- 1Department of Dermatology, University of Wisconsin-Madison, Madison, WI, United States
- 2William S. Middleton Memorial Veterans Hospital, Madison, WI, United States
Frizzled (FZD) proteins are receptors for the WNT family ligands. Inherited human diseases and genetic experiments using knockout mice have revealed a central role of FZDs in multiple aspects of embryonic development and tissue homeostasis. Misregulated FZD signaling has also been found in many cancers. Recent studies on three out of the ten mammalian FZDs in melanoma have shown that they promote tumor cell proliferation and invasion, via the activation of the canonical WNT/β-catenin or non-canonical PCP signaling pathway. In this concise review, we summarize our current knowledge of individual FZDs in melanoma, discuss the involvement of both the canonical and non-canonical pathways, and describe ongoing efforts to target the FZD receptors for melanoma treatment.
1 Introduction
Frizzled (FZD) family proteins belong to the G-protein coupled receptors (GPCRs), which are the largest group of cell surface receptors found in humans (1). There are six classes of GPCRs: Rhodopsin family (Class A), secretin receptor family (Class B), metabotropic glutamate/pheromone family (Class C), fungal mating pheromone receptor family (Class D), cyclic AMP receptor family (Class E), and FZD/Smoothened (SMO) family (Class F) (2). Compared to other GPCRs, FZD family receptors are thought to be atypical as the activity level of G protein signaling mediated by FZD is much lower than that of a typical GPCR (3). Spontaneous and targeted mutations in mammalian Frizzled genes have revealed their essential roles in various developmental and homeostatic processes, including palate and heart morphogenesis, tissue polarity, vascular formation and maintenance, and the development of the central nervous system, kidney, and bone (4). Increasing evidence also suggests that FZDs play an important role in cancer development and progression (5).
Frizzled receptors can activate three signaling pathways: canonical WNT/β-catenin pathway, non-canonical planar cell polarity (PCP) pathway, and WNT/calcium signaling pathway (4, 6). The canonical WNT/β-catenin signaling pathway is characterized by the stabilization of β-catenin upon ligand binding (5). The PCP pathway controls the cell/tissue polarity along the body axis, when mutated, results in various developmental defects in the body (7, 8). The WNT/calcium signaling pathway is defined by the regulation of intracellular Ca2+ levels and the activation of many calcium-sensitive enzymes (9). Although the activation of these three signaling pathways seems distinct and independent, convergent models of signaling networks where several pathways act in a coordinated and interdependent manner have been proposed (10). The role of Frizzled receptors in development and other cancers has been well-reviewed elsewhere (4, 5, 11). Here, we focus on summarizing our current knowledge of FZDs in melanoma. We describe the role of individual FZDs in melanoma, discuss the involvement of both the canonical WNT/β-catenin and PCP pathways, and highlight potential targeted therapy on the FZD signaling pathways for melanoma treatment.
2 FZDs and WNT ligands
There are ten members in the Frizzled family, FZD1-10 in humans and Fzd1-10 in mice. They are between 500 to 700 amino acids in length and can be divided into four sub-families based on the amino acid sequence homology (4). Results of the detailed comparison are shown in Figure 1 using the Clustal Omega multiple sequence alignment tool (12). In humans, FZD3 and FZD6 share 53% identity, FZD4, FZD9, and FZD10 share 52-65% identity, FZD1, FZD2, and FZD7 share approximately 78-80% identity, and FZD5 and FZD8 share 69% identity. FZDs from different sub-families share 34-53% identity. These numbers are very similar for mouse Frizzled proteins. Common structures of FZDs include an extracellular N-terminus, seven hydrophobic transmembrane domains, and an intracellular C-terminus. The N-terminus contains a conserved 120 amino acids cysteine-rich domain (CRD) connected to the first transmembrane helix by a hydrophilic linker of 70-120 amino acids (13). FZD utilizes CRD as a necessary and sufficient binding site for various ligands, including the WNT proteins, R-spondin, and Frizzled-related proteins (14–17). More precise WNT binding sites on CRD were determined by resolving the crystal structure of CRDs from mouse Fzd8 and secreted Frizzled-related protein 3, and it was shown that CRDs are predominantly α-helical held in place by the disulfide bonds formed by the ten conserved cysteines (18). The co-crystal structure of Xenopus Wnt8 in complex with mouse Fzd8-CRD well explained how a Wnt ligand engages the Fzd receptor (19). The first crystal structure of full-length human FZD has been resolved recently in a ligand-free state at a resolution of ~2 Å, paving the way to for better understanding the function of FZDs (20).
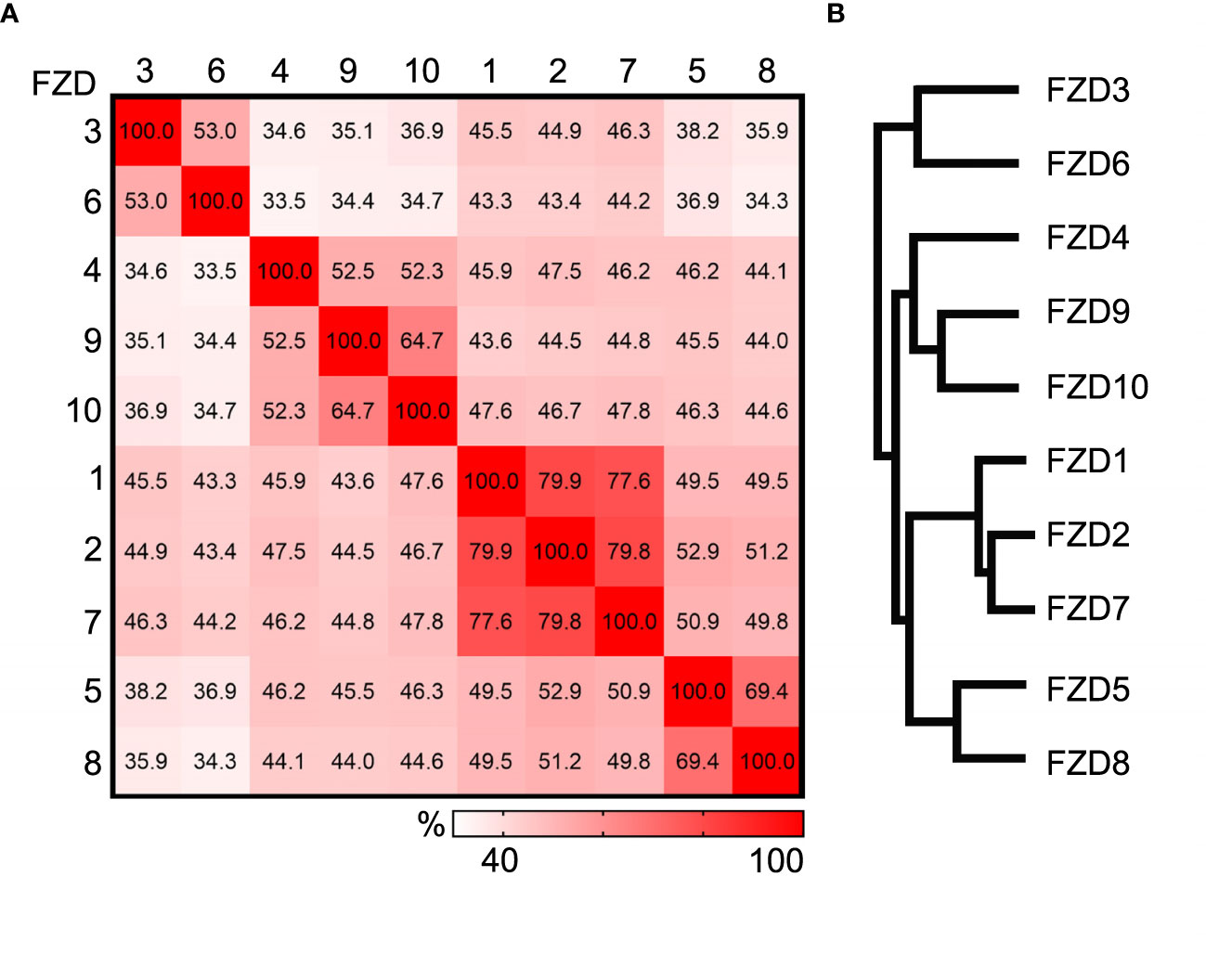
Figure 1 Comparison of human Frizzled proteins. (A) Heat plot showing the percent identity matrix of the 10 human Frizzled amino acid sequences. (B) Phylogram showing the relatedness of the 10 human FZDs. Sequence compared using the Clustal Omega multiple sequence alignment tool.
There are 19 WNT in mammals, and they are typically between 350 to 420 amid acids in length. WNT proteins from the same sub-families share 60-85% identity, and WNTs from different sub-families share about 30-50% identity (Figure 2). Like FZDs, WNT proteins are widely expressed in many tissues. For example, the expression of 13 Wnt genes and nine Fzd genes can be detected in the developing mouse skin (21–23). The 190 possible combinations from 19 WNT ligands and 10 FZDs make the study of the paired role of WNT/FZD extremely difficult. Moreover, the existence of non-WNT ligands for FZD receptors and non-FZD receptors for WNT ligands further complicates the pathway activation. For example, Norrin, a secreted protein encoded by the Norrie disease gene, can bind specifically to the Fzd4 receptor and regulate the angiogenesis and endothelial barrier function (24). Although not related to Wnt, Norrin can mimic Wnt for Frizzled recognition and promote Fzd4 clustering and activation (25). Wnt5a can bind to both Frizzled receptors and receptor tyrosine kinase-like orphan receptors (ROR1 and ROR2). The signaling outputs of Wnt5a often depend on the availability of receptor/coreceptor and the competition of other Wnt ligands binding to receptors (26, 27). It has been reported that Wnt5a can both activate and inhibit canonical Wnt signaling (28). Its binding to the ROR receptors is believed to activate non-canonical PCP signaling (29, 30).
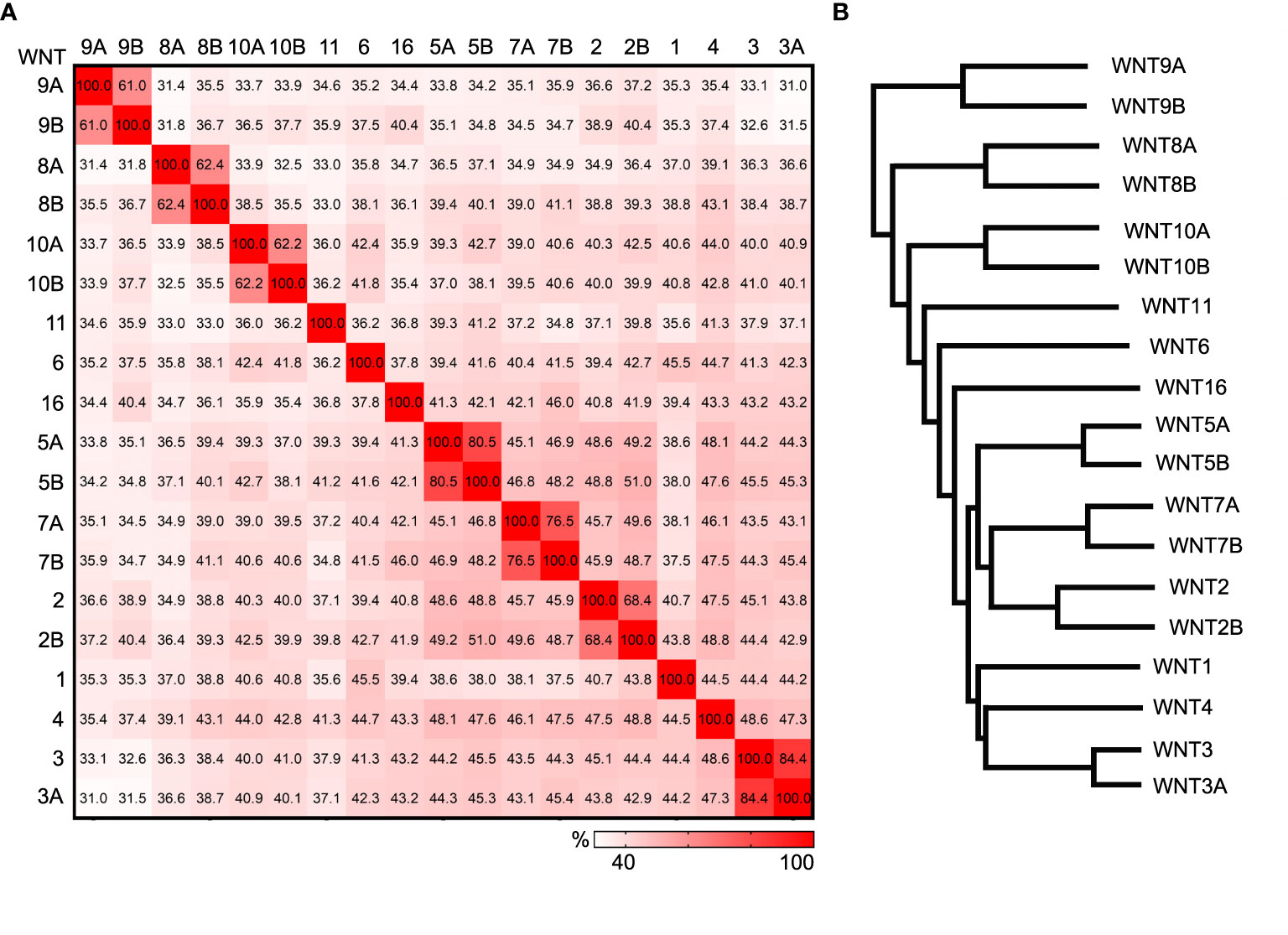
Figure 2 Comparison of human WNT ligands. (A) Heat plot showing the percent identity matrix of the 19 human WNT amino acid sequences. (B) Phylogram showing the relatedness of the 19 human WNTs. Sequence compared using the Clustal Omega multiple sequence alignment tool.
3 Canonical WNT/β-Catenin signaling and melanoma
The activation of the canonical WNT signaling pathway involves the inhibition of β-catenin degradation complex, resulting in the accumulation of β-catenin in the cytoplasm and the translocation of β-catenin from the cytoplasm into the nucleus to regulate transcription in combination with LEF/TCF transcription factors (5, 6). The canonical WNT signaling plays an important role in multiple steps of normal melanocyte development, including the migration of its precursor neural crest cell, melanocyte lineage specification, and terminal differentiation (31). β-catenin can directly interact with microphthalmia-associated transcription factor (MITF), a key regulator of melanocytes, to promote melanocyte stem cell proliferation (32). In melanoma, the canonical WNT/β-catenin signaling appears to have a cancer-promoting role, similarly as in many other cancers (33). Mutations in components of the canonical WNT signaling pathway genes, such as APC, AXIN1, and CTNNB1, are found at a frequency of 10%, 2.9%, and 5.9% (34). The pro-cancer role of the canonical WNT/β-catenin signaling in melanoma has been well supported by studies with the Pten/BRaf mouse model of melanoma. Silencing the canonical Wnt signaling by β-catenin knockout can slow down tumor cell proliferation and inhibit melanoma metastasis, while activation of the canonical Wnt signaling by β-catenin stabilization can accelerate tumor cell proliferation and melanoma metastasis (35). Activation of the WNT/β-catenin signaling can also promote melanomagenesis by bypassing the oncogen-induced senescence (36–38) or enabling immune evasion (39).
4 Non-canonical WNT signaling and melanoma
Most of our knowledge about the role of non-canonical WNT signaling pathway in melanoma came from the studies on WNT5A. Gene expression profiling initially identified that WNT5A expression correlated with cell motility and invasiveness of human melanoma cells. This increase in melanoma cell invasion was not related to the canonical WNT signaling activation, as no increase in β-catenin expression or nuclear translocation was observed. Instead, protein kinase C (PKC) activation was dramatically increased upon WNT5A overexpression (40). Further studies suggested a phenotypic switch model of melanoma cells, in which the expression of WNT5A and CTNNB1 (encodes β-catenin) dictates the proliferation or invasion status. Cells with high CTNNB1 and low WNT5A are highly proliferative, and cells with low CTNNB1 and high WNT5A are highly invasive (41, 42). Tumor cells need to switch from high-CTNNB1, low WNT5A to low-CTNNB1, high WNT5A to initiate metastasis and move away from the primary site. Once they arrive at the site of metastasis, they need to switch back to the proliferative phenotype to colonize at the new location. A recent study shows that WNT5A regulates the expression of the tyrosine kinase receptors AXL and MER to promote tumor dormancy and drive dissemination (43). It remains unclear which receptors WNT5A signals through in regulating the phenotype switch, although evidence for ROR2 and FZD5 both exist (40, 44).
5 FZDs and melanoma
5.1. FZD1, FZD2 and FZD7
Mouse knockout studies have revealed a critical function of Fzd1/2/7 in palate closure, convergent extension of the neural tube, and heart development (45, 46). Among the ten FZDs, FZD7 is the most studied member in cancer. Overexpression of FZD7 in cancer cells (e.g., intestinal cancer, hepatocellular carcinoma, and breast cancer) often results in increased cell proliferation and tumor growth (11, 47). Thus, targeted inhibition of FZD7 has been considered as a promising approach for cancer therapy. Limited studies in melanoma also indicate a similar pro-oncogenic role of FZD7. Upregulation of FZD7 expression in melanoma cells contributes to the drug resistance to the BRAF inhibitor PLX4720 (48). Knockdown of FZD7 in melanoma cells inhibits the formation of xenograft tumors and metastatic growth in the lung following intravenous injection (49). A recent study has shown that the WNT11-FZD7-DAAM1 axis activates Rho-ROCK1/2-Myosin II in melanoma and plays a crucial role in regulating tumor-initiating potential, local invasion, and distant metastasis formation (50). The role of FZD1 and FZD2 in melanoma has not been investigated.
5.2 FZD3 and FZD6
Activation of the canonical WNT signaling can be monitored in vitro using HEK293 cells carrying a luciferase reporter under the control of 7 LEF/TCF binding sites (Super TOP-FLASH, STF cells) (24). FZD3 and FZD6 are believed to signal mainly through the non-canonical PCP pathway since they do not activate canonical WNT signaling when cotransfected with many WNTs in the STF cells (45). Knockout studies in mice show that Fzd3 is required for axon guidance of thalamocortical neurons and spinal sensory neurons (51, 52). Fzd6 is required in patterning hair follicles and follicle-associated structures (53, 54). Together, Fzd3 and Fzd6 also play redundant roles in neural tube closure and patterning hair cells in the inner ear (55).
Both FZD3 and FZD6 have been found to play a pro-cancer role in melanoma. FZD3 is overexpressed in ~20% of human patient melanoma samples (56). Knockdown of FZD3 in patient-derived melanoma cells reduces melanoma cell proliferation and progression when engrafted into NSG mice (57). We recently found that FZD6 is also overexpressed in multiple melanoma cell lines and patient tissues. Knockdown or knockout of FZD6 does not affect cell proliferation but slows down cell invasion. Moreover, knockout of Fzd6 dramatically reduces lung metastasis in the Pten/BRaf mouse model of melanoma (58). It will be interesting to dissect the mechanistic difference between FZD3 and FZD6 in melanoma, as they clearly regulate different aspects of melanoma cell behaviors (FZD3 in cell proliferation and FZD6 in cell invasion).
5.3 FZD5 and FZD8
The role of FZD5 and FZD8 in melanoma has not been studied directly. Antibodies against FZD5 have been shown to be able to block melanoma cell migration and invasion in vitro, possibly by the inhibition of PKC activity (40). An FZD8-related asialylated antiproliferative factor can inhibit the proliferation of two melanoma cell lines in vitro, Hs839T and A375 (59). Due to the caveats of the specificity of the chemical inhibitors or blocking antibodies, further studies are needed to directly determine the function of FZD5 and FZD8 in melanoma.
5.4 FZD4, FZD9, and FZD10
FZD4 is believed to signal through the canonical Wnt signaling (4). It binds with WNT7A and WNT7B and non-WNT ligand Norrin (24, 60, 61). They can form a signaling complex with co-receptors LRP5 and TSPAN12 to regulate the vascular formation and maintain the blood-retina barrier and the blood-brain barrier (62, 63). FZD9 plays a role in the development of B-cell, dentate gyrus, and bone (64–66). The role of FZD4 and FZD9 in melanoma has not been studied. Expression of FZD10 has been reported in many cancers, including melanoma. In colon cancer, the cytoplasmic staining of FZD10 increases as the tumor progress. Interestingly, an opposite pattern has been observed in melanoma and gastric cancer, as the cytoplasmic staining of FZD10 is much lower in metastatic tumors than in early-stage tumors (67). The significance of the dynamic expression of FZD10 in melanoma is unknown.
6 Therapeutic potential of FZD receptors
GPCRs are important drug targets as they are involved in various diseases. As of 2018, about 34% (n=475) of drugs approved by the Food and Drug Administration (FDA) target 108 GPCR family members (68). Given the general cancer-promoting role of FZDs, several strategies have also been explored to inhibit FZDs for cancer treatment. For example, antibodies targeting the extracellular domain of FZD5, FZD7, and FZD10 have been successfully applied to inhibit the individual FZD-induced tumor growth in pancreatic ductal adenocarcinoma, Wilms’ tumor, and synovial sarcoma, respectively (69–71). The monoclonal antibody OMP-18R5 (vantictumab), initially identified by binding to FZD7, can interact with four other FZD receptors (FZD1, FZD2, FZD5, and FZD8) and block the canonical WNT signaling induced by multiple WNT family members. This antibody can inhibit the growth of a range of tumor types, including lung, breast, colon, and pancreatic tumors in xenograft studies (72). OMP-54F28 (ipafricept), a recombinant fusion protein consisting of the FZD8 CRD and a human IgG1 Fc fragment, has been designed as a decoy receptor to sequester WNTs and prevent them from binding to FZD8 (73). This recombinant protein is effective in inhibiting FZD8-mediated solid tumor growth in preclinical models, although no notable anti-cancer response has been observed in clinical trials (74–76). Similarly, a soluble extracellular peptide of FZD7 has been utilized as a decoy receptor for inhibiting FZD7 in hepatocellular carcinoma cells (77). The idea of disrupting the FZD-induced signaling pathways to treat cancer has also been tried in melanoma (78). FJ9 is a compound designed to disrupt the interaction between the FZD7 and the PDZ domain of Dishevelled. Melanoma cell line LOX treated with FJ9 in vitro can inhibit tumor cell growth and cause significant apoptosis. The effects appear to be related to the inhibition of the canonical WNT signaling. Although the in vivo effect of FJ9 on melanoma growth has not been tested, it does inhibit the growth of non-small cell lung cancer xenografts in nude mice.
MicroRNA (miRNA)-based gene therapies have shown promise in preclinical studies for cancer treatment, and several have advanced into clinical testing (79). The discovery of various miRNA(s) of FZD receptors has paved the way for designing suitable miRNA-based therapeutic strategies against different cancers, including melanoma. For example, miR-485-5p has been found to target the 3’-untranslated region (3’-UTR) of FZD7. It can inhibit melanoma cell invasion and proliferation in vitro by suppressing FZD7 expression, thus providing a promising therapeutic target for melanoma treatment (80). FZD6 expression can be negatively regulated by several miRNAs, such as miR-199a-5p, miR-125b, and miR-20b (81, 82). Given the pro-invasion role recently identified for FZD6 in melanoma, it will be interesting to see if these miRNAs can be used for preventing melanoma progression and metastasis.
7 Conclusion
Genetic studies in animal models have revealed critical functions of FZD receptors in embryonic development and adult tissue homeostasis. Increasing evidence also suggests a role of FZDs in many cancers. FZD activation, either via the canonical WNT/β-catenin or the non-canonical pathway, appears to have a universal cancer-promoting role in melanoma. Although known at a descriptive level, FZD3, FZD7, and the most recent addition of FZD6 are involved in melanoma cell survival and invasion. The function of the other seven FZDs in melanoma remains to be studied. Perhaps the greatest challenge is to define the role of individual WNT/FZD pairs in melanoma, not only in cell survival and invasion, but also in drug resistance, immune escape, and tumor microenvironment. A detailed, mechanistic understanding of the FZD signaling pathway in melanoma is needed for developing potential therapy. Effort is already underway to target FZD receptors for cancer treatment, including blocking antibodies, peptide inhibitors, small molecule inhibitors, and miRNA-based therapies. Finding an effective but specific targeted therapy on FZDs for melanoma is still a challenging task, given the complexity of the signaling pathway and broad expression of FZDs in many normal tissues. Specific delivery of the drug(s) to melanoma cells will greatly improve the safety and effectiveness of treatment.
Author contributions
SU and BD wrote the main body of the paper. MN and HC revised the review. HC conceived and directed the idea of the manuscript. All authors contributed to the article and approved the submitted version.
Funding
HC was supported by the Department of Veterans Affairs VA Merit Review Awards I01CX002308 and the Gary S. Wood Dermatology Research Bascom Endowed Professorship.
Conflict of interest
The authors declare that the research was conducted in the absence of any commercial or financial relationships that could be construed as a potential conflict of interest.
Publisher’s note
All claims expressed in this article are solely those of the authors and do not necessarily represent those of their affiliated organizations, or those of the publisher, the editors and the reviewers. Any product that may be evaluated in this article, or claim that may be made by its manufacturer, is not guaranteed or endorsed by the publisher.
References
1. Wootten D, Christopoulos A, Marti-Solano M, Babu MM, Sexton PM. Mechanisms of signalling and biased agonism in G protein-coupled receptors. Nat Rev Mol Cell Biol (2018) 19(10):638–53. doi: 10.1038/s41580-018-0049-3
2. Foord SM, Bonner TI, Neubig RR, Rosser EM, Pin JP, Davenport AP, et al. International union of pharmacology. XLVI. G protein-coupled receptor list. Pharmacol Rev (2005) 57(2):279–88. doi: 10.1124/pr.57.2.5
3. Nichols AS, Floyd DH, Bruinsma SP, Narzinski K, Baranski TJ. Frizzled receptors signal through G proteins. Cell Signal (2013) 25(6):1468–75. doi: 10.1016/j.cellsig.2013.03.009
4. Wang Y, Chang H, Rattner A, Nathans J. Frizzled receptors in development and disease. Curr Top Dev Biol (2016) 117:113–39. doi: 10.1016/bs.ctdb.2015.11.028
5. MacDonald BT, Tamai K, He X. Wnt/beta-catenin signaling: components, mechanisms, and diseases. Dev Cell (2009) 17(1):9–26. doi: 10.1016/j.devcel.2009.06.016
6. Nusse R, Clevers H. Wnt/β-catenin signaling, disease, and emerging therapeutic modalities. Cell (2017) 169(6):985–99. doi: 10.1016/j.cell.2017.05.016
7. McNeill H. Planar cell polarity: Keeping hairs straight is not so simple. Cold Spring Harb Perspect Biol (2010) 2(2):a003376. doi: 10.1101/cshperspect.a003376
8. Butler MT, Wallingford JB. Planar cell polarity in development and disease. Nat Rev Mol Cell Biol (2017) 18(6):375–88. doi: 10.1038/nrm.2017.11
9. Kühl M. The WNT/calcium pathway: Biochemical mediators, tools and future requirements. Front Biosci (2004) 9:967–74. doi: 10.2741/1307
10. Thrasivoulou C, Millar M, Ahmed A. Activation of intracellular calcium by multiple wnt ligands and translocation of β-catenin into the nucleus: A convergent model of Wnt/Ca2+ and wnt/β-catenin pathways. J Biol Chem (2013) 288(50):35651–9. doi: 10.1074/jbc.M112.437913
11. Zeng CM, Chen Z, Fu L. Frizzled receptors as potential therapeutic targets in human cancers. Int J Mol Sci (2018) 19(5):1–18. doi: 10.3390/ijms19051543
12. Madeira F, Pearce M, Tivey AR, Basutkar P, Lee J, Edbali O, et al. Search and sequence analysis tools services from EMBL-EBI in 2022. Nucleic Acids Res (2022) 50(W1):W276–W279. doi: 10.1093/nar/gkac240
13. MacDonald BT, He X. Frizzled and LRP5/6 receptors for wnt/β-catenin signaling. Cold Spring Harbor Perspect Biol (2012) 4(12):a007880. doi: 10.1101/cshperspect.a007880
14. Bhanot P, Brink M, Samos S, Hsieh J, Wang Y, Macke J, et al. A new member of the frizzled family from drosophila functions as a wingless receptor. Nature (1996) 382(6588):225–30. doi: 10.1038/382225a0
15. Hsieh J-C, Rattner A, Smallwood PM, Nathans J. Biochemical characterization of wnt-frizzled interactions using a soluble, biologically active vertebrate wnt protein. Proc Natl Acad Sci (1999) 96(7):3546–51. doi: 10.1073/pnas.96.7.3546
16. Rulifson EJ, Wu CH, Nusse R. Pathway specificity by the bifunctional receptor frizzled is determined by affinity for wingless. Mol Cell (2000) 6(1):117–26. doi: 10.1016/S1097-2765(05)00018-3
17. Wu CH, Nusse R. Ligand receptor interactions in the wnt signaling pathway in drosophila. J Biol Chem (2002) 277(44):41762–9. doi: 10.1074/jbc.M207850200
18. Dann CE, Hsieh JC, Rattner A, Sharma D, Nathans J, Leahy DJ. Insights into wnt binding and signalling from the structures of two frizzled cysteine-rich domains. Nature (2001) 412(6842):86–90. doi: 10.1038/35083601
19. Janda CY, Waghray D, Levin AM, Thomas C, Garcia KC. Structural basis of wnt recognition by frizzled. Science (2012) 337(6090):59–64. doi: 10.1126/science.1222879
20. Yang S, Wu Y, Xu TH, de Waal PW, He Y, Pu M, et al. Crystal structure of the frizzled 4 receptor in a ligand-free state. Nature (2018) 560(7720):666–70. doi: 10.1038/s41586-018-0447-x
21. Simonson L, Oldham E, Chang H. Overactive Wnt5a signaling disrupts hair follicle polarity during mouse skin development. Development (2022) 149(22):1–12. doi: 10.1242/dev.200816
22. Reddy ST, Andl T, Lu MM, Morrisey EE, Millar SE. Expression of frizzled genes in developing and postnatal hair follicles. J Invest Dermatol (2004) 123(2):275–82. doi: 10.1111/j.0022-202X.2004.23215.x
23. Reddy S, Andl T, Bagasra A, Lu MM, Epstein DJ, Morrisey EE, et al. Characterization of wnt gene expression in developing and postnatal hair follicles and identification of Wnt5a as a target of sonic hedgehog in hair follicle morphogenesis. Mech Dev (2001) 107(1-2):69–82. doi: 10.1016/S0925-4773(01)00452-X
24. Xu Q, Wang Y, Dabdoub A, Smallwood PM, Williams J, Woods C, et al. Vascular development in the retina and inner ear: Control by norrin and frizzled-4, a high-affinity ligand-receptor pair. Cell (2004) 116(6):883–95. doi: 10.1016/S0092-8674(04)00216-8
25. Chang TH, Hsieh FL, Zebisch M, Harlos K, Elegheert J, Jones EY. Structure and functional properties of norrin mimic wnt for signalling with Frizzled4, Lrp5/6, and proteoglycan. Elife (2015) 4:1–27. doi: 10.7554/eLife.06554
26. Mikels AJ, Nusse R. Purified Wnt5a protein activates or inhibits beta-catenin-TCF signaling depending on receptor context. PloS Biol (2006) 4(4):e115. doi: 10.1371/journal.pbio.0040115
27. He X, Nathans J, Dawid I, Varmus HA. A member of the frizzled protein family mediating axis induction by wnt-5A. Science (1997) 275(5306):1652–4. doi: 10.1126/science.275.5306.1652
28. van Amerongen R, Fuerer C, Mizutani M, Nusse R. Wnt5a can both activate and repress wnt/β-catenin signaling during mouse embryonic development. Dev Biol (2012) 369(1):101–14. doi: 10.1016/j.ydbio.2012.06.020
29. Oishi I, Suzuki H, Onishi N, Takada R, Kani S, Ohkawara B. The receptor tyrosine kinase Ror2 is involved in non-canonical Wnt5a/JNK signalling pathway. Genes Cells (2003) 8(7):645–54. doi: 10.1046/j.1365-2443.2003.00662.x
30. Hikasa H, Shibata M, Hiratani I, Taira M. The xenopus receptor tyrosine kinase Xror2 modulates morphogenetic movements of the axial mesoderm and neuroectoderm via wnt signaling. Development (2002) 129(22):5227–39. doi: 10.1242/dev.129.22.5227
31. Mort RL, Jackson IJ, Patton EE. The melanocyte lineage in development and disease. Development (2015) 142(4):620–32. doi: 10.1242/dev.106567
32. Schepsky A, Bruser K, Gunnarsson GJ, Goodall J, Hallsson JH, Goding CR, et al. The microphthalmia-associated transcription factor mitf interacts with β-catenin to determine target gene expression. Mol Cell Biol (2006) 26(23):8914–27. doi: 10.1128/MCB.02299-05
33. Zhan T, Rindtorff N, Boutros M. Wnt signaling in cancer. Oncogene (2017) 36(11):1461–73. doi: 10.1038/onc.2016.304
34. Gajos-Michniewicz A, Czyz M. WNT signaling in melanoma. Int J Mol Sci (2020) 21(14):4852. doi: 10.3390/ijms21144852
35. Damsky WE, Curley DP, Santhanakrishnan M, Rosenbaum LE, Platt JT, Rothberg BEG, et al. β-catenin signaling controls metastasis in braf-activated pten-deficient melanomas. Cancer Cell (2011) 20(6):741–54. doi: 10.1016/j.ccr.2011.10.030
36. Delmas V, Beermann F, Martinozzi S, Carreira S, Ackermann J, Kumasaka M, et al. Beta-catenin induces immortalization of melanocytes by suppressing p16INK4a expression and cooperates with n-ras in melanoma development. Genes Dev (2007) 21(22):2923–35. doi: 10.1101/gad.450107
37. Juan J, Muraguchi T, Iezza G, Sears RC, McMahon M. Diminished WNT -> β-catenin -> c-MYC signaling is a barrier for malignant progression of BRAFV600E-induced lung tumors. Genes Dev (2014) 28(6):561–75. doi: 10.1101/gad.233627.113
38. Pawlikowski JS, McBryan T, van Tuyn J, Drotar ME, Hewitt RN, Maier AB, et al. Wnt signaling potentiates nevogenesis. Proc Natl Acad Sci USA (2013) 110(40):16009–14. doi: 10.1073/pnas.1303491110
39. Nsengimana J, Laye J, Filia A, O’Shea S, Muralidhar S, Poźniak J, et al. β-catenin-mediated immune evasion pathway frequently operates in primary cutaneous melanomas. J Clin Invest (2018) 128(5):2048–63. doi: 10.1172/JCI95351
40. Weeraratna AT, Jiang Y, Hostetter G, Rosenblatt K, Duray P, Bittner M, et al. Wnt5a signaling directly affects cell motility and invasion of metastatic melanoma. Cancer Cell (2002) 1(3):279–88. doi: 10.1016/S1535-6108(02)00045-4
41. Hoek KS, Schlegel NC, Brafford P, Sucker A, Ugurel S, Kumar R, et al. Metastatic potential of melanomas defined by specific gene expression profiles with no BRAF signature. Pigment Cell Res (2006) 19(4):290–302. doi: 10.1111/j.1600-0749.2006.00322.x
42. Webster MR, Weeraratna AT. A wnt-er migration: the confusing role of β-catenin in melanoma metastasis. Sci Signal (2013) 6(268):pe11. doi: 10.1126/scisignal.2004114
43. Fane ME, Chhabra Y, Alicea GM, Maranto DA, Douglass SM, Webster MR, et al. Stromal changes in the aged lung induce an emergence from melanoma dormancy. Nature (2022) 606(7913):396–405. doi: 10.1038/s41586-022-04774-2
44. O'Connell MP, Fiori JL, Xu M, Carter AD, Frank BP, Camilli TC, et al. The orphan tyrosine kinase receptor, ROR2, mediates Wnt5A signaling in metastatic melanoma. Oncogene (2010) 29(1):34–44. doi: 10.1038/onc.2009.305
45. Yu H, Ye X, Guo N, Nathans J. Frizzled 2 and frizzled 7 function redundantly in convergent extension and closure of the ventricular septum and palate: Evidence for a network of interacting genes. Development (2012) 139(23):4383–94. doi: 10.1242/dev.083352
46. Yu H, Smallwood PM, Wang Y, Vidaltamayo R, Reed R, Nathans J. Frizzled 1 and frizzled 2 genes function in palate, ventricular septum and neural tube closure: general implications for tissue fusion processes. Development (2010) 137(21):3707–17. doi: 10.1242/dev.052001
47. Phesse T, Flanagan D, Vincan E. Frizzled7: A promising achilles' heel for targeting the wnt receptor complex to treat cancer. Cancers (Basel) (2016) 8(5):1–33. doi: 10.3390/cancers8050050
48. Anastas JN, Kulikauskas RM, Tamir T, Rizos H, Long GV, Von Euw EM, et al. WNT5A enhances resistance of melanoma cells to targeted BRAF inhibitors. J Clin Invest (2014) 124(7):2877–90. doi: 10.1172/JCI70156
49. Tiwary S, Xu L. FRIZZLED7 is required for tumor initiation and metastatic growth of melanoma cells. PloS One (2016) 11(1):e0147638. doi: 10.1371/journal.pone.0147638
50. Rodriguez-Hernandez I, Maiques O, Kohlhammer L, Cantelli G, Perdrix-Rosell A, Monger J, et al. WNT11-FZD7-DAAM1 signalling supports tumour initiating abilities and melanoma amoeboid invasion. Nat Commun (2020) 11(1):5315. doi: 10.1038/s41467-020-18951-2
51. Hua ZL, Smallwood PM, Nathans J. Frizzled3 controls axonal development in distinct populations of cranial and spinal motor neurons. Elife (2013) 2:e01482. doi: 10.7554/eLife.01482
52. Wang Y, Thekdi N, Smallwood PM, Macke JP, Nathans J. Frizzled-3 is required for the development of major fiber tracts in the rostral CNS. J Neurosci (2002) 22(19):8563–73. doi: 10.1523/JNEUROSCI.22-19-08563.2002
53. Chang H, Nathans J. Responses of hair follicle-associated structures to loss of planar cell polarity signaling. Proc Natl Acad Sci USA (2013) 110(10):E908–17. doi: 10.1073/pnas.1301430110
54. Guo N, Hawkins C, Nathans J. Frizzled6 controls hair patterning in mice. Proc Natl Acad Sci USA (2004) 101(25):9277–81. doi: 10.1073/pnas.0402802101
55. Wang Y, Guo N, Nathans J. The role of Frizzled3 and Frizzled6 in neural tube closure and in the planar polarity of inner-ear sensory hair cells. J Neurosci (2006) 26(8):2147–56. doi: 10.1523/JNEUROSCI.4698-05.2005
56. Siemers NO, Holloway JL, Chang H, Chasalow SD, Ross-MacDonald PB, Voliva CF, et al. Genome-wide association analysis identifies genetic correlates of immune infiltrates in solid tumors. PloS One (2017) 12(7):e0179726. doi: 10.1371/journal.pone.0179726
57. Li C, Nguyen V, Clark KN, Zahed T, Sharkas S, Filipp FV, et al. Down-regulation of FZD3 receptor suppresses growth and metastasis of human melanoma independently of canonical WNT signaling. Proc Natl Acad Sci USA (2019) 116(10):4548–57. doi: 10.1073/pnas.1813802116
58. Dong B, Simonson L, Vold S, Oldham E, Barten L, Ahmad N, et al. Frizzled 6 promotes melanoma cell invasion but not proliferation by regulating canonical Wnt signaling and EMT. J Invest Dermatol (2022) S0022-202X(22):02660-4. doi: 10.1016/j.jid.2022.09.658
59. Koch KR, Zhang CO, Kaczmarek P, Barchi J, Guo L, Shahjee HM, et al. The effect of a novel frizzled 8-related antiproliferative factor on in vitro carcinoma and melanoma cell proliferation and invasion. Invest New Drugs (2012) 30(5):1849–64. doi: 10.1007/s10637-011-9746-x
60. Cho C, Smallwood PM, Nathans J. Reck and Gpr124 are essential receptor cofactors for Wnt7a/Wnt7b-specific signaling in mammalian CNS angiogenesis and blood-brain barrier regulation. Neuron (2017) 95(5):1221–5. doi: 10.1016/j.neuron.2017.08.032
61. Zhou Y, Nathans J. Gpr124 controls CNS angiogenesis and blood-brain barrier integrity by promoting ligand-specific canonical wnt signaling. Dev Cell (2014) 31(2):248–56. doi: 10.1016/j.devcel.2014.08.018
62. Ye X, Wang Y, Cahill H, Yu M, Badea TC, Smallwood PM, et al. Norrin, frizzled-4, and Lrp5 signaling in endothelial cells controls a genetic program for retinal vascularization. Cell (2009) 139(2):285–98. doi: 10.1016/j.cell.2009.07.047
63. Wang Y, Rattner A, Zhou Y, Williams J, Smallwood PM, Nathans J. Norrin/Frizzled4 signaling in retinal vascular development and blood brain barrier plasticity. Cell (2012) 151(6):1332–44. doi: 10.1016/j.cell.2012.10.042
64. Ranheim EA, Kwan HC, Reya T, Wang YK, Weissman IL, Francke U. Frizzled 9 knock-out mice have abnormal b-cell development. Blood (2005) 105(6):2487–94. doi: 10.1182/blood-2004-06-2334
65. Zhao C, Avilés C, Abel RA, Almli CR, McQuillen P, Pleasure SJ. Hippocampal and visuospatial learning defects in mice with a deletion of frizzled 9, a gene in the williams syndrome deletion interval. Development (2005) 132(12):2917–27. doi: 10.1242/dev.01871
66. Albers J, Schulze J, Beil FT, Gebauer M, Baranowsky A, Keller J, et al. Control of bone formation by the serpentine receptor frizzled-9. J Cell Biol (2011) 192(6):1057–72. doi: 10.1083/jcb.201008012
67. Scavo MP, Fucci L, Caldarola L, Mangia A, Azzariti A, Simone G, et al. Frizzled-10 and cancer progression: Is it a new prognostic marker? Oncotarget (2018) 9(1):824–30. doi: 10.18632/oncotarget.23159
68. Hauser AS, Chavali S, Masuho I, Jahn LJ, Martemyanov KA, Gloriam DE, et al. Pharmacogenomics of GPCR drug targets. Cell (2018) 172(1-2):41–54.e19. doi: 10.1016/j.cell.2017.11.033
69. Pode-Shakked N, Harari-Steinberg O, Haberman-Ziv Y, Rom-Gross E, Bahar S, Omer D, et al. Resistance or sensitivity of wilms' tumor to anti-FZD7 antibody highlights the wnt pathway as a possible therapeutic target. Oncogene (2011) 30(14):1664–80. doi: 10.1038/onc.2010.549
70. Nagayama S, Fukukawa C, Katagiri T, Okamoto T, Aoyama T, Oyaizu N. Therapeutic potential of antibodies against FZD 10, a cell-surface protein, for synovial sarcomas. Oncogene (2005) 24(41):6201–12. doi: 10.1038/sj.onc.1208780
71. Steinhart Z, Pavlovic Z, Chandrashekhar M, Hart T, Wang X, Zhang X. Genome-wide CRISPR screens reveal a wnt-FZD5 signaling circuit as a druggable vulnerability of RNF43-mutant pancreatic tumors. Nat Med (2017) 23(1):60–8. doi: 10.1038/nm.4219
72. Gurney A, Axelrod F, Bond CJ, Cain J, Chartier C, Donigan L, et al. Wnt pathway inhibition via the targeting of frizzled receptors results in decreased growth and tumorigenicity of human tumors. Proc Natl Acad Sci USA (2012) 109(29):11717–22. doi: 10.1073/pnas.1120068109
73. Le PN, McDermott JD, Jimeno A. Targeting the wnt pathway in human cancers: therapeutic targeting with a focus on OMP-54F28. Pharmacol Ther (2015) 146:1–11. doi: 10.1016/j.pharmthera.2014.08.005
74. Le PN, Keysar SB, Miller B, Eagles JR, Chimed TS, Reisinger J, et al. Wnt signaling dynamics in head and neck squamous cell cancer tumor-stroma interactions. Mol Carcinog (2019) 58(3):398–410. doi: 10.1002/mc.22937
75. Jimeno A, Gordon M, Chugh R, Messersmith W, Mendelson D, Dupont J, et al. A first-in-Human phase I study of the anticancer stem cell agent ipafricept (OMP-54F28), a decoy receptor for wnt ligands, in patients with advanced solid tumors. Clin Cancer Res (2017) 23(24):7490–7. doi: 10.1158/1078-0432.CCR-17-2157
76. Moore KN, Gunderson CC, Sabbatini P, McMeekin DS, Mantia-Smaldone G, Burger RA, et al. A phase 1b dose escalation study of ipafricept (OMP54F28) in combination with paclitaxel and carboplatin in patients with recurrent platinum-sensitive ovarian cancer. Gynecol Oncol (2019) 154(2):294–301. doi: 10.1016/j.ygyno.2019.04.001
77. Wei W, Chua MS, Grepper S, So SK. Soluble frizzled-7 receptor inhibits wnt signaling and sensitizes hepatocellular carcinoma cells towards doxorubicin. Mol Cancer (2011) 10:16. doi: 10.1186/1476-4598-10-16
78. Fujii N, You L, Xu Z, Uematsu K, Shan J, He B, et al. An antagonist of dishevelled protein-protein interaction suppresses beta-catenin-dependent tumor cell growth. Cancer Res (2007) 67(2):573–9. doi: 10.1158/0008-5472.CAN-06-2726
79. Rupaimoole R, Slack FJ. MicroRNA therapeutics: towards a new era for the management of cancer and other diseases. Nat Rev Drug Discovery (2017) 16(3):203–22. doi: 10.1038/nrd.2016.246
80. Wu J, Li J, Ren J, Zhang D. MicroRNA-485-5p represses melanoma cell invasion and proliferation by suppressing Frizzled7. BioMed Pharmacother (2017) 90:303–10. doi: 10.1016/j.biopha.2017.03.064
81. Kim BK, Yoo HI, Kim I, Park J, Yoon SK. FZD6 expression is negatively regulated by miR-199a-5p in human colorectal cancer. BMB Rep (2015) 48(6):360–6. doi: 10.5483/BMBRep.2015.48.6.031
Keywords: frizzled, Wnt/β-catenin signaling, planar cell polarity, cancer, melanoma
Citation: Umar SA, Dong B, Nihal M and Chang H (2022) Frizzled receptors in melanomagenesis: From molecular interactions to target identification. Front. Oncol. 12:1096134. doi: 10.3389/fonc.2022.1096134
Received: 11 November 2022; Accepted: 07 December 2022;
Published: 23 December 2022.
Edited by:
Michael Eccles, University of Otago, New ZealandReviewed by:
Meredith Tennis, University of Colorado Denver, United StatesCopyright © 2022 Umar, Dong, Nihal and Chang. This is an open-access article distributed under the terms of the Creative Commons Attribution License (CC BY). The use, distribution or reproduction in other forums is permitted, provided the original author(s) and the copyright owner(s) are credited and that the original publication in this journal is cited, in accordance with accepted academic practice. No use, distribution or reproduction is permitted which does not comply with these terms.
*Correspondence: Hao Chang, aGNoYW5nQGRlcm1hdG9sb2d5Lndpc2MuZWR1