- Department of Gastroenterology, The Affiliated Hospital of Qingdao University, Qingdao, China
Chronic pancreatitis (CP) is a chronic progressive inflammatory disease of the pancreas, caused by multiple factors and accompanied by irreversible impairment of pancreatic internal and external secretory functions. Pathologically, atrophy of the pancreatic acini, tissue fibrosis or calcification, focal edema, inflammation, and necrosis are observed. Clinical manifestations include recurrent or persistent abdominal pain, diarrhea, emaciation, and diabetes. In addition, CP is prone to develop into pancreatic cancer(PC) due to persistent inflammation and fibrosis. The disease course is prolonged and the clinical prognosis is poor. Currently, clinical treatment of CP is still based on symptomatic treatment and there is a lack of effective etiological treatment. Encouragingly, experiments have shown that a variety of active substances have great potential in the etiological treatment of chronic pancreatitis. In this paper, we will review the pathogenesis of CP, as well as the research progress on anti-inflammatory and anti-fibrotic therapies, which will provide new ideas for the development of subsequent clinical studies and formulation of effective treatment programs, and help prevent CP from developing into pancreatic cancer and reduce the prevalence of PC as much as possible.
1 Introduction
Chronic pancreatitis (CP) is a pathological fibro-inflammatory disease of the pancreas that is characterized by persistent pathological reactions to internal and external injury or stress, resulting in intractable abdominal pain, pancreatic exocrine and endocrine dysfunction, decreased quality of life, and reduced life expectancy (1). The incidence and prevalence of CP are increasing, and its clinical prognosis is poor (2–4). In the world, the prevalence of CP is relatively stable, and over time, it has remained at about 35-100 per 100000 adults. The incidence rate increases year by year, about 5/100000 person years, and the incidence rate of males is twice as high as that of females (5). Among Asian countries, the statistical data in 2011 showed that the incidence rate in Japan was 14.0/100000 people, and the prevalence rate was 52.4/100000 people. The prevalence of CP in India is the highest in the world at 125/100000 people. In China, the prevalence rate of chronic pancreatitis was about 13.5/100000 people in 2003, and it was increasing year by year. At present, the latest incidence and prevalence of CP are under investigation (6). It is not clear whether the increase in epidemiological data really reflects a higher disease burden or simply a higher sensitivity of diagnostic tests (7).
CP is systematically classified according to the etiology of toxic, idiopathic, hereditary, autoimmune, recurrent, and obstructive pancreatitis (8). Some CP patients have acute pancreatitis, which is the initial event that starts the inflammatory process (9, 10). Subsequently, multiple risk factors such as pancreaticobiliary disease, alcohol, and smoking aggravate and maintain the inflammatory response of the pancreas. The pathogenesis of some idiopathic pancreatitis is often related to genetic variation, autoimmunity and other factors. In China, pancreaticobiliary obstruction is the main cause of CP (11, 12). In Western countries and Japan, 40–70% of CP cases are attributed to alcohol abuse (13). Alcohol metabolites directly damage acinar cells and play a role in promoting and maintaining chronic inflammation and fibrosis of the pancreas in CP (14). Smoking is an independent pathogenic factor of CP, with risk increasing in a dose-dependent manner (15).Another study have pointed out that the clinical characteristics of smoking related CP are different from CP with other etiologies. An independent chronic pancreatitis subtype should be added, which is caused by smoking related factors and has different characteristics from idiopathic pancreatitis or alcoholic pancreatitis (16).
The pathogenesis of CP is complex and involves changes in multiple protease- related genes. Loss of chymotrypsin C function and pancreatic secretory trypsin inhibitor (product of the SPINK1 gene) mutations increase the risk of CP (17, 18). PRSS1 (encoding cationic trypsinogen) mutation can reduce the risk of CP (19–21). The histopathological manifestations of the pancreas in patients with advanced CP are infiltration of inflammatory cells, deposition of extracellular matrix (ECM), atrophy of acinar structure, and replacement of fibrotic tissue, which gradually aggravate the clinical symptoms of CP and become an important reason for the poor prognosis of patients.
Pancreatic cancer (PC) is an important cause of cancer death, causing a serious health burden worldwide. CP has been proved to be a risk factor for PC. The persistent inflammation and fibrosis of CP are likely to develop into precancerous lesions of pancreatic cancer and eventually lead to pancreatic cancer. Therefore, it is very meaningful to intervene the disease at the early stage of CP to prevent its progression and deterioration.
At present, the main clinical treatment for CP is to relieve symptoms and prevent related complications. No specific treatment is available for the progression of CP (22, 23). However, in vivo and in vitro tests have shown that various active substances have achieved good results in targeting the etiology of CP. This paper summarizes the pathogenesis of inflammatory reactions and fibrosis during the course of CP, as well as the research progress on anti-inflammatory and anti- fibro tic treatments, in the hopes of promoting subsequent clinical research and formulate effective treatment plans.
2 Mechanism of CP inflammation and fibrosis and its development to PC
Inflammation and fibrosis of pancreas run through the whole course of CP. The activation of trypsin and other proteases, as the initial step of CP, damages acinar cells, further leading to the infiltration of multiple inflammatory cells such as macrophages (24). Immunocytes produce various types of pro-inflammatory cytokines that initiate the activation of pancreatic stellate cells. Recent studies have shown that the pathogenesis of CP involves changes in multiple protease- related genes, and inflammation and fibrosis of the pancreas are dynamic pathophysiological processes involving the regulation of multiple signaling pathways. Among them, pancreatic acinar cells, macrophages, and pancreatic stellate cells (PSCs) are key cells that jointly regulate the inflammatory and fibrotic processes of CP (25–27) (Figure 1).
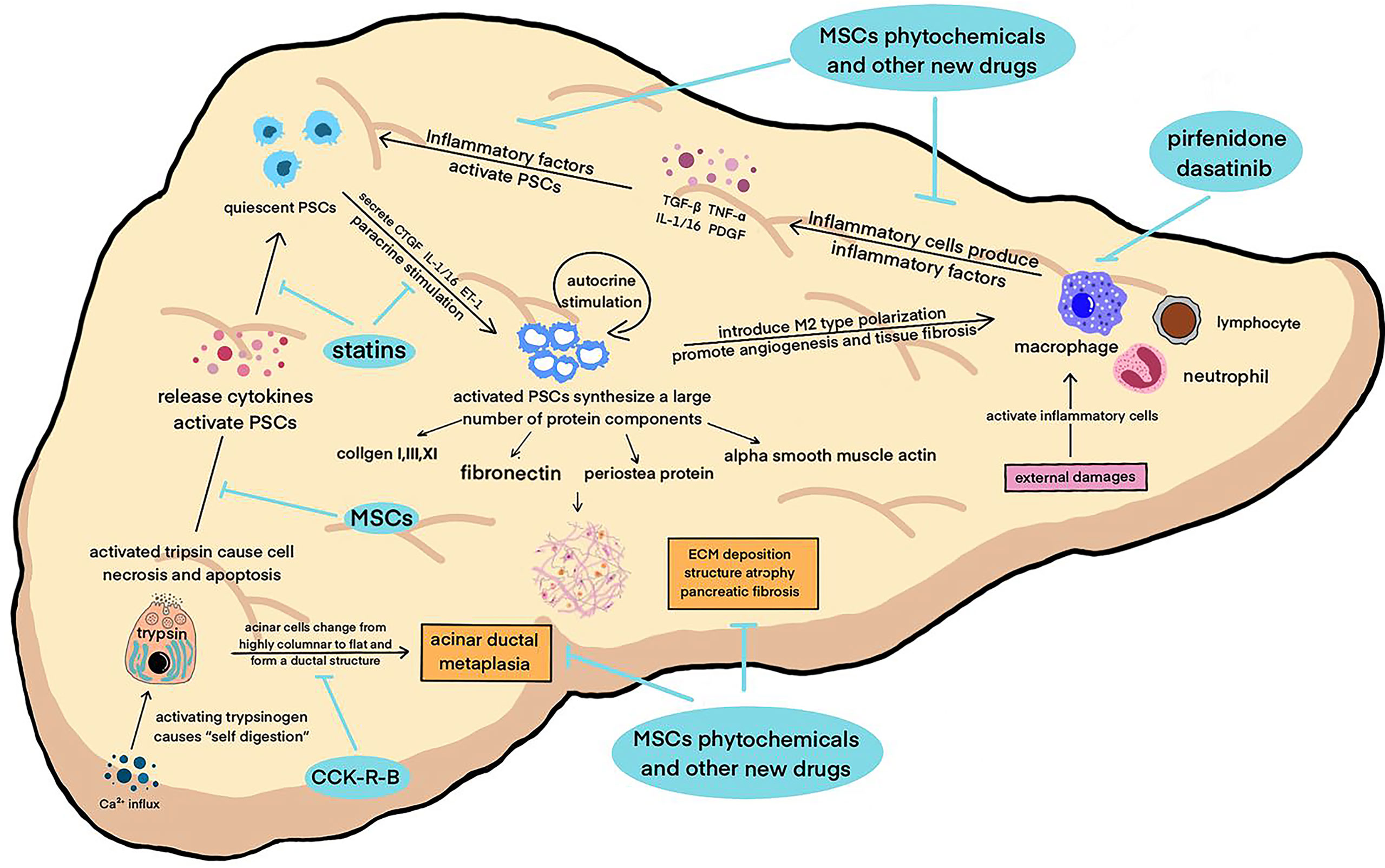
Figure 1 A variety of pathogenic factors damage the pancreas, activate macrophages and other inflammatory cells to secrete inflammatory factors, activate pancreatic enzymes in acinar cells at the same time, start “self digestion”, and lead to necrosis and apoptosis of tissue cells and the destruction of pancreatic structure. These factors work together to activate PSCs, which in turn can secrete inflammatory factors, aggravate the inflammatory reaction and cause a vicious circle. Activated PSCs can secrete smooth muscle protein, collagen fibers and other fibrosis mediators, leading to ECM deposition and pancreatic tissue fibrosis. The above pathophysiological processes continued to promote the progress of CP.
2.1 PSCs play an important role in pancreatic fibrosis
Located in the periacinar region, with long cytoplasmic processes extending to the basolateral side of acinar cells, quiescent PSCs can maintain the integrity of pancreatic acinar cells and stabilize the synthesis and degradation of the ECM (28). Pancreatic injury can promote acinar cells, macrophages, and neutrophils to secrete inflammatory factors, such as transforming growth factor β (TGF-β), tumor necrosis factor α (TNF-α), Interleukin-1/16 (IL-1/16), and platelet-derived growth factor (PDGF), which rapidly activate PSCs (29). After being activated, PSCs can also secrete connective tissue growth factor (CTGF), IL-1/16, and endothelin-1 (ET-1), and then further promote the activation of PSCs through autocrine and paracrine signaling, which forms a vicious cycle (26). At present, TGF-β is considered the inflammatory factor with the strongest activation effect on PSCs. This effect is mainly achieved by regulating the extracellular signal- regulated protein kinase (ERK) and Smad2/3 signaling (30, 31). In addition, this mechanism also influences the phosphorylation level of three subtypes of the MAPK family, including c-Jun amino- terminal kinase (JNK), p38, and ERK (32–34).
Activated PSCs are characterized by the loss of perinuclear lipid droplets and active mitosis, and the endoplasmic reticulum is in a highly stressed state, showing high motility and contractility (35). The expression of multiple cytokine (e.g., PDGF and IL-1/16) receptors increases, and a large number of protein components constituting the ECM are synthesized, including collagen I, III, and XI fibronectin, and periosteal protein. PSCs develop a myofibroblast- like phenotype and express alpha smooth muscle actin (α-SMA), an activation marker. A large number of the ECM is deposited and degraded. These effects work together and gradually lead to pancreatic fibrosis (36–38).
2.2 Pancreatic acinar cells participate in the pathophysiological process of CP from multiple aspects
Researchers have proposed that intracellular calcium overload activates the NF- κβ signaling pathway, which then interacts with multiple signaling pathways to activate trypsinogen (39). Trypsin activation starts the “self- digestion” of acinar cells, and plasma membrane permeability is enhanced (24). Trypsin and other digestive enzymes cause necrosis and apoptosis of surrounding cells and destroy normal pancreatic tissue structure, resulting in pancreatic tissue atrophy (40). The dysfunction of acinar cells is another important factor in inducing the pathological response of CP, in addition to apoptosis and necrosis. A previous study confirmed that after the autophagic flow of acinar cells was destroyed, trypsinogen was activated and a large number of cytokines were released, which promoted PSC activation and initiated the process of fibrosis (41). At the same time, acinar cells in pancreatic tissue with CP gradually change from highly columnar to flat and form a ductal structure. This reaction is called acinar ductal metaplasia (ADM). Metaplastic acinar cells highly express cytokeratin (CK), and their molecular phenotype is very similar to that of pancreatic intraepithelial neoplasia (PanIN) cells. ADM is not only a promoter of pancreatic fibrosis but also a manifestation of it. It is considered an early event in pancreatic ductal adenocarcinoma (PDAC) prior to PanIN (42–45).
2.3 Inflammatory cells, such as macrophages, initiate and exacerbate inflammation and fibrosis in CP
The histopathological feature of CP is the infiltration of lymphocytes, neutrophils and macrophages, among which macrophages are considered to be the main inflammatory cells involved with fibrosis (46, 47). Previous studies have confirmed that necrosis and apoptosis of acinar cells can activate macrophages, which can induce PSC activation by releasing pro-inflammatory factors and create a positive feedback cycle by promoting PSCs to secrete more cytokines (48, 49). The latest research found that the polarization of macrophages affects the development direction of CP fibrosis. Activated PSCs can induce M2 (selectively activated macrophages) polarization of macrophages. M2- type macrophages have been shown to play a significant role in angiogenesis and promote tissue fibrosis (50–53).
2.4 CP develops to the precancerous lesion of PC and eventually to PC
During the development of CP to pancreatic cancer, we have observed the metastasis of pancreatic acinar cells, adm and PanIN. PanIN is defined as a non-invasive precancerous lesion. Its microstructure is papillary or flat, composed of columnar and cubic duct cells, and rich in mucus. They are classified into PanIN-1, PanIN-2 and PanIN-3 according to their degree of heterotypicity. PanIN usually exists in the pancreatic tissue near invasive pancreatic cancer and participates in the spread and metastasis of PC. Many case reports have confirmed that PanIN eventually develops into PC (54). The changes of cancer related genes have already appeared in pancreatic PanIN such as KRAS and TP53 mutations (55). Compared with other types of pancreatitis, hereditary CP is more prone to PanIN, which also suggests that the transformation of its pathological state is related to genetic factors.
When pancreatic injury persists, the inflammatory cascade is triggered, and a variety of inflammatory and pancreatic tissue cells interact to participate in the inflammatory and fibrotic responses of CP. Oxidative stress damage, autophagy, mitochondrial dysfunction, and endoplasmic reticulum stress have been shown to be multiple parallel mechanisms of CP pathogenesis (56). Similarly, in PC, the reaction of inflammatory cytokines leads to the proliferation of acinar, ductal and stellate cells, the transition from epithelial cells to mesenchymal cells, and progressive tumorigenesis (57). Understanding the pathogenesis will identify gene loci, specific cells, and downstream signaling pathways involved in CP and PC that can act as new treatment targets, providing favorable conditions for the discovery of specific drugs to prevent and delay fibrosis and disease deterioration (58).
3 Research progress of anti-inflammatory and anti-fibrotic therapies
3.1 Mesenchymal stem cells (MSCs)
MSCs are self-renewing pluripotent stem cells with multidirectional differentiation potential, and their application in organ transplantation and other aspects has been extensively explored (59, 60). MSCs have been shown to have remarkable therapeutic effects in a variety of diseases such as ulcerative colitis and idiopathic pulmonary fibrosis (61, 62)
The application of MSCs in CP can be summarized in two main aspects. First, MSCs can be used to restore pancreatic secretory function during total pancreatectomy and autologous islet transplantation (63). Second, MSCs have unique anti-inflammatory and immunomodulatory effects, which can reduce the inflammatory response during CP, inhibit fibrosis, and rescue pancreatic function (62, 64).
Here, we summarize the latest experimental results on MSCs for CP treatment (Table 1).
3.1.1 Inhibits inflammation and pancreatic fibrosis
Studies have shown that a large number of inflammatory factors, such as IL-18, TNF-α, and growth factor TGF-B1 are significantly reduced in animal models of CP treated with MSCs, and tissue immune cell infiltration is alleviated. In vitro experiments also showed that when co-cultured with MSCs, the production of inflammatory factors in acinar cells and PSCs was inhibited and the inflammatory response of macrophages was reduced (65–70).
Pancreatic histopathology showed that MSC treatment improved collagen deposition, repaired damaged cells and Langerhans giant cells, reduced the expression of α-SMA, and reduced immune cell infiltration. Serum analysis showed that MSCs affected ECM metabolism by decreasing levels of hyaluronic acid and fibronectin (69, 70). Activation of PSCs is a key link that leads to pancreatic fibrosis. MSCs can downregulate the inflammatory response of PSCs and inhibit the activation of its downstream signaling molecules, indicating that MSC treatment can reduce pancreatic fibrosis by affecting PSC activity (67).
3.1.2 Improves pancreas morphology and increases body weight
Compared to the CP group, mice injected with MSCs showed a significant increase in pancreatic volume and weight. Most animals with CP showed severe jaundice and decreased activity, while the general condition of MSC treated mice showed significant improvement. The body weight of the MSC group increased gradually and the total weight gain was greater than that of the control group (67).
3.1.3 Improve pancreatic enzyme levels and reduce blood lipid levels
Compared with the untreated group, the decrease of protein expression and amylase activity in the pancreas during CP was reversed after MSC treatment, and abnormally elevated serum pancreatic enzyme levels decreased. Therefore, MSC treatment can improve exocrine function and attenuate the pancreatic injury caused by CP (70).
Compared with the CP group, serum total cholesterol (TC), triglycerides (TG), and low density lipoprotein cholesterol (LDL-C) levels significantly decreased and high density lipoprotein cholesterol (HDL-C) levels significantly increased in the MSC treatment group. Ahmed et al. proposed that normalization of the lipid profile of the MSC-treated subjects was achieved by improving β-c ell function and insulin resistance. MSCs can also secrete bioactive factors and differentiate into β-c ells to rescue pancreatic endocrine function (70, 71).
3.1.4 Homing of MSCs and the differentiation into acinar cells
When inflammation occurs, MSCs respond to the signals sent by the tissues and are recruited to the inflamed or damaged parts requiring repair, which is called homing. A great number of labeled MSCs were found in the pancreatic tissue of the MSC group, whereas no MSCs were found in the pancreatic tissue of the control group (65). Moreover, most MSCs observed in the pancreas were amylase positive, indicating that MSCs not only migrated to the damaged pancreas but also differentiated into amylase acinar- like cells to repair the pancreatic tissue damage (67).
When stem cells are co-cultured with pancreatic acinar cells, they can differentiate into cells expressing amylopsin, and the differentiated cells had the same gene expression profile as pancreatic acinar cells, which indicates that MSC can repair damaged pancreatic tissue by directly differentiating into acinar cells (67).
3.1.5 Reduces pancreatic cell apoptosis
Compared with healthy rats, apoptosis of pancreatic tissue cells in CP rats increased, which may be due to MSCs promoting the transcription and translation of apoptotic genes such as Bax, p53 and caspase-3. However, after MSC treatment, the expression of pro- apoptotic proteins decreased, and the expression of the anti-apoptotic protein Bcl-2 obviously increased, which indicates that MSCs could alleviate pancreatic injury by reducing pancreatic cell apoptosis (68, 69).
3.2 Phytochemicals
In recent years, phytochemicals have been widely used in clinical practice and have shown positive effects. Experiments have confirmed that phytochemicals have great potential in the treatment of CP and PC by reducing inflammation of the pancreas and slowing the progression of pancreatic fibrosis through a variety of different mechanisms and pathways (Table 2).
3.2.1 Curcumin
Curcumin is an active substance with strong anti-inflammatory and anti-fibrosis effects, which is isolated and purified from Curcuma. In addition, polyphenols have antioxidant, antibacterial, anti- angiogenic, and anti-platelet aggregation properties. Because of these properties, curcumin can protect and prevent many diseases such as cancer, autoimmune diseases, lung, liver and cardiovascular diseases (72). It can improve liver fibrosis by inhibiting the proliferation of hepatic astrocytes, and improve myocardial fibrosis by modulating ECM metabolism (73).
In vivo experiments showed that curcumin inhibited the proliferation and activation of PSCs and decreased the expression of pro-fibrosis substances, such as type I collagen (Col I-α1) and fibronectin1 (FN1) in PSCs (74). As long as one tenth of the effective concentration of curcumin, curcumin analogues (L49H37) can induce PSC apoptosis, and its antiproliferative effect on PSCs is stronger than that of curcumin (75).
Curcumin has been tested on a variety of PC cell lines, and the results show that curcumin can induce apoptosis through EGFR downregulation and inhibition of NF-KB pathway. In vivo experiments show that curcumin and its analogues can inhibit angiogenesis and reduce the invasion of PC in mice, while liposome curcumin shows better effect. In addition, clinical trials have shown that oral curcumin is well tolerated in patients with PC and curcumin combined with gemcitabine shows better efficacy than alone (76).
However, the medicinal effects of curcumin may be overestimated, and no relevant drugs have been applied clinically to date. This may be related to the complexity of curcuma extract, which makes it difficult to purify the active components (77).
3.2.2 Rhein
Rhein is extracted from the rhizome of rhubarb, which has many pharmacological properties, including anti-inflammatory and anti-angiogenesis effects (36). In a CP model, rhein alone or in combination with Salvia miltiorrhiza can significantly reduce the immune activity of α-SMA and TGF-β, the main activators of fibrosis, and inhibit the activation of PSCs, blocking a key node in fibrosis development. The deposition of ECM protein FN and Col I-α1 in the exocrine parenchyma also decreased. Researchers confirmed that this was mainly achieved by alleviating oxidative and endoplasmic reticulum stress and inhibiting SHH/GLI1 signal transduction (78).
3.2.3 Green tea catechin derivatives
Epigallocatechin gallate (EGCG) is a phenolic compound extracted from green tea that exhibits strong antioxidant activity. EGCG inhibits the proliferation and migration of PSCs by inhibiting the PDGF- mediated signaling pathway. EGCG can also inhibit the transformation of ethanol- stimulated PSCs from a normal static phenotype to a myofibroblast- like phenotype (79, 80).
At present, research on EGCG is limited to the cellular level, and further animal experiments are needed to verify the role of EGCG in the treatment of CP.
3.2.4 Resveratrol
When plants are attacked by external stimuli or pathogens, they will synthesize a polyphenol substance called resveratrol. Based on the current experimental results, resveratrol, similar to phenolic compounds such as rhein, can significantly impair the transcription and expression of several fibrosis mediators in PSCs and inhibit the proliferation and activation of PSC s. In vitro experiments showed that resveratrol could inhibit the proliferation, activation and migration of PSCs, which was initiated by reactive oxygen species (ROS). This may be related to the downregulation of microRNA 21 transcription levels (81).
Resveratrol improved the degree of pancreatic fibrosis in a CP model and effectively reduced PSC activation, ECM deposition, and the destruction of acinar structure in pancreatic tissue. The underlying mechanism may involve the accumulation of Mist1 in the nucleus and the inhibition of Akt and p38 MAPK signal transduction (81).
Previous studies have shown that resveratrol can inhibit the proliferation of PC cells, induce apoptosis and cell cycle arrest, inhibit the metastasis and invasion of cancer cells, and enhance the radiosensitivity of cancer cells (82). The latest experiment confirmed that resveratrol mainly affects NF- κ B signaling pathway to reduce the severity of pancreatitis and inhibit the occurrence of premalignant lesions such as ADM/PanINs, preventing the progression of PC (83).
3.2.5 Ellagic acid
Ellagic acid is a natural polyphenol component widely existing in various soft fruits, nuts and other plant tissues which can reduce inflammation in chronic inflammatory diseases, such as Crohn’s disease and ulcerative colitis (84).
Studies have shown that ellagic acid can inhibit the activation of PSCs in vitro and prevent the differentiation of activated PSCs into fibroblasts (85). Rats treated with ellagic acid for ten weeks showed significantly reduced severity of pancreatitis and fibrosis. In addition, ellagic acid can inhibit the production of ROS in PSCs, which reduces TGF- β 1 and platelet growth factor levels (86).
3.2.6 Isoglycyrrhizin
Isoglycyrrhizin is a bioactive component isolated from Glycyrrhiza roots and has been used for the prevention and treatment of cervical cancer, leukemia, and other diseases (87).
A previous experiment confirmed that after the treatment of CP rats with ILG, the pancreatic fibrosis was improved and the macrophage infiltration was significantly reduced. The follow-up team’s in vitro study on human PSCs showed that ILG could inhibit the activation and migration of human PSCs by downregulating the activities of ERK1/2 and JNK1/2. In addition, ILG weakens the M1 polarization trend of macrophages by affecting the NF-κB signaling pathway, but does not affect M2 polarization. The above experimental results suggest that ILG is a candidate drug for CP worthy of further study (88).
3.2.7 Ganoderma lucidum polysaccharide
Ganoderma lucidum polysaccharide (GLP) is an important bioactive component extracted from Ganoderma lucidum, a common component of traditional Chinese medicine. It has been proved to have great health promoting value, such as anti-oxidative, anti-inflammatory, and anti-cancer effects (89, 90).
A study confirmed that three kinds of Ganoderma polysaccharides (GLPS3-I, -II, and -III) derived from fermentation broth, cultured mycelium, and fruiting bodies can significantly improve CP in mice. The mechanism of action mainly involves increasing the activity of antioxidant superoxide dismutase (SOD) and glutathione peroxidase, reducing the content of malondialdehyde (MDA), and reducing the levels of IL-1β and interferon-γ. In addition, GLPS3 - II extracted from mycelium performs better in the overall improvement of CP mice. It also changed the composition and diversity of intestinal flora and increases the proportion of beneficial bacteria. These results suggest that GLPS3 can affect CP development by changing the gut microflora (91, 92).
3.2.8 Inonotus obliquus polysaccharide
Inonotus obliquus polysaccharide (IOP) is considered the main bioactive component of the medicinal mushroom Inonotus obliquus and has been shown to have anti-tumor and anti-oxidative properties (93).
Researchers purified it to obtain three polysaccharide components, IOP-I, II, and III, which were infused into a mouse model of CP. The results showed that purified IOP had pharmacological activity against CP, which mainly manifested as the slowing down of body weight loss, improvement of pancreatic fibrosis, and reduction in inflammation. When the dose of IOP was 400 mg/kg, optimal efficiency was achieved (94).
3.2.9 Apigenin
Apigenin is a flavonoid that has many biological activities such as anti-tumor and cardio- cerebrovascular protection (95). A study showed that apigenin reduced the expression of collagen and fibronectin, reduced the pancreatic stress response to injury, retained acinar units, and alleviated acinar cell damage. With the prolongation of time and the increase of apigenin dosage, the viability of astrocytes gradually weakened and the apoptosis gradually increased. This may be inextricably related to the attenuation of the pro-inflammatory signaling pathway of TNF (96).
In addition, apigenin and its analogues showed significant efficacy in improving pancreatitis fibrosis even at very low doses (0.5 mg/kg) (97).
3.2.10 Olive leaf extract
Olive leaf extracts improved CP in adult rats. The CP group showed a significant decrease in body weight and an increase in serum glucose, insulin, amylase, and lipase levels. A large increase in MDA levels and decrease in SOD levels were observed. After olive leaf extract treatment, the above parameters significantly improved, pancreatic degenerative changes were reduced, and TGF- β and IL-6 levels also decreased (98). There have been few reports of olive leaf extract improving CP, and further experiments are needed to confirm this.
3.3 Antioxidants
Antioxidants are commonly used in the clinical treatment of CP and are expected to alleviate persistent abdominal pain caused by inflammation and other pathological reactions (99). However, recent studies have shown that antioxidant treatment cannot reduce pain in patients with CP (100), nor can it improve the quality of life of patients in other respects (101). Whether the use of antioxidants can change the inflammatory state of CP or delay the pathological process remains controversial. Some studies have shown that antioxidant treatment can improve oxidative stress. However, other researchers have suggested that antioxidant supplementation can only increase the level of antioxidants in the body and has no practical significance in reducing inflammation, improving pancreatic fibrosis, and alleviating clinical symptoms (102). Interestingly, research on antioxidant therapy targeting the Nrf2 antioxidant pathway has yielded relatively consistent positive results. We will next focus on the research results on dimethyl fumarate, an Nrf2 activator, in the treatment of CP (103).
3.3.1 Dimethyl fumarate
Dimethyl fumarate (DMF) alleviates ROS- induced cytotoxicity by activating Nrf2, which stimulates the production of glutathione (GSH), a reactive oxygen scavenger. DMF has been proven to have therapeutic effects on renal and pulmonary fibrosis (104). Recent studies on CP have suggested that DMF- treated rats had reduced pancreatic atrophy, acinar structure damage, and pancreatic histological severity scores; significantly improved glucose tolerance, and increased exocrine tissue volume. Continuous intake of DMF effectively improved histopathological abnormalities and islet cell function (105).
3.4 Other methods
3.4.1 Pirfenidone
Pirfenidone is a pyridine ketone compound with a broad-spectrum anti-fibrotic effect that has shown good results in the treatment of idiopathic pulmonary fibrosis, liver fibrosis, and other fibrotic diseases (106, 107).
Pirfenidone ameliorates pancreatic tissue atrophy, acinar cell loss, and inflammatory responses when the pancreas is in a state of sustained injury. In in vitro experiments, pirfenidone reduced the profibrotic phenotype and infiltration of pancreatic macrophages and altered the cytokine environment in the pancreas before pathological changes occurred. No therapeutic effect of pirfenidone was observed in the absence of macrophages. In addition, pirfenidone has been shown to reduce collagen secretion, cytokine levels, and fibrosis marker expression in PSCs. These results indicate that this drug has great potential in the treatment of CP, but its efficacy as a therapeutic agent for CP needs further in vivo and in vitro tests and clinical trials (108).
3.4.2 Dasatinib
Dasatinib is a multi- tyrosine kinase inhibitor commonly used in the treatment of acute and chronic leukemia (109). In vitro experiments confirmed that dasatinib had a significant inhibitory effect on the proliferation and activation of PSCs and M1 and M2 polarization of macrophages and hindered macrophage recruitment and crosstalk with PSCs. In a rat CP model, dasatinib also improved pancreatic fibrosis and reduced macrophage infiltration (110).
3.4.3 Chemical pancreatectomy
Saleh et al. studied a method of “chemical resection of the pancreas,” which ablates pancreatic exocrine glands by flushing acetic acid through the pancreatic duct, prevents the inflammatory cascade caused by premature activation of pancreatic enzymes before other tissues of the pancreas are destroyed, and rescues the function of the damaged pancreas. They showed that after acetic acid treatment, inflammation of the pancreatic reserved tissue was reduced, the islet tissue was intact, pain was reduced, glucose tolerance improved, and insulin secretion increased. This operation can be easily performed in humans through endoscopic retrograde cholangiopancreatography. Dietary enzyme supplements are routinely used to replace exocrine function of the pancreas after surgery. However, chemical damage to the exocrine gland of the pancreas is an invasive procedure, and clinical experiments are needed to confirm safety (111).
3.4.4 Cholecystokinin receptor antagonist
Cholecystokinin (CCK) is often used to induce experimental CP (112). Previous studies have shown that plasma CCK levels in CP patients are significantly elevated. It was also found that CCK could directly activate PSCs and induce collagen synthesis (113). After treatment with a CCK receptor blockers, the pancreatic weight of mice recovered faster and serum lipase levels decreased. Histopathology showed that inflammatory infiltration of the pancreas and metaplasia of the acinar ducts was reduced, which may be related to the decreased expression of genes related to pancreatic selective fibrosis (114).
3.4.5 Replacement therapy improves the status of pancreatic FGF21 deficiency
FGF21 is a hormone secreted by the liver, and its main role is related to glucose metabolism, lipid metabolism and insulin resistance. In the CP model induced by chemical agents, the content of FGF21 was significantly increased (115). The onset of pancreatitis is initially induced by ATF4, with a concomitant increase in FGF21, which may indicate a preliminary attempt to overcome pancreatic tissue damage. However, with the persistence of pancreatitis, subsequent ATF3 induction effectively inhibits FGF21 transcription and plays an important role in contributing to CP. The expression of FGF21 is down regulated in a pancreatitis model. Supplementation with FGF21 can directly act on the exocrine function of the pancreas to reverse pancreatitis, which is manifested as a reduction in plasma amylase levels, alleviation of pancreatitis edema, and inflammatory infiltration and necrosis. The same effect can be achieved by blocking the induction of ATF3 and increasing the expression of FGF21 (116–118).
3.4.6 Statins
Previous in vitro experiments have shown that lovastatin, a hydroxymethylglutaryl coenzyme A reductase inhibitor, can interfere with the activation of PSCs by blocking Ras signal. Because of its antioxidant characteristic, statins can reduce the production of interleukin-10(IL-10), alleviate pancreatitis and fibrosis, and improve exocrine function when applied to the rat model of CP. The combination of lisinopril and lovastatin can inhibit pancreatic fibrosis in rats with alcoholic CP after distal pancreatectomy, which is reflected in the down-regulation of PSCs activity (119). In addition, researchers confirmed that long-term use of statins can also prevent pancreatitis after endoscopic retrograde cholangiopancreatography(ERCP) (120).
At present, randomized controlled clinical trials on whether simvastatin can help prevent recurrent acute pancreatitis(RAP) and acute attack of CP, and avoid the natural progression of AP to CP is under way (121, 122). We expect that they can obtain gratifying results. Other experts have explored the relationship between statins and the incidence and prognosis of pancreatic cancer(PC): statins can reduce the risk of PC in high-risk groups and improve the survival rate of patients with grade I or II PC and locally resectable PC (123). In contrast, another Danish cohort study showed that statins was not associated with the risk of PC in patients with CP (120). The reason for the difference is due to different races or other influencing factors need to be further discussed.
Statins can slow down the progress of CP mainly because it can improve lipid metabolism and effectively fight inflammation. As a classic drug for the treatment of coronary atherosclerosis, it has been widely used in clinical. If its efficacy for CP can be determined, clinical application will be easier than other new drugs.
3.4.7 Vitamin D
Vitamin D(VD) is an important steroid hormone regulating calcium and phosphorus levels in the body, and plays an important role in the treatment of bone diseases. Recent studies have also confirmed that it has multiple effects such as immune regulation, anti-inflammation and anti-fibrosis. VD deficiency is a common problem in patients with CP (124), but whether VD deficiency can be recognized as an independent risk factor of CP remains controversial.
In vivo and in vitro experiments have confirmed that VD and its analogues can inhibit the development of pancreatitis and pancreatic ductal adenocarcinoma(PDAC) by inhibiting the activation of PSCs (125, 126). Clinical trials show that oral or intravenous VD can improve VD deficiency in patients with CP, alleviate its related symptoms, and improve the quality of life of patients, but the effect is only shown when VD is deficient (127). When the deficiency is corrected, VD supplementation will no longer have a significant effect on patients.
VD can inhibit the development of CP mainly due to its anti-inflammatory, anti-fibrosis and immunomodulatory effects (128). In addition, VD can also inhibit the cell cycle of a variety of cells, inhibit cell proliferation, interfere with the dedifferentiation of tumor cells, and induce apoptosis (129–131), which is of great significance to prevent CP from developing into PC and the deterioration of PC (132).
4 Conclusions and future perspectives
With continuing research the risk factors and pathophysiological mechanisms of CP have been clarified, patient management guidelines have been standardized, and endoscopic technology and surgery have become important methods for treating CP complications in the late stages of the disease. It is unfortunate that current clinical treatments for CP are based on symptomatic treatment. At present, there are no effective means to prevent the progression of CP and improve pancreatic function. In addition, as a high-risk factor for pancreatic cancer, if the development of pancreatic fibrosis in patients with CP is not effectively controlled, they will be at a higher risk of carcinogenesis.
Here we have summarized the current progress in etiological treatment of CP from the aspects of regenerative medicine, traditional Chinese medicine, biological therapy, and immune- targeted therapy. Different drugs and treatment methods affect disease progression through some similar pathways, such as reducing the level of inflammatory factors and oxidative stress to improve the inflammatory state of the pancreas and prevent the occurrence of precancerous lesions. In addition, PSCs play an important regulatory role, and therefore, are excellent therapeutic targets for preventing the progression of pancreatic fibrosis.
Although it has been effectively validated in cell- and animal-based trials, most drugs and therapeutic methods have not yet entered clinical trials. In view of the increasing disease burden of CP and PC, we should encourage further in vivo and in vitro experiments to provide more reliable evidence for the early application of effective treatment methods in clinical practice.
Author contributions
B-QL and X-YLiu reviewed literature and originally drafted the manuscript. TM, T-HZ, PZ, QZ, and YZ contributed to editing and embellished the manuscript. X-YLi approved the final version of the manuscript. All authors contributed to the article and approved the submitted version.
Funding
National Natural Science Foundation of China (Grant 82270676), 2021Shandong Province Graduate Education and Teaching Reform Research Project (SDYJG21110), Qingdao Chinese Medicine Technology Project (2021-zyym26).
Acknowledgments
We would like to thank Editage (www.editage.com) for English language editing. We thank all the authors for helping with the writing and publication of this article.
Conflict of interest
The authors declare that the research was conducted in the absence of any commercial or financial relationships that could be construed as a potential conflict of interest.
Publisher’s note
All claims expressed in this article are solely those of the authors and do not necessarily represent those of their affiliated organizations, or those of the publisher, the editors and the reviewers. Any product that may be evaluated in this article, or claim that may be made by its manufacturer, is not guaranteed or endorsed by the publisher.
References
1. Whitcomb DC, Frulloni L, Garg P, Greer JB, Schneider A, Yadav D, et al. Chronic pancreatitis: An international draft consensus proposal for a new mechanistic definition. Pancreatology (2016) 16(2):218–24. doi: 10.1016/j.pan.2016.02.001
2. Capurso G, Archibugi L, Pasquali P, Aceti A, Balducci P, Bianchi P, et al. Prevalence of chronic pancreatitis: Results of a primary care physician-based population study. Digest Liver Dis Off J Ital Soc Gastroenterol Ital Assoc Study Liver (2017) 49(5):535–9. doi: 10.1016/j.dld.2016.12.024
3. Olesen SS, Mortensen LH, Zinck E, Becker U, Drewes AM, Nøjgaard C, et al. Time trends in incidence and prevalence of chronic pancreatitis: A 25-year population-based nationwide study. United Eur Gastroenterol J (2021) 9(1):82–90. doi: 10.1177/2050640620966513
4. Spagnolo DM, Greer PJ, Ohlsen CS, Mance S, Ellison M, Breze C, et al. Acute and chronic pancreatitis disease prevalence, classification, and comorbidities: A cohort study of the UK BioBank. Clin Trans Gastroenterol (2022) 13(1):e00455. doi: 10.14309/ctg.0000000000000455
5. Maatman TK, Zyromski NJ. Chronic pancreatitis. Curr Problems Surg (2021) 58(3):100858. doi: 10.1016/j.cpsurg.2020.100858
6. Zou WB, Ru N, Wu H, Hu LH, Ren X, Jin G, et al. Guidelines for the diagnosis and treatment of chronic pancreatitis in China (2018 edition). Hepatobiliary Pancreatic Dis Int HBPD Int (2019) 18(2):103–9. doi: 10.1016/j.hbpd.2019.02.004
7. Beyer G, Habtezion A, Werner J, Lerch MM, Mayerle J. Chronic pancreatitis. Lancet (London England) (2020) 396(10249):499–512. doi: 10.1016/S0140-6736(20)31318-0
8. Whitcomb DC. Pancreatitis: TIGAR-O version 2 Risk/Etiology checklist with topic reviews, updates, and use primers. Clin Trans Gastroenterol (2019) 10(6):e00027. doi: 10.14309/ctg.0000000000000027
9. Machicado JD, Yadav D. Epidemiology of recurrent acute and chronic pancreatitis: Similarities and differences. Digest Dis Sci (2017) 62(7):1683–91. doi: 10.1007/s10620-017-4510-5
10. Hori Y, Vege SS, Chari ST, Gleeson FC, Levy MJ, Pearson RK, et al. Classic chronic pancreatitis is associated with prior acute pancreatitis in only 50% of patients in a large single-institution study. Pancreatol Off J Int Assoc Pancreatol (IAP) (2019) 19(2):224–9. doi: 10.1016/j.pan.2019.02.004
11. Petersen B, Schaffalitzky de Muckadell OB. [Autoimmune pancreatitis]. Ugeskrift Laeger (2019) 181(46):V07190398.
12. Khandelwal A, Inoue D, Takahashi N. Autoimmune pancreatitis: an update. Abdominal Radiol (New York) (2020) 45(5):1359–70. doi: 10.1007/s00261-019-02275-x
13. Masamune A, Kikuta K, Kume K, Hamada S, Tsuji I, Takeyama Y, et al. Nationwide epidemiological survey of chronic pancreatitis in Japan: introduction and validation of the new Japanese diagnostic criteria 2019. J Gastroenterol (2020) 55(11):1062–71. doi: 10.1007/s00535-020-01704-9
14. Takahashi T, Miao Y, Kang F, Dolai S, Gaisano HY. Susceptibility factors and cellular mechanisms underlying alcoholic pancreatitis. Alcoholism Clin Exp Res (2020) 44(4):777–89. doi: 10.1111/acer.14304
15. Jeon CY, Feldman R, Althouse A, AlKaade S, Brand RE, Guda N, et al. Lifetime smoking history and cohort-based smoking prevalence in chronic pancreatitis. Pancreatol Off J Int Assoc Pancreatol (IAP) (2021) 29:S1424-3903(21)00473-7. doi: 10.1016/j.pan.2021.05.302
16. Hao L, Liu Y, Dong ZQ, Yi JH, Wang D, Xin L, et al. Clinical characteristics of smoking-related chronic pancreatitis. Front Cell Infect Microbiol (2022) 12:939910. doi: 10.3389/fcimb.2022.939910
17. Takáts A, Berke G, Gede N, Németh BC, Witt H, Głuszek S, et al. Risk of chronic pancreatitis in carriers of loss-of-function CTRC variants: A meta-analysis. PloS One (2022) 17(5):e0268859. doi: 10.1371/journal.pone.0268859
18. Giefer MJ, Lowe ME, Werlin SL, Zimmerman B, Wilschanski M, Troendle D, et al. Early-onset acute recurrent and chronic pancreatitis is associated with PRSS1 or CTRC gene mutations. J Pediatr (2017) 186:95–100. doi: 10.1016/j.jpeds.2017.03.063
19. Zou WB, Tang XY, Zhou DZ, Qian YY, Hu LH, Yu FF, et al. SPINK1, PRSS1, CTRC, and CFTR genotypes influence disease onset and clinical outcomes in chronic pancreatitis. Clin Trans Gastroenterol (2018) 9(11):204. doi: 10.1038/s41424-018-0069-5
20. Liu X, Tu M, Dai X, Zhu Y, Ge Q, Gao W, et al. PRSS1 and SPINK1 mutations in alcoholic and idiopathic chronic pancreatitis. J Nanosci Nanotechnol (2017) 17(4):2358–362. doi: 10.1166/jnn.2017.12626
21. Szabó A, Toldi V, Gazda LD, Demcsák A, Tőzsér J, Sahin-Tóth M. Defective binding of SPINK1 variants is an uncommon mechanism for impaired trypsin inhibition in chronic pancreatitis. J Biol Chem (2021) 296:100343. doi: 10.1016/j.jbc.2021.100343
22. Singh VK, Yadav D, Garg PK. Diagnosis and management of chronic pancreatitis: A review. Jama (2019) 322(24):2422–34. doi: 10.1001/jama.2019.19411
23. Mann R, Boregowda U, Vyas N, Gajendran M, Umapathy CP, Sayana H, et al. Current advances in the management of chronic pancreatitis. Dis-a-month DM (2021) 67(12):101225. doi: 10.1016/j.disamonth.2021.101225
24. Saluja A, Dudeja V, Dawra R, Sah RP. Early intra-acinar events in pathogenesis of pancreatitis. Gastroenterology (2019) 156(7):1979–93. doi: 10.1053/j.gastro.2019.01.268
25. Rosendahl J, Witt H. [Pathogenesis of chronic pancreatitis]. Der Internist (2021) 62(10):1007–14. doi: 10.1007/s00108-021-01150-6
26. Manohar M, Verma AK, Venkateshaiah SU, Sanders NL, Mishra A. Pathogenic mechanisms of pancreatitis. World J Gastrointestinal Pharmacol Ther (2017) 8(1):10–25. doi: 10.4292/wjgpt.v8.i1.10
27. Habtezion A. Inflammation in acute and chronic pancreatitis. Curr Opin Gastroenterol (2015) 31(5):395–9. doi: 10.1097/MOG.0000000000000195
28. Apte MV, Pirola RC, Wilson JS. Pancreatic stellate cells: a starring role in normal and diseased pancreas. Front Physiol (2012) 3:344. doi: 10.3389/fphys.2012.00344
29. Bynigeri RR, Jakkampudi A, Jangala R, Subramanyam C, Sasikala M, Rao GV, et al. Pancreatic stellate cell: Pandora's box for pancreatic disease biology. World J Gastroenterol (2017) 23(3):382–405. doi: 10.3748/wjg.v23.i3.382
30. Tang D, Wu Q, Zhang J, Zhang H, Yuan Z, Xu J, et al. Galectin-1 expression in activated pancreatic satellite cells promotes fibrosis in chronic pancreatitis/pancreatic cancer via the TGF-β1/Smad pathway. Oncol Rep (2018) 39(3):1347–55. doi: 10.3892/or.2018.6202
31. Lin H, Dong B, Qi L, Wei Y, Zhang Y, Cai X, et al. Inhibitory smads suppress pancreatic stellate cell activation through negative feedback in chronic pancreatitis. Ann Trans Med (2021) 9(5):384. doi: 10.21037/atm-20-4282
32. An W, Zhu JW, Jiang F, Jiang H, Zhao JL, Liu MY, et al. Fibromodulin is upregulated by oxidative stress through the MAPK/AP-1 pathway to promote pancreatic stellate cell activation. Pancreatol Off J Int Assoc Pancreatol (IAP) (2020) 20(2):278–87 doi: 10.1016/j.pan.2019.09.011
33. Xu XF, Liu F, Xin JQ, Fan JW, Wu N, Zhu LJ, et al. Respective roles of the mitogen-activated protein kinase (MAPK) family members in pancreatic stellate cell activation induced by transforming growth factor-β1 (TGF-β1). Biochem Biophys Res Commun (2018) 501(2):365–73. doi: 10.1016/j.bbrc.2018.04.176
34. Jin G, Hong W, Guo Y, Bai Y, Chen B. Molecular mechanism of pancreatic stellate cells activation in chronic pancreatitis and pancreatic cancer. J Cancer (2020) 11(6):1505–15. doi: 10.7150/jca.38616
35. Zhang X, Jin T, Huang X, Liu X, Liu Z, Jia Y, et al. Effects of the tumor suppressor PTEN on biological behaviors of activated pancreatic stellate cells in pancreatic fibrosis. Exp Cell Res (2018) 373(1-2):132–44. doi: 10.1016/j.yexcr.2018.10.005
36. Ramakrishnan P, Loh WM, Gopinath SCB, Bonam SR, Fareez IM, Mac Guad R, et al. Selective phytochemicals targeting pancreatic stellate cells as new anti-fibrotic agents for chronic pancreatitis and pancreatic cancer. Acta Pharm Sin B (2020) 10(3):399–413. doi: 10.1016/j.apsb.2019.11.008
37. Xue R, Jia K, Wang J, Yang L, Wang Y, Gao L, et al. A rising star in pancreatic diseases: Pancreatic stellate cells. Front Physiol (2018) 9:754. doi: 10.3389/fphys.2018.00754
38. Shimosegawa T. A new insight into chronic pancreatitis. Tohoku J Exp Med (2019) 248(4):225–38. doi: 10.1620/tjem.248.225
39. Swain SM, Romac JM, Vigna SR, Liddle RA. Piezo1-mediated stellate cell activation causes pressure-induced pancreatic fibrosis in mice. JCI Insight (2022) 7(8):e158288. doi: 10.1172/jci.insight.158288
40. Lee PJ, Papachristou GI. New insights into acute pancreatitis. Nat Rev Gastroenterol Hepatol (2019) 16(8):479–96. doi: 10.1038/s41575-019-0158-2
41. Gukovsky I, Pandol SJ, Mareninova OA, Shalbueva N, Jia W, Gukovskaya AS. Impaired autophagy and organellar dysfunction in pancreatitis. J Gastroenterol Hepatol (2012) 27 Suppl 2(Suppl 2):27–32. doi: 10.1111/j.1440-1746.2011.07004.x
42. Ding L, Liou GY, Schmitt DM, Storz P, Zhang JS, Billadeau DD. Glycogen synthase kinase-3β ablation limits pancreatitis-induced acinar-to-ductal metaplasia. J Pathol (2017) 243(1):65–77. doi: 10.1002/path.4928
43. Li S, Xie K. Ductal metaplasia in pancreas. Biochim Biophys Acta Rev Cancer (2022) 1877(2):188698. doi: 10.1016/j.bbcan.2022.188698
44. Liu J, Akanuma N, Liu C, Naji A, Halff GA, Washburn WK, et al. TGF-β1 promotes acinar to ductal metaplasia of human pancreatic acinar cells. Sci Rep (2016) 6:30904. doi: 10.1038/srep30904
45. Parte S, Nimmakayala RK, Batra SK, Ponnusamy MP. Acinar to ductal cell trans-differentiation: A prelude to dysplasia and pancreatic ductal adenocarcinoma. Biochim Biophys Acta Rev Cancer (2022) 1877(1):188669. doi: 10.1016/j.bbcan.2021.188669
46. Hu F, Lou N, Jiao J, Guo F, Xiang H, Shang D. Macrophages in pancreatitis: Mechanisms and therapeutic potential. Biomed Pharmacother Biomed Pharmacother (2020) 131:110693. doi: 10.1016/j.biopha.2020.110693
47. Van Gassen N, Staels W, Van Overmeire E, De Groef S, Sojoodi M, Heremans Y, et al. Concise review: Macrophages: Versatile gatekeepers during pancreatic β-cell development, injury, and regeneration. Stem Cells Trans Med (2015) 4(6):555–63. doi: 10.5966/sctm.2014-0272
48. Zhang H, Liu B, Xu XF, Jiang TT, Zhang XQ, Shi YL, et al. Pathophysiology of chronic pancreatitis induced by dibutyltin dichloride joint ethanol in mice. World J Gastroenterol (2016) 22(10):2960–70. doi: 10.3748/wjg.v22.i10.2960
49. Sendler M, Weiss FU, Golchert J, Homuth G, van den Brandt C, Mahajan UM, et al. Cathepsin b-mediated activation of trypsinogen in endocytosing macrophages increases severity of pancreatitis in mice. Gastroenterology (2018) 154(3):704–718.e10. doi: 10.1053/j.gastro.2017.10.018
50. Wu J, Zhang L, Shi J, He R, Yang W, Habtezion A, et al. Macrophage phenotypic switch orchestrates the inflammation and repair/regeneration following acute pancreatitis injury. EBioMedicine (2020) 58:102920. doi: 10.1016/j.ebiom.2020.102920
51. Shapouri-Moghaddam A, Mohammadian S, Vazini H, Taghadosi M, Esmaeili SA, Mardani F, et al. Macrophage plasticity, polarization, and function in health and disease. J Cell Physiol (2018) 233(9):6425–40. doi: 10.1002/jcp.26429
52. Kishore A, Petrek M. Roles of macrophage polarization and macrophage-derived miRNAs in pulmonary fibrosis. Front Immunol (2021) 12:678457. doi: 10.3389/fimmu.2021.678457
53. Lu Y, Lu G, Gao L, Zhu Q, Xue J, Zhang J, et al. The proresolving lipid mediator Maresin1 alleviates experimental pancreatitis via switching macrophage polarization. Mediators Inflammation (2021) 2021:6680456. doi: 10.1155/2021/6680456
54. Pinho AV, Chantrill L, Rooman I. Chronic pancreatitis: a path to pancreatic cancer. Cancer Lett (2014) 345(2):203–9. doi: 10.1016/j.canlet.2013.08.015
55. Tu J, Huang Z, Wang Y, Wang M, Yin Z, Mei X, et al. Transcriptome analysis of the procession from chronic pancreatitis to pancreatic cancer and metastatic pancreatic cancer. Sci Rep (2021) 11(1):3409. doi: 10.1038/s41598-021-83015-4
56. Gukovskaya AS, Gukovsky I, Algül H, Habtezion A. Autophagy, inflammation, and immune dysfunction in the pathogenesis of pancreatitis. Gastroenterology (2017) 153(5):1212–26. doi: 10.1053/j.gastro.2017.08.071
57. Kandikattu HK, Venkateshaiah SU, Mishra A. Chronic pancreatitis and the development of pancreatic cancer. Endocrine Metab Immune Disord Drug Targets (2020) 20(8):1182–210. doi: 10.2174/1871530320666200423095700
58. Kleeff J, Whitcomb DC, Shimosegawa T, Esposito I, Lerch MM, Gress T, et al. Chronic pancreatitis. Nat Rev Dis Primers (2017) 3:17060. doi: 10.1038/nrdp.2017.60
59. Li T, Xia M, Gao Y, Chen Y, Xu Y. Human umbilical cord mesenchymal stem cells: an overview of their potential in cell-based therapy. Expert Opin Biol Ther (2015) 15(9):1293–306. doi: 10.1517/14712598.2015.1051528
60. Rad F, Ghorbani M, Mohammadi Roushandeh A, Habibi Roudkenar M. Mesenchymal stem cell-based therapy for autoimmune diseases: emerging roles of extracellular vesicles. Mol Biol Rep (2019) 46(1):1533–49. doi: 10.1007/s11033-019-04588-y
61. Hu J, Zhao G, Zhang L, Qiao C, Di A, Gao H, et al. Safety and therapeutic effect of mesenchymal stem cell infusion on moderate to severe ulcerative colitis. Exp Ther Med (2016) 12(5):2983–9. doi: 10.3892/etm.2016.3724
62. Tzouvelekis A, Toonkel R, Karampitsakos T, Medapalli K, Ninou I, Aidinis V, et al. Mesenchymal stem cells for the treatment of idiopathic pulmonary fibrosis. Front Med (2018) 5:142. doi: 10.3389/fmed.2018.00142
63. Ellis C, Ramzy A, Kieffer TJ. Regenerative medicine and cell-based approaches to restore pancreatic function. Nat Rev Gastroenterol Hepatol (2017) 14(10):612–28. doi: 10.1038/nrgastro.2017.93
64. Ma Z, Zhou J, Yang T, Xie W, Song G, Song Z, et al. Mesenchymal stromal cell therapy for pancreatitis: Progress and challenges. Med Res Rev (2021) 41(4):2474–88. doi: 10.1002/med.21801
65. Zhou CH, Li ML, Qin AL, Lv SX, Wen T, Zhu XY, et al. Reduction of fibrosis in dibutyltin dichloride-induced chronic pancreatitis using rat umbilical mesenchymal stem cells from wharton's jelly. Pancreas (2013) 42(8):1291–302. doi: 10.1097/MPA.0b013e318296924e
66. Kawakubo K, Ohnishi S, Fujita H, Kuwatani M, Onishi R, Masamune A, et al. Effect of fetal membrane-derived mesenchymal stem cell transplantation in rats with acute and chronic pancreatitis. Pancreas (2016) 45(5):707–13. doi: 10.1097/MPA.0000000000000541
67. Sun Z, Gou W, Kim DS, Dong X, Strange C, Tan Y, et al. Adipose stem cell therapy mitigates chronic pancreatitis via differentiation into acinar-like cells in mice. Mol Ther J Am Soc Gene Ther (2017) 25(11):2490–501. doi: 10.1016/j.ymthe.2017.06.016
68. Xu X, Yu H, Sun L, Zheng C, Shan Y, Zhou Z, et al. Adipose−derived mesenchymal stem cells ameliorate dibutyltin dichloride−induced chronic pancreatitis by inhibiting the PI3K/AKT/mTOR signaling pathway. Mol Med Rep (2020) 21(4):1833–40. doi: 10.3892/mmr.2020.10995
69. Kong L, Xu X, Zhang H, Zhou Y, Huang H, Chen B, et al. Human umbilical cord-derived mesenchymal stem cells improve chronic pancreatitis in rats via the AKT-mTOR-S6K1 signaling pathway. Bioengineered (2021) 12(1):1986–96. doi: 10.1080/21655979.2021.1928441
70. Taha HS, Moustafa EM, Moawed FS, Hegazy MG. Curative role of mesenchymal stromal cells in chronic pancreatitis: Modulation of MAPK and TGF-β1/SMAD factors. Int J Immunopathol Pharmacol (2021) 35:20587384211054036. doi: 10.1177/20587384211054036
71. Ahmed SM, Morsi M, Ghoneim NI, Abdel-Daim MM, El-Badri N. Mesenchymal stromal cell therapy for pancreatitis: A systematic review. Oxid Med Cell Longevity (2018) 2018:3250864. doi: 10.1155/2018/3250864
72. Hasanzadeh S, Read MI, Bland AR, Majeed M, Jamialahmadi T, Sahebkar A. Curcumin: an inflammasome silencer. Pharmacol Res (2020) 159:104921. doi: 10.1016/j.phrs.2020.104921
73. Gorabi AM, Hajighasemi S, Kiaie N, Rosano GMC, Sathyapalan T, Al-Rasadi K, et al. Anti-fibrotic effects of curcumin and some of its analogues in the heart. Heart Failure Rev (2020) 25(5):731–43. doi: 10.1007/s10741-019-09854-6
74. Lin Z, Zheng LC, Zhang HJ, Tsang SW, Bian ZX. Anti-fibrotic effects of phenolic compounds on pancreatic stellate cells. BMC Complementary Altern Med (2015) 15:259. doi: 10.1186/s12906-015-0789-y
75. Gundewar C, Ansari D, Tang L, Wang Y, Liang G, Rosendahl AH, et al. Antiproliferative effects of curcumin analog L49H37 in pancreatic stellate cells: a comparative study. Ann Gastroenterol (2015) 28(3):391–8.
76. Hosseini M, Hassanian SM, Mohammadzadeh E, ShahidSales S, Maftouh M, Fayazbakhsh H, et al. Therapeutic potential of curcumin in treatment of pancreatic cancer: Current status and future perspectives. J Cell Biochem (2017) 118(7):1634–8. doi: 10.1002/jcb.25897
77. Baker M. Deceptive curcumin offers cautionary tale for chemists. Nature (2017) 541(7636):144–5. doi: 10.1038/541144a
78. Liang X, Han M, Zhang X, Sun X, Yu K, Liu C, et al. Dahuang danshen decoction inhibits pancreatic fibrosis by regulating oxidative stress and endoplasmic reticulum stress. Evidence-Based Complementary Altern Med eCAM (2021) 2021:6629729. doi: 10.1155/2021/6629729
79. Masamune A, Kikuta K, Satoh M, Suzuki N, Shimosegawa T. Green tea polyphenol epigallocatechin-3-gallate blocks PDGF-induced proliferation and migration of rat pancreatic stellate cells. World J Gastroenterol (2005) 11(22):3368–74. doi: 10.3748/wjg.v11.i22.3368
80. Asaumi H, Watanabe S, Taguchi M, Tashiro M, Nagashio Y, Nomiyama Y, et al. Green tea polyphenol (-)-epigallocatechin-3-gallate inhibits ethanol-induced activation of pancreatic stellate cells. Eur J Clin Invest (2006) 36(2):113–22. doi: 10.1111/j.1365-2362.2006.01599.x
81. Yan B, Cheng L, Jiang Z, Chen K, Zhou C, Sun L, et al. Resveratrol inhibits ROS-promoted activation and glycolysis of pancreatic stellate cells via suppression of miR-21. Oxid Med Cell Longevity (2018) 2018:1346958. doi: 10.1155/2018/1346958
82. Xu Q, Zong L, Chen X, Jiang Z, Nan L, Li J, et al. Resveratrol in the treatment of pancreatic cancer. Ann New York Acad Sci (2015) 1348(1):10–9. doi: 10.1111/nyas.12837
83. Qian W, Xiao Q, Wang L, Qin T, Xiao Y, Li J, et al. Resveratrol slows the tumourigenesis of pancreatic cancer by inhibiting NFκB activation. Biomed Pharmacother Biomed Pharmacother (2020) 127:110116. doi: 10.1016/j.biopha.2020.110116
84. Derosa G, Maffioli P, Sahebkar A. Ellagic acid and its role in chronic diseases. Adv Exp Med Biol (2016) 928:473–9. doi: 10.1007/978-3-319-41334-1_20
85. Suzuki N, Masamune A, Kikuta K, Watanabe T, Satoh K, Shimosegawa T. Ellagic acid inhibits pancreatic fibrosis in male wistar Bonn/Kobori rats. Digest Dis Sci (2009) 54(4):802–10. doi: 10.1007/s10620-008-0423-7
86. Aslan A, Beyaz S, Gok O, Can MI, Erman F, Erman O. The impact of ellagic acid on some apoptotic gene expressions: a new perspective for the regulation of pancreatic nrf-2/NF-κB and Akt/VEGF signaling in CCl(4)-induced pancreas damage in rats. Immunopharmacol Immunotoxicol (2021) 43(2):145–52. doi: 10.1080/08923973.2020.1869255
87. Peng F, Du Q, Peng C, Wang N, Tang H, Xie X, et al. A review: The pharmacology of isoliquiritigenin. Phytother Res PTR (2015) 29(7):969–77. doi: 10.1002/ptr.5348
88. Wang LJ, He L, Hao L, Guo HL, Zeng XP, Bi YW, et al. Isoliquiritigenin ameliorates caerulein-induced chronic pancreatitis by inhibiting the activation of PSCs and pancreatic infiltration of macrophages. J Cell Mol Med (2020) 24(17):9667–81. doi: 10.1111/jcmm.15498
89. Seweryn E, Ziała A, Gamian A. Health-promoting of polysaccharides extracted from ganoderma lucidum. Nutrients (2021) 13(8):2725. doi: 10.3390/nu13082725
90. Zhang J, Liu Y, Tang Q, Zhou S, Feng J, Chen H. Polysaccharide of ganoderma and its bioactivities. Adv Exp Med Biol (2019) 1181:107–34. doi: 10.1007/978-981-13-9867-4_4
91. Li K, Yu M, Hu Y, Ren G, Zang T, Xu X, et al. Three kinds of ganoderma lucidum polysaccharides attenuate DDC-induced chronic pancreatitis in mice. Chem Biol Interact (2016) 247:30–8. doi: 10.1016/j.cbi.2016.01.013
92. Li K, Zhuo C, Teng C, Yu S, Wang X, Hu Y, et al. Effects of ganoderma lucidum polysaccharides on chronic pancreatitis and intestinal microbiota in mice. Int J Biol Macromol (2016) 93(Pt A):904–12. doi: 10.1016/j.ijbiomac.2016.09.029
93. Lu Y, Jia Y, Xue Z, Li N, Liu J, Chen H. Recent developments in inonotus obliquus (Chaga mushroom) polysaccharides: Isolation, structural characteristics, biological activities and application. Polymers (2021) 13(9):1441. doi: 10.3390/polym13091441
94. Hu Y, Sheng Y, Yu M, Li K, Ren G, Xu X, et al. Antioxidant activity of inonotus obliquus polysaccharide and its amelioration for chronic pancreatitis in mice. Int J Biol Macromol (2016) 87:348–56. doi: 10.1016/j.ijbiomac.2016.03.006
95. Imran M, Aslam Gondal T, Atif M, Shahbaz M, Batool Qaisarani T, Hanif Mughal M, et al. Apigenin as an anticancer agent. Phytother Res PTR (2020) 34(8):1812–28. doi: 10.1002/ptr.6647
96. Mrazek AA, Bhatia V, Falzon M, Spratt H, Chao C, Hellmich MR. Apigenin decreases acinar cell damage in pancreatitis. Pancreas (2019) 48(5):711–8. doi: 10.1097/MPA.0000000000001310
97. Salehi B, Venditti A, Sharifi-Rad M, Kręgiel D, Sharifi-Rad J, Durazzo A, et al. The therapeutic potential of apigenin. Int J Mol Sci (2019) 20(6):1350. doi: 10.3390/ijms20061305
98. Romeh GH, El-Safty FEA, El-Mehi AE, Faried MA. Antioxidant, anti-inflammatory, and anti-fibrotic properties of olive leaf extract protect against l-arginine induced chronic pancreatitis in the adult male albino rat. Anat Cell Biol (2022) 55(2):205–16. doi: 10.5115/acb.21.187
99. Gupte A, Goede D, Tuite R, Forsmark CE. Chronic pancreatitis. BMJ (Clin Res ed) (2018) 361:k2126. doi: 10.1136/bmj.k2126
100. Rupasinghe SN, Siriwardena AK. Long-term outcome of patients with chronic pancreatitis treated with micronutrient antioxidant therapy. Hepatobiliary Pancreatic Dis Int HBPD Int (2017) 16(2):209–14. doi: 10.1016/S1499-3872(16)60128-5
101. Mohta S, Singh N, Gunjan D, Kumar A, Saraya A. Systematic review and meta-analysis: Is there any role for antioxidant therapy for pain in chronic pancreatitis. JGH Open Open Access J Gastroenterol Hepatol (2021) 5(3):329–36. doi: 10.1002/jgh3.12433
102. Singh N, Ahuja V, Sachdev V, Upadhyay AD, Goswami R, Ramakrishnan L, et al. Antioxidants for pancreatic functions in chronic pancreatitis: A double-blind randomized placebo-controlled pilot study. J Clin Gastroenterol (2020) 54(3):284–93. doi: 10.1097/MCG.0000000000001178
103. Kojayan GG, Alizadeh RF, Li S, Ichii H. Reducing pancreatic fibrosis using antioxidant therapy targeting Nrf2 antioxidant pathway: A possible treatment for chronic pancreatitis. Pancreas (2019) 48(10):1259–62. doi: 10.1097/MPA.0000000000001433
104. Grzegorzewska AP, Seta F, Han R, Czajka CA, Makino K, Stawski L, et al. Dimethyl fumarate ameliorates pulmonary arterial hypertension and lung fibrosis by targeting multiple pathways. Sci Rep (2017) 7:41605. doi: 10.1038/srep41605
105. Zhang WX, Zhao JH, Ping FM, Liu ZJ, Gu JX, Lu XQ. Effect of dimethyl fumarate on rats with chronic pancreatitis. Asian Pacific J Trop Med (2016) 9(3):261–4. doi: 10.1016/j.apjtm.2016.01.023
106. Sgalla G, Franciosa C, Simonetti J, Richeldi L. Pamrevlumab for the treatment of idiopathic pulmonary fibrosis. Expert Opin Investigational Drugs (2020) 29(8):771–7. doi: 10.1080/13543784.2020.1773790
107. Fang Q, Liu S, Cui J, Zhao R, Han Q, Hou P, et al. Mesoporous polydopamine loaded pirfenidone target to fibroblast activation protein for pulmonary fibrosis therapy. Front Bioengineering Biotechnol (2022) 10:920766. doi: 10.3389/fbioe.2022.920766
108. Palathingal Bava E, George J, Iyer S, Sahay P, Tarique M, Jain T, et al. Pirfenidone ameliorates chronic pancreatitis in mouse models through immune and cytokine modulation. Pancreatol Off J Int Assoc Pancreatol (IAP) (2022) 22(5):553–63 doi: 10.1016/j.pan.2022.05.002
109. Lindauer M, Hochhaus A, Dasatinib. Recent results in cancer research. fortschritte der krebsforschung. Progres Dans Les Recherches Sur Le Cancer (2018) 212:29–68. doi: 10.1007/978-3-319-91439-8_2
110. Zeng XP, Wang LJ, Guo HL, He L, Bi YW, Xu ZL, et al. Dasatinib ameliorates chronic pancreatitis induced by caerulein via anti-fibrotic and anti-inflammatory mechanism. Pharmacol Res (2019) 147:104357. doi: 10.1016/j.phrs.2019.104357
111. Saleh M, Sharma K, Kalsi R, Fusco J, Sehrawat A, Saloman JL, et al. Chemical pancreatectomy treats chronic pancreatitis while preserving endocrine function in preclinical models. J Clin Invest (2021) 131(3):e143301. doi: 10.1172/JCI143301
112. Keppel Hesselink JM. Rediscovery of ceruletide, a CCK agonist, as an analgesic drug. J Pain Res (2020) 13:123–30. doi: 10.2147/JPR.S232714
113. Berna MJ, Seiz O, Nast JF, Benten D, Bläker M, Koch J, et al. CCK1 and CCK2 receptors are expressed on pancreatic stellate cells and induce collagen production. J Biol Chem (2010) 285(50):38905–14. doi: 10.1074/jbc.M110.125534
114. Nadella S, Ciofoaia V, Cao H, Kallakury B, Tucker RD, Smith JP. Cholecystokinin receptor antagonist therapy decreases inflammation and fibrosis in chronic pancreatitis. Digest Dis Sci (2020) 65(5):1376–84. doi: 10.1007/s10620-019-05863-5
115. Coate KC, Hernandez G, Thorne CA, Sun S, Le TDV, Vale K, et al. FGF21 is an exocrine pancreas secretagogue. Cell Metab (2017) 25(2):472–80. doi: 10.1016/j.cmet.2016.12.004
116. Hernandez G, Luo T, Javed TA, Wen L, Kalwat MA, Vale K, et al. Pancreatitis is an FGF21-deficient state that is corrected by replacement therapy. Sci Trans Med (2020) 12(525):eaay5186. doi: 10.1126/scitranslmed.aay5186
117. Crunkhorn S. Restoring FGF21 reverses pancreatitis. Nat Rev Drug Discovery (2020) 19(2):89. doi: 10.1038/d41573-020-00004-4
118. Jelenik T, Dille M, Müller-Lühlhoff S, Kabra DG, Zhou Z, Binsch C, et al. FGF21 regulates insulin sensitivity following long-term chronic stress. Mol Metab (2018) 16:126–38. doi: 10.1016/j.molmet.2018.06.012
119. Nichita¸lo ME, Kravchenko DA, Shpon'ka IS, Medvetski¸ EB, Savitskaia IM, Bulik II, et al. [Inhibition of the stellate cells using lisinopril and lovastatin for prophylaxis of pancreatic stump fibrosis after performance of distal resection in a model of chronic alcoholic pancreatitis]. Klin Khirurhiia (2013) 2):64–6.
120. Hadi YB, Naqvi SF, Abdelqader A, Kupec J, Nasr J. Reduced risk of post ERCP pancreatitis in statin users. BMC Gastroenterol (2020) 20(1):125. doi: 10.1186/s12876-020-01264-5
121. Cárdenas-Jaén K, Vaillo-Rocamora A, Gracia Á, Garg PK, Zapater P, Papachristou GI, et al. Simvastatin in the prevention of recurrent pancreatitis: Design and rationale of a multicenter triple-blind randomized controlled trial, the SIMBA trial. Front Med (2020) 7:494. doi: 10.3389/fmed.2020.00494
122. Goodman MT, Lo SK, Yadav D, Wu BU, Jamil LH, Kwok KK, et al. Double-blinded, placebo-controlled trial of simvastatin to prevent recurrent pancreatitis. Pancreas (2022) 51(1):e10–2. doi: 10.1097/MPA.0000000000001955
123. Choi JH, Lee SH, Huh G, Chun JW, You MS, Paik WH, et al. The association between use of statin or aspirin and pancreatic ductal adenocarcinoma: A nested case-control study in a Korean nationwide cohort. Cancer Med (2019) 8(17):7419–30. doi: 10.1002/cam4.2617
124. Jøker-Jensen H, Mathiasen AS, Køhler M, Rasmussen HH, Drewes AM, Olesen SS. Micronutrient deficits in patients with chronic pancreatitis: prevalence, risk factors and pitfalls. Eur J Gastroenterol Hepatol (2020) 32(10):1328–34. doi: 10.1097/MEG.0000000000001866
125. Bläuer M, Sand J, Laukkarinen J. Physiological and clinically attainable concentrations of 1,25-dihydroxyvitamin D3 suppress proliferation and extracellular matrix protein expression in mouse pancreatic stellate cells. Pancreatol Off J Int Assoc Pancreatol (IAP) (2015) 15(4):366–71. doi: 10.1016/j.pan.2015.05.044
126. Kang ZS, Wang C, Han XL, Du JJ, Li YY, Zhang C. Design, synthesis and biological evaluation of non-secosteriodal vitamin d receptor ligand bearing double side chain for the treatment of chronic pancreatitis. Eur J Med Chem (2018) 146:541–53. doi: 10.1016/j.ejmech.2018.01.073
127. Wiese M, Gärtner S, Doller J, Tran QT, Frost F, Bannert K, et al. Nutritional management of chronic pancreatitis: A systematic review and meta-analysis of randomized controlled trials. J Gastroenterol Hepatol (2021) 36(3):588–600. doi: 10.1111/jgh.15230
128. Triantos C, Aggeletopoulou I, Thomopoulos K, Mouzaki A. Vitamin d-liver disease association: Biological basis and mechanisms of action. Hepatol (Baltimore Md) (2021) 74(2):1065–73. doi: 10.1002/hep.31699
129. Cantorna MT, Rogers CJ, Arora J. Aligning the paradoxical role of vitamin d in gastrointestinal immunity. Trends Endocrinol Metabol: TEM (2019) 30(7):459–66. doi: 10.1016/j.tem.2019.04.005
130. Gesmundo I, Silvagno F, Banfi D, Monica V, Fanciulli A, Gamba G, et al. Calcitriol inhibits viability and proliferation in human malignant pleural mesothelioma cells. Front Endocrinol (2020) 11:559586. doi: 10.3389/fendo.2020.559586
131. Sergeev IN. Vitamin d status and vitamin d-dependent apoptosis in obesity. Nutrients (2020) 12(5):1392. doi: 10.3390/nu12051392
Keywords: chronic pancreatitis, anti-inflammatory agents, fibrosis, therapeutics, mesenchymal stem cell, phytochemicals
Citation: Li B-Q, Liu X-Y, Mao T, Zheng T-H, Zhang P, Zhang Q, Zhang Y and Li X-Y (2022) The research progress of anti-inflammatory and anti-fibrosis treatment of chronic pancreatitis. Front. Oncol. 12:1050274. doi: 10.3389/fonc.2022.1050274
Received: 21 September 2022; Accepted: 11 November 2022;
Published: 24 November 2022.
Edited by:
Nilabja Sikdar, Indian Statistical Institute, Kolkata, IndiaReviewed by:
Biswarup Basu, Chittaranjan National Cancer Institute, IndiaChongyi Jiang, Fudan University, China
Copyright © 2022 Li, Liu, Mao, Zheng, Zhang, Zhang, Zhang and Li. This is an open-access article distributed under the terms of the Creative Commons Attribution License (CC BY). The use, distribution or reproduction in other forums is permitted, provided the original author(s) and the copyright owner(s) are credited and that the original publication in this journal is cited, in accordance with accepted academic practice. No use, distribution or reproduction is permitted which does not comply with these terms.
*Correspondence: Xiao-Yu Li, lixiaoyu05@163.com
†These authors have contributed equally to this work