of the Ferroptosis Inhibitor Glutathione Peroxidase-4 in Multiple Sclerosis and Experimental Autoimmune Encephalomyelitis. J Neurochem (2019) 148(3):426–39. doi: 10.1111/jnc.14604
14. Gai C, Yu M, Li Z, Wang Y, Ding D, Zheng J, et al. Acetaminophen Sensitizing Erastin-Induced Ferroptosis via Modulation of Nrf2/heme Oxygenase-1 Signaling Pathway in Non-Small-Cell Lung Cancer. J Cell Physiol (2020) 235(4):3329–39. doi: 10.1002/jcp.29221
15. Yang WS, SriRamaratnam R, Welsch ME, Shimada K, Skouta R, Viswanathan VS, et al. Regulation of Ferroptotic Cancer Cell Death by GPX4. Cell (2014) 156(1-2):317–31. doi: 10.1016/j.cell.2013.12.010
16. Li Q, Han X, Lan X, Gao Y, Wan J, Durham F, et al. Inhibition of Neuronal Ferroptosis Protects Hemorrhagic Brain. JCI Insight (2017) 2(7):e90777. doi: 10.1172/jci.insight.90777
17. Abdalkader M, Lampinen R, Kanninen KM, Malm TM, Liddell JR. Targeting Nrf2 to Suppress Ferroptosis and Mitochondrial Dysfunction in Neurodegeneration. Front Neurosci (2018) 12:466. doi: 10.3389/fnins.2018.00466
18. Bhakat KK, Mantha AK, Mitra S. Transcriptional Regulatory Functions of Mammalian AP-Endonuclease (APE1/Ref-1), an Essential Multifunctional Protein. Antioxid Redox Signal (2009) 11(3):621–38. doi: 10.1089/ARS.2008.2198
19. den Hartog G, Chattopadhyay R, Ablack A, Hall EH, Butcher LD, Bhattacharyya A, et al. Regulation of Rac1 and Reactive Oxygen Species Production in Response to Infection of Gastrointestinal Epithelia. PloS Pathog (2016) 12(1):e1005382. doi: 10.1371/journal.ppat.1005382
20. Kang B, Mu S, Yang Q, Guo S, Chen X, Guo H. Ape1 Protects Against MPP+-Induced Neurotoxicity Through ERK1/2 Signaling in PC12 Cells. Neuroreport (2017) 28(1):10–6. doi: 10.1097/WNR.0000000000000712
21. McNeill DR, Narayana A, Wong HK, Wilson DM 3rd. Inhibition of Ape1 Nuclease Activity by Lead, Iron, and Cadmium. Environ Health Perspect (2004) 112(7):799–804. doi: 10.1289/ehp.7038
22. Demple B, Herman T, Chen DS. Cloning and Expression of APE, the cDNA Encoding the Major Human Apurinic Endonuclease: Definition of a Family of DNA Repair Enzymes. Proc Natl Acad Sci USA (1991) 88(24):11450–4. doi: 10.1073/pnas.88.24.11450
23. Robson CN, Hickson ID. Isolation of cDNA Clones Encoding a Human Apurinic/Apyrimidinic Endonuclease That Corrects DNA Repair and Mutagenesis Defects in E. Coli Xth (Exonuclease III) Mutants. Nucleic Acids Res (1991) 19(20):5519–23. doi: 10.1093/nar/19.20.5519
24. Kelley MR, Georgiadis MM, Fishel ML. APE1/Ref-1 Role in Redox Signaling: Translational Applications of Targeting the Redox Function of the DNA Repair/Redox Protein APE1/Ref-1. Curr Mol Pharmacol (2012) 5(1):36–53. doi: 10.2174/1874467211205010036
25. Stetler RA, Gao Y, Leak RK, Weng Z, Shi Y, Zhang L, et al. APE1/Ref-1 Facilitates Recovery of Gray and White Matter and Neurological Function After Mild Stroke Injury. Proc Natl Acad Sci USA (2016) 113(25):E3558–67. doi: 10.1073/pnas.1606226113
26. Mantha AK, Dhiman M, Taglialatela G, Perez-Polo RJ, Mitra S. Proteomic Study of Amyloid Beta (25-35) Peptide Exposure to Neuronal Cells: Impact on APE1/Ref-1’s Protein-Protein Interaction. J Neurosci Res (2012) 90(6):1230–9. doi: 10.1002/jnr.23018
27. Ma X, Dang C, Min W, Diao Y, Hui W, Wang X, et al. Downregulation of APE1 Potentiates Breast Cancer Cells to Olaparib by Inhibiting PARP-1 Expression. Breast Cancer Res Treat (2019) 176(1):109–17. doi: 10.1007/s10549-019-05189-w
28. Badgley MA, Kremer DM, Maurer HC, DelGiorno KE, Lee HJ, Purohit V, et al. Cysteine Depletion Induces Pancreatic Tumor Ferroptosis in Mice. Science (2020) 368(6486):85–9. doi: 10.1126/science.aaw9872
29. Hirschhorn T, Stockwell BR. The Development of the Concept of Ferroptosis. Free Radic Biol Med (2019) 133:130–43. doi: 10.1016/j.freeradbiomed.2018.09.043
30. Ingold I, Berndt C, Schmitt S, Doll S, Poschmann G, Buday K, et al. Selenium Utilization by GPX4 Is Required to Prevent Hydroperoxide-Induced Ferroptosis. Cell (2018) 172(3):409–22.e21. doi: 10.1016/j.cell.2017.11.048
31. Hao J, Du H, Liu F, Lu JC, Yang XC, Cui W. Apurinic/apyrimidinic Endonuclease/Redox Factor 1 (APE1) Alleviates Myocardial Hypoxia-Reoxygenation Injury by Inhibiting Oxidative Stress and Ameliorating Mitochondrial Dysfunction. Exp Ther Med (2019) 17(3):2143–51. doi: 10.3892/etm.2019.7212
32. Yoo DG, Song YJ, Cho EJ, Lee SK, Park JB, Yu JH, et al. Alteration of APE1/ref-1 Expression in Non-Small Cell Lung Cancer: The Implications of Impaired Extracellular Superoxide Dismutase and Catalase Antioxidant Systems. Lung Cancer (2008) 60(2):277–84. doi: 10.1016/j.lungcan.2007.10.015
33. Tudek B. Base Excision Repair Modulation as a Risk Factor for Human Cancers. Mol Aspects Med (2007) 28(3-4):258–75. doi: 10.1016/j.mam.2007.05.003
34. Zhang H, Ba S, Yang Z, Wang T, Lee JY, Li T, et al. Graphene Quantum Dot-Based Nanocomposites for Diagnosing Cancer Biomarker APE1 in Living Cells. ACS Appl Mater Interfaces (2020) 12(12):13634–43. doi: 10.1021/acsami.9b21385
35. Tell G, Damante G, Caldwell D, Kelley MR. The Intracellular Localization of APE1/Ref-1: More Than a Passive Phenomenon? Antioxid Redox Signal (2005) 7(3-4):367–84. doi: 10.1089/ars.2005.7.367
36. Al-Attar A, Gossage L, Fareed KR, Shehata M, Mohammed M, Zaitoun AM, et al. Human Apurinic/Apyrimidinic Endonuclease (APE1) Is a Prognostic Factor in Ovarian, Gastro-Oesophageal and Pancreatico-Biliary Cancers. Br J Cancer (2010) 102(4):704–9. doi: 10.1038/sj.bjc.6605541
37. Lu J, Holmgren A. The Thioredoxin Antioxidant System. Free Radic Biol Med (2014) 66:75–87. doi: 10.1016/j.freeradbiomed.2013.07.036
38. Hirota K, Matsui M, Iwata S, Nishiyama A, Mori K, Yodoi J. AP-1 Transcriptional Activity Is Regulated by a Direct Association Between Thioredoxin and Ref-1. Proc Natl Acad Sci USA (1997) 94(8):3633–8. doi: 10.1073/pnas.94.8.3633
39. Lukosz M, Jakob S, Buchner N, Zschauer TC, Altschmied J, Haendeler J. Nuclear Redox Signaling. Antioxid Redox Signal (2010) 12(6):713–42. doi: 10.1089/ars.2009.2609
40. Wei SJ, Botero A, Hirota K, Bradbury CM, Markovina S, Laszlo A, et al. Thioredoxin Nuclear Translocation and Interaction With Redox Factor-1 Activates the Activator Protein-1 Transcription Factor in Response to Ionizing Radiation. Cancer Res (2000) 60(23):6688–95.
41. Ando K, Hirao S, Kabe Y, Ogura Y, Sato I, Yamaguchi Y, et al. A New APE1/Ref-1-Dependent Pathway Leading to Reduction of NF-kappaB and AP-1, and Activation of Their DNA-Binding Activity. Nucleic Acids Res (2008) 36(13):4327–36. doi: 10.1093/nar/gkn416
42. Shibuki H, Katai N, Yodoi J, Uchida K, Yoshimura N. Lipid Peroxidation and Peroxynitrite in Retinal Ischemia-Reperfusion Injury. Invest Ophthalmol Vis Sci (2000) 41(11):3607–14.
43. Fukuse T, Hirata T, Yokomise H, Hasegawa S, Inui K, Mitsui A, et al. Attenuation of Ischaemia Reperfusion Injury by Human Thioredoxin. Thorax (1995) 50(4):387–91. doi: 10.1136/thx.50.4.387
44. Llabani E, Hicklin RW, Lee HY, Motika SE, Crawford LA, Weerapana E, et al. Diverse Compounds From Pleuromutilin Lead to a Thioredoxin Inhibitor and Inducer of Ferroptosis. Nat Chem (2019) 11(6):521–32. doi: 10.1038/s41557-019-0261-6
45. Bai L, Yan F, Deng R, Gu R, Zhang X, Bai J. Thioredoxin-1 Rescues MPP(+)/MPTP-Induced Ferroptosis by Increasing Glutathione Peroxidase 4. Mol Neurobiol (2021) 58(7):3187–97. doi: 10.1007/s12035-021-02320-1
46. Bridges RJ, Natale NR, Patel SA. System Xc(-) Cystine/Glutamate Antiporter: An Update on Molecular Pharmacology and Roles Within the CNS. Br J Pharmacol (2012) 165(1):20–34. doi: 10.1111/j.1476-5381.2011.01480.x
47. Cao X, Hou J, An Q, Assaraf YG, Wang X. Towards the Overcoming of Anticancer Drug Resistance Mediated by P53 Mutations. Drug Resist Update (2020) 49:100671. doi: 10.1016/j.drup.2019.100671
48. Liu Y, Zhang Z, Li Q, Zhang L, Cheng Y, Zhong Z. Mitochondrial APE1 Promotes Cisplatin Resistance by Downregulating ROS in Osteosarcoma. Oncol Rep (2020) 44(2):499–508. doi: 10.3892/or.2020.7633
49. Guo J, Xu B, Han Q, Zhou H, Xia Y, Gong C, et al. Ferroptosis: A Novel Anti-Tumor Action for Cisplatin. Cancer Res Treat (2018) 50(2):445–60. doi: 10.4143/crt.2016.572
50. Roh JL, Kim EH, Jang H, Shin D. Nrf2 Inhibition Reverses the Resistance of Cisplatin-Resistant Head and Neck Cancer Cells to Artesunate-Induced Ferroptosis. Redox Biol (2017) 11:254–62. doi: 10.1016/j.redox.2016.12.010
51. Deng F, Sharma I, Dai Y, Yang M, Kanwar YS. Myo-Inositol Oxygenase Expression Profile Modulates Pathogenic Ferroptosis in the Renal Proximal Tubule. J Clin Invest (2019) 129(11):5033–49. doi: 10.1172/JCI129903
52. Sasada T, Nakamura H, Ueda S, Iwata S, Ueno M, Takabayashi A, et al. Secretion of Thioredoxin Enhances Cellular Resistance to Cis-Diamminedichloroplatinum (II). Antioxid Redox Signal (2000) 2(4):695–705. doi: 10.1089/ars.2000.2.4-695
53. Jiang Y, Guo C, Vasko MR, Kelley MR. Implications of Apurinic/Apyrimidinic Endonuclease in Reactive Oxygen Signaling Response After Cisplatin Treatment of Dorsal Root Ganglion Neurons. Cancer Res (2008) 68(15):6425–34. doi: 10.1158/0008-5472.CAN-08-1173
54. Sies H, Berndt C, Jones DP. Oxidative Stress. Annu Rev Biochem (2017) 86:715–48. doi: 10.1146/annurev-biochem-061516-045037
55. Fan Z, Wirth AK, Chen D, Wruck CJ, Rauh M, Buchfelder M, et al. Nrf2-Keap1 Pathway Promotes Cell Proliferation and Diminishes Ferroptosis. Oncogenesis (2017) 6(8):e371. doi: 10.1038/oncsis.2017.65
56. Shin D, Kim EH, Lee J, Roh JL. Nrf2 Inhibition Reverses Resistance to GPX4 Inhibitor-Induced Ferroptosis in Head and Neck Cancer. Free Radic Biol Med (2018) 129:454–62. doi: 10.1016/j.freeradbiomed.2018.10.426
57. Ma CS, Lv QM, Zhang KR, Tang YB, Zhang YF, Shen Y, et al. NRF2-GPX4/SOD2 Axis Imparts Resistance to EGFR-Tyrosine Kinase Inhibitors in Non-Small-Cell Lung Cancer Cells. Acta Pharmacol Sin (2020) 42(4):613–23. doi: 10.1038/s41401-020-0443-1
58. Bai T, Lei P, Zhou H, Liang R, Zhu R, Wang W, et al. Sigma-1 Receptor Protects Against Ferroptosis in Hepatocellular Carcinoma Cells. J Cell Mol Med (2019) 23(11):7349–59. doi: 10.1111/jcmm.14594
59. Thakur S, Sarkar B, Dhiman M, Mantha AK. Organophosphate-Pesticides Induced Survival Mechanisms and APE1-Mediated Nrf2 Regulation in Non-Small-Cell Lung Cancer Cells. J Biochem Mol Toxicol (2021) 35(2):e22640. doi: 10.1002/jbt.22640
60. Sriramajayam K, Peng D, Lu H, Zhou S, Bhat N, McDonald OG, et al. Activation of NRF2 by APE1/REF1 Is Redox-Dependent in Barrett’s Related Esophageal Adenocarcinoma Cells. Redox Biol (2021) 43:101970. doi: 10.1016/j.redox.2021.101970
61. Sarkar B, Dhiman M, Mittal S, Mantha AK. Curcumin Revitalizes Amyloid Beta (25-35)-Induced and Organophosphate Pesticides Pestered Neurotoxicity in SH-SY5Y and IMR-32 Cells via Activation of APE1 and Nrf2. Metab Brain Dis (2017) 32(6):2045–61. doi: 10.1007/s11011-017-0093-2
62. Kang R, Kroemer G, Tang D. The Tumor Suppressor Protein P53 and the Ferroptosis Network. Free Radic Biol Med (2019) 133:162–8. doi: 10.1016/j.freeradbiomed.2018.05.074
63. Tarangelo A, Magtanong L, Bieging-Rolett KT, Li Y, Ye J, Attardi LD, et al. P53 Suppresses Metabolic Stress-Induced Ferroptosis in Cancer Cells. Cell Rep (2018) 22(3):569–75. doi: 10.1016/j.celrep.2017.12.077
64. Xie Y, Zhu S, Song X, Sun X, Fan Y, Liu J, et al. The Tumor Suppressor P53 Limits Ferroptosis by Blocking DPP4 Activity. Cell Rep (2017) 20(7):1692–704. doi: 10.1016/j.celrep.2017.07.055
65. Chu B, Kon N, Chen D, Li T, Liu T, Jiang L, et al. ALOX12 Is Required for P53-Mediated Tumour Suppression Through a Distinct Ferroptosis Pathway. Nat Cell Biol (2019) 21(5):579–91. doi: 10.1038/s41556-019-0305-6
66. Jiang L, Kon N, Li T, Wang SJ, Su T, Hibshoosh H, et al. Ferroptosis as a P53-Mediated Activity During Tumour Suppression. Nature (2015) 520(7545):57–62. doi: 10.1038/nature14344
67. Berkers CR, Maddocks OD, Cheung EC, Mor I, Vousden KH. Metabolic Regulation by P53 Family Members. Cell Metab (2013) 18(5):617–33. doi: 10.1016/j.cmet.2013.06.019
68. Ou Y, Wang SJ, Li D, Chu B, Gu W. Activation of SAT1 Engages Polyamine Metabolism With P53-Mediated Ferroptotic Responses. Proc Natl Acad Sci USA (2016) 113(44):E6806–E12. doi: 10.1073/pnas.1607152113
69. Shintoku R, Takigawa Y, Yamada K, Kubota C, Yoshimoto Y, Takeuchi T, et al. Lipoxygenase-Mediated Generation of Lipid Peroxides Enhances Ferroptosis Induced by Erastin and RSL3. Cancer Sci (2017) 108(11):2187–94. doi: 10.1111/cas.13380
70. Dixon SJ, Patel DN, Welsch M, Skouta R, Lee ED, Hayano M, et al. Pharmacological Inhibition of Cystine-Glutamate Exchange Induces Endoplasmic Reticulum Stress and Ferroptosis. Elife (2014) 3:e02523. doi: 10.7554/eLife.02523
71. Guan Z, Chen J, Li X, Dong N. Tanshinone IIA Induces Ferroptosis in Gastric Cancer Cells Through P53-Mediated SLC7A11 Down-Regulation. Biosci Rep (2020) 40(8). doi: 10.1042/BSR20201807
72. Gao M, Monian P, Quadri N, Ramasamy R, Jiang X. Glutaminolysis and Transferrin Regulate Ferroptosis. Mol Cell (2015) 59(2):298–308. doi: 10.1016/j.molcel.2015.06.011
73. Zhu J, Zhang C, Qing Y, Cheng Y, Jiang X, Li M, et al. Genistein Induces Apoptosis by Stabilizing Intracellular P53 Protein Through an APE1-Mediated Pathway. Free Radic Biol Med (2015) 86:209–18. doi: 10.1016/j.freeradbiomed.2015.05.030
74. Shah F, Logsdon D, Messmann RA, Fehrenbacher JC, Fishel ML, Kelley MR. Exploiting the Ref-1-APE1 Node in Cancer Signaling and Other Diseases: From Bench to Clinic. NPJ Precis Oncol (2017) 1. doi: 10.1038/s41698-017-0023-0
75. Reuter S, Gupta SC, Chaturvedi MM, Aggarwal BB. Oxidative Stress, Inflammation, and Cancer: How Are They Linked? Free Radic Biol Med (2010) 49(11):1603–16. doi: 10.1016/j.freeradbiomed.2010.09.006
76. Visconti R, Grieco D. New Insights on Oxidative Stress in Cancer. Curr Opin Drug Discov Devel (2009) 12(2):240–5.
77. Albanese A, Daly LA, Mennerich D, Kietzmann T, See V. The Role of Hypoxia-Inducible Factor Post-Translational Modifications in Regulating Its Localisation, Stability, and Activity. Int J Mol Sci (2020) 22(1). doi: 10.3390/ijms22010268
78. Cui Y, Zhang Y, Zhao X, Shao L, Liu G, Sun C, et al. ACSL4 Exacerbates Ischemic Stroke by Promoting Ferroptosis-Induced Brain Injury and Neuroinflammation. Brain Behav Immun (2021) 93:312–21. doi: 10.1016/j.bbi.2021.01.003
79. Jiang Y, Mao C, Yang R, Yan B, Shi Y, Liu X, et al. EGLN1/c-Myc Induced Lymphoid-Specific Helicase Inhibits Ferroptosis Through Lipid Metabolic Gene Expression Changes. Theranostics (2017) 7(13):3293–305. doi: 10.7150/thno.19988
80. Speer RE, Karuppagounder SS, Basso M, Sleiman SF, Kumar A, Brand D, et al. Hypoxia-Inducible Factor Prolyl Hydroxylases as Targets for Neuroprotection by "Antioxidant" Metal Chelators: From Ferroptosis to Stroke. Free Radic Biol Med (2013) 62:26–36. doi: 10.1016/j.freeradbiomed.2013.01.026
81. Yang M, Chen P, Liu J, Zhu S, Kroemer G, Klionsky DJ, et al. Clockophagy Is a Novel Selective Autophagy Process Favoring Ferroptosis. Sci Adv (2019) 5(7):eaaw2238. doi: 10.1126/sciadv.aaw2238
82. Bai Y, Meng L, Han L, Jia Y, Zhao Y, Gao H, et al. Lipid Storage and Lipophagy Regulates Ferroptosis. Biochem Biophys Res Commun (2019) 508(4):997–1003. doi: 10.1016/j.bbrc.2018.12.039
83. Jiang L, Zeng H, Ni L, Qi L, Xu Y, Xia L, et al. HIF-1alpha Preconditioning Potentiates Antioxidant Activity in Ischemic Injury: The Role of Sequential Administration of Dihydrotanshinone I and Protocatechuic Aldehyde in Cardioprotection. Antioxid Redox Signal (2019) 31(3):227–42. doi: 10.1089/ars.2018.7624
84. Ji W, Wang L, He S, Yan L, Li T, Wang J, et al. Effects of Acute Hypoxia Exposure With Different Durations on Activation of Nrf2-ARE Pathway in Mouse Skeletal Muscle. PloS One (2018) 13(12):e0208474. doi: 10.1371/journal.pone.0208474
85. Otterbein LE, Soares MP, Yamashita K, Bach FH. Heme Oxygenase-1: Unleashing the Protective Properties of Heme. Trends Immunol (2003) 24(8):449–55. doi: 10.1016/s1471-4906(03)00181-9
86. Dong H, Qiang Z, Chai D, Peng J, Xia Y, Hu R, et al. Nrf2 Inhibits Ferroptosis and Protects Against Acute Lung Injury Due to Intestinal Ischemia Reperfusion via Regulating SLC7A11 and HO-1. Aging (Albany NY) (2020) 12(13):12943–59. doi: 10.18632/aging.103378
87. Tang Z, Ju Y, Dai X, Ni N, Liu Y, Zhang D, et al. HO-1-Mediated Ferroptosis as a Target for Protection Against Retinal Pigment Epithelium Degeneration. Redox Biol (2021) 43:101971. doi: 10.1016/j.redox.2021.101971
88. Feng X, Wang S, Sun Z, Dong H, Yu H, Huang M, et al. Ferroptosis Enhanced Diabetic Renal Tubular Injury via HIF-1alpha/HO-1 Pathway in Db/Db Mice. Front Endocrinol (Lausanne) (2021) 12:626390. doi: 10.3389/fendo.2021.626390
89. Kupershmidt L, Weinreb O, Amit T, Mandel S, Bar-Am O, Youdim MB. Novel Molecular Targets of the Neuroprotective/Neurorescue Multimodal Iron Chelating Drug M30 in the Mouse Brain. Neuroscience (2011) 189:345–58. doi: 10.1016/j.neuroscience.2011.03.040
90. Bianchi L, Tacchini L, Cairo G. HIF-1-Mediated Activation of Transferrin Receptor Gene Transcription by Iron Chelation. Nucleic Acids Res (1999) 27(21):4223–7. doi: 10.1093/nar/27.21.4223
91. Fishel ML, Kelley MR. The DNA Base Excision Repair Protein Ape1/Ref-1 as a Therapeutic and Chemopreventive Target. Mol Aspects Med (2007) 28(3-4):375–95. doi: 10.1016/j.mam.2007.04.005
92. Huang LE, Arany Z, Livingston DM, Bunn HF. Activation of Hypoxia-Inducible Transcription Factor Depends Primarily Upon Redox-Sensitive Stabilization of Its Alpha Subunit. J Biol Chem (1996) 271(50):32253–9. doi: 10.1074/jbc.271.50.32253
93. Logsdon DP, Grimard M, Luo M, Shahda S, Jiang Y, Tong Y, et al. Regulation of HIF1alpha Under Hypoxia by APE1/Ref-1 Impacts CA9 Expression: Dual Targeting in Patient-Derived 3d Pancreatic Cancer Models. Mol Cancer Ther (2016) 15(11):2722–32. doi: 10.1158/1535-7163.MCT-16-0253
94. Fishel ML, Jiang Y, Rajeshkumar NV, Scandura G, Sinn AL, He Y, et al. Impact of APE1/Ref-1 Redox Inhibition on Pancreatic Tumor Growth. Mol Cancer Ther (2011) 10(9):1698–708. doi: 10.1158/1535-7163.MCT-11-0107
95. Zou GM, Maitra A. Small-Molecule Inhibitor of the AP Endonuclease 1/REF-1 E3330 Inhibits Pancreatic Cancer Cell Growth and Migration. Mol Cancer Ther (2008) 7(7):2012–21. doi: 10.1158/1535-7163.MCT-08-0113
96. Liang C, Zhang X, Yang M, Dong X. Recent Progress in Ferroptosis Inducers for Cancer Therapy. Adv Mater (2019) 31(51):e1904197. doi: 10.1002/adma.201904197
97. Hu Z, Zhang H, Yang SK, Wu X, He D, Cao K, et al. Emerging Role of Ferroptosis in Acute Kidney Injury. Oxid Med Cell Longev (2019) 2019:8010614. doi: 10.1155/2019/8010614
98. Weiland A, Wang Y, Wu W, Lan X, Han X, Li Q, et al. Ferroptosis and Its Role in Diverse Brain Diseases. Mol Neurobiol (2019) 56(7):4880–93. doi: 10.1007/s12035-018-1403-3
99. Yang Y, Luo M, Zhang K, Zhang J, Gao T, Connell DO, et al. Nedd4 Ubiquitylates VDAC2/3 to Suppress Erastin-Induced Ferroptosis in Melanoma. Nat Commun (2020) 11(1):433. doi: 10.1038/s41467-020-14324-x
100. Yu M, Gai C, Li Z, Ding D, Zheng J, Zhang W, et al. Targeted Exosome-Encapsulated Erastin Induced Ferroptosis in Triple Negative Breast Cancer Cells. Cancer Sci (2019) 110(10):3173–82. doi: 10.1111/cas.14181
101. Hao S, Yu J, He W, Huang Q, Zhao Y, Liang B, et al. Cysteine Dioxygenase 1 Mediates Erastin-Induced Ferroptosis in Human Gastric Cancer Cells. Neoplasia (2017) 19(12):1022–32. doi: 10.1016/j.neo.2017.10.005
102. Zhou HH, Chen X, Cai LY, Nan XW, Chen JH, Chen XX, et al. Erastin Reverses ABCB1-Mediated Docetaxel Resistance in Ovarian Cancer. Front Oncol (2019) 9:1398. doi: 10.3389/fonc.2019.01398
103. Yu H, Yang C, Jian L, Guo S, Chen R, Li K, et al. Sulfasalazineinduced Ferroptosis in Breast Cancer Cells is Reduced by the Inhibitory Effect of Estrogen Receptor on the Transferrin Receptor. Oncol Rep (2019) 42(2):826–38. doi: 10.3892/or.2019.7189
104. Kim EH, Shin D, Lee J, Jung AR, Roh JL. CISD2 Inhibition Overcomes Resistance to Sulfasalazine-Induced Ferroptotic Cell Death in Head and Neck Cancer. Cancer Lett (2018) 432:180–90. doi: 10.1016/j.canlet.2018.06.018
105. Yamaguchi Y, Kasukabe T, Kumakura S. Piperlongumine Rapidly Induces the Death of Human Pancreatic Cancer Cells Mainly Through the Induction of Ferroptosis. Int J Oncol (2018) 52(3):1011–22. doi: 10.3892/ijo.2018.4259
106. Sui X, Zhang R, Liu S, Duan T, Zhai L, Zhang M, et al. RSL3 Drives Ferroptosis Through GPX4 Inactivation and ROS Production in Colorectal Cancer. Front Pharmacol (2018) 9:1371. doi: 10.3389/fphar.2018.01371
107. Park TJ, Park JH, Lee GS, Lee JY, Shin JH, Kim MW, et al. Quantitative Proteomic Analyses Reveal That GPX4 Downregulation During Myocardial Infarction Contributes to Ferroptosis in Cardiomyocytes. Cell Death Dis (2019) 10(11):835. doi: 10.1038/s41419-019-2061-8
108. Jaeschke H, Ramachandran A, Chao X, Ding WX. Emerging and Established Modes of Cell Death During Acetaminophen-Induced Liver Injury. Arch Toxicol (2019) 93(12):3491–502. doi: 10.1007/s00204-019-02597-1
109. Hassannia B, Wiernicki B, Ingold I, Qu F, Van Herck S, Tyurina YY, et al. Nano-Targeted Induction of Dual Ferroptotic Mechanisms Eradicates High-Risk Neuroblastoma. J Clin Invest (2018) 128(8):3341–55. doi: 10.1172/JCI99032
110. Kong Z, Liu R, Cheng Y. Artesunate Alleviates Liver Fibrosis by Regulating Ferroptosis Signaling Pathway. BioMed Pharmacother (2019) 109:2043–53. doi: 10.1016/j.biopha.2018.11.030
111. Li ZJ, Dai HQ, Huang XW, Feng J, Deng JH, Wang ZX, et al. Artesunate Synergizes With Sorafenib to Induce Ferroptosis in Hepatocellular Carcinoma. Acta Pharmacol Sin (2020) 42(2):301–10. doi: 10.1038/s41401-020-0478-3
112. Zhang H, Deng T, Liu R, Ning T, Yang H, Liu D, et al. CAF Secreted miR-522 Suppresses Ferroptosis and Promotes Acquired Chemo-Resistance in Gastric Cancer. Mol Cancer (2020) 19(1):43. doi: 10.1186/s12943-020-01168-8
113. Lu Q, Wang M, Gui Y, Hou Q, Gu M, Liang Y, et al. Rheb1 Protects Against Cisplatin-Induced Tubular Cell Death and Acute Kidney Injury via Maintaining Mitochondrial Homeostasis. Cell Death Dis (2020) 11(5):364. doi: 10.1038/s41419-020-2539-4
114. Yao X, Zhang Y, Hao J, Duan HQ, Zhao CX, Sun C, et al. Deferoxamine Promotes Recovery of Traumatic Spinal Cord Injury by Inhibiting Ferroptosis. Neural Regen Res (2019) 14(3):532–41. doi: 10.4103/1673-5374.245480
115. Yoshida M, Minagawa S, Araya J, Sakamoto T, Hara H, Tsubouchi K, et al. Involvement of Cigarette Smoke-Induced Epithelial Cell Ferroptosis in COPD Pathogenesis. Nat Commun (2019) 10(1):3145. doi: 10.1038/s41467-019-10991-7
116. Zhang Y, Fan BY, Pang YL, Shen WY, Wang X, Zhao CX, et al. Neuroprotective Effect of Deferoxamine on Erastininduced Ferroptosis in Primary Cortical Neurons. Neural Regener Res (2020) 15(8):1539–45. doi: 10.4103/1673-5374.274344
117. Li Z, Jiang L, Chew SH, Hirayama T, Sekido Y, Toyokuni S. Carbonic Anhydrase 9 Confers Resistance to Ferroptosis/Apoptosis in Malignant Mesothelioma Under Hypoxia. Redox Biol (2019) 26:101297. doi: 10.1016/j.redox.2019.101297
118. Song Z, Xiang X, Li J, Deng J, Fang Z, Zhang L, et al. Ruscogenin Induces Ferroptosis in Pancreatic Cancer Cells. Oncol Rep (2020) 43(2):516–24. doi: 10.3892/or.2019.7425
119. Liu P, Feng Y, Li H, Chen X, Wang G, Xu S, et al. Ferrostatin-1 Alleviates Lipopolysaccharide-Induced Acute Lung Injury via Inhibiting Ferroptosis. Cell Mol Biol Lett (2020) 25:10. doi: 10.1186/s11658-020-00205-0
120. Fang X, Wang H, Han D, Xie E, Yang X, Wei J, et al. Ferroptosis as a Target for Protection Against Cardiomyopathy. Proc Natl Acad Sci USA (2019) 116(7):2672–80. doi: 10.1073/pnas.1821022116
121. Ito K, Eguchi Y, Imagawa Y, Akai S, Mochizuki H, Tsujimoto Y. MPP+ Induces Necrostatin-1- and Ferrostatin-1-Sensitive Necrotic Death of Neuronal SH-SY5Y Cells. Cell Death Discov (2017) 3:17013. doi: 10.1038/cddiscovery.2017.13
122. Mao XY, Zhou HH, Jin WL. Ferroptosis Induction in Pentylenetetrazole Kindling and Pilocarpine-Induced Epileptic Seizures in Mice. Front Neurosci (2019) 13:721. doi: 10.3389/fnins.2019.00721
123. Chen X, Zhang B, Liu T, Feng M, Zhang Y, Zhang C, et al. Liproxstatin-1 Attenuates Morphine Tolerance Through Inhibiting Spinal Ferroptosis-Like Cell Death. ACS Chem Neurosci (2019) 10(12):4824–33. doi: 10.1021/acschemneuro.9b00539
124. Feng Y, Madungwe NB, Imam Aliagan AD, Tombo N, Bopassa JC. Liproxstatin-1 Protects the Mouse Myocardium Against Ischemia/Reperfusion Injury by Decreasing VDAC1 Levels and Restoring GPX4 Levels. Biochem Biophys Res Commun (2019) 520(3):606–11. doi: 10.1016/j.bbrc.2019.10.006
125. Friedmann Angeli JP, Schneider M, Proneth B, Tyurina YY, Tyurin VA, Hammond VJ, et al. Inactivation of the Ferroptosis Regulator Gpx4 Triggers Acute Renal Failure in Mice. Nat Cell Biol (2014) 16(12):1180–91. doi: 10.1038/ncb3064
126. Carlson BA, Tobe R, Yefremova E, Tsuji PA, Hoffmann VJ, Schweizer U, et al. Glutathione Peroxidase 4 and Vitamin E Cooperatively Prevent Hepatocellular Degeneration. Redox Biol (2016) 9:22–31. doi: 10.1016/j.redox.2016.05.003
127. Kang R, Zeng L, Zhu S, Xie Y, Liu J, Wen Q, et al. Lipid Peroxidation Drives Gasdermin D-Mediated Pyroptosis in Lethal Polymicrobial Sepsis. Cell Host Microbe (2018) 24(1):97–108 e4. doi: 10.1016/j.chom.2018.05.009
128. Hambright WS, Fonseca RS, Chen L, Na R, Ran Q. Ablation of Ferroptosis Regulator Glutathione Peroxidase 4 in Forebrain Neurons Promotes Cognitive Impairment and Neurodegeneration. Redox Biol (2017) 12:8–17. doi: 10.1016/j.redox.2017.01.021
129. Kenny EM, Fidan E, Yang Q, Anthonymuthu TS, New LA, Meyer EA, et al. Ferroptosis Contributes to Neuronal Death and Functional Outcome After Traumatic Brain Injury. Crit Care Med (2019) 47(3):410–8. doi: 10.1097/CCM.0000000000003555
130. Li Q, Li QQ, Jia JN, Sun QY, Zhou HH, Jin WL, et al. Baicalein Exerts Neuroprotective Effects in FeCl3-Induced Posttraumatic Epileptic Seizures via Suppressing Ferroptosis. Front Pharmacol (2019) 10:638. doi: 10.3389/fphar.2019.00638
131. Okazaki T, Chung U, Nishishita T, Ebisu S, Usuda S, Mishiro S, et al. A Redox Factor Protein, Ref1, Is Involved in Negative Gene Regulation by Extracellular Calcium. J Biol Chem (1994) 269(45):27855–62. doi: 10.1016/S0021-9258(18)46865-2
132. Sun Z, Zhu Y, Aminbuhe, Fan Q, Peng J, Zhang N. Differential Expression of APE1 in Hepatocellular Carcinoma and the Effects on Proliferation and Apoptosis of Cancer Cells. Biosci Trends (2018) 12(5):456–62. doi: 10.5582/bst.2018.01239
133. Tang W, Lin D, Chen M, Li Z, Zhang W, Hu W, et al. PTEN-Mediated Mitophagy and APE1 Overexpression Protects Against Cardiac Hypoxia/Reoxygenation Injury. In Vitro Cell Dev Biol Anim (2019) 55(9):741–8. doi: 10.1007/s11626-019-00389-6
134. Manguinhas R, Fernandes AS, Costa JG, Saraiva N, Camoes SP, Gil N, et al. Impact of the APE1 Redox Function Inhibitor E3330 in Non-Small Cell Lung Cancer Cells Exposed to Cisplatin: Increased Cytotoxicity and Impairment of Cell Migration and Invasion. Antioxid (Basel) (2020) 9(6):550. doi: 10.3390/antiox9060550
135. Mahoney-Sanchez L, Bouchaoui H, Ayton S, Devos D, Duce JA, Devedjian JC. Ferroptosis and Its Potential Role in the Physiopathology of Parkinson’s Disease. Prog Neurobiol (2021) 196:101890. doi: 10.1016/j.pneurobio.2020.101890
136. Tian Y, Lu J, Hao X, Li H, Zhang G, Liu X, et al. FTH1 Inhibits Ferroptosis Through Ferritinophagy in the 6-OHDA Model of Parkinson’s Disease. Neurotherapeutics (2020) 17(4):1796–812. doi: 10.1007/s13311-020-00929-z
137. Caston RA, Gampala S, Armstrong L, Messmann RA, Fishel ML, Kelley MR. The Multifunctional APE1 DNA Repair-Redox Signaling Protein as a Drug Target in Human Disease. Drug Discov Today (2021) 26(1):218–28. doi: 10.1016/j.drudis.2020.10.015
138. Xanthoudakis S, Curran T. Identification and Characterization of Ref-1, a Nuclear Protein That Facilitates AP-1 DNA-Binding Activity. EMBO J (1992) 11(2):653–65. doi: 10.1002/j.1460-2075.1992.tb05097.x
139. Cardoso AA, Jiang Y, Luo M, Reed AM, Shahda S, He Y, et al. APE1/Ref-1 Regulates STAT3 Transcriptional Activity and APE1/Ref-1-STAT3 Dual-Targeting Effectively Inhibits Pancreatic Cancer Cell Survival. PloS One (2012) 7(10):e47462. doi: 10.1371/journal.pone.0047462
140. Nishi T, Shimizu N, Hiramoto M, Sato I, Yamaguchi Y, Hasegawa M, et al. Spatial Redox Regulation of a Critical Cysteine Residue of NF-Kappa B In Vivo. J Biol Chem (2002) 277(46):44548–56. doi: 10.1074/jbc.M202970200
141. Zhang X, Du L, Qiao Y, Zhang X, Zheng W, Wu Q, et al. Ferroptosis is Governed by Differential Regulation of Transcription in Liver Cancer. Redox Biol (2019) 24:101211. doi: 10.1016/j.redox.2019.101211
Keywords: APEX1, ferroptosis, HIF, Nrf2, p53
Citation: Guo N, Chen Y, Zhang Y, Deng Y, Zeng F and Li X (2022) Potential Role of APEX1 During Ferroptosis. Front. Oncol. 12:798304. doi: 10.3389/fonc.2022.798304
Received: 20 October 2021; Accepted: 02 February 2022;
Published: 03 March 2022.
Edited by:
Harikumar K. B., Rajiv Gandhi Centre for Biotechnology, IndiaReviewed by:
Pradeep Reddy Cingaram, University of Miami, United StatesHongbao Yang, China Pharmaceutical University, China
Copyright © 2022 Guo, Chen, Zhang, Deng, Zeng and Li. This is an open-access article distributed under the terms of the Creative Commons Attribution License (CC BY). The use, distribution or reproduction in other forums is permitted, provided the original author(s) and the copyright owner(s) are credited and that the original publication in this journal is cited, in accordance with accepted academic practice. No use, distribution or reproduction is permitted which does not comply with these terms.
*Correspondence: Xiang Li, bGl4MjAwOUAxMjYuY29t; Fancai Zeng, emZjYWlAc3dtdS5lZHUuY24=
†These authors have contributed equally to this work