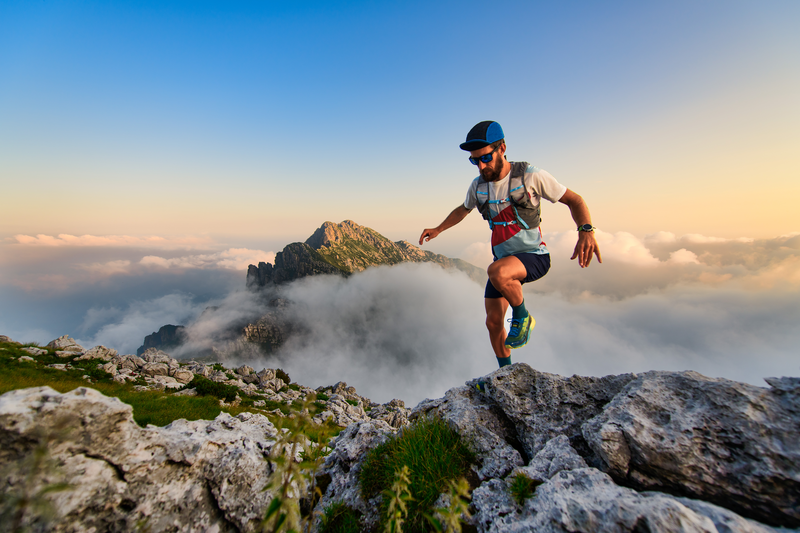
95% of researchers rate our articles as excellent or good
Learn more about the work of our research integrity team to safeguard the quality of each article we publish.
Find out more
ORIGINAL RESEARCH article
Front. Oncol. , 29 November 2022
Sec. Hematologic Malignancies
Volume 12 - 2022 | https://doi.org/10.3389/fonc.2022.1058482
Objective: To investigate the effect of iron overload (IO) on red blood cell (RBC) lifespan in MDS patients with the use of carbon monoxide breath test
Methods: The red blood cell lifespan of 93 patients with myelodysplastic syndrome (MDS) and 22 healthy volunteers in the control group were measured by alveolar gas carbon monoxide (CO) assay, with the detection of liver iron concentration, iron metabolism index, erythropoietin (EPO) concentration, peripheral blood inflammatory cytokines, etc. The MDS patients were divided into the severe IO group, mild IO group and non IO group according to liver iron concentration. The effect of IO on RBC lifespan was analyzed in MDS patients.
Results: The RBC lifespan of MDS patients in the severe IO group was significantly lower than that in the mild IO group (p<0.05), while the RBC life span in the mild IO group was significantly lower than that in the non IO group (p<0.05). The expression of inflammatory cytokines in the severe IO group was significantly higher than that of the mild and non IO groups. After receiving iron removal treatment(ICT), the expression of inflammatory cytokines was decreased significantly, and the RBC lifespan was significantly prolonged (p<0.05).Besides, liver iron concentration was significantly positively correlated with EPO concentration, while EPO concentration was significantly negatively correlated with RBC lifespan, especially in the MDS-RS subgroup. The RBC lifespan in the EPO>1000 group was significantly lower than that in the EPO<1000 group.
Conclusion: IO can shorten RBC lifespan in MDS patients, which may be result from the increase of endogenous EPO and the over-expression of inflammatory cytokines. After ICT, the ineffective hematopoiesis caused by increased EPO may reduced and the decrease of inflammatory cytokine may significantly prolong the RBC lifespan in MDS patients.
Myelodysplastic syndromes (MDS) patients may suffer from iron overload as a result of a longterm transfusion of red blood cells (RBC) and/or combined with abnormal iron metabolism. Most of the causes of anemia in MDS patients are related to ineffective hematopoiesis caused by morbid hematopoiesis. In the process of division and maturation, young RBCs are destroyed or die before they mature and enter the peripheral circulation for some reasons. There is an obvious contradiction between the decrease of peripheral blood cells and the excessive proliferation and apoptosis of bone marrow cells in MDS patients. The increased level of apoptosis may be the main reason for the ineffective hematopoiesis of bone marrow in MDS patients (1). The pathological phenotype of low-risk MDS patients, especially MDS-RA and RARs sub-types, is mainly limited to erythroid cell injury, and their erythroid cell apoptosis begins in the early stage of stem cells. In contrast, the percentage of apoptotic cells in high-risk MDS patients (with too many primordial cells) is lower than that in normal subjects, which is similar to the percentage observed in acute leukemia (2).
In recent years, a new technology of rapid and simple CO breath test for the determination of RBC life span is being applied in clinic (3). The lifespan of RBCs in circulation reflects the quality or intrinsic defect of mature RBC. Under physiological conditions, the production and destruction of RBCs is in dynamic balance. The average life span of RBC in healthy adults is 115 (70 ~ 140) days. In the domestic consensus, while the average life span of RBC in normal people with CO breath test method is 126d, and the 95% confidence interval is 75-177d (4). After excluding hemolysis caused by other reasons, such as autoimmune hemolysis, drug-related hemolysis, PNH, congenital spherocytosis, G-6-PD deficiency, hemoglobinopathy, etc., the lifespan of RBC can be used to evaluate the degree of excessive apoptosis or destruction of RBC.
Erythrocytes which contain neither nucleus nor mitochondria, undergo apoptosis mainly by the increase of cytoplasmic Ca2+ concentration, the increase of reactive oxygen species(ROS), the formation of ceramide and the induction of protein phosphorylation. With the stimulation of ROS, RBCs promote the synthesis and release of platelet activating factor(PAF), then activate and stimulate sphingomyelinases, which lead to the production of ceramide. Further, ceramide activates intracellular invertase, catalyzes the translocation of phosphatidylserine (PS) existing in the inner membrane to the outer membrane, exposes phosphatidylserine, and induces erythrocyte apoptosis (5). Iron overload leads to the production of intracellular free radicals, especially in MDS-RA and RARs subtypes, which leads to oxidative damage and induces apoptosis of hematopoietic progenitor cells (6). In vitro, excessive iron load promotes apoptosis of immature erythrocyte through increased intracellular ROS generation. Erythroid burst forming unit colony and differentiation into mature erythrocytes were significantly inhibited. Iron removal treatment(ICT) can reduce the level of ROS in cells, inhibit apoptosis, and restore the differentiation to mature RBCs (7).
Does IO shorten the life span of RBCs in MDS patient? Have been an improvement in the lifespan of RBC after ICT? Does the high expression of EPO in vivo influence the lifespan of RBC in MDS patients? Do the increased number of bone marrow blasts and the expression of inflammatory cytokines affect the life span of RBC? This study hopes to answer these questions.
This study was conducted in accordance with the Declaration of Helsinki and was approved by the Ethics Committee of our hospital [No.2022-KY-171(K)]. Written informed consent was obtained from all participants.
We retrospectively identified and assessed patients with a consecutive series of MDS from July 2021 to October 2022, and excluded cases who were combined with hemolysis caused by other reasons, such as autoimmune hemolysis, PNH, congenital spherocytosis, G-6-PD deficiency, hemoglobinopathy, etc. After exclusion checks, 93 patients with MDS, 22 healthy volunteers were included in this study. MDS diagnosis was performed in accordance with the 2019 expert consensus (8).Typing was in accordance with the 2016 WHO classification standards (9). IPSS-R scores were used for evaluation and grading (10). The liver iron content in patients with MDS was evaluated by energy spectrum CT. Based on the relevant literature, we defined liver virtual iron content (LVIC) < 1.34(mg/ml) as the normal group, 1.34 ≤ LVIC < 1.85 (mg/ml) as the mild IO group, 1.85 ≤ LVIC < 2.69 (mg/ml) as the moderate IO group, 2.69 ≤ LVIC < 4.03 (mg/ml) as the severe IO group, and 4.03 (mg/ml) ≤ VIC as the extremely severe IO group (11). According to the LVIC, we divided patients with MDS into severe IO group(LVIC classification: severe and extremely severe IO groups), mild IO group(LVIC classification:mild and moderate IO groups)and non IO group(normal group). Healthy volunteers were included into the control group. The clinical characteristics of the patients are shown in Table 1.
The patients with MDS in the low-risk group had taken the drugs of cyclosporine, thalidomide and other immunomodulatory in combination with the hematopoiesis promoting treatments. The patients with MDS in the medium and high-risk group were treated with demethylation (azacytidine or decitabine), while tAML patients were treated with demethylation in combination with Bcl-2 inhibitor (venecla) or allogeneic hematopoietic stem cell transplantation(allo-HSCT). 72 patients with MDS had a history of RBC transfusion, among which 35 patients were complicated with RBC transfusion dependence(TD). The median volume of RBC transfusion in the past year was 16 (4~62) U. Among them, 28 cases who had an expected survival of ≥ 1 year, with the total RBC transfusion of more than 80 U (1 u RBC for red blood cells isolated from 200 ml whole blood), the test of adjusted ferritin (ASF) ≥ 1000 ng/ml and the RBC transfusion dependence received the treatment of iron chelation therapy (ICT) (12): desferriamine mesylate 20-60 mg • kg-1 • d-1 is continuously pumped subcutaneously for 12 hours or desilaros 30 mg • kg-1 • d-1 is taken orally, for 14 days as a course (13). The lifespan of RBC was measured in 16 patients before and after ICT. All patients had more than 4 courses of ICT, of which 12 cases completed 8 courses or more, and 7 cases completed 10 courses or more. There was no history of RBC infusion in healthy volunteers, with ASF <500 ng/ml when RBC lifespan was tested. All MDS patients in this study did not receive mitoxantrone and platinum recently.
We evaluated alveolar endogenous CO concentration by automated instrument(i.e. ELS TESTER, model RBCS-01, Seekya Biotec Co. Ltd, Shenzhen, China) with the used of non-dispersive infrared spectroscopy, and collected the paired samples of room air and alveolar gas for the RBC lifespan measurements conducted by Levitt’s formula (4). The samples of alveolar gas were collected in the morning (8.00– 11.30) without fasting requirement, while smoking patients were asked to quit smoking for 24 hours. After a deep inspiration, each participant held his or her breath for 10’s and then exhaled into a collection system using a mouth-piece (Figure 1A). The collection system discarded the first 300 ml of dead space gas and then directed subsequent alveolar air automatically into a foil collection bag. If needed, the procedure was repeated until the collected air sample reached the collection bags capacity of 1000 ml. The filled bag was detached and sealed. Atmospheric samples were collected just after breath sampling. Alveolar gas and atmospheric samples were stored at room temperature and analyzed within 5 days. On the same day as alveolar gas sampling, peripheral vein blood samples were collected for routine hemoglobin measurement.The working flow chart of gas circuit of measuring instrument is illustrated in Figure 1B. The instrument operator connected the paired air-alveolar gas samples to inlets, entered the participants blood hemoglobin concentration data, and then pressed the start button, triggering the instrument to complete a series of automated measurements encompassing the following steps. First, the quality of a collected alveolar sample was checked by measuring its CO2 concentration. Only alveolar gas samples containing <5% CO2 were considered to be diluted. Second, in order to eliminate molecules that might interfere with the infrared detection of CO, the sample gas was advanced into a de-interference system with an absorbent mixture consisting mainly of soda asbestos. The absorbed interference molecules were mainly H2O and CO2. Third, a paired measurement technique was employed to determine the CO concentration difference between alveolar gas and atmospheric air. Fourth, any endogenous alveolar samples found to be diluted (i.e. having <5% CO2) were normalized to a 5% CO2 status. Any endogenous alveolar sample in which dilution was found (i.e., CO2 content <5%) was normalized to the state of 5% CO2. Finally, the lifespan of RBC was calculated by Levitt’s formula (14) based on the parameters of the pre-imputed hemoglobin concentration and corrected (if necessary) endogenous alveolar CO concentration. The instrument reports the following data for the subject: alveolar CO2, endogenous alveolar CO, and RBC lifespan.
Figure 1 Experimental set up. (A) Breath sample collecting system. (B) Overview of measurement instruments structure.
Serum ferritin (SF) and erythropoietin (EPO) were determined by radioimmunoassay. Serum iron and total iron binding capacity were detected by ferrous nitrogen colorimetry. C-reactive protein (CRP) was detected by immunoturbidimetry. When CRP > 10 mg/l, ASF = sf/log 10 CRP and CRP ≤ 10 mg/l, ASF = SF (15), the data of SF and CRP was collected on the same day.
The same 2 × 192-layer DECT scanner (Siemens SOMATOM Force) was used for all examinations. The detection protocol used dual energy (De, 100 and 150 kvp) with a thin filter to improve the separation of the two spectra. The image data was processed with the software prototype (DE IronVNC; syngo.via Frontier; Siemens Healthineers). The liver map was made by decomposing the basic materials into air, water, and iron. Skilled CT diagnosis radiologists performed the image analysis. The VIC of the liver was measured by placing three large freehand regions of interest(ROIs) at the hepatic vault and about 1 cm below the hepatic capsule, excluding the larger vessels near the portal vein and inferior vena cava. Moreover, ROIs included the left lobe and the anterior part of the right lobe.
Interleukin(IL)-6, IL-10, Tumor necrosis factor (TNF)-α, Interferon (IFN)-y, IL-17A,IL-1β, IL-12p70, IFN-a, IL-8, IL-2, IL-4 and IL-5 in peripheral blood were detected by ELISA. The detection was carried out in strict accordance with the product instructions. None of the patients had infectious fever at the time of sampling.
SPSS 19.0 was used for statistical analysis. The normal distribution data was expressed as “mean ± standard deviation”. The independent sample t-test was employed for the comparison of the two groups of data, and the paired t-test was used for the data before and after ICT.The data with non normal distribution was expressed in the median. Mann Whitney U test was used for the comparison between the two groups. Wilcoxon test was used for the comparison between the two groups before and after treatment, while Spearman analysis was applied for correlation analysis, in which P < 0.05 indicated that the difference was statistically significant.
In the MDS group, 83.9% of patients develop anemia and 87.1% of patients suffer from the lifespan shortening of RBC, while in the control group, none of patients develop anemia and the lifespan of RBC was all within the normal range(>75d). The correlations between hemoglobin and CO concentration, hemoglobin and RBC life span are shown in Figure 2. The effect of iron overload on the life span of RBC and the changes of RBC life span after ICT in MDS patients are shown in Figure 3. The expression of inflammatory cytokines in peripheral blood and the changes after ICT in MDS patients are shown in Figure 4. The correlation between EPO concentration and RBC lifespan and the differences of RBC lifespan in the EPO<1000 and EPO >1000 mIU/ml group among different MDS subgroups are shown in Figure 5. The correlation between the number of bone marrow primordial cells and the lifespan of RBC in the high-risk group MDS patients, and the effect of demethylation treatment on the lifespan of RBC in the high-risk group MDS patients are shown in Figure 6. In 5 tAML patients, the lifespan of RBC has been decreased during the treatment of demethylated and Bcl-2 inhibitor. However, after the recovery of hematopoietic, the lifespan of RBC has been increased. Since the number of cases is too small, the effect of Bcl-2 inhibitor on RBC life span cannot be determined.
Figure 2 There were 115 cases in the MDS group and the control group. The correlation analysis results showed that: (A) The hemoglobin was significantly positively correlated with the RBC life span(r=0.8103, p<0.0001), (B) The hemoglobin was significantly negatively correlated with the concentration of CO (r=-0.3287, p=0.0003).
Figure 3 (A) The RBC life span of mild IO group was significantly lower than that in non IO group (p<0.01); and the severe IO group was significantly lower than that of the mild IO group (p<0.01); and the non 10 group was significantly lower than that of the control group (p<0.01). (B) 16 MDS patients in IO group were tested for RBC life span before and after ICT. The results showed that the RBC life span in 10 group increased significantly after ICT (p<0.01).
Figure 4 (A) 15 MDS patients with severe IO, 10 MDS patients with severe IO but after ICT, and 32 MDS patients with mild IO received peripheral blood cytokine detection. IL-6 (12.3 ± 4.8 vs 0.9±0.3), IL-10 (9.9 ± 3.3 vs 0.4 ± 0.3), TNF-α (10.2±4.1 vs 1.3±0.6). IFN-y (7.8±3.2 vs 0.6±0.3), IL-17A (35.4±12.5 vs 0.8±0.3), IL-1β (5.6 ± 2.1 vs 2.1±1.5), IL-12p70 (11.2 ± 3.7 vs 1.4±0.5), IFN-a (13.5±4.8 vs 1.3±0.5) and IL-8 (27.8 ± 11.5 vs 2.1 ± 1.2) in severe IO group were significantly higher than those in mild IO group (*p<0.05), while in severe IO group but with regular ICT, IL-6 (12.3±4.8 vs 6.6±2.5), IL-10 (9.9±3.3 vs 4.1±2.8), TNF-α (10.2±4.1 vs 3.8±1.4). IFN-y (7.8±3.2 vs 2.7±1.4), IL-17A (35.4±12.5 vs 18.9±7.9), IL-1β (5.6±2.1 vs 2.6±1.4), IL-12p70 (11.2±3.7 vs 3.1±1.2), IFN-a (13.5±4.8 vs 4.742.1) and IL-8 (27.8±11.5 vs 16.748.9) were significantly lower than those in severe 10 patients without ICT (*p<0.05); However, there was no significant difference in the expression of IL-2, IL-4 and IL-5 (#p>0.05). (B) 12 MDS patients with severe IO tested those cytokines before and after ICT. After ICT, IL-6 (16.5±7.6 vs 5.8 ± 2.6), IL-10 (11.5±4.3 vs 4.7±3.1), TNF-α (13.2±5.4 vs 4.1±1.7). IFN-y (9.6±5.3 vs 2.9±1.7), IL-17A (41.6±16.8 vs 16.5±8.6), IL-1B The expression of cytokines such as (5.8±2.5 vs 2.3±1.6), IL-12p70 (12.9±3.8 vs 2.8±1.4), IFN-a (16.8±5.7 vs 4.2±2.6) and IL-8 (35.2±13.5 vs 15.1 ± 9.6) was significantly lower than before (*p<0.05), and there was no significant difference in the expression of IL-2, IL-4 and IL-5 (#p>0.05).
Figure 5 27 MDS patients in non IO group and 36 MDS patients in IO group who were not use EPO within one month were included in the analysis. There were 12 cases in MDS-RS and 51 cases in non MDS-RS subgroup. The results showed that: (A) the RBC life span in EPO>1000mlU /ml was significantly lower than that of EPO<1000mlU/ml (50.81±29.29 vs 26.23±15.87, p<0.001). (B) There was a significant positive correlation between liver VIC and EPO concentration (r=0.4931, p<0.001). In MDS-RS subgroup, (C) EPO concentration in severe IO group was significantly higher than that in mild IO group (6802.50 ± 2709.02 vs 934.22 ± 763.49, p<0.001), (D) and EPO concentration was significantly negatively correlated with RBC life span (r=-0.8077, p<0.01). In the non MDS-RS subgroup, (E) EPO concentration in severe 10 group was also significantly higher than that in the mild IO group (9290.09±8638.86 vs 1050.10 ± 930.81, p<0.001), (F) and EPO concentration was significantly negatively correlated with RBC life span (r=-0.3754, p<0.01).
Figure 6 34 high-risk MDS and tAML patients(except 6 patients who underwent allogeneic stem cell transplantation) were included in the analysis. (A) There was no significant correlation between the number of bone marrow primordial cells and RBC life span (r=-0.1347, p=0.4474). (B) 23 patients with medium and high-risk MDS patients were detected RBC life span before and after demethylation drug treatment. It shows that demethylation treatment has no significant effect on the RBC life span (p=0.711).
70% of the endogenous CO in human body comes from the degradation of Hb after the destruction of RBCs, and the lung is the only way for the body to excrete CO. One mole of Hb is degraded to produce four moles of CO. The amount of Hb degradation in 24h can be calculated by measuring the endogenous CO concentration in alveolar gas. The formula that the total amount of Hb in the whole body is divided by the amount of Hb degradation in 24h is used to estimate the value of erythrocyte life in the detection period. The decomposition degree of RBCs in the body can be indirectly estimated by detecting the concentration of CO. Thus, the high concentration of CO can infer the high heme produced by the decomposition of RBCs. This study shows that the amount of hemoglobin in MDS patients is significantly negatively correlated with carbon monoxide (CO) concentration and is significantly positively correlated with the life span of RBC. The life span of RBC in MDS patients is significantly lower than that of the control group. For MDS patients, the shortening lifespan of RBC indicates the high rate of erythrocyte decomposition, which may be corresponds to the high degree of erythrocyte damage or apoptosis.
The life span of RBC in the severe IO MDS group is significantly lower than that in the mild IO MDS group and the non IO MDS group. After ICT, the lifespan of RBC in the IO patients are increased significantly. These results indicate that the IO can shorten the lifespan of RBC in MDS patients, which may be relevant to the aggravation of erythrocyte damage or apoptosis in patients.IO can cause the increase of reactive oxygen species (ROS) in the body. Previous studies have shown that the increase of reactive oxygen species production and oxidative stress in mouse models affects the expression of FoxO transcription factor, p38 mitogen activated protein kinase (MAPK), ataxia telangiectasia mutant (ATM) protein and other signal pathways. These changes are related to the loss of hematopoietic stem cells. Through these pathways, the signaling of reactive oxygen species may be involved in regulating the proliferation and apoptosis of normal and tumor hematopoietic progenitor cells (16). Previous studies in our center also shows that iron overload can promote erythroid cell apoptosis in patients with MDS by regulating HIF-1a/ROS signaling pathway (17), and promote mitochondrial breakage of mesenchymal stromal cells in patients with MDS by activating ampk/mff/drp1 pathway (18).
The reaction of erythroid system to tissue hypoxia is the pathophysiological basis of ineffective erythropoiesis. Tissue hypoxia increases the expression of erythropoietin (EPO), which induces the formation of new erythrocytes. In anemia cases related to ineffective erythropoiesis, the imbalance between red blood cell supply and demand still exists, in spite of tissue hypoxia and increased EPO. Therefore, patients with ineffective erythropoiesis are usually accompanied by too many young erythrocytes in their bone marrow (19). This study shows that the life span of RBC in MDS patients with EPO>1000 mIU/ml is significantly lower than that in MDS patients with EPO<1000 mIU/ml, which indicates that the life span of RBC in MDS patients with high EPO concentration decreases more seriously. This may be caused by that excessive concentration of endogenous EPO in MDS patients stimulates a large number of erythroid hyperplasia, and excessive ineffective hematopoiesis shortens the overall life expectancy of RBC. Moreover, there was a significant positive correlation between liver iron concentration and EPO. It suggests that iron overload affects the expression of endogenous EPO in MDS patients. Our previous research results show that heavy iron overload will promote the increase of endogenous EPO but affect the expression of EPO-stat5 pathway and inactivate EPO signal pathway (20). EPO concentration decreased after ICT treatment (21). It is speculated that the reason may be related to the increase of EPO production due to the feedback stimulus caused by blocked EPO signal.
The subgroup analysis shows that EPO concentration is significantly negatively correlated with RBC lifespan in both MDS-RS and MDS non RS groups. The expression of EPO in the severe IO group was significantly higher than that in the mild IO group. The correlation between EPO and RBC lifespan in the MDS-RS subgroup is significantly higher than that in the MDS non RS group. It indicates that Iron overload leads to the increase of endogenous EPO expression, and the increase of EPO leads to the increase of erythroblast and ineffective hematopoiesis, which is one of the reasons for shortening the life span of RBC in MDS patients.
In high-risk MDS patients, there is no significant correlation between the number of primordial cells and RBC lifespan. It shows that in MDS patients, bone marrow invasion of tumor cell leads to the function of impaired hematopoietic, which mainly affects the number of red blood cells but has no significant impact on RBC life.
Treatment related anemia has always been an unavoidable problem in the treatment of cancer patients. Lang et al. (22) shows that in addition to bone marrow inhibition, mitoxantrone and platinum in chemotherapy drugs can also promote the decline of red blood cells, resulting in obvious anemia and shortened red blood cell lifespan. This experiment shows that the treatment of demethylation only causes bone marrow suppression, but there is no significant difference in the lifespan of red blood cells before and after treatment. The change of erythrocyte lifespan is related to the remission of the disease. The treatment of demethylation has no significant effect on the lifespan of erythrocyte. However, the number of cases is small, and the effect of Bcl-2 inhibitor needs to be further clarified by increasing the number of cases.
Our study showes that the expressions of inflammatory cytokines IL-6, TNF-α, IFN-y, IL-17A, IL-1β, IL-12p70, IFN-a and IL-8 in peripheral blood of MDS IO group are significantly higher than those in the non IO group. It has been proved that iron overload could induced M1 polarization in RAW 264.7 macrophages, which is associated with the induction of ROS production in iron-treated cells with the characterization of elevated TNF (23). M1 macrophage polarization caused by Th1 cytokines, such as TNF and IFN-γ produce majorly proinflammatory cytokines, such as TNF, IL-1 α, IL-1 β, IL-6, IL-12, IL-23 and low levels of IL-10. The pro-inflammatory activity of these macrophages promote DNA damage by the release of intracellular free radicals (6, 24). Apoptosis triggered by bone marrow micro-environment and/or intrinsic cell is also regulated by different levels of cytokines. Inflammatory cytokines also reduce the sensitivity of EPO (25). ROS induces heme oxygenase-1 (HO-1) expression (26). HO-1 is an enzyme that catalyzes the degradation of heme and releases equimolar amounts of carbon monoxide (CO), biliverdin (BV) and Fe2 +. HO-1 and its products CO and BV are generally related to promote the antioxidant, anti-inflammatory and immunosuppressive activities of macrophages (27). The inhibition of HO-1 activity blocks the expression of IL-10 in LPS-stimulated mouse macrophages (28). IL-10 is an inflammatory inhibitor. Our study detects that the expression of IL-10 in IO group is increased, which may be related to the increased expression of HO-1. However, after the degradation of HO-1-mediated heme, the release of Fe2+ shows a pro-oxidative effect and cytotoxicity (29), may aggravate the in situ hemolysis and shorten the RBC lifespan in MDS patients.These inflammatory cytokines were decreased significantly after ICT. Regular ICT treatment can significantly reduce the expression of these inflammatory cytokines even in MDS patients with a severe increase of LVIC. The decrease of inflammatory factors may also be one of the reasons why the lifespan of RBC is prolonged.
In conclusion, iron overload is significantly shortens the lifespan of RBC in MDS patients. The shortening of RBC lifespan is not equal to the increase of ineffective hematopoiesis,but iron overload causes the increase of endogenous EPO expression, which leads to the increase of erythroblasts. The increase of ineffective hematopoiesis may be one of the reasons for shortening the lifespan of erythrocytes in MDS patients. The increase of inflammatory cytokines caused by iron overload may damage the erythroblast of MDS combined with morbid hematopoiesis, which is also the reason for shortening the lifespan of erythrocytes.Iron removal therapy can prolong the lifespan of RBC by reducing the production of endogenous EPO and the expression of inflammatory cytokines. Deficiencies of this study: the number of cases in the whole and each subgroup is small, and the influence of erythrocyte transfusion before RBC lifespan testing cannot be completely excluded, so it is best to conduct a large sample of prospective ROT experiments to further confirm.
The raw data supporting the conclusions of this article will be made available by the authors, without undue reservation.
The studies involving human participants were reviewed and approved by Ethics Committee of Shanghai Sixth People’s Hospital. The patients/participants provided their written informed consent to participate in this study.
YZ: Experimental design, statistical analysis and thesis writing. YQ: Collection and arrangement of experimental data. L-XS and CX: Assist to collect and arrange experimental data. C-KC: Provide the overall idea and thesis modification. All authors contributed to the article and approved the submitted version.
The authors declare that the research was conducted in the absence of any commercial or financial relationships that could be construed as a potential conflict of interest.
All claims expressed in this article are solely those of the authors and do not necessarily represent those of their affiliated organizations, or those of the publisher, the editors and the reviewers. Any product that may be evaluated in this article, or claim that may be made by its manufacturer, is not guaranteed or endorsed by the publisher.
1. Invernizzi R, Travaglino E. Increased apoptosis as a mechanism of ineffective erythropoiesis in myelodysplastic syndromes. Clin Leuk (2008) 2:113–20. doi: 10.3816/CLK.2008.n.014
2. Invernizzi R, Travaglino E, Lunghi M, Klersy C, Bernasconi P, Cazzola M, et al. Survivin expression in acute leukemias and myelodysplastic syndromes. Leuk Lymph (2004) 45:2229–37. doi: 10.1080/10428190412331283251
3. Gao Q, Ye L, Zhang F. Clinical application and significance of the technique detecting the lifespan of red blood cells. Chin J Hematol (2019) 40(5):447–8. doi: 10.3760/cma.j.issn.0253-2727.2019.05.021
4. Zhang HD, Ma YJ, Liu QF, Ye TZ, Meng FY, Zhou YW, et al. Human erythrocyte lifespan measured by levitt's CO breath test with newly developed automatic instrument. J Breath Res (2018) 12(3):036003. doi: 10.1088/1752-7163/aaacf1
5. Lang PA, Kempe DS, Tanneur V, Eisele K, Klarl BA, Myssina S, et al. Stimulation of erythrocyte ceramide for mation by platelet activating factor. J Cell Sci (2005) 118(6):1233 –1243. doi: 10.1242/jcs.01730
6. Pham CG, Bubici C, Zazzeroni F, Papa S, Jones J, Alvarez K, et al. Ferritin heavy chain upregulation by NF-kappaB inhibits TNF alpha induced apoptosis by suppressing reactive oxygen species. Cell (2004) 119:529–42. doi: 10.1016/j.cell.2004.10.017
7. Taoka K, Kumano K, Nakamura F, Hosoi M, Goyama S, Imai Y, et al. The effect of iron overload and chelation on erythroid differentiation. Int J Hematol (2011) 95(2):149–59. doi: 10.1007/s12185-011-0988-3
8. Chinese Society of Hematology. Chinese Medical association. Chinese guidelines for diagnosis and treatment of myelodysplastic syndromes. Chin J Hematol (2019) 40(2):89–97. doi: 10.3760/cma.j.issn.0253-2727
9. Arber DA, Orazi A, Hasserjian R, Thiele J, Borowitz MJ, Le Beau MM, et al. The 2016 revision to the world health organization classification of myeloid neoplasms and acute leukemia. Blood (2016) 127:2391–405. doi: 10.1182/blood-2016-03-643544
10. Greenberg PL, Tuechler H, Schanz J, Sanz G, Garcia-Manero G, Solé F, et al. Revised international prognostic scoring system for myelodysplastic syndromes. Blood (2012) 120:2454–65. doi: 10.1182/blood-2012-03-420489
11. Zhang Y, Xiao C, Li J, Song LX, Zhao YS, Han S, et al. Comparative study on iron content detection by energy spectral CT and MRI in MDS patients. Front Oncol (2021) 11:646946. doi: 10.3389/fonc.2021.646946
12. Malcovati L, Porta MG, Pascutto C, Invernizzi R, Boni M, Travaglino E, et al. Prognostic factors and life expectancy in myelodysplastic syndromes classified according to WHO criteria: a basis for clinical decision making. J Clin Oncol (2005) 23(30):7594–7603. doi: 10.1200/JCO.2005.01.7038
13. Gattermann N. Guidelines on iron chelation therapy in patients with myelodysplastic syndromes and transfusional iron overload. Leuk Res (2007) 31 Suppl 3:S10–15. doi: 10.1016/S0145-2126(07)70461-7
14. Furne JK, Springfield JR, Ho SB, Levitt MD. Simplification of the end-alveolar carbon monoxide technique to assess erythrocyte survival. J Lab Clin Med (2003) 142(1):52–7. doi: 10.1016/S0022-2143(03)00086-6
15. Armand P, Kim HT, Rhodes J, Sainvil MM, Cutler C, Ho VT, et al. Iron overload in patients with acute leukemia or MDS undergoing myeloablative stem cell transplantation. Biol Blood Marrow Transpl (2011) 17(6):852–60. doi: 10.1016/j.bbmt.2010.09.006
16. Ghaffari S. Oxidative stress in the regulation of normal and neoplastic hematopoiesis. Antioxid Redox Signal (2008) 10:1923–40. doi: 10.1089/ars.2008.2142
17. Zheng Q-q, Zhao Y-s, Guo J, Zhao S-d, Song L-x, Fei C-m, et al. Iron overload promotes erythroid apoptosis through regulating HIF-1a/ROS signaling pathway in patients with myelodysplastic syndrome. Leuk Res: A Forum Stud Leuk Normal Hemopoiesis (2017) 58:55–62. doi: 10.1016/j.leukres.2017.04.005
18. Zheng Q, Zhao Y, Guo J, Zhao S, Fei C, Xiao C, et al. Iron overload promotes mitochondrial fragmentation in mesenchymal stromal cells from myelodysplastic syndrome patients through activation of the AMPK/MFF/Drp1 pathway. Cell Death Dis (2018) 9(5):515. doi: 10.1038/s41419-018-0552-7
19. Camaschella C, Nai A. Ineffective erythropoiesis and regulation of iron status in iron loading anaemias. Br J Hematol (2016) 172(4):512–23. doi: 10.1111/bjh.13820
20. Zhang Y. Effect of iron chelation therapy on EPO-STAT5signalling pathway and EPO resistance in iron overloaded low-risk myelodysplastic syndrome patients. Hematology (2020) 25:1, 1–10. doi: 10.1080/16078454.2019.1700330
21. Zhang Y, Xiao C, Li J, Song LX, Zhao YS, Zhao JG, et al. Influencing factors of iron metabolism assessment in patients with myelodysplastic syndrome: A retrospective study. Chin J Hematol (2022) 43(4):293–9. doi: 10.3760/cma.j.issn.0253-2727.2022.04.005
22. Lang E, Bissinger R, Qadri SM, Lang F. Suicidal death of erythrocytes in cancer and its chemotherapy: A potential target in the treatment of tumor-associated anemia. Int J Cancer (2017) 141(8):1522–8. doi: 10.1002/ijc.30800
23. Zhou Y, Que KT, Zhang Z, Yi ZJ, Zhao P, Yu Y, et al. Iron overloaded polarizes macrophage to proinflammation phenotype through ROS/acetyl-p53 pathway. Cancer Med (2018) 7:4012–22. doi: 10.1002/cam4.1670
24. Sindrilaru A, Peters T, Wieschalka S, Baican C, Baican A, Peter H, et al. An unrestrained proinflammatory M1 macrophage population induced by iron impairs wound healing in humans and mice. J Clin Investig (2011) 121:985–97. doi: 10.1172/JCI44490
25. Musto P, Matera R, Minervini MM, Checchia-de Ambrosio C, Bodenizza C, Falcone A, et al. Low serum levels of tumor necrosis factor and interleukin-1 β in myelodysplastic syndromes responsive to recombinant erythropoietin. Haematologica (1994) 79:265–8.
26. Srisook K, Kim C, Cha YN. Molecular mechanisms involved in enhancing HO-1 expression: De-repression by heme and activation by Nrf2, the “one-two” punch. Antioxid Redox Signal (2005) 7:1674–87. doi: 10.1089/ars.2005.7.1674
27. Costa DL, Amaral EP, Andrade BB, Sher A. Modulation of inflammation and immune responses by heme oxygenase-1: Implications for infection with intracellular pathogens. Antioxidants (2020) 9:1205. doi: 10.3390/antiox9121205
28. Lee TS, Chau LY. Heme oxygenase-1 mediates the anti-inflammatory effect of interleukin-10 in mice. Nat Med (2002) 8:240–6. doi: 10.1038/nm0302-240
Keywords: MDS, erythrocyte lifespan, EPO, cytokines, iron removal therapy
Citation: Zhang Y, Qian Y, Song L-x, Xiao C and Chang C-k (2022) The use of carbon monoxide breath test to detect the effect of iron overload on erythrocyte lifespan in MDS. Front. Oncol. 12:1058482. doi: 10.3389/fonc.2022.1058482
Received: 30 September 2022; Accepted: 07 November 2022;
Published: 29 November 2022.
Edited by:
Tadeusz Robak, Medical University of Lodz, PolandReviewed by:
Li Bao, Beijing Jishuitan Hospital, ChinaCopyright © 2022 Zhang, Qian, Song, Xiao and Chang. This is an open-access article distributed under the terms of the Creative Commons Attribution License (CC BY). The use, distribution or reproduction in other forums is permitted, provided the original author(s) and the copyright owner(s) are credited and that the original publication in this journal is cited, in accordance with accepted academic practice. No use, distribution or reproduction is permitted which does not comply with these terms.
*Correspondence: Chun-kang Chang, Y2hhbmdjaHVua2FuZ0BzanR1LmVkdS5jbg==
†These authors have contributed equally to this work
Disclaimer: All claims expressed in this article are solely those of the authors and do not necessarily represent those of their affiliated organizations, or those of the publisher, the editors and the reviewers. Any product that may be evaluated in this article or claim that may be made by its manufacturer is not guaranteed or endorsed by the publisher.
Research integrity at Frontiers
Learn more about the work of our research integrity team to safeguard the quality of each article we publish.