- 1Department of Geriatrics, The Third People’s Hospital of Hangzhou, Hangzhou, China
- 2Zhejiang Provincial Key Laboratory of Geriatrics, Department of Geriatrics, Zhejiang Hospital, Hangzhou, China
Background: Radioresistance is the major obstacle after cancer radiotherapy. The dysregulation of long non-coding RNAs (lncRNAs) was closely related the radioresistance response. This meta-analysis was aimed to interpret the relationship between lncRNAs and radiotherapy responses in different cancers.
Method: The studies were selected from databases including PubMed, ISI Web of Science, Embase, Google Scholar, PMC, and CNKI (China National Knowledge Infrastructure). The publication time was limited to before March 20, 2021. The hazard ratios (HRs) and 95% confidence interval were calculated with random-effects models. Subgroup analyses, sensitivity analyses, and publication bias were also conducted.
Result: Twenty-seven lncRNAs in 14 cancer types were investigated, in which 23 lncRNAs were upregulated and four lncRNAs were downregulated. Dysregulation of these lncRNAs were found to be related to radioresistance response. The pooled HR and 95% confidence interval for the combined up-regulated lncRNAs was 1.73 (95% CI=1.50-2.00; P< 0.01) and down-regulated lncRNAs was 2.09 (95% CI= 1.60-2.72; P< 0.01). The HR values of the subgroup analysis for glioma (HR= 2.22, 95% CI= 1.79-2.74; p< 0.01), non-small cell lung cancer (HR=1.48, 95% CI=1.18-1.85; P<0.01), nasopharyngeal carcinoma (HR=4.26; 95% CI= 1.58-11.46; P< 0.01), and breast cancer (HR=1.29; 95% CI= 1.08-1.54; P< 0.01) were obtained. Moreover, the expression of lncRNAs was significantly related to overall survival of patients no matter if the sample size was >50 or not. In addition, the HR values of the subgroup analysis for lncRNA H19 (HR=2.68; 95% CI= 1.92-3.74; P <0.01), lncRNA FAM201A (HR=2.15; 95% CI= 1.15-3.99; P <0.01), and lncRNA HOTAIR (HR=1.22; 95% CI= 0.98-1.54; P =0.08) were also obtained.
Conclusion: LncRNAs can induce cancer radioresistance by regulating cell death-related signaling pathways. Results indicated that lncRNAs, especially lncRNA H19, FAM201A, and HOTAIR, could be considered as a predictive theragnostic biomarker to evaluate radiotherapy response.
Introduction
Cancer is one of the dominant factors causing global death, and deaths caused by cancer have far exceeded those caused by infectious diseases such as human immunodeficiency virus (HIV) and tuberculosis (TB) (1). GLOBOCAN estimated that 19.3 million new cases of cancer and almost 10.0 million deaths from cancer occurred in 2020 (2). Worldwide, the cancer burden is expected to be 28.4 million cases in 2040, which is a 47% rise from 2020. Limited access to timely diagnosis and effective treatment led to disparities in cancer survival between different locations. Advances in surgery, chemotherapy, radiotherapy, as well as other newer targeted therapies, had significantly reduced morbidity and improved the overall survival of cancer patients over the past decades (3). Among these treatments, about one-half of cancer patients with persistent or recurrent tumors receive radiotherapy (RT) (4, 5). However, radioresistance, a major hurdle for the clinical cancer treatment, widely induced deterioration of cancer including invasion, metastasis, poor prognosis, and overall survival of cancer patients (6, 7). Radioresistance is involved in multiple biological changes, for example, evasion of apoptosis, altered DNA damage response, and enhanced DNA repair (8). As a result, patients with radioresistant characteristics always require higher doses of irradiation to obtain effective treatment, but this treatment manner would lead to more serious side effects (9). Therefore, it is essential to explore novel biological markers that lead to radioresistance or radiosensitivity to benefit the treatment of radioresistant cancer patients and improve the outcome of radiotherapy.
Recently, it has been demonstrated that the dysregulation of long non-coding RNAs (lncRNAs) after ionizing radiation (IR) was different between radioresistant and radiosensitive patients (10–14). LncRNAs, a series of transcripts of more than 200 nucleotides, are generally defined as no or limited protein-coding potential (15). Numerous studies have indicated that lncRNAs are involved in gene expression regulating, transcription modulation, post-transcription modulation, as well as epigenetic modification of biological process (16, 17). Moreover, lncRNAs also play a vital role in multiple signal transduction pathways in cancer progression and metastasis, including miRNA silencing, DNA damage, cell cycle control, and hormone-driven disease states (18). Currently, a series of functional lncRNAs have been identified as key factors to induce radioresistance response by regulating the expression of their target genes at transcriptional or post-transcriptional levels (19–21). For instance, lncRNACCAT2 and lncRNARpph1 as strategic components augmenting the radiation therapy in esophageal squamous cell carcinoma (22, 23). Besides, lncRNA-p21 was indicated to promote the radiotherapy sensitivity of gastric cancer (24). Moreover, many lncRNAs such as lncRNATP73-AS1 (25), lncRNAFAM201A (26), lncRNA01600 (27), lncRNANCK1-AS1 (28), lncRNAMAGI2-AS3 (29), and lncRNAAGAP2-AS1 (30) have also been proven to participate in radioresistance response during cancer treatment. In addition, a recent study also identified that lncRNA FAM133B-2 showed radiosensitivity response in nasopharyngeal cancer cells by targeting miR-34a-5p/CDK6 axis (31).
However, these previous studies only focused on one single lncRNA based on small sample size or one study center. The association between lncRNAs and cancer radioresistance is still poorly understood. Therefore, more functional lncRNAs related to the radioresistance or radiosensitivity response need to be explored based on multiple centers and big sample size. Meta-analysis pooled large amount of available data and provided more precise estimates. Therefore, this study was performed to provide novel insights into lncRNAs associated with radioresistance and radiosensitivity of various cancers by a systematic review and meta-analysis. Studies were selected based on the inclusion and exclusion criteria. The extracted HRs and the corresponding 95% CIs were calculated followed by sensitivity analyses, publication bias assessment, as well as subgroup analysis.
Materials and Methods
The present meta-analysis was guided by the Preferred Reporting Items for Systematic Reviews and Meta-analysis (PRISMA) guidelines (32).
Search Strategy
The topic related literature was searched by two authors independently through PubMed, PMC, ISI Web of Science, Embase, Google Scholar, Cochrane Library, the Chinese National Knowledge Infrastructure (CNKI), and Chinese Biomedical Literature Database (CBM). The studies were limited to English or Chinese language which published before June 30, 2021. The terms “long non-coding RNA,” “lncRNAs,” “radioresistance,” “radiosensitivity,” “cancer,” “tumors,” “neoplasm,” and “overall survival” were used to search the related studies. Articles in the references listed in the studies were also searched to avoid missing the relevant studies.
Inclusion and Exclusion Criteria
Inclusion and exclusion criteria were chosen as the following. Retrieved studies were included if they met the following characteristics: 1) human individuals; 2) the diagnosis of cancer disease was clinically confirmed according to the disease guideline; 3) the expression of lncRNAs and its related overall survival were evaluated; 4) lncRNAs were involved in radioresistance or radiosensitivity in cancer therapy; 5) case group size, control group size, HR value, and its related 95%CIs were provided; 6) the publication language was limited to Chinese or English.
Studies were excluded if they met the following characteristics: 1) cell line or animal studies, case reports, comments, abstract, or review articles; 2) not related to radioresistance or radiosensitivity and cancer; 3) repeated studies; 4) insufficient data to calculate the HR value; 5) studies not related to the topics.
Data Extraction and Quality Assessment
Two authors retrieved the following data independently from the included studies: first author, publication year, country, sample characteristic, sample source, type of cancer, involved lncRNAs, detection method, related therapy, the case and control size, and the HR value and its 95CIs. If the included studies didn’t provide detailed data, the HR value and its 95CIs would be calculated based on the overall survival curves. If there was any inconsistency, the third researcher checked and resolved.
For further assessment of the quality of the included studies, two of the authors independently evaluated studies using the Newcastle-Ottawa Scale (NOS) (33). Studies scoring less than 6 were excluded in the present meta-analysis. Disagreements were resolved by discussion.
Statistical Analysis
The hazard ratios (HRs) with 95% confidence intervals (CIs) of lncRNAs and patients in included studies were extracted, and then the HRs and 95% CI as the effect magnitude were calculated as the amount of the combined effect. Subgroup analysis was conducted according to cancers difference, case sample size >50 or not, and the different expression trend of lncRNAs. The heterogeneity across studies was assessed using the I2 statistic. When I2 value was 25%, 50%, and 75%, it was low, moderate, and high degrees of heterogeneity, respectively. The pooled effect was conducted by the random-effects model when I2 value was > 75%. Otherwise, a fixed-effects model was used. The Begg and Egger’s tests were used to assess potential publication bias. All statistical analyses were conducted by Stata software version 12.0 (Stata Corp LP, TX, USA). The statistical significance was considered as a P-value< 0.05.
Results
Characteristics and Quality of the Included Studies and Individuals
Figure 1 shows the flowchart of study selection and exclusion. Three hundred eleven studies were initially searched from PubMed (n = 492), and other databases (n = 1234). After removing duplicated studies, 634 articles were considered relevant to the topic. After full-text screening according to inclusion and exclusion criteria, some records were removed because they were case report, review, or comments. Finally, a total of 28 studies with lncRNAs expression involved in radiosensitivity or radioresistance during cancer therapy were included, which contained a total of 4986 cancer patients.
The characteristics of the studies and individuals included are presented in Table 1. The results of NOS quality score of the eligible studies ranged from 6 to 9, as also illustrated in Table 1. And then, the eligible articles were further reviewed and data extracted. The included studies in the current systematic review and meta-analysis were published between 2015 and 2020 and conducted in Canada and China. The sources of the malignant tumor included glioma (35, 36, 38, 41, 43), neck squamous carcinoma (HNSCC) (57), esophageal cancer (EC) (22), breast cancer (34, 42, 46, 47), colorectal cancer (39, 58), non-small cell lung cancer (NSCLC) (40, 45, 50–52), ovarian cancer (OC) (48), cardiac carcinoma (49), thyroid carcinoma (44), prostate cancer (54), and nasopharyngeal carcinoma (NPC) (37, 56). Sample sizes included in these studies varied from 31 to 955 between the studies. The frozen or formalin-fixed tissue samples were used in these eligible studies, and lncRNAs were detected by qPCR or qRT-PCR assay.
Systematic Review
In total, 27 lncRNAs in 14 cancer types were studied in our systematic review and meta-analysis. Twenty-three lncRNAs were upregulated and four were downregulated compared with healthy individuals. Higher expression of lncRNA01057 in glioma, lncRNA00520 in HNSCC, lncRNA CCAT2 in EC, lncRNA HOTAIR in breast cancer and colorectal cancer, lncRNA Rpph1 in EC, lncRNA FAM201A in NSCLC, lncRNA LINC02582 in breast cancer, lncRNA 00511 in breast cancer, lncRNA FAM83H-AS1 in OC, lncRNA H19 in cardiac carcinoma, lncRNA CYTOR in NSCLC, lncRNA 00483 in NSCLC, lncRNA UCA1 in prostate cancer, lncRNA PVT1 in NSCLC, lncRNA MALAT1 in NPC were associated with radioresistance. While lower expression of lncRNA TPTEP1, DRAIC in glioma, lncRNA NKILA in laryngeal cancer, and lncRNA MEG3 in thyroid carcinoma were correlated to radioresistance.
Then, we searched the target protein of these lncRNAs in RDPDB database (http://rbpdb.ccbr.utoronto.ca/index.php), and conducted the protein-protein interaction (PPI) network based on the STRING database (https://string-db.org/), which covered almost all functional interactions between the expressed proteins (59). Shown as Figure 2A, the 24 target proteins were uploaded into the STRING database for analysis. Eighteen nodes and 69 edges were contained in the network; in total, the average node degree is 7.67. Nodes represented the core targets and the extended targets, edges represented the connection between the genes, and the degree value represented the association intensity. The ELAVL1, SRSF1, PTBP1, and HNRNPA1 were the core protein in this PPI network based on degree ranking which was calculated by cytohubba (60). In addition, there was a co-expression relationship between ELAVL1, PTBP1, HNRNPA1, and SRSF proteins, shown as Figure 2B. These target genes involved in RNA splicing, mRNA processing, negative regulation of mRNA metabolic process, cellular response to fibroblast growth factors stimulus, and so on, which is shown in Figure 2C curved based on Metascape database (http://metascape.org/). Our results indicate that these lncRNAs play key roles in radioresistance during cancer therapy by involving these pathways.
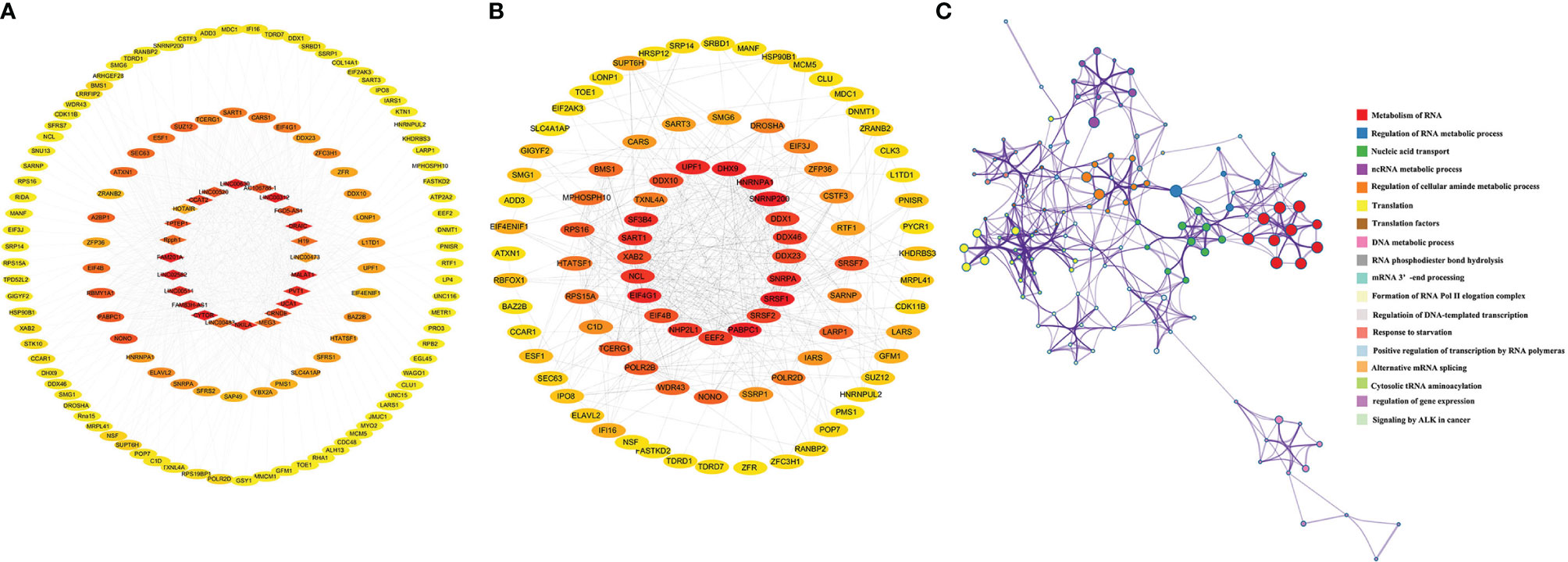
Figure 2 The interaction network of targets protein and gene function prediction of lncRNAs pathways involved in radiosensitivity. (A) The interaction network of targets protein of lncRNAs from STRING database (https://www.string-db.org/). (B) Coexpression scores based on RNA expression patterns, and on protein co-regulation provided by STRING. (C) Gene network of enriched terms by Metascape (https://metascape.org/), a subset of enriched terms has been selected and rendered as a network plot, where terms with a similarity > 0.3 are connected by edges. We select the terms with the best p-values from each of the 20 clusters, with the constraint that there are no more than 15 terms per cluster and no more than 250 terms in total, where each node represents an enriched term.
Meta-Analysis
Twenty-nine comparisons were included to evaluate the lncRNAs level response to radioresistance during cancer therapy in cancer patients; enrolling a total of 4986 subjects. Shown as Figure 3A, the pooled HR and 95% CIs for the combined up-regulated lncRNAs was 1.73 (95% CI=1.50-2.00; P< 0.01) under the random-effects model, and the pooled HR and 95% CIs for the combined down-regulated lncRNAs was 2.09 (95% CI =1.60-2.72; P<0.01). In addition, subgroup meta-analyses that focused on lncRNAs and the difference of cancers was performed, shown as Figure 3B. We found that the dysregulation of lncRNAs was significantly related to poor overall survival (OS) in glioma patients (HR= 2.22, 95% CI =1.79-2.74; P<0.01). Four studies investigated the association between lncRNAs expression and OS in patients with breast cancer, and results indicated that the up-regulated expression of lncRNAs could predict poor OS in patients with breast cancer (HR= 1.29, 95% CI =1.08-1.54; P<0.01). Five studies discussed the relationship between lncRNAs expression with OS in patients with NSCLC, which demonstrated that a higher expression of these five lncRNAs was related to poor OS in NSCLC patients (HR= 1.48, 95% CI =1.18-1.85; P<0.01). Two studies involved the relationship between lncRNAs expression with OS in EC patients. However, the results suggested that a higher expression of these two lncRNAs was not related to OS in EC patients (HR= 1.57, 95% CI =0.90-2.75; P=1.09) and colorectal cancer patients (HR= 1.30, 95% CI =0.49-3.43; P=0.602). Furthermore, subgroup meta-analyses focusing on the sample size was also performed. As shown in Figure 3C, the dysregulation of lncRNAs was significantly related to OS in cancer patients no matter if the case sample size was >50 or not. Shown as Figure 3D, two studies discussed the relationship between lncRNAFAM201A and the OS in cancer patients (HR=2.15; 95% CI= 1.15-3.99; P <0.01). Another two studies discussed the relationship between lncRNAH19 and the OS in cancer patients (HR=2.68; 95% CI= 1.92-3.74; P <0.01). However, there was no significant relationship between lncRNA HOTAIR and the OS in cancer patients (HR=1.22; 95% CI= 0.98-1.54; P =0.08).
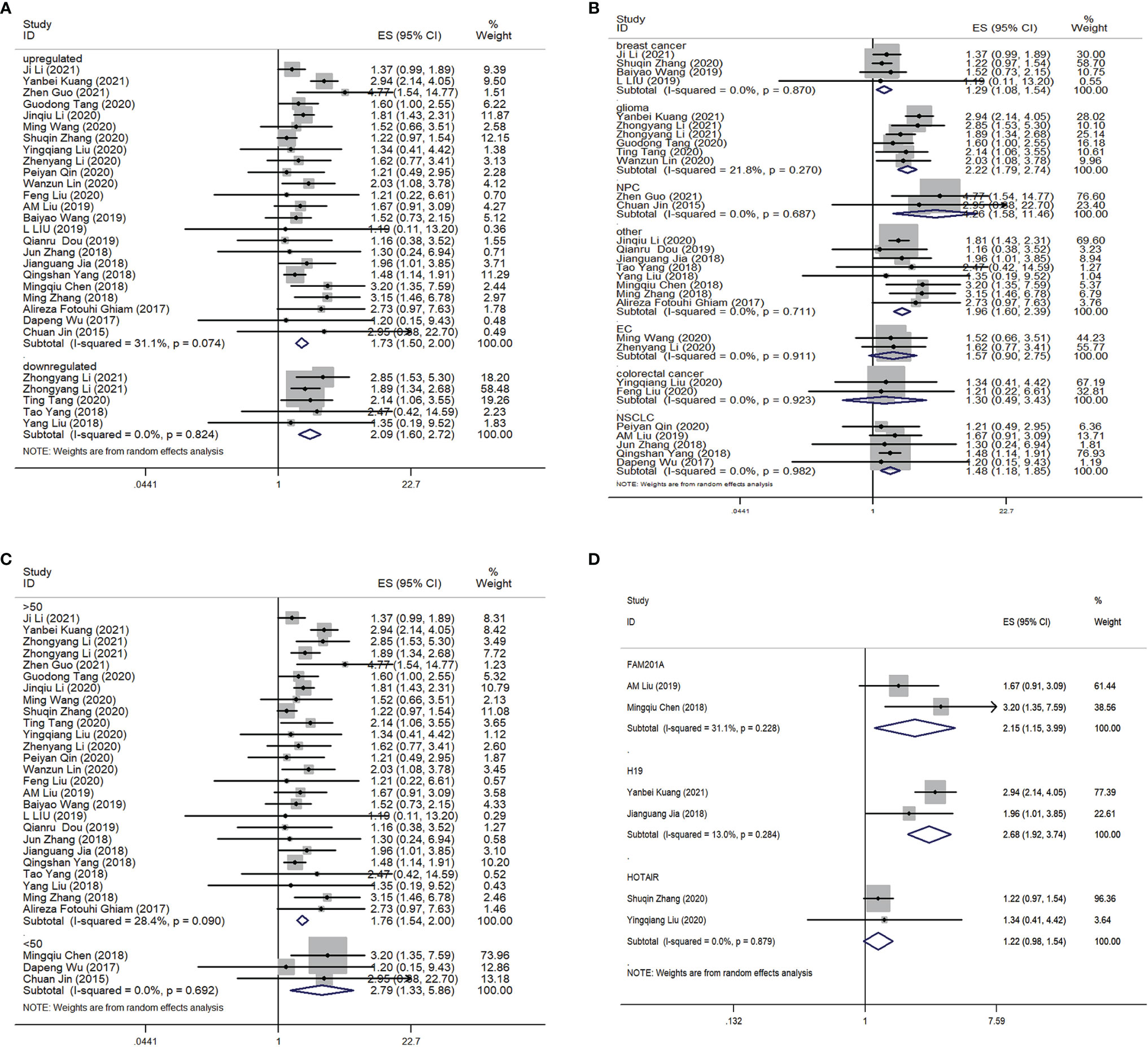
Figure 3 Forest plot for the association between the lncRNAs expression levels with OS. (A) Subgroup analysis of the association between lncRNAs expression level and cancer patients according to the up-regulation and down-regelation of lncRNAs. (B) Subgroup analysis of the association between lncRNAs expression level and cancer patients according to the difference of cancer types. (C) Subgroup analysis of the association between lncRNAs expression level and cancer patients according to the difference of samples size. (D) Subgroup analysis of the association between different lncRNAs expression level and the OS of cancer patients. OS, overall survival.
In the present meta-analysis, sensitivity analysis and publication bias were also performed. Egger’s test and Begg’s showed that there was no risk of publication bias in this meta-analysis, shown as Supplementary Figure S1.
Discussion
There is a growing investigation to demonstrate that lncRNAs play an important role in radioresistance or radiosensitivity. However, previous individual studies couldn’t provide strong evidence due to small sample size and different research standards. Therefore, we performed this present meta-analysis to evaluate the relationship between the expression level of lncRNAs and radioresistance or radiosensitivity in cancer patients. Here, we retrieved all publicly available lncRNAs and integrated data extracted from 19 related articles including 4986 cancer subjects. The results indicate that the upregulation or downregulation of lncRNAs are significantly related to radioresistance response in cancer patients. Down-regulation of TPTEP1, MEG3, and UCA1 and up-regulation of LINC01057, LINC00520, CCAT2, HOTAIR, Rpph1, FAM201A, LINC02582, LINC00511, FAM83H-AS1, H19, CYTOR, LINC00483, NKILA, PVT1, and MALAT1 are deeply involved in the radiotherapy of cancer patients. These findings are novel and provide insights into the potential biological markers in targeting radiotherapy response.
Traditionally, there are four kinds of biomarkers in cancer biology, including diagnostic biomarkers, prognostic biomarkers, diagnostic companion biomarkers, and predictive theragnostic biomarkers. The last biomarker had been used as an indicator of normal biological processes, pathogenic processes, or responses to an exposure or intervention (61). Consequently, exploring novel uses of each type of biomarker, especially predictive theragnostic biomarkers, is crucial to improving patient management and treatment outcome. However, radioresistance is a major factor that leads to poor prognosis for cancer patients. Radiotherapy always induced DNA damage or excessive ROS by ionizing radiation to activate apoptotic signaling pathways in cancer cells, leading to cell death (62). In addition, crucial molecules at cell cycle checkpoints could regulate and arrest cell cycle progression. Therefore, cell cycle arrest was closely related to radioresistance or radiosensitivity response. For example, down-regulation of metastasis-associated lung adenocarcinoma transcript 1 (MALAT1) could negatively regulate miR-145 levels and increase radiosensitivity, which may induce G2/M arrest and affect DNA repair (56). However, MALAT1 could also induce the radioresistance response during esophageal squamous cell carcinoma (ESCC) treatment. The reason was that MALAT1 decreased the expression of CDC kinase subunit 1 (CKS1) and increased the G2/M arrest response by inhibiting the expression of p27 (63). Similarly, the hox transcript antisense intergenic lncRNA (HOTAIR) increased radioresistance response by decreasing the level of p21 and inducing cell cycle arrest at the S phase (64). Furthermore, our meta-analysis revealed that overexpression of MALAT1 and HOTAIR contributes to increased radioresistance in NPC, colorectal cancer, and breast cancer patients. Except for DNA damage, apoptosis was the main mode of cancer cell death induced by radiotherapy (65). For example, HOTAIR acted as a key inhibitor to partly turn off the Wnt/β-catenin pathway in pancreatic ductal adenocarcinoma cells (66), and as a result, the radiosensitivity response was reduced. Increasing the level of urothelial carcinoma associated 1 (UCA1) induced the radioresistance response by increasing Akt activation in prostate cancer cells (54), which was consistent with the result from our meta-analysis. However, lncRNAs modulated gene expression through multiple mechanisms. For example, they could act as competing endogenous RNAs (ceRNAs) to prevent miRNA from interacting with shared mRNA, resulting a lncRNA-miRNA-mRNA regulatory network to affect the expression of downstream target (43). For example, lncRNA colon-cancer-associated transcript-1 (CCAT1) negatively regulated miR-148b expression (23) and 201-member A (FAM201A) (45) regulated ataxia telangiectasia mutation and mammalian target of rapamycin (mTOR) expression via miR-101 (26)to mediate the radiosensitivity of breast cancer and ESCC. In addition, the lncRNAs plasmacytoma variant translocation 1 (PVT1) acted as sponger of miR-195 to reduce radioresistance of NSCLC (55). Therefore, combined with our meta-analysis, we could draw a conclusion that all of these lncRNAs could act as potential predictive markers for radioresistance response.
In the present meta-analysis, tissue samples were used to assess lncRNAs expression in the included literature. However, lncRNAs from ncRNAs from serum or plasma samples allow for more collection, lower expense, and more convenient analysis. Therefore, there are several limitations in this meta-analysis. First, there were a small number of eligible studies, and some of the sample sizes included in these studies were < 50. Second, these eligible studies explored multiple kinds of cancers or tumors, so there were not enough studies to calculate the pooled HR value of one sole kind of cancer. Third, most studies were conducted in China and only one study was investigated in Caucasians. Therefore, the results may not interpret well to populations all over the world. Finally, most studies explored absolute differences in lncRNAs and HR values were applied for the current meta-analysis, which may lead to bias and decreased accuracy of the results. Further studies are necessary to be conducted with larger samples, multiple centers, populations, as well as more kinds of cancer.
Conclusion
In conclusion, this systematic review and meta-analysis explored the association between lncRNAs expression and radiotherapeutic response in various cancers. Furthermore, our meta-analysis provided a series of potential lncRNAs which could indicate patients at high risk of radresistance response; allowing doctors to improve their therapeutic strategies to overcome radresistance. Therefore, more work is needed to explore how lncRNAs interact with signaling molecules to induce radiosensitivity or radioresistance as well as to evaluate the therapeutic diagnosis value of lncRNAs in radiotherapy.
Data Availability Statement
The raw data supporting the conclusions of this article will be available by email the corresponding authors.
Author Contributions
PL and GM were responsible for the design of this work. WX and QR performed the database search and data collection. WX and QW was responsible for the data analysis. JY and PL drafted this article. All authors reviewed this draft and approved the final manuscript.
Funding
This study was supported by the project of Science and Technology plan of Hangzhou Municipal Health Bureau in 2018 (2018Z05); the projection of Zhejiang Health Commission (2018KY593); the project of Hangzhou Medical Key Discipline Construction; the Natural Science Foundation of Zhejiang Province (LQ20H250001), funds from the Health Bureau of Zhejiang Province (2019RC091, 2020ZB009), the project of Hangzhou technology plan (ZD20220005).
Conflict of Interest
The authors declare that the research was conducted in the absence of any commercial or financial relationships that could be construed as a potential conflict of interest.
Publisher’s Note
All claims expressed in this article are solely those of the authors and do not necessarily represent those of their affiliated organizations, or those of the publisher, the editors and the reviewers. Any product that may be evaluated in this article, or claim that may be made by its manufacturer, is not guaranteed or endorsed by the publisher.
Supplementary Material
The Supplementary Material for this article can be found online at: https://www.frontiersin.org/articles/10.3389/fonc.2022.767750/full#supplementary-material
References
1. Cortes J, Perez-Garcia JM, Llombart-Cussac A, Curigliano G, El Saghir NS, Cardoso F, et al. Enhancing Global Access to Cancer Medicines. CA Cancer J Clin (2020) 70(2):105–24. doi: 10.3322/caac.21597
2. Sung H, Ferlay J, Siegel RL, Laversanne M, Soerjomataram I, Jemal A, et al. Global Cancer Statistics 2020: GLOBOCAN Estimates of Incidence and Mortality Worldwide for 36 Cancers in 185 Countries. CA Cancer J Clin (2021) 71(3):209–49. doi: 10.3322/caac.21660
3. Mahvi DA, Liu R, Grinstaff MW, Colson YL, Raut CP. Local Cancer Recurrence: The Realities, Challenges, and Opportunities for New Therapies. CA Cancer J Clin (2018) 68(6):488–505. doi: 10.3322/caac.21498
4. Jaffray DA. Image-Guided Radiotherapy: From Current Concept to Future Perspectives. Nat Rev Clin Oncol (2012) 9(12):688–99. doi: 10.1038/nrclinonc.2012.194
5. Herrera FG, Bourhis J, Coukos G. Radiotherapy Combination Opportunities Leveraging Immunity for the Next Oncology Practice. CA Cancer J Clin (2017) 67(1):65–85. doi: 10.3322/caac.21358
6. Schaue D, McBride WH. Opportunities and Challenges of Radiotherapy for Treating Cancer. Nat Rev Clin Oncol (2015) 12(9):527–40. doi: 10.1038/nrclinonc.2015.120
7. Thariat J, Hannoun-Levi JM, Sun Myint A, Vuong T, Gerard JP. Past, Present, and Future of Radiotherapy for the Benefit of Patients. Nat Rev Clin Oncol (2013) 10(1):52–60. doi: 10.1038/nrclinonc.2012.203
8. Goldstein M, Kastan MB. The DNA Damage Response: Implications for Tumor Responses to Radiation and Chemotherapy. Annu Rev Med (2015) 66:129–43. doi: 10.1146/annurev-med-081313-121208
9. Podralska M, Ciesielska S, Kluiver J, van den Berg A, Dzikiewicz-Krawczyk A, Slezak-Prochazka I. Non-Coding RNAs in Cancer Radiosensitivity: MicroRNAs and lncRNAs as Regulators of Radiation-Induced Signaling Pathways. Cancers (Basel) (2020) 12(6):1662. doi: 10.3390/cancers12061662
10. Li G, Liu Y, Liu C, Su Z, Ren S, Wang Y, et al. Genome-Wide Analyses of Long Noncoding RNA Expression Profiles Correlated With Radioresistance in Nasopharyngeal Carcinoma via Next-Generation Deep Sequencing. BMC Cancer (2016) 16:719. doi: 10.1186/s12885-016-2755-6
11. Liu SJ, Malatesta M, Lien BV, Saha P, Thombare SS, Hong SJ, et al. CRISPRi-Based Radiation Modifier Screen Identifies Long non-Coding RNA Therapeutic Targets in Glioma. Genome Biol (2020) 21(1):83. doi: 10.1186/s13059-020-01995-4
12. Momeny M, Sankanian G, Hamzehlou S, Yousefi H, Esmaeili F, Alishahi Z, et al. Cediranib, an Inhibitor of Vascular Endothelial Growth Factor Receptor Kinases, Inhibits Proliferation and Invasion of Prostate Adenocarcinoma Cells. Eur J Pharmacol (2020) 882:173298. doi: 10.1016/j.ejphar.2020.173298
13. Zhou J, Cao S, Li W, Wei D, Wang Z, Li G, et al. Time-Course Differential lncRNA and mRNA Expressions in Radioresistant Hypopharyngeal Cancer Cells. Oncotarget (2017) 8(25):40994–1010. doi: 10.18632/oncotarget.17343
14. Friedrich M, Wiedemann K, Reiche K, Puppel SH, Pfeifer G, Zipfel I, et al. The Role of lncRNAs TAPIR-1 and -2 as Diagnostic Markers and Potential Therapeutic Targets in Prostate Cancer. Cancers (Basel) (2020) 12(5):1122. doi: 10.3390/cancers12051122
15. Kopp F, Mendell JT. Functional Classification and Experimental Dissection of Long Noncoding RNAs. Cell (2018) 172(3):393–407. doi: 10.1016/j.cell.2018.01.011
16. Chan JJ, Tay Y. Noncoding RNA:RNA Regulatory Networks in Cancer. Int J Mol Sci (2018) 19(5):1310. doi: 10.3390/ijms19051310
17. Wei CW, Luo T, Zou SS, Wu AS. The Role of Long Noncoding RNAs in Central Nervous System and Neurodegenerative Diseases. Front Behav Neurosci (2018) 12:175. doi: 10.3389/fnbeh.2018.00175
18. Sahu A, Singhal U, Chinnaiyan AM. Long Noncoding RNAs in Cancer: From Function to Translation. Trends Cancer (2015) 1(2):93–109. doi: 10.1016/j.trecan.2015.08.010
19. Bi Z, Li Q, Dinglin X, Xu Y, You K, Hong H, et al. Nanoparticles (NPs)-Meditated LncRNA AFAP1-AS1 Silencing to Block Wnt/beta-Catenin Signaling Pathway for Synergistic Reversal of Radioresistance and Effective Cancer Radiotherapy. Adv Sci (Weinh) (2020) 7(18):2000915. doi: 10.1002/advs.202000915
20. Jiang H, Hu X, Zhang H, Li W. Down-Regulation of LncRNA TUG1 Enhances Radiosensitivity in Bladder Cancer via Suppressing HMGB1 Expression. Radiat Oncol (2017) 12(1):65. doi: 10.1186/s13014-017-0802-3
21. Zhu J, Chen S, Yang B, Mao W, Yang X, Cai J. Molecular Mechanisms of lncRNAs in Regulating Cancer Cell Radiosensitivity. Biosci Rep (2019) 39(8):BSR20190590. doi: 10.1042/BSR20190590
22. Li ZY, Li HF, Zhang YY, Zhang XL, Wang B, Liu JT. Value of Long non-Coding RNA Rpph1 in Esophageal Cancer and its Effect on Cancer Cell Sensitivity to Radiotherapy. World J Gastroenterol (2020) 26(15):1775–91. doi: 10.3748/wjg.v26.i15.1775
23. Wang M, Wang L, He X, Zhang J, Zhu Z, Zhang M, et al. lncRNA CCAT2 Promotes Radiotherapy Resistance for Human Esophageal Carcinoma Cells via the Mir−145/P70s6k1 and P53 Pathway. Int J Oncol (2020) 56(1):327–36. doi: 10.3892/ijo.2019.4929
24. Chen L, Yuan D, Yang Y, Ren M. LincRNA-P21 Enhances the Sensitivity of Radiotherapy for Gastric Cancer by Targeting the Beta-Catenin Signaling Pathway. J Cell Biochem (2019) 120(4):6178–87. doi: 10.1002/jcb.27905
25. Song W, Zhang J, Xia Q, Sun M. Down-Regulated lncRNA TP73-AS1 Reduces Radioresistance in Hepatocellular Carcinoma via the PTEN/Akt Signaling Pathway. Cell Cycle (2019) 18(22):3177–88. doi: 10.1080/15384101.2019.1671089
26. Chen M, Liu P, Chen Y, Chen Z, Shen M, Liu X, et al. Long Noncoding RNA FAM201A Mediates the Radiosensitivity of Esophageal Squamous Cell Cancer by Regulating ATM and mTOR Expression via miR-101. Front Genet (2018) 9:611. doi: 10.3389/fgene.2018.00611
27. Xu M, Gong S, Li Y, Zhou J, Du J, Yang C, et al. Identifying Long Non-Coding RNA of Prostate Cancer Associated With Radioresponse by Comprehensive Bioinformatics Analysis. Front Oncol (2020) 10:498. doi: 10.3389/fonc.2020.00498
28. Hu H, Li H, Feng X. Downregulation of lncRNA NCK1-AS1 Inhibits Cancer Cell Migration and Invasion in Nasopharyngeal Carcinoma by Upregulating miR-135a. Cancer Manag Res (2019) 11:10531–7. doi: 10.2147/CMAR.S221326
29. Cheng W, Shi X, Lin M, Yao Q, Ma J, Li J. LncRNA MAGI2-AS3 Overexpression Sensitizes Esophageal Cancer Cells to Irradiation Through Down-Regulation of HOXB7 via EZH2. Front Cell Dev Biol (2020) 8:552822. doi: 10.3389/fcell.2020.552822
30. Zhang F, Sang Y, Chen D, Wu X, Wang X, Yang W, et al. M2 Macrophage-Derived Exosomal Long non-Coding RNA AGAP2-AS1 Enhances Radiotherapy Immunity in Lung Cancer by Reducing microRNA-296 and Elevating NOTCH2. Cell Death Dis (2021) 12(5):467. doi: 10.1038/s41419-021-03700-0
31. Huang D, Zhu X, Wang Y, Yu H, Pu Y. Long non-Coding RNA FAM133B-2 Represses the Radio-Resistance of Nasopharyngeal Cancer Cells by Targeting miR-34a-5p/CDK6 Axis. Aging (Albany NY) (2020) 12(17):16936–50. doi: 10.18632/aging.103600
32. Moher D, Liberati A, Tetzlaff J, Altman DG, Group P. Preferred Reporting Items for Systematic Reviews and Meta-Analyses: The PRISMA Statement. J Clin Epidemiol (2009) 62(10):1006–12. doi: 10.1016/j.jclinepi.2009.06.005
33. Stang A. Critical Evaluation of the Newcastle-Ottawa Scale for the Assessment of the Quality of Nonrandomized Studies in Meta-Analyses. Eur J Epidemiol (2010) 25(9):603–5. doi: 10.1007/s10654-010-9491-z
34. Li J, Lei C, Chen B, Zhu Q. LncRNA FGD5-AS1 Facilitates the Radioresistance of Breast Cancer Cells by Enhancing MACC1 Expression Through Competitively Sponging miR-497-5p. Front Oncol (2021) 11:671853. doi: 10.3389/fonc.2021.671853
35. Kuang Y, Bing Z. LncRNA H19 Upregulation Participates in the Response of Glioma Cells to Radiation. Biomed Res Int (2021) 2021:1728352. doi: 10.1155/2021/1728352
36. Li Z, Cai S, Li H, Gu J, Tian Y, Cao J, et al. Developing a lncRNA Signature to Predict the Radiotherapy Response of Lower-Grade Gliomas Using Co-Expression and ceRNA Network Analysis. BioMed Res Int (2021) 11:622880. doi: 10.3389/fonc.2021.622880
37. Guo Z, Wang YH, Xu H. LncRNA Linc00312 Suppresses Radiotherapy Resistance by Targeting DNA-PKcs and Impairing DNA Damage Repair in Nasopharyngeal Carcinoma. Cell Death Dis (2021) 12(1):69. doi: 10.1038/s41419-020-03302-2
38. Lin W, Huang Z, Xu Y, Chen X, Chen T, Ye Y, et al. A three-lncRNA Signature Predicts Clinical Outcomes in Low-Grade Glioma Patients After Radiotherapy. Aging (Albany NY) (2020) 12(10):9188–204. doi: 10.18632/aging.103189
39. Liu F, Huang W, Hong J, Cai C, Zhang W, Zhang J, et al. Long Noncoding RNA LINC00630 Promotes Radio-Resistance by Regulating BEX1 Gene Methylation in Colorectal Cancer Cells. IUBMB Life (2020) 72(7):1404–14. doi: 10.1002/iub.2263
40. Qin P, Li Y, Liu J, Wang N. Knockdown of LINC00473 Promotes Radiosensitivity of non-Small Cell Lung Cancer Cells via Sponging miR-513a-3p. Free Radic Res (2020) 54(10):756–64. doi: 10.1080/10715762.2020.1841900
41. Tang G, Luo L, Zhang J, Zhai D, Huang D, Yin J, et al. lncRNA LINC01057 Promotes Mesenchymal Differentiation by Activating NF-κb Signaling in Glioblastoma. Free Radic Res (2021) 498:152–64. doi: 10.1016/j.canlet.2020.10.047
42. Zhang S, Wang B, Xiao H, Dong J, Li Y, Zhu C, et al. LncRNA HOTAIR Enhances Breast Cancer Radioresistance Through Facilitating HSPA1A Expression via Sequestering miR-449b-5p. Thorac Cancer (2020) 11(7):1801–16. doi: 10.1111/1759-7714.13450
43. Tang T, Wang LX, Yang ML, Zhang RM. lncRNA TPTEP1 Inhibits Stemness and Radioresistance of Glioma Through Mir−106a−5p−Mediated P38 MAPK Signaling. Mol Med Rep (2020) 22(6):4857–67. doi: 10.3892/mmr.2020.11542
44. Liu Y, Yue P, Zhou T, Zhang F, Wang H, Chen X. LncRNA MEG3 Enhances (131)I Sensitivity in Thyroid Carcinoma via Sponging miR-182. BioMed Pharmacother (2018) 105:1232–9. doi: 10.1016/j.biopha.2018.06.087
45. Liu AM, Zhu Y, Huang ZW, Lei L, Fu SZ, Chen Y. Long Noncoding RNA FAM201A Involves in Radioresistance of non-Small-Cell Lung Cancer by Enhancing EGFR Expression via miR-370. Eur Rev Med Pharmacol Sci (2019) 23(13):5802–14. doi: 10.26355/eurrev_201907_18319
46. Wang B, Zheng J, Li R, Tian Y, Lin J, Liang Y, et al. Long Noncoding RNA LINC02582 Acts Downstream of miR-200c to Promote Radioresistance Through CHK1 in Breast Cancer Cells. Cell Death Dis (2019) 10(10):764. doi: 10.1038/s41419-019-1996-0
47. Liu L, Zhu Y, Liu AM, Feng Y, Chen Y. Long Noncoding RNA LINC00511 Involves in Breast Cancer Recurrence and Radioresistance by Regulating STXBP4 Expression via miR-185. Eur Rev Med Pharmacol Sci (2019) 23(17):7457–68. doi: 10.26355/eurrev_201909_18855
48. Dou Q, Xu Y, Zhu Y, Hu Y, Yan Y, Yan H. LncRNA FAM83H-AS1 Contributes to the Radioresistance, Proliferation, and Metastasis in Ovarian Cancer Through Stabilizing HuR Protein. Eur J Pharmacol (2019) 852:134–41. doi: 10.1016/j.ejphar.2019.03.002
49. Jia J, Zhang X, Zhan D, Li J, Li Z, Li H, et al. LncRNA H19 Interacted With miR-130a-3p and miR-17-5p to Modify Radio-Resistance and Chemo-Sensitivity of Cardiac Carcinoma Cells. Cancer Med (2019) 8(4):1604–18. doi: 10.1002/cam4.1860
50. Zhang J, Li W. Long Noncoding RNA CYTOR Sponges miR-195 to Modulate Proliferation, Migration, Invasion and Radiosensitivity in Nonsmall Cell Lung Cancer Cells. Biosci Rep (2018) 38(6). doi: 10.1042/bsr20181599
51. Yang QS, Li B, Xu G, Yang SQ, Wang P, Tang HH, et al. Long Noncoding RNA LINC00483/microRNA-144 Regulates Radiosensitivity and Epithelial-Mesenchymal Transition in Lung Adenocarcinoma by Interacting With HOXA10. J Cell Physiol (2019) 234(7):11805–21. doi: 10.1002/jcp.27886
52. Yang T, Li S, Liu J, Yin D, Yang X, Tang Q. lncRNA-NKILA/NF-κb Feedback Loop Modulates Laryngeal Cancer Cell Proliferation, Invasion, and Radioresistance. Cancer Med (2018) 7(5):2048–63. doi: 10.1002/cam4.1405
53. Zhang M, Gao C, Yang Y, Li G, Dong J, Ai Y, et al. Long Noncoding RNA CRNDE/PRC2 Participated in the Radiotherapy Resistance of Human Lung Adenocarcinoma Through Targeting p21 Expression. Oncol Res (2018) 26(8):1245–55. doi: 10.3727/096504017X14944585873668
54. Fotouhi Ghiam A, Taeb S, Huang X, Huang V, Ray J, Scarcello S, et al. Long non-Coding RNA Urothelial Carcinoma Associated 1 (UCA1) Mediates Radiation Response in Prostate Cancer. Oncotarget (2017) 8(3):4668–89. doi: 10.18632/oncotarget.13576
55. Wu D, Li Y, Zhang H, Hu X. Knockdown of Lncrna PVT1 Enhances Radiosensitivity in Non-Small Cell Lung Cancer by Sponging Mir-195. Cell Physiol Biochem (2017) 42(6):2453–66. doi: 10.1159/000480209
56. Jin C, Yan B, Lu Q, Lin Y, Ma L. The Role of MALAT1/miR-1/Slug Axis on Radioresistance in Nasopharyngeal Carcinoma. Tumour Biol (2016) 37(3):4025–33. doi: 10.1007/s13277-015-4227-z
57. Martinez I, Li J, Liu X, Nan S, Xu C. Silencing of Long non-Coding RNA LINC00520 Promotes Radiosensitivity of Head and Neck Squamous Cell Carcinoma Cells. Cancer Res (2020) 54(4):254–70. doi: 10.1080/10715762.2020.1752373
58. Fan S, Liu Y, Chen X, Chen X, Liu J, Gu H, et al. Long non-Coding RNA HOTAIR Knockdown Enhances Radiosensitivity Through Regulating microRNA-93/ATG12 Axis in Colorectal Cancer. Thorac Cancer (2020) 11(3):175. doi: 10.1038/s41419-020-2268-8
59. Lv X, Xu Z, Xu G, Li H, Wang C, Chen J, et al. Investigation of the Active Components and Mechanisms of Schisandra Chinensis in the Treatment of Asthma Based on a Network Pharmacology Approach and Experimental Validation. Food Funct (2020) 11(4):3032–42. doi: 10.1039/d0fo00087f
60. Chin CH, Chen SH, Wu HH, Ho CW, Ko MT, Lin CY. Cytohubba: Identifying Hub Objects and Sub-Networks From Complex Interactome. BMC Syst Biol (2014) 8(Suppl 4):S11. doi: 10.1186/1752-0509-8-S4-S11
61. Boileve A, Hilmi M, Delaye M, Tijeras-Raballand A, Neuzillet C. Biomarkers in Hepatobiliary Cancers: What is Useful in Clinical Practice? Cancers (Basel) (2021) 13(11). doi: 10.3390/cancers13112708
62. Rey S, Schito L, Koritzinsky M, Wouters BG. Molecular Targeting of Hypoxia in Radiotherapy. Adv Drug Delivery Rev (2017) 109:45–62. doi: 10.1016/j.addr.2016.10.002
63. Ganoth D, Bornstein G, Ko TK, Larsen B, Tyers M, Pagano M, et al. The Cell-Cycle Regulatory Protein Cks1 is Required for SCF(Skp2)-Mediated Ubiquitinylation of P27. Nat Cell Biol (2001) 3(3):321–4. doi: 10.1038/35060126
64. Jing L, Yuan W, Ruofan D, Jinjin Y, Haifeng Q. HOTAIR Enhanced Aggressive Biological Behaviors and Induced Radio-Resistance via Inhibiting P21 in Cervical Cancer. Tumour Biol (2015) 36(5):3611–9. doi: 10.1007/s13277-014-2998-2
65. Zaman S, Wang R, Gandhi V. Targeting the Apoptosis Pathway in Hematologic Malignancies. Leuk Lymphoma (2014) 55(9):1980–92. doi: 10.3109/10428194.2013.855307
Keywords: long non-coding RNAs, radioresistance, theragnostic biomarkers, cancer, meta-analysis
Citation: Lin P, Xing W, Ren Q, Wang Q, Yan J and Mao G (2022) LncRNAs as Theragnostic Biomarkers for Predicting Radioresistance in Cancer: A Systematic Review and Meta-Analysis. Front. Oncol. 12:767750. doi: 10.3389/fonc.2022.767750
Received: 15 September 2021; Accepted: 21 April 2022;
Published: 25 May 2022.
Edited by:
Hong Zheng, Stanford University, United StatesReviewed by:
Ganesh Kumar Maurya, Banaras Hindu University, IndiaSimone Moertl, Federal Office for Radiation Protection, Germany
Copyright © 2022 Lin, Xing, Ren, Wang, Yan and Mao. This is an open-access article distributed under the terms of the Creative Commons Attribution License (CC BY). The use, distribution or reproduction in other forums is permitted, provided the original author(s) and the copyright owner(s) are credited and that the original publication in this journal is cited, in accordance with accepted academic practice. No use, distribution or reproduction is permitted which does not comply with these terms.
*Correspondence: Genxiang Mao, bWFvZ2VueGlhbmdAMTYzLmNvbQ==; Jing Yan, emppY3VAdmlwLjE2My5jb20=
†They contribute equally to this work