- 1Department of Nuclear Medicine, The Affiliated Hospital of Southwest Medical University, Luzhou, Sichuan, China
- 2Nuclear Medicine and Molecular Imaging Key Laboratory of Sichuan Province, Luzhou, Sichuan, China
- 3Nuclear Medicine Institute of Southwest Medical University, Luzhou, Sichuan, China
- 4Department of Orthopaedics, The Affiliated Hospital of Southwest Medical University, Luzhou, Sichuan, China
Purpose: In this study, DOTA-IBA was radiolabeled with 68Ga and we determined the optimum labelling conditions and assessed the biological properties of 68Ga-DOTA-IBA. We investigated the biodistribution of 68Ga-DOTA-IBA in normal animals and undertook PET/CT imaging in humans. Finally, we explored the feasibility 68Ga-DOTA-IBA as a bone imaging agent and demonstrated its potential for the therapeutic release of 177Lu/225Ac-DOTA-IBA.
Methods: The controlled variables method was used to assess the impact of variables on the radiochemical purity of 68Ga-DOTA-IBA. The biological properties of 68Ga-DOTA-IBA were investigated.68Ga-DOTA-IBA micro-PET/CT imaging was performed on animals. Volunteers were recruited for 68Ga-DOTA-IBA imaging and data were compared to 99mTc-MDP imaging studies to calculate the target to non-target ratio (T/NT) of the lesions.
Results: The prepared 68Ga-DOTA-IBA had a radiochemical purity of >97% and demonstrated good biological properties with a good safety profile in normal mice. PET/CT imaging of the animals showed rapid blood clearance with high contrast between the bone and stroma. Human imaging showed that 68Ga-DOTA-IBA could detect more lesions compared to 99mTc-MDP and had a higher targeted to untargeted ratio.
Conclusions: 68Ga-DOTA-IBA is an osteophilic radiopharmaceutical that can be synthesized using a simple labelling method. 68Ga-DOTA-IBA has high radiochemical purity and is stable in vitro stability. It is rapidly cleared from the blood, has low toxicity and has strong targeting to the bone with long retention times. We also found that it is rapidly cleared in non-target tissues and has high contrast on whole-body bone imaging. 68Ga-DOTA-IBA PET/CT has potential as a novel bone imaging bone modality in patients with metastatic disease.
1. Introduction
Cancer remains a significant threat to human health, particularly advanced diseases that have metastasized to the bones. Prostate, breast, lung, liver and thyroid cancers have a high propensity to metastasize to the bones (1–3). Nuclear imaging techniques are widely used in the diagnosis of bone metastases using agents such as [18F] 18F-NaF and [99mTc] 99mTc-methylene diphosphonate (MDP). [18F] 18F-NaF requires an accelerator for generation limiting its use in the clinic. Also, [99mTc] 99mTc-MDP often shows false negatives for lesions with no obvious osteogenic response. The value of bisphosphonates in the diagnosis and treatment of skeletal disorders is well established as they have a strong affinity for bone (4). Gallium-68 [68Ga] is used clinically as a positron imaging agent and has several advantages. Specifically, [68Ga] can be obtained using a germanium gallium generator (68Ge/68Ga), it is a β-emitter and has a half-life of 67.71 minutes. 68Ga/177Lu [Lutetium] is a diagnostic and therapeutic nuclide (5).
Ibandronic (IBA) acid is a third generation bisphosphonate. Studies have reported the labelling of metal ions with IBA acid using complex methods requiring larger amounts of precursors and products that have toxic side effects (6, 7). Previous studies have shown that the bifunctional chelate DOTA can be used for the complexation of metal compounds (5). In this study, we combined DOTA with bisphosphate to form a new compound, DOTA-IBA that was then combined with 68Ga to form the novel probe, 68Ga-DOTA-IBA. In this paper, we describe the preparation conditions and characterize the biological and imaging properties of 68Ga-DOTA-IBA.
2. Materials and methods
2.1. Materials
68Ga solution (0.4 M HCL) was eluted from a 68Ge/68Ga generator (ITG 101, EckertZiegler, Germany). The molecular design of DOTA-IBA was provided by our laboratory and the drug synthesis was provided by the Shanghai New Drug Development Company. Sodium acetate, thin-layer chromatography silica plates and other reagents were purchased from the Shanghai Maclean Biochemical Company. Animal micro positron emission computed tomography (micro-PET/CT; SIEMENS Inveon TM Siemens, Germany), PET/CT (United Imaging 780 Shanghai United Imaging Medical Technology Co., Ltd.). Other equipment, chemicals and animals used in the experiments were provided by the Sichuan Provincial Key Laboratory of Nuclear Medicine and Molecular Imaging. All studies were approved by the Ethics Committee of Southwest Medical University.
2.2. Radiolabeling and quality control
A controlled variables approach was used to investigate the effect of the various factors on the radiochemical purity of the markers. The solution was prepared by mixing a certain amount of DOTA-IBA solution (1mg/1ml), sodium acetate solution and 68GaCl3 solution in sequence. The pH of the solution was adjusted with 0.1 M hydrochloric acid and 0.25 M sodium acetate. The reaction was performed at a specific temperature for a specific time. The solution was cooled and the pH was adjusted to 4-5 before being sterilized and filtered.
Thin layer chromatography silica plates were used as a support and 0.1 M sodium citrate solution was used as a solvent to unfold the system. Quality control images of 68Ga-DOTA-IBA: 68Ga-DOTA-IBA at the origin (Rf=0.3-0.4); while free 68GaCl3 moved ahead of the strip with the solvent (Rf=0.9-1.0).
The optimum reaction parameters were 10 μg (10 μL) of DOTA-IBA, 1 ml of sodium acetate and 4 ml of 68GaCl3. The radiochemical purity of the prepared 68Ga-DOTA-IBA was >97%. The pH was 4-5, the reaction temperature was 95°C and the reaction time was 15 min.
2.3. In vitro stability
The radiochemical purity of 68Ga-DOTA-IBA under optimal labelling conditions was determined by incubation in 0.9% NaCl and fresh human serum at 37°C. Paper chromatography was performed at 15 min, 30 min, 1 h, 2 h and 4 h. The experiments were repeated three times and the results are expressed as mean ± standard deviation.
2.3.1. Plasma protein binding rate
Fresh human plasma (0.1 ml) and freshly prepared 0.5 mCi 68Ga-DOTA-IBA were added to the test tubes and incubated at 37°C for 2 hours. A 25% trichloroacetic acid solution (1.0 mL) was then added to the tubes and centrifuged for 5 min. The supernatants were collected and the process was repeated three times. The CPM of the supernatant and the precipitate were measured separately using a gamma counter and the plasma protein binding rate (PPB) of 68Ga-DOTA-IBA was calculated according to the formula; PPB= [(A-background radioactive count)/(A+B-background radioactive count×2)] × 100%. All results are expressed as the mean ± standard deviation.
2.3.2. Lipid and water distribution
Freshly prepared 0.5 mCi 68Ga-DOTA-IBA was added to the tubes before shaking in a vortex mixer for 20 minutes followed by centrifugation for 5 minutes. The upper liquid (organic phase) and the lower liquid (aqueous phase) were collected separately in test tubes. The radioactivity counts of the organic and aqueous phases were measured separately using a gamma counter. The formula lipid-water distribution coefficients (logP) were calculated according to the formula; LogP=log [(B-background radioactive count)/(C-background radioactive count)]. The results were expressed as mean ± standard deviation.
2.4. Mouse toxicity tests
24 mice were randomly divided into four experimental groups consisting of a control group and 68Ga-DOTA-IBA groups at low, medium and high doses. Each group had equal numbers of male and female animals. The control group was injected with 0.2 ml of 0.9% NaCl and the experimental groups were injected with 0.1 mCi, 0.5 mCi and 1.0 mCi of 68Ga-DOTA-IBA solution, respectively. The body weights and general conditions of the mice were observed for 4 weeks. Routine blood tests and liver and kidney function were performed after 2 and 4 weeks in each group. After the 4th week of observation, tissues and organs were harvested from the mice for pathological examination (Ethics committee approval No: KY2022114).
2.4.1. Mouse biodistribution studies
30 healthy mice were divided into 5 groups. All mice were injected with 68Ga-DOTA-IBA 0.1 mCi (approximately 0.2ml) via the tail vein. Mice were executed under anesthesia at 15 min, 30 min, 1 h, 2 h and 4 h after dosing. Blood, heart, liver, spleen, lung, kidney, stomach, small intestine, brain, femur and muscle tissues were sampled and the radioactivity counts of the different tissues were measured using a gamma counter. The counts were corrected for attenuation and the %ID/g was calculated for each time point. The results were expressed as the mean ± standard deviation.
2.5. 68Ga-DOTA-IBA imaging analysis in a New Zealand rabbit model
Anesthetized normal New Zealand rabbits were injected with 1.0-2.0 mCi (0.5-0.6 ml) of 68Ga-DOTA-IBA under optimal labelling conditions from a marginal ear vein. Whole-body static imaging was performed at 1 h and 3 h after injection using a United Imaging 780 PET/CT instrument. PET images were acquired with a time window of 3.48 ns, an energy range of 350-650 KeV, a 128×128 matrix and a 10 min acquisition time. CT scans were obtained using a tube voltage of 80 Kv, a tube current of 500 Ua and a scan time of 10 min.
2.6. 68Ga-DOTA-IBA imaging study in normal mice
Whole-body bone imaging using micro-PET/CT was performed at 30 mins, 1.5 h and 3 h after tail vein injection of 68Ga-DOTA-IBA 0.2 mCi in anaesthetized mice. The PET imaging parameters were as follows; a time window of 3.48 ns, energy range 350-650 KeV, matrix 128×128, acquisition 10 min. CT scans were obtained at a tube voltage of 80 Kv, and a tube current of 500 As with a scan time of 10 mins.
2.7. Establishment of a bone metastasis model and imaging studies
A bone metastasis model was established in nude mice by intertibial bone marrow injection. 25 μL of PC-3 prostate cancer cell culture medium was injected into the left tibia of healthy nude mice. 3-4 weeks after inoculation, the mice were scanned by micro-CT (SIEMENS InveonTM, Munich, Germany) to determine the condition of the bone. The appearance of bone destruction (osteolytic, osteogenic or mixed) in the left tibia indicated that the model was successful. The mouse was anaesthetized with gas anaesthesia (isoflurane) and 68Ga-DOTA-IBA 0.1 mCi (50 μl) was injected into the tail vein with an insulin needle under optimal labelling conditions. Whole-body bone imaging was performed 1 h after the injection. Micro-PET/CT and PET acquisitions were performed as described above for normal mice.
2.8. 68Ga-DOTA-IBA imaging in patients
2.8.1. Patient selection
68Ga-DOTA-IBA and 99mTc-MDP whole-body bone imaging were performed in 5 patients and the imaging agents were compared. The patients included 2 males and 3 females who had a mean age of 54.2 years (34-66 years). The interval between the two examinations was > 3 days < 7 days.
The inclusion criteria were patients who had already undergone 99mTc-MDP imaging, had no previous bisphosphonate treatment 1 month before the examination and were able to cooperate well during the examination. The exclusion criteria were patients who has used bisphosphonates within the past month, inability to cooperate during the test and patients who were breastfeeding or pregnant.
All of the above patients were recruited under written consent. This study was approved by the hospital ethics committee and was conducted in compliance with the Helsinki Declaration. Before the 68Ga-DOTA-IBA PET/CT examination, patients had routine laboratory blood tests including routine blood counts, and liver and kidney function tests at 7 and 15 days to determine the impact of 68Ga-DOTA-IBA on biochemical parameters (Clinical Ethics Registration Number: ChiCTR2200064487).
2.8.2. 68Ga-DOTA-IBA PET/CT imaging
The patients were weighed before the examination. An intravenous injection of 68Ga-DOTA-IBA with a radiochemical purity of >97% at 0.1 mCi per kg body weight was given. Prior to injection, patients were advised to drink plenty of water and instructed to empty their bladder before imaging. Images were acquired at a tube voltage of 120 Kv, a tube current of 100 mAs and a layer thickness of 5.0 mm. The scanning ranged from the top of the head to the palms of both feet (whole body scan) and was acquired with the patients in a supine position. The foot advanced scanning mode involved a total acquisition of 10-11 beds at 120 s/bed with a subset number 33, an iteration number 3 and a 512×512 matrix. When the reconstruction was complete, the images were processed using PET/CT post-processing software.
2.8.3. Analysis of 68Ga-DOTA-IBA and 99mTc-MDP images
68Ga-DOTA-IBA and 99mTc-MDP images were analyzed independently in a double-blind manner by two nuclear medicine physicians with > 5 years of experience in diagnostic imaging. Based on the results of the 68Ga-DOTA-IBA and 99mTc-MDP imaging agents, the lesions were classified as osteolytic, osteogenic, mixed and normal bone. A lesion was defined as a region of abnormal radioactivity when the uptake value was > the surrounding normal bone background.
2.9. Statistical analysis
Statistical analysis was performed using SPSS 26.0. All quantitative information was expressed as the mean ± standard deviation. In the toxicity test, changes in the body weights of each group of mice were compared using repeated measures ANOVA. A P-value threshold of 0.05 was set to indicate statistical significance.
3. Results
3.1. Radiolabeling and quality control
68Ga-DOTA-IBA is a third generation bisphosphonate derivative that targets bone metastases. The structure of the 68Ga-DOTA-IBA is shown in Figure 1. The optimum preparation conditions were 10 μg (10 μL) of DOTA-IBA, 1 ml of sodium acetate solution and 4 ml of 68GaCl3 drench solution. The radiochemical purity of the prepared 68Ga-DOTA-IBA was >97%. The pH of the reaction was 4-5, the reaction temperature was 95°C and the reaction time was 15 min. Thin layer chromatography silica plates were used as a support and 0.1 M sodium citrate solution was used as a solvent to unfold the system. Quality control images of 68Ga-DOTA-IBA, 68Ga-DOTA-IBA at the origin (Rf=0.3-0.4), while the free 68GaCl3 moved in front of the strip with the solvent (Rf=0.9-1.0).
3.2. In vitro stability of 68Ga-DOTA-IBA
The in vitro stability data for 68Ga-DOTA-IBA under different conditions are summarized in Table 1. 68Ga-DOTA-IBA had good in vitro stability at room temperature (26 ± 2°C).
3.3. Plasma protein binding of 68Ga-DOTA-IBA
The PPB of freshly prepared 68Ga-DOTA-IBA under optimal labelling conditions was 80.8 ± 0.61% after 1 h incubation in plasma.
3.4. 68Ga-DOTA-IBA lipid water distribution coefficient
The logP of the lipid-water distribution coefficient of freshly prepared 68Ga-DOTA-IBA using the optimal labelling conditions was -2.26 ± 0.03. These data indicated that 68Ga-DOTA-IBA has a higher level of water solubility and is less lipid soluble.
3.5. 68Ga-DOTA-IBA animal toxicity test study
All groups of mice in the toxicity test groups showed no abnormalities in the basic health condition at 4 weeks after injection of 68Ga-DOTA-IBA. No significant differences in mouse weights were observed between the four experimental groups (P>0.05). At the end of the observation period, samples were isolated from the mice for pathological analysis. The data were discussed with a pathologist and no significant differences in the number, size, morphology and proportion of cells in the tissues of the mice in the high, medium and low dose groups were observed compared to the saline control (Figure 2 and Supplement Figure 1–3 HE).
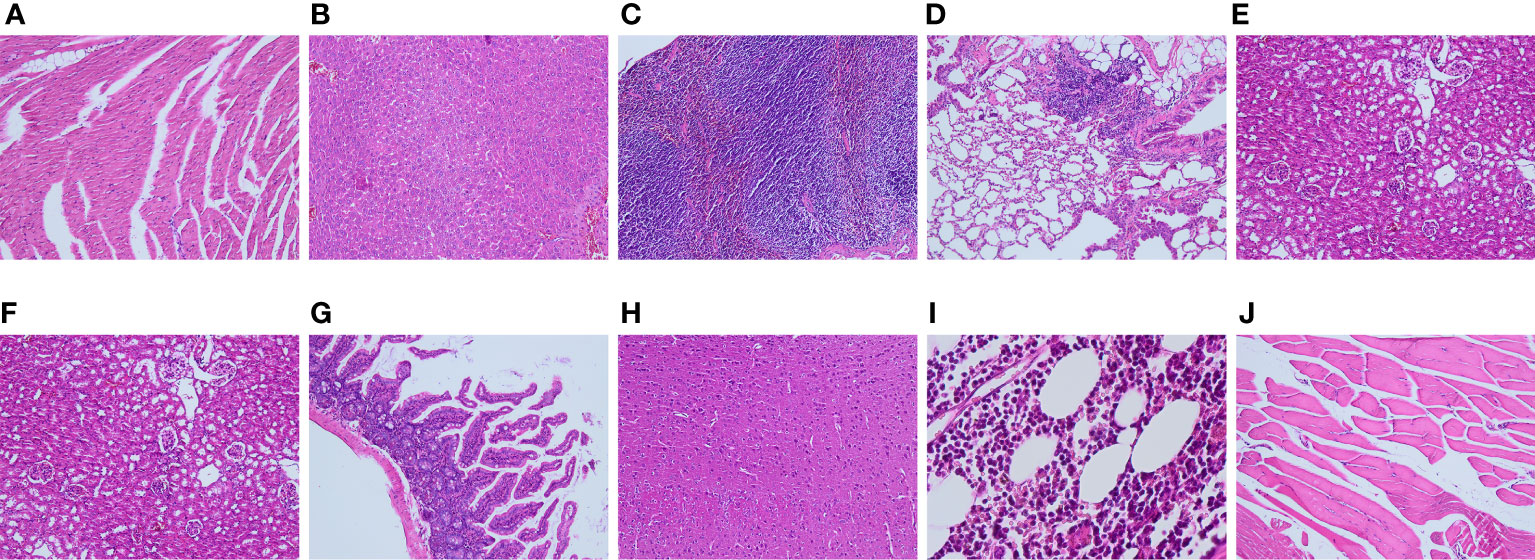
Figure 2 Tissue and organ pathology of mice injected with 1.0 mCi of 68Ga-DOTA-IBA (high dose group) for 4 weeks at different magnifications [(A): heart; (B): liver; (C): spleen; (D): lung; (E): kidney; (F): stomach; (G): intestine; (H): brain; (I): bone marrow; (J): muscle)].
3.6. In vivo distribution of 68Ga-DOTA-IBA in mice
The results of the in vivo distribution of 68Ga-DOTA-IBA studies in mice are summarized in Table 2. From Table 2, the blood clearance of 68Ga-DOTA-IBA was rapid with only 0.325 ± 0.103% ID/g blood retention at 4 h. Bone had a higher uptake of 68Ga-DOTA-IBA that reached a maximum after 2 h (8.365 ± 1.849% ID/g). Uptake was also high in the kidney as 68Ga-DOTA-IBA is mainly excreted through the urinary tract.
3.7. Imaging analysis of 68Ga-DOTA-IBA in New Zealand rabbits
Whole-body static bone imaging was performed in New Zealand rabbits at 1 and 3h after intravenous injection of freshly prepared 68Ga-DOTA-IBA 2.0 mCi under optimal labelling conditions via the ear margins as shown in Figure 3. 1 h after injection of 68Ga-DOTA-IBA, the rabbit’s urinary tract was clearly visualized and the whole body bone could be seen clearly with the whole spine and limb joints being the most visible. At 3 h after injection, the whole body bone remained clearly visible. In summary, 68Ga-DOTA-IBA is a radiopharmaceutical that is excreted through the kidney, and has rapid soft tissue clearance and high skeletal uptake with a long retention time in bone lesions.
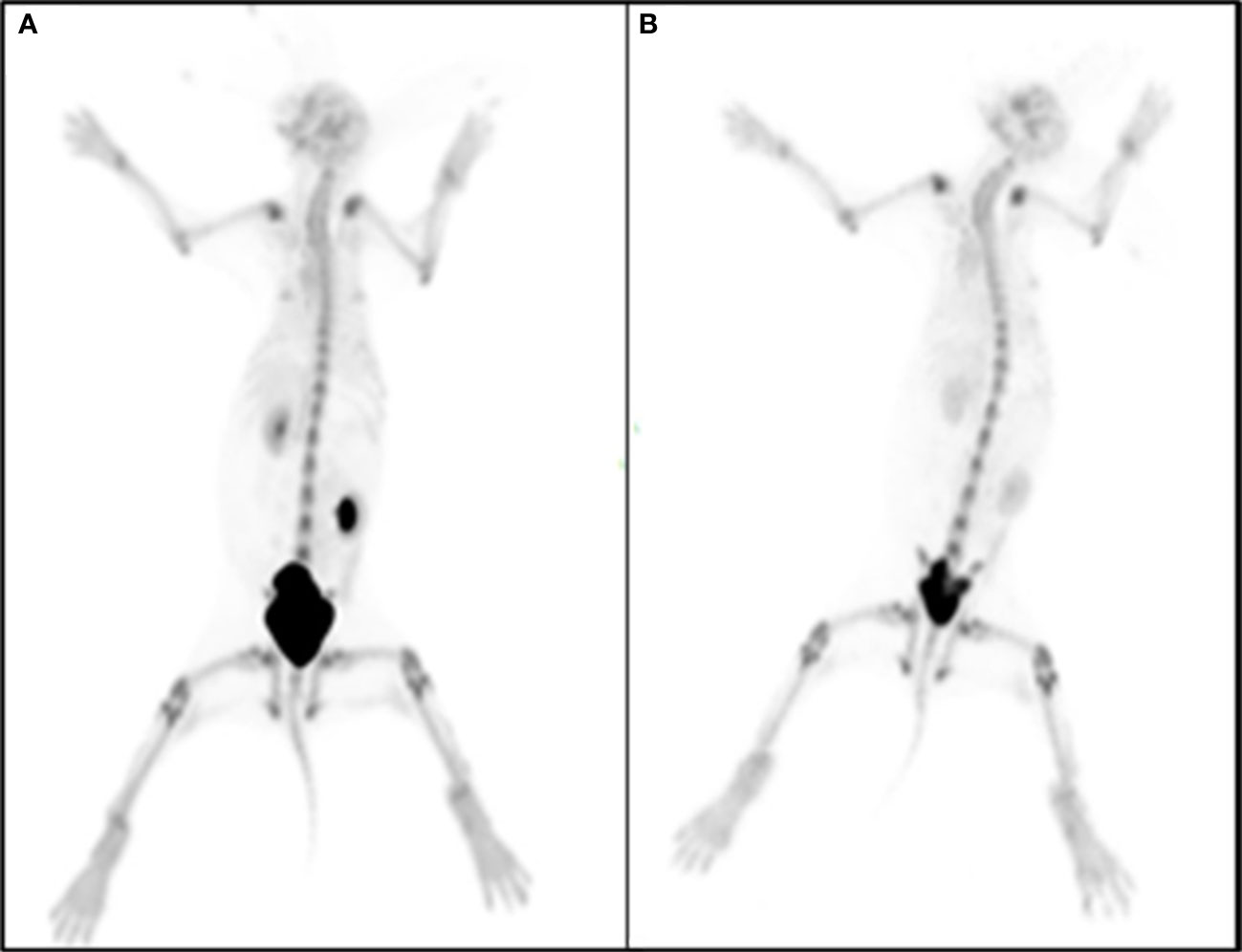
Figure 3 New Zealand rabbits were injected with 68Ga-DOTA-IBA 2.0 mCi at 1 h (A) and 3 h (B) after whole body bone visualization.
3.8. Imaging study of 68Ga-DOTA-IBA in mice
Whole-body bone images acquired at 30 min, 1.5 h and 3 h after tail vein injection of 68Ga-DOTA-IBA 0.2 mCi in mice are shown in Figure 4.
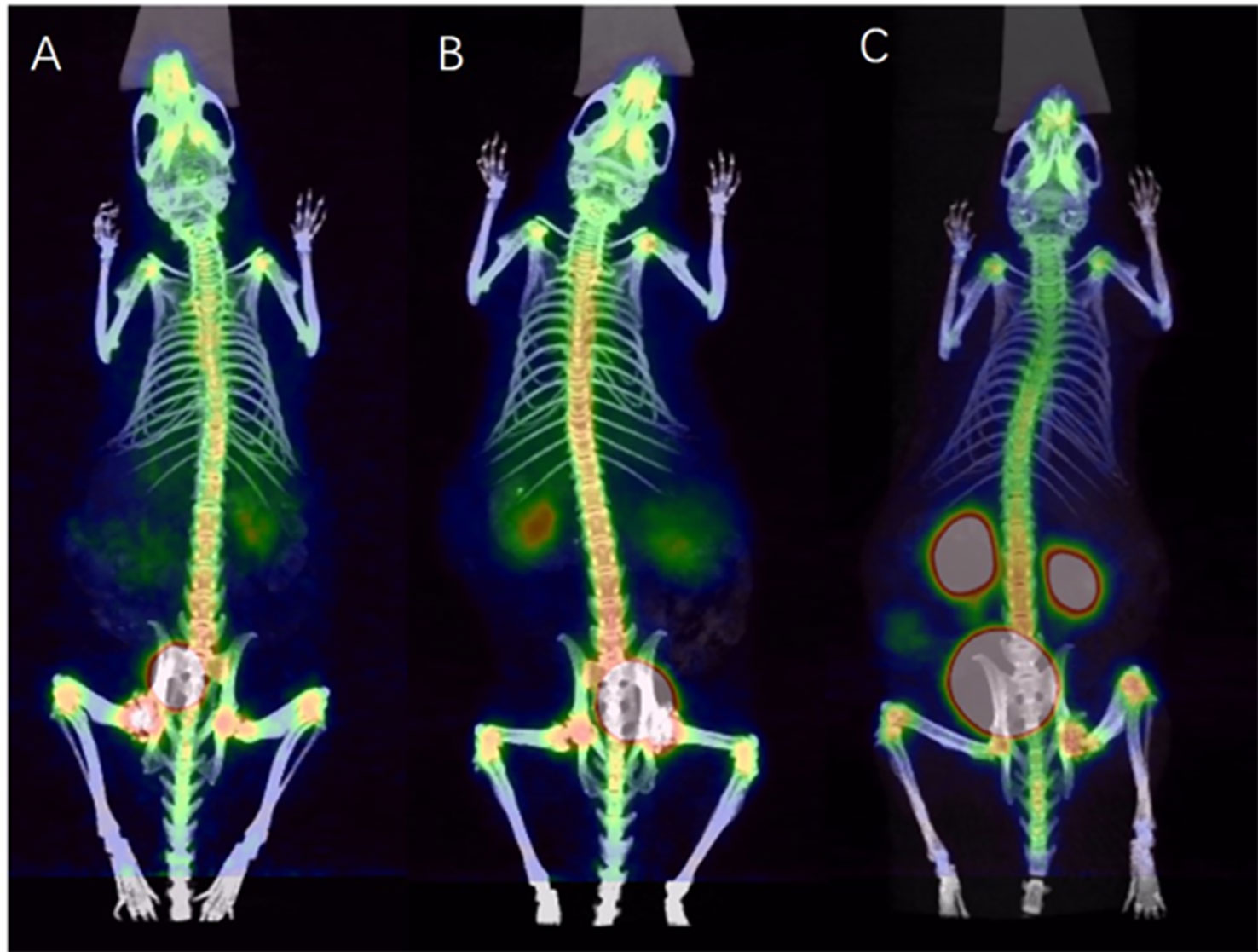
Figure 4 PET/CT images of 68Ga-DOTA-IBA in normal mice at different time points [(A): 30 min; (B):1.5 h; (C):3 h].
3.8. Imaging analysis of 68Ga-DOTA-IBA in a BALB/c nude mouse model
A whole-body bone image of a BALB/c nude mouse (PC-3) injected with 68Ga-DOTA-IBA 0.2 mCi from the tail vein at 1.5 h is shown in Figure 5. The model rat showed significant bone destruction in the left tibia with high developer uptake (the arrow) and a SUVmax of 10.3. The T/NT ratio was 6.3 for the lesion.
3.10. Preliminary imaging study of 68Ga-DOTA-IBA
A total of 5 patients were recruited to the study that consisted of two males and three females who were 34-66 years old with a mean age of 54.2 years. The patient information is summarized in Table 3.

Table 3 Summary of the basic patient information and clinical diagnosis for 99mTc-MDP imaging results with 68Ga-DOTA-IBA.
From the presented images, 68Ga-DOTA-IBA was comparable to 99mTc-MDP in the display of skeletal lesions. 68Ga-DOTA-IBA was more sensitive at showing small lesions compared to 99mTc-MDP. The targeted to non-targeted ratio (T/NT) was calculated for 99mTc-MDP SPETCT/CT as 3.2-5.3 and 5.8-9.1 for 68Ga-DOTA-IBA PET/CT indicating that 68Ga-DOTA-IBA PET/CT lesions had a high targeted to non-targeted (T/NT) ratio (Figures 6–9).
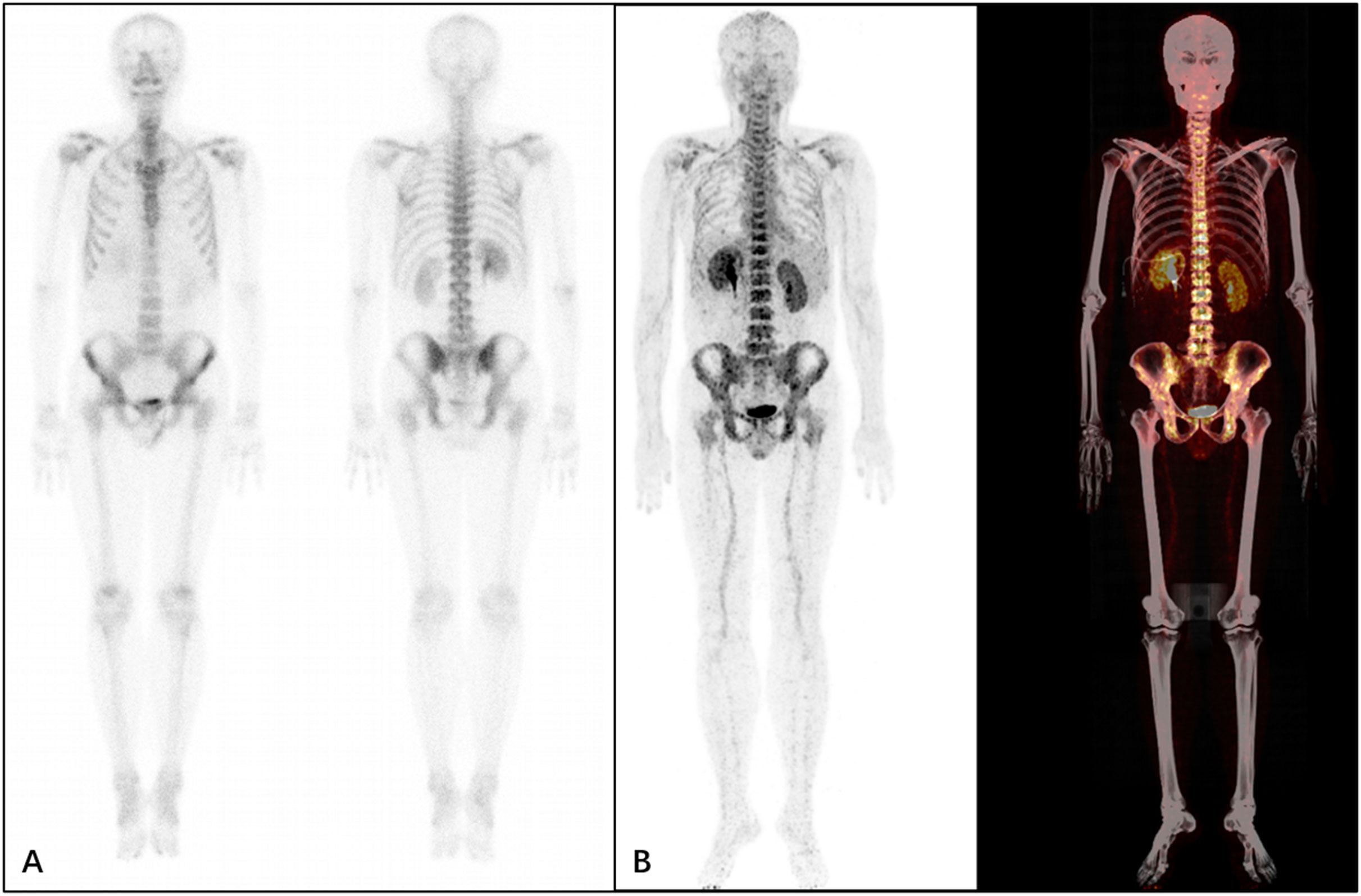
Figure 6 A 34-year-old male patient who underwent resection of a left lung tumor that was discovered 6 months previously. The patient underwent 99mTc-MDP (A) and68Ga-DOTA-IBA (B) imaging. It was observed from the MIP image that the patient’s whole body bones are clear with no abnormal concentrations of developer in the whole body bones.
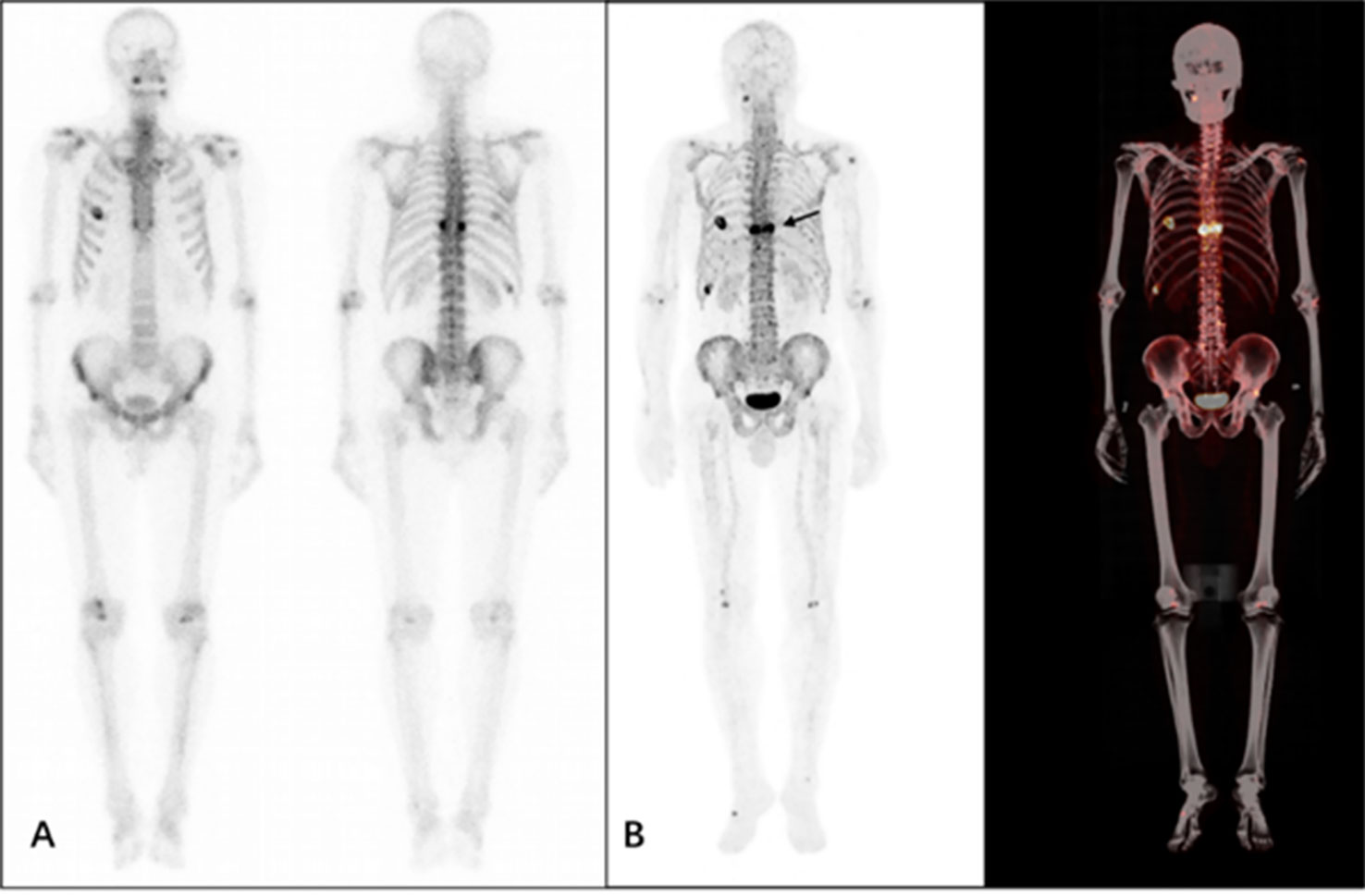
Figure 7 A 58-year-old male patient who underwent surgery for prostate cancer 2 months previously. The patient underwent 99mTc-MDP (A) and 68Ga-DOTA-IBA (B) imaging. From the MIP image, multiple foci of abnormal developer concentrations are visible in the thoracic spine and rib cage. The SUVmax of the lesion shown is approximately 7.8, T/NT 5.8 (arrow).
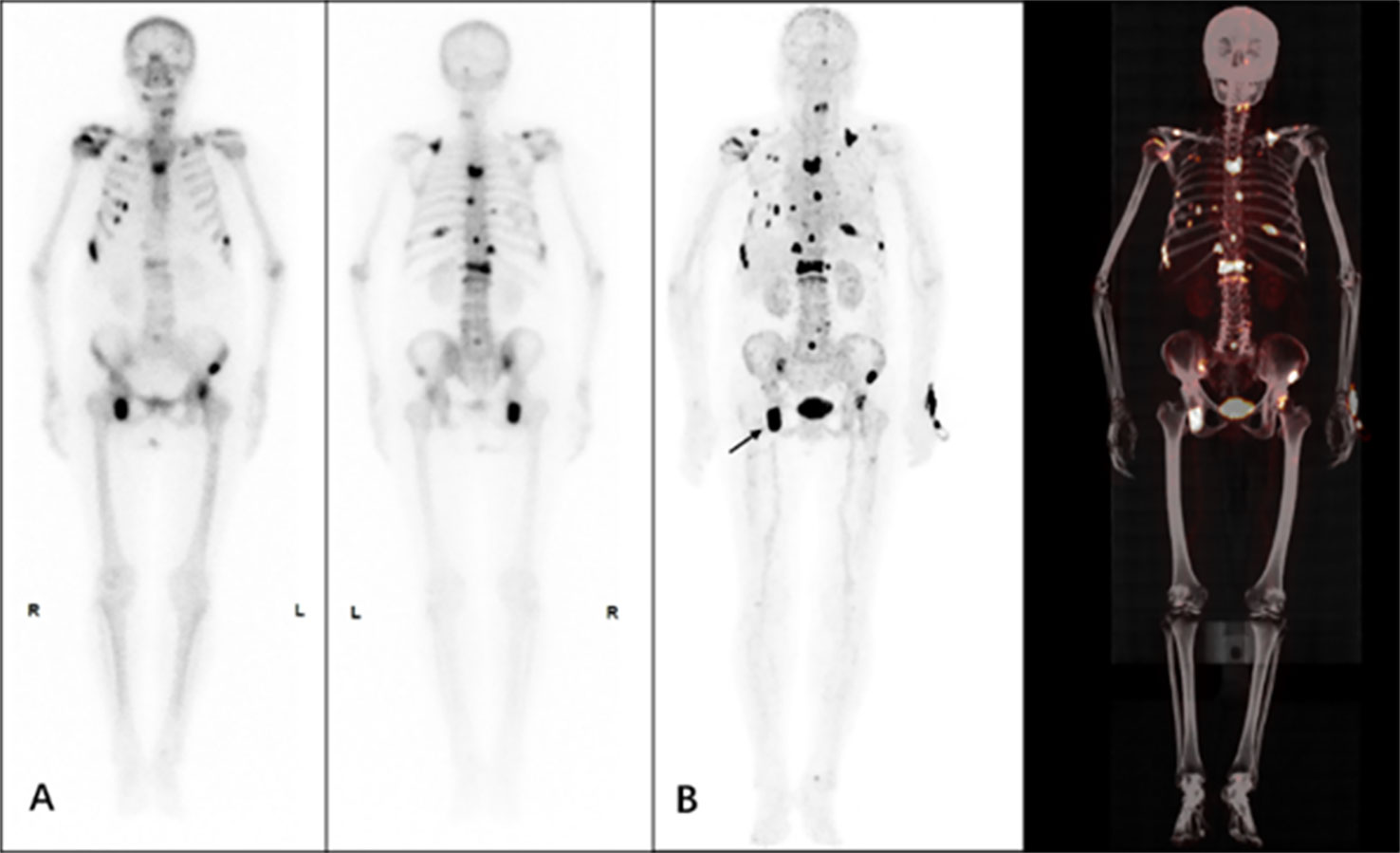
Figure 8 A 60-year-old female patient, who underwent surgery for left breast cancer 3 years previously who had recent generalized skeletal pain. The patient underwent 99mTc-MDP (A) and 68Ga-DOTA-IBA (B) imaging. From the MIP image multiple bone lesions were detected throughout the body with multiple foci of abnormal developer concentrations and a SUVmax of approximately 10.2 for the lesion, T/NT 9.1 (arrow).
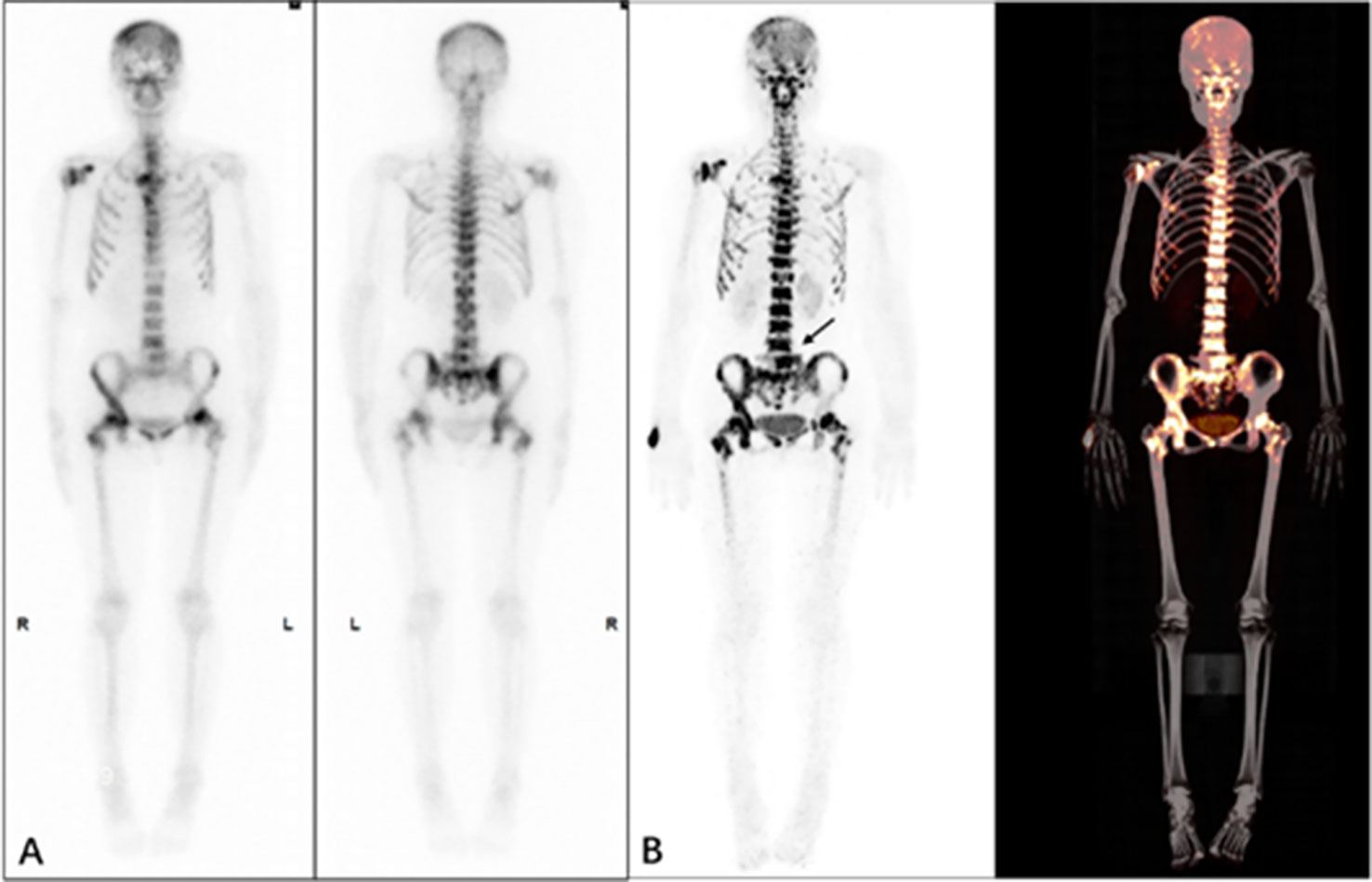
Figure 9 A 66-year-old female patient who underwent surgery for left breast cancer one year ago and now has generalized skeletal pain. The patient underwent 99mTc-MDP (A) and 68Ga-DOTA-IBA (B) imaging. From the MIP image, multiple foci of abnormal developer concentrations can be seen throughout the mesial bones and pelvic bones with a SUVmax of approximately 6.8 for the lesion, T/NT 5.2 (arrow).
4. Discussion
The clinical use of radiopharmaceuticals has led to improvements in the diagnosis and treatment of cancer and cardiovascular and cerebrovascular diseases (8–12). The continued development of novel radionuclides and molecular probes has led to their increased use in therapeutic radiology applications (13–17). Radionuclide bone imaging is currently the most commonly used method to evaluate abnormalities in bone metabolism. 99mTc-MDP and 18F-NaF are the most commonly used bone imaging agents in the diagnosis of bone metastases (8, 18–20). The amount of precursors used in the synthesis of radiopharmaceuticals is critically important in achieving high purity using the smallest amount of precursors for labelling. The DOTA-IBA used in this study is a new precursor and the addition of DOTA allowed the precursor dosage to be reduced to microgram levels. This can significantly reduce the side effects of bisphosphonates for the benefit of patients. Our results show that the radiochemical purity of the 68Ga-DOTA-IBA marker was >97% for the specific labelling conditions used. These findings demonstrate the high stability of the compound and its utility as an effective radiopharmaceutical.
Our in vitro data showed that 68Ga-DOTA-IBA is stable at room temperature (26 ± 2°C) and in saline after preparation and that it remains radiochemically pure at > 97% after 4 h. 68Ga-DOTA-IBA was less stable at room temperature (26 ± 2°C), in saline at 37°C and in serum but the overall stability was fair. The results of the plasma protein binding rate showed that 68Ga-DOTA-IBA had a higher plasma protein binding rate compared to 99mTc-MDP which has also been observed in other studies (6). These observations may be related to the large molecular weight of DOTA-IBA with a large MDP that can more effectively bind to plasma proteins.
The safety of radiopharmaceuticals is critically important and the aim is to minimize the required dose to maximize imaging or therapeutic efficacy. We showed that after the administration of different doses of 68Ga-DOTA-IBA, the mice were not significantly abnormal compared to animals in the control group. These data indicate that 68Ga-DOTA-IBA has low toxicity and a strong safety profile supporting its use for subsequent labelling of therapeutic nucleophiles. From the in vivo distribution of 68Ga-DOTA-IBA in mice, it can be seen that 68Ga-DOTA-IBA shows rapid blood clearance because 68Ga-DOTA-IBA is mostly excreted through the urinary tract, so the uptake by the kidneys is relatively high (this is related to the kidneys as the main excretory organ). We observed that bone has a high uptake of 68Ga-DOTA-IBA that is maintained for 4 h. It also has a high target to non-target ratio of 68Ga-DOTA-IBA suggesting that it has strong bone-targeting properties. The findings are consistent with the previous results of other 68Ga–bisphosphonate agents (5). In the mouse model imaging studies, 68Ga-DOTA-IBA had a high uptake at the lesion and a high target to non-target ratio.
DOTA-IBA contains DOTA and is convenient, efficient and highly labelled when chelating with metal ions and requires very low amounts of precursors. According to our imaging studies, 68Ga-DOTA-IBA was sensitive and had a high target to non-target ratio for bone metastases. If the nuclide is replaced by the therapeutic nuclide 177Lu/225Ac and labelled as 177Lu/225Ac -DOTA-IBA, it is expected that 68Ga/177Lu/225Ac-DOTA-IBA could potentially be used for the diagnosis and treatment of bone metastases.
Despite the interesting observations reported in this study, our approach has several limitations that need to be refined in future studies. Although 5 volunteers were recruited for the imaging study, the overall sample size in this study was small and did not allow for accurate diagnostic assessment. The comparative study of 68Ga-DOTA-IBA with sodium fluoride was not covered in this study and will be further explored in subsequent studies. Follow-up studies will be performed in larger patient cohorts to validate our findings to facilitate the widespread clinical application of our approach in patients with bone metastases.
5. Conclusions
In this study, a novel positron-labelled bisphosphonate radiopharmaceutical, 68Ga-DOTA-IBA, was successfully prepared. This new imaging agent is simple to prepare, requires a short reaction time, has a high label yield and is stable in vitro. Toxicity results showed that it is safe and non-toxic. 68Ga-DOTA-IBA has good bone targeting properties, has a high target to non-target ratio and is rapidly cleared from the body. Preclinical PET/CT images demonstrated that 68Ga-DOTA-IBA has a higher bone targeting and a higher target to non-target ratio for bone metastases. 68Ga-DOTA-IBA is a bone-friendly positron radiopharmaceutical with excellent properties that can be used for the imaging of bone metastases.
Data availability statement
The original contributions presented in the study are included in the article/Supplementary Material. Further inquiries can be directed to the corresponding authors.
Ethics statement
This study was performed in line with the principles of the Declaration of Helsinki. Study approval was obtained from the hospital’s ethics committee and conducted between September 2021 and August 2022 (Ethics committee approval No.: KY2022114) and (Clinical Ethics Registration Number: ChiCTR2200064487). The patients/participants provided their written informed consent to participate in this study.
Author contributions
All authors listed have made a substantial, direct, and intellectual contribution to the work and approved it for publication.
Funding
This study was supported in part by research foundation projects from Luzhou Science & Technology Department (20107) and The Affiliated Hospital of Southwest Medical University (20087).
Conflict of interest
The authors declare that the research was conducted in the absence of any commercial or financial relationships that could be construed as a potential conflict of interest.
Publisher’s note
All claims expressed in this article are solely those of the authors and do not necessarily represent those of their affiliated organizations, or those of the publisher, the editors and the reviewers. Any product that may be evaluated in this article, or claim that may be made by its manufacturer, is not guaranteed or endorsed by the publisher.
Supplementary material
The Supplementary Material for this article can be found online at: https://www.frontiersin.org/articles/10.3389/fonc.2022.1027792/full#supplementary-material
References
1. Chow E, Loblaw A, Harris K, Doyle M, Goh P, Chiu H, et al. Dexamethasone for the prophylaxis of radiation-induced pain flare after palliative radiotherapy for bone metastases: a pilot study. Support Care Cancer. (2007) 15(6):643–7. doi: 10.1007/s00520-007-0217-z
2. Gdowski AS, Ranjan A, Vishwanatha JK. Current concepts in bone metastasis, contemporary therapeutic strategies and ongoing clinical trials. J Exp Clin Cancer Res (2017) 36(1):108. doi: 10.1186/s13046-017-0578-1
3. Endo H, Hashimoto T, Fujioka M, Murata K. [A case of metastatic breast cancer showing extraosseous accumulation of 99mTc-HMDP in axillary lymph nodes on bone scintigraphy]. Nihon Igaku Hoshasen Gakkai Zasshi. (2001) 61(13):730–2.
4. Luckman SP, Hughes DE, Coxon FP, Graham R, Russell G, Rogers MJ. Nitrogen-containing bisphosphonates inhibit the mevalonate pathway and prevent post-translational prenylation of GTP-binding proteins, including ras. J Bone Miner Res (1998) 13(4):581–9. doi: 10.1359/jbmr.1998.13.4.581
5. Meckel M, Bergmann R, Miederer M, Roesch F. Bone targeting compounds for radiotherapy and imaging: *Me(III)-DOTA conjugates of bisphosphonic acid, pamidronic acid and zoledronic acid. EJNMMI Radiopharm Chem (2017) 1(1):14. doi: 10.1186/s41181-016-0017-1
6. Xu Q, Zhang S, Zhao Y, Feng Y, Liu L, Cai L, et al. Radiolabeling, quality control, biodistribution, and imaging studies of (177) Lu-ibandronate. J Labelled Comp Radiopharm. (2019) 62(1):43–51. doi: 10.1002/jlcr.3694
7. Xu T, Wang Y, Chen Z, Liu H, Yang S, Liu G, et al. Preparation, characterization, and preliminary imaging study of [(188)Re]Re-ibandronate as a novel theranostic radiopharmaceutical for bone metastasis. Contrast Media Mol Imaging. (2022) 2022:7684076. doi: 10.1155/2022/7684076
8. Love C, Din AS, Tomas MB, Kalapparambath TP, Palestro CJ. Radionuclide bone imaging: an illustrative review. Radiographics (2003) 23(2):341–58. doi: 10.1148/rg.232025103
9. Zhang C, Lin KS, Benard F. Molecular imaging and radionuclide therapy of melanoma targeting the melanocortin 1 receptor. Mol Imaging. (2017) 16:1536012117737919. doi: 10.1177/1536012117737919
10. Kendi AT, Moncayo VM, Nye JA, Galt JR, Halkar R, Schuster DM. Radionuclide therapies in molecular imaging and precision medicine. PET Clin (2017) 12(1):93–103. doi: 10.1016/j.cpet.2016.08.006
11. Partington SL, Valente AM, Landzberg M, Grant F, Di Carli MF, Dorbala S. Clinical applications of radionuclide imaging in the evaluation and management of patients with congenital heart disease. J Nucl Cardiol (2016) 23(1):45–63. doi: 10.1007/s12350-015-0185-5
12. Zhuang H, Duarte PS, Pourdehnad M, Maes A, Van Acker F, Shnier D, et al. The promising role of 18F-FDG PET in detecting infected lower limb prosthesis implants. J Nucl Med (2001) 42(1):44–8.
13. Zidan L, Iravani A, Oleinikov K, Ben-Haim S, Gross DJ, Meirovitz A, et al. Efficacy and safety of (177)Lu-DOTATATE in lung neuroendocrine tumors: A bicenter study. J Nucl Med (2022) 63(2):218–25. doi: 10.2967/jnumed.120.260760
14. Satapathy S, Bhattacharya A, Sood A, Kapoor R, Gupta R, Sood A, et al. Hematological markers as predictors of treatment outcomes with lutetium 177 ((177)Lu)-DOTATATE in patients with advanced neuroendocrine tumors. Cancer Biother Radiopharm. (2022) 37(1):23–9. doi: 10.1089/cbr.2021.0053
15. Makis W, McCann K, McEwan AJ. Extraventricular neurocytoma treated with 177Lu DOTATATE PRRT induction and maintenance therapies. Clin Nucl Med (2015) 40(3):234–6. doi: 10.1097/RLU.0000000000000668
16. Umbricht CA, Benesova M, Schmid RM, Turler A, Schibli R, van der Meulen NP, et al. (44)Sc-PSMA-617 for radiotheragnostics in tandem with (177)Lu-PSMA-617-preclinical investigations in comparison with (68)Ga-PSMA-11 and (68)Ga-PSMA-617. EJNMMI Res (2017) 7(1):9. doi: 10.1186/s13550-017-0257-4
17. Heinzel A, Boghos D, Mottaghy FM, Gaertner F, Essler M, von Mallek D, et al. (68)Ga-PSMA PET/CT for monitoring response to (177)Lu-PSMA-617 radioligand therapy in patients with metastatic castration-resistant prostate cancer. Eur J Nucl Med Mol Imaging. (2019) 46(5):1054–62. doi: 10.1007/s00259-019-4258-6
18. Bassett LW, Gold RH, Webber MM. Radionuclide bone imaging. Radiol Clin North Am (1981) 19(4):675–702. doi: 10.1016/S0033-8389(22)01349-5
19. Panagiotidis E, Lam K, Mistry A, Seshadri N, Vinjamuri S. Skeletal metastases and benign mimics on NaF PET/CT: A pictorial review. AJR Am J Roentgenol (2018) 211(1):W64–74. doi: 10.2214/AJR.17.19110
Keywords: DOTA-IBA, 68 Ga, PET/CT, 99m Tc-MDP, SPECT
Citation: Wang Y, Wang Q, Chen Z, Yang J, Liu H, Peng D, Lei L, Liu L, Wang L, Xing N, Qiu L, Feng Y and Chen Y (2022) Preparation, biological characterization and preliminary human imaging studies of 68Ga-DOTA-IBA. Front. Oncol. 12:1027792. doi: 10.3389/fonc.2022.1027792
Received: 25 August 2022; Accepted: 01 November 2022;
Published: 14 December 2022.
Edited by:
Francoise Degoul, Institut National de la Santé et de la Recherche Médicale (INSERM), FranceReviewed by:
Nan Li, Beijing Cancer Hospital, ChinaFrancoise Degoul, Institut National de la Santé et de la Recherche Médicale (INSERM), France
Copyright © 2022 Wang, Wang, Chen, Yang, Liu, Peng, Lei, Liu, Wang, Xing, Qiu, Feng and Chen. This is an open-access article distributed under the terms of the Creative Commons Attribution License (CC BY). The use, distribution or reproduction in other forums is permitted, provided the original author(s) and the copyright owner(s) are credited and that the original publication in this journal is cited, in accordance with accepted academic practice. No use, distribution or reproduction is permitted which does not comply with these terms.
*Correspondence: Lin Qiu, cWl1bGluMTcxMTEyMTAwNDFAMTYzLmNvbQ==; Yue Feng, ZmVuZ3l1ZTIwMEAxNjMuY29t; Yue Chen, Y2hlbnl1ZTU1MjNAMTI2LmNvbQ==
†These authors have contributed equally to this work