- 1Department of Internal Medicine, Faculty of Health Sciences, University of the Witwatersrand, Johannesburg, South Africa
- 2Department of Internal Medicine, Common Epithelial Cancer Research Centre, Faculty of Health Sciences, University of the Witwatersrand, Johannesburg, South Africa
- 3Hematology/Oncology Division, School of Medicine, Indiana University, Indianapolis, IN, United States
- 4Department of Biochemistry and Molecular Medicine, School of Medicine and Health Science, The George Washington University, Washington, DC, United States
- 5Department of Anatomical Pathology, School of Pathology, Faculty of Health Sciences, University of the Witwatersrand, Parktown, Johannesburg, South Africa
Colorectal cancer (CRC) is the second leading cause of cancer-related deaths globally, with nearly half of patients detected in the advanced stages. This is due to the fact that symptoms associated with CRC often do not appear until the cancer has reached an advanced stage. This suggests that CRC is a cancer with a slow progression, making it curable and preventive if detected in its early stage. Therefore, there is an urgent clinical need to improve CRC early detection and personalize therapy for patients with this cancer. Recently, liquid biopsy as a non-invasive or nominally invasive approach has attracted considerable interest for its real-time disease monitoring capability through repeated sample analysis. Several studies in CRC have revealed the potential for liquid biopsy application in a real clinical setting using circulating RNA/miRNA, circulating tumor cells (CTCs), exosomes, etc. However, Liquid biopsy still remains a challenge since there are currently no promising results with high specificity and specificity that might be employed as optimal circulatory biomarkers. Therefore, in this review, we conferred the plausible role of less explored liquid biopsy components like mitochondrial DNA (mtDNA), organoid model of CTCs, and circulating cancer-associated fibroblasts (cCAFs); which may allow researchers to develop improved strategies to unravel unfulfilled clinical requirements in CRC patients. Moreover, we have also discussed immunotherapy approaches to improve the prognosis of MSI (Microsatellite Instability) CRC patients using neoantigens and immune cells in the tumor microenvironment (TME) as a liquid biopsy approach in detail.
Introduction
Colorectal cancer (CRC) is third in terms of the most common (6.1%) and second in terms of deadly (9.2%) disease worldwide. It is estimated that by the year 2035, the total number of deaths from rectal and colon cancer will increase by 60% and 71.5%, respectively. Overall survival (OS) 5 years after primary diagnosis in stage I–II is 87-90%, decreasing to 68–72% in stage III, and futher lowering to 11–14% in stage IV metastatic CRC (mCRC) (1–5). Today therapeutic algorithms for CRC contain endoscopic and surgical resection, systemic adjuvant chemotherapy, radiation therapy, targeted therapy, and immunotherapy (6, 7). Due to the poor response of numerous colorectal patients to existing therapeutic approaches and since CRC survival is highly dependent on primary diagnosis and early treatment, a known significant biomarker that can predict the beneficial response as early as possible is immediately required. To date, tissue biopsy is one of the best standard options for tumor identification. Though, the main drawback is that it is problematic to screen disease development over frequent biopsies due to recurrent injury and poor patient compliance. Tissue removal also carries hazards, and it is unapproachable for some cancer cases (8, 9). Also, biopsy has some significant boundaries: it is invasive, expensive, painful has technical boundaries related to tumor location, and is not effective in pointing to tumor cells subpopulations (10–12). Indeed, there is a critical need to find a minimally invasive or non-invasive method to screen the high-risk population and detect CRC presence in asymptomatic patients at an earlier and curable stage.
The awareness of liquid biopsy is that of identifying circulating biomarkers to distinguish cancer cells released from the primary tumor and/or metastasis sites (13–15). The meaning of ‘liquid biopsy’ describes the importance of identifying cancer-derived biomarkers in blood or other body fluids, such as stool, saliva, cerebrospinal fluid or urine (16–22). The very noteworthy targeted constituent studied in liquid biopsy is circulating tumor DNA (ctDNA), circulating tumor cells (CTC), circulating tumor RNAs, and exosomes (23–27). Although they are the most studied component for liquid biopsy, CTCs alone cannot be considered as a clinical diagnostic tool due to the debate over their clinical utility (28). However, it has been reported that tumor cells communicate not only with additional malignant cells, but also with the constituent of the tumor microenvironment (TME), suggesting their stability in circulation is highly reliable on TME (29, 30). So, here we hypothesized that CTC research should be commenced concurrently with other TME components, such as, cancer associated fibroblasts (CAFs), various immune cells, extra-cellular vesicles (EVs) etc. Furthermore, another noninvasive approach being studied is the use of ctDNA, exosomal miRNAs, and proteomics; which though in primary stages, needs to be elucidated in-depth. Additionally, we have highlighted the benefits of immunotherapy treatment for MSI-high (MSI-H) CRC patients and use of neoantigens and immune cells as a liquid biopsy approach for better prognosis.
Overall in this review, we have described the concept of liquid biopsy and its applications in the management of CRC patients (Figure 1). Furthermore, we have highlighted the role of less explored components, such as organoid models of CTC, immune cells in TME, mitochondrial DNA (mtDNA), and neoantigens in the liquid biopsy approach. These approaches could be used noninvasively to gain knowledge about molecular characterization and the mechanism of disease progression in CRC.
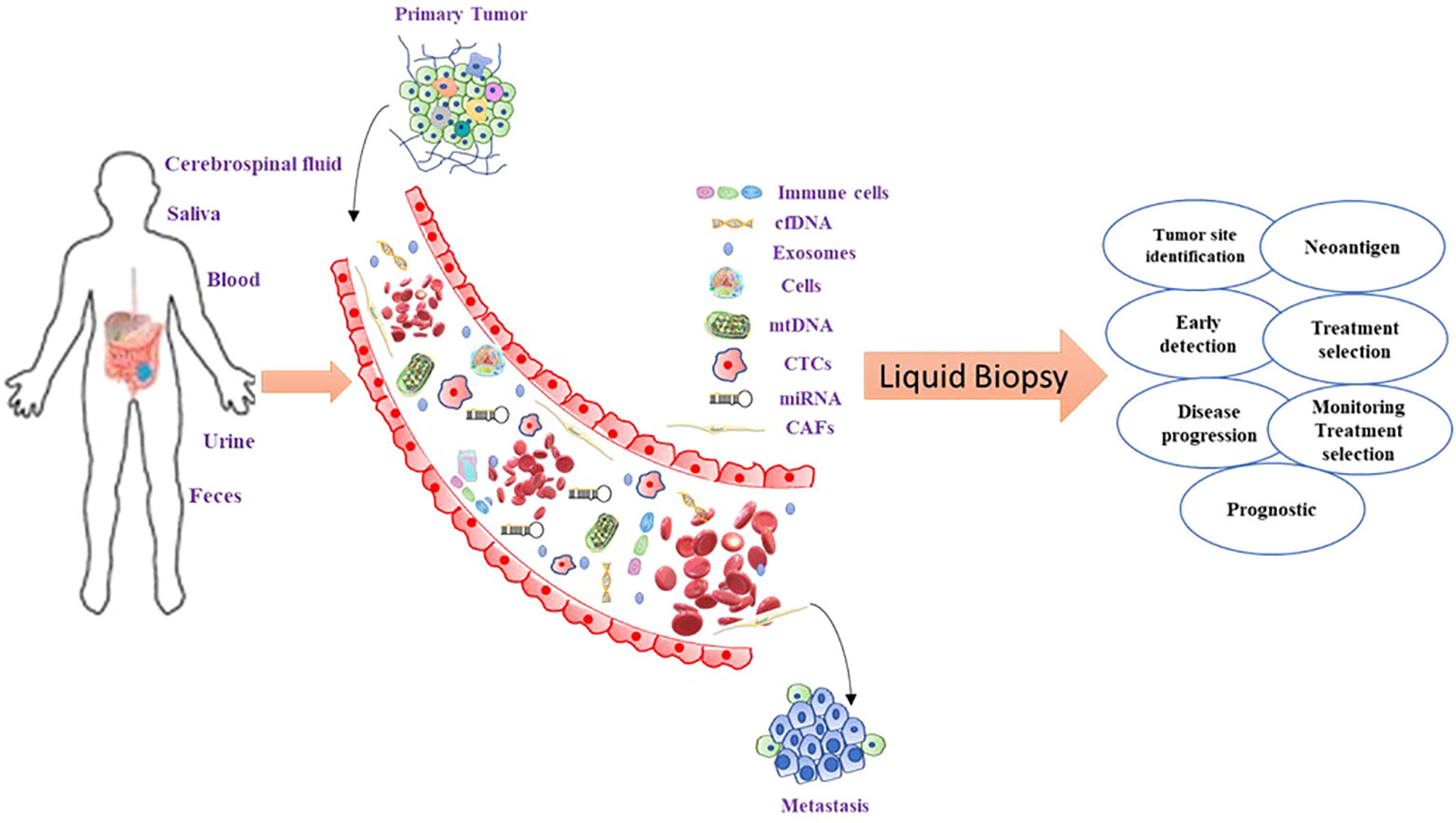
Figure 1 Liquid biopsy components in CRC and their clinical utility. CTCs are shedded from the tumor into the blood vessels where they can release their components: nucleic acids and exosomes with tumor-specific cargo material. For the analysis of these molecules, blood can be taken out, and plasma or serum further processed for the extraction of the desired constituents. From the blood circulation, these molecules can be filtered into saliva and urine which can also be collected and further analyzed. Each of these constituents delivers one or more levels of tumor information. The quantity of the concentration of single proteins or panels including numerous tumor proteins is the present gold standard in cancer management.
Tumor-microenvironment components
The awareness of the tumor microenvironment (TME) has been proposed more than one hundred years ago. In the meantime, cancer research has discovered many several noteworthy roles of TME components not only in cancer metastasis, but also in cancer metabolism and development (31–33). TME consists of a web of cancer cells, stromal cells, immune cells, CAFs, exosomes, and extracellular matrix. In this composition, immune cells and stromal cells are the two major non-tumor cell types in addition to tumor cells (34). Interestingly, TME Web found it possible to achieve immune organization of cancers concerning prognosis, chemotherapy, and prediction of immunotherapy response (35–37). In the current scenario, several studies on cancer have shown that TME meaningfully affects cancer cell proliferation and development and recommends potential worth in the diagnosis and prediction of cancer prognosis (38–40). In addition, it has been suggested that TME is highly affected by the development of CRC (41–43). Furthermore, the TME components play significant roles in defining CRC with poor prediction and immune escape (44, 45). Together, the significant function of TME in the development and metastasis of CRCs and the investigation of the essential molecular mechanism that makes the interaction between the transformation of TME and the progression of CRCs have fascinated important considerations over the past era. But until now, a comprehensive understanding of the TME in CRC development and metastasis has yet to be discovered.
Circulating tumor cells and circulating cancer-associated fibroblasts: Symbiotic siblings and potential drug targets
CTCs are the representative of the cancer cells detached from the primary tumor which enter into the circulatory system (blood, lymphatic system) to cause metastasis (46, 47). Undoubtedly, CTCs have been used as a dynamic component of liquid biopsies to investigate the presence of residual cancer cells, track treatment response, and prediction of disease recurrence, which is suggestive of the fact that CTCs could play a critical role in the early diagnosis and prognosis of various cancers, including the development of personalized therapeutic options (48–53). Compared to other cancer biomarkers, CTCs are cancer cells that could carry biological and molecular evidence of cancer cells that supports single-cell analysis and directly provide information about ongoing alterations in cancer cells at all different stages of disease progression (54–56). Based on the evidence, CTCs have a favorable role in early prediction, therapeutic observation, and disease progression and would be a significant drug target for various cancers (57–60). The existence of clusters of CTCs has been reported during the last decade, and several groups have described the clinical relevance of CTC clusters. Although the prognostic value of CTC has been well validated, limitations are preventing the use of CTC enumeration in routine clinical practice concerning the use of CTCs either as a clinical marker for early cancer detection, or as a surrogate endpoint in interventional studies (61). These limitations include uncertainty about the specificity of CTC detection assays and justifiable concerns that CTC detection alone may be misleading or inadequate, especially when applied in the early detection of metastases. Additional biomarker assays can enhance the specificity and broaden the application of “liquid biopsies” in early cancer detection, monitoring disease progression, and determining response to therapy (Figure 2). To relate to a single cancer cell, CTC clusters are comparatively low and rare in circulation, but reveal noteworthy, better resistance to apoptosis and additional metastatic potential (62–64). Likewise, research on clusters of CTCs in the peripheral blood of patients with CRC has revealed that the clusters of CTCs are not malignant, but relatively tumor-derived endothelial cells connected to the vascular features; particularly, the separation and counting of these clusters of CTCs can distinguish between healthy individuals and patients with early-stage CRC with a high degree of precision (IIa) (48, 65).
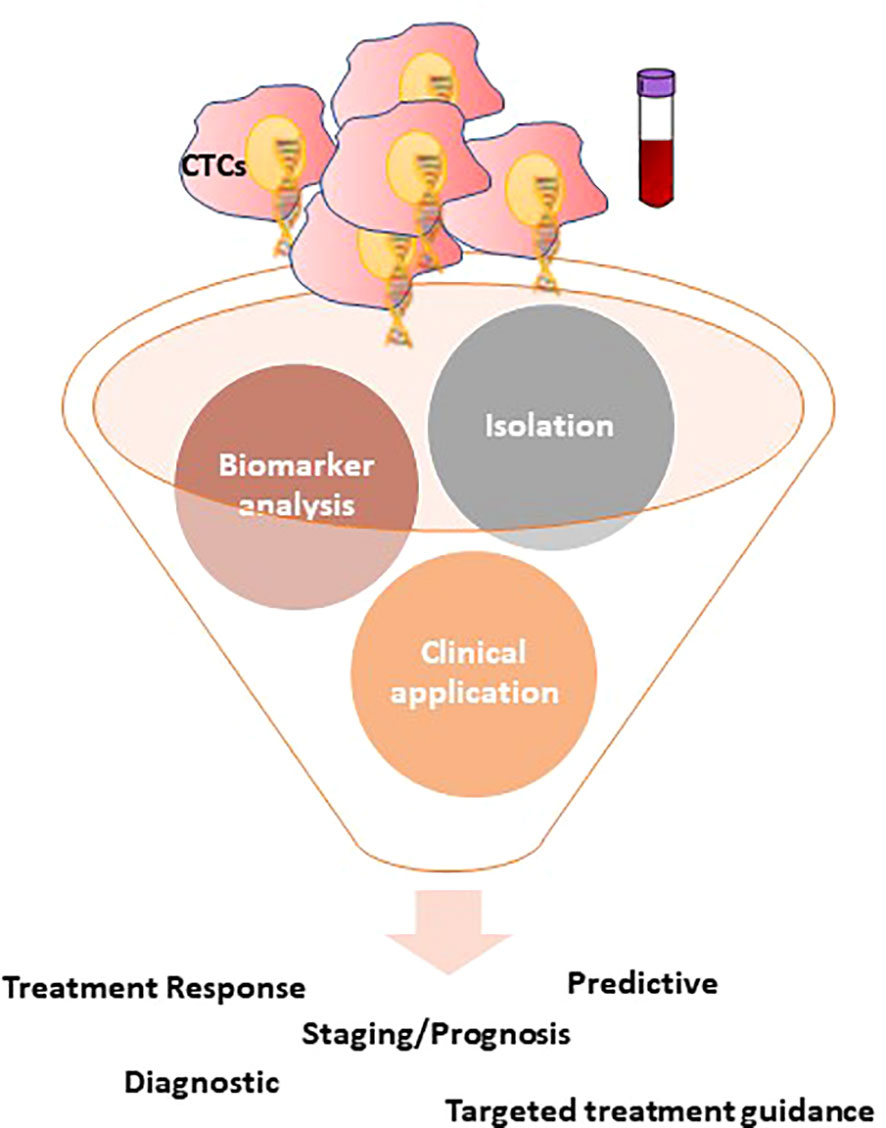
Figure 2 Overview of the CTCs detection technologies and the potential clinical applications of CTCs in CRC. CTCs isolation can usually be divided into two groups: physical isolation designed to exploit the differing physical belongings of blood components, such as size, deformity, and charge; and biological isolation, often utilizing antibody-based capture methods to enrich CTCs or deplete various blood cells. Following isolation, CTCs are open to a variety of downstream applications, focusing primarily on one of three categories: enumeration, characterization, and expansion.
Because CTCs can be detected in the peripheral blood of cancer patients, it follows that a “liquid biopsy” to detect tumor components in blood will not only contain tumor cells but will also contain other cellular components of TME. Cancer-associated fibroblasts (CAFs) – responsible for cancer cell proliferation, migration, invasion, drug resistance, and other important biological processes through secretion of cytokines, chemokines, and growth factors - are a heterogeneous population and an essential component of cells in TME (Figure 3) (66). Various studies have revealed their inevitable role in the regulation of almost all hallmarks of cancer, resulting in tumor progression and metastasis (67–69). According to Dr. Paget’s seed and soil theory, the seed has been repeatedly studied as cancer stem cells (CSCs), resident? cancer cells, and more recently as CTCs; whereas soil is represented as the TME (70). It is very well known that in the CTC population, most CTCs die at an early stage when they enter the circulation due to the collective effects of environmental and mechanical factors, for example, oxidative and sheer stress and immune response (48, 71). Consequently, only a few drug-resistant cells can escape and spread by undergoing a series of modifications to survive the changing environment. By looking at this theory, it is proposed that caves form clusters with CTCs to provide a suitable TME to CTCs and/or CTSCs (circulating tumor stem cells) for their persistence during metastasis in the circulation.
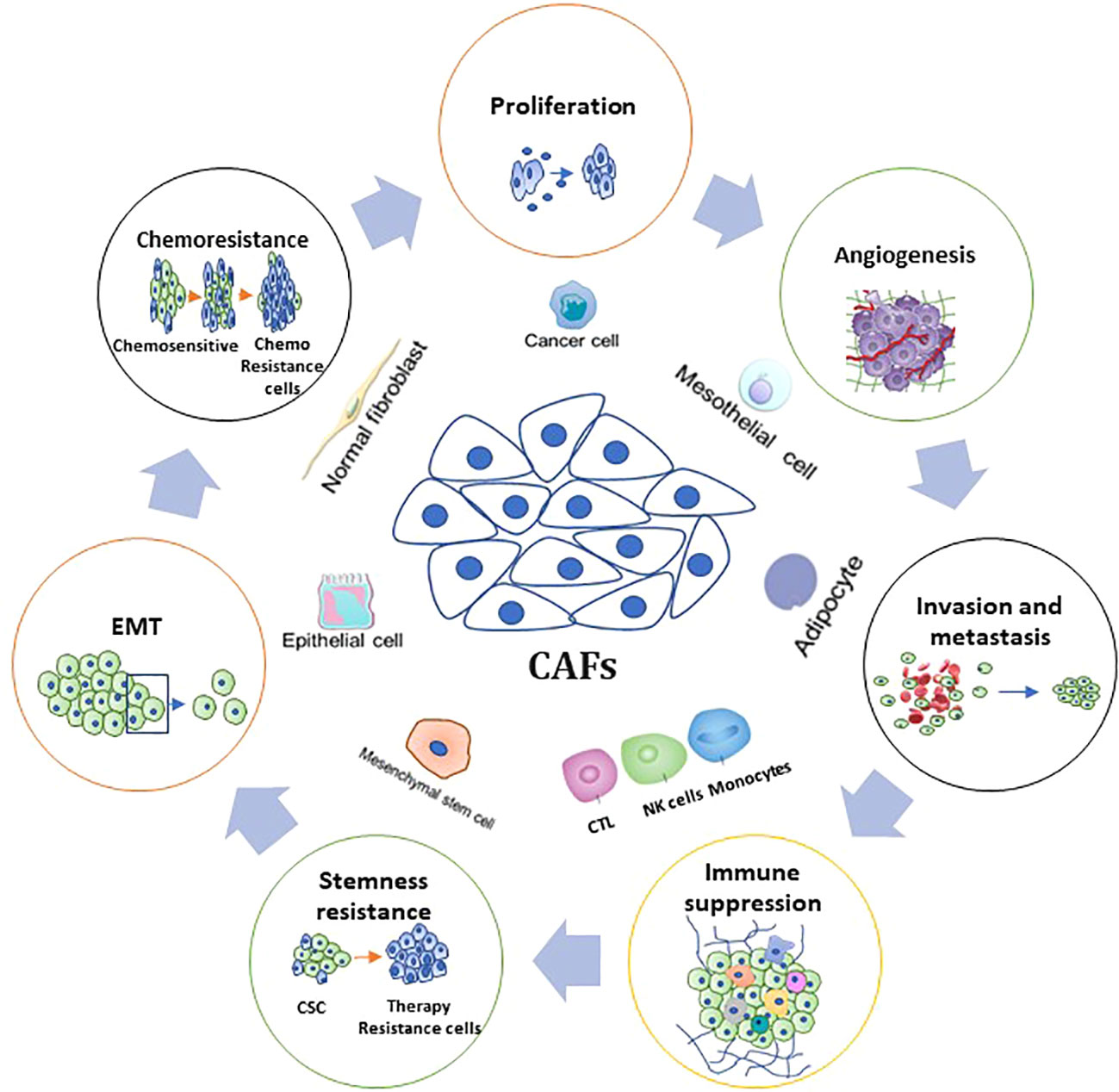
Figure 3 Fundamental Functions and associated mechanisms of CAFs in CRC hallmarks. The figure shows the role of CAFs in CRC biology, including tumorigenesis, proliferation, angiogenesis, invasion and metastasis, stemness, therapy resistance, and tumor immunity.
In CRC, various studies have reported clinical applications of CTC for early diagnosis, prognosis, and treatment monitoring using different techniques (48). In addition to this, recent studies revealed the importance of the CTC cell line in classifying cancer-associated proteins (neoantigens) and pathways connected to cancer cell stemness and metastasis, as well as in assessing anticancer drug sensitivity (72–76). Agarwal et al. identified clusters of cCAFs/CTCs and discovered that the cumulative number of these clusters is associated with cancer growth and metastasis (77). Although these studies have shown the presence of CAFs outside of the primary tumor site or metastatic lesions, there has been little direct evidence showing the presence of CAFs in the circulation of cancer patients in a clinical setting. In addition to this, several biomarkers, genes, and proteins have been extremely highly expressed in CAFs and also have poorer disease progression and overall survival in CRC (Figure 4). To date, the importance and use of CTCs in clinical setting for CRC is increasingly being established (Table 1) (26, 78–88), but the low population and vast heterogeneity of CTCs in addition to the progress of diagnosis and analysis approaches have few common approvals to use CTCs as a new biomarker. Thus, impeding cCAF/CTC complex formation or dismantling them, as well as clusters with other types of cells, may open new frontiers for controlling cancer or preventing metastasis.
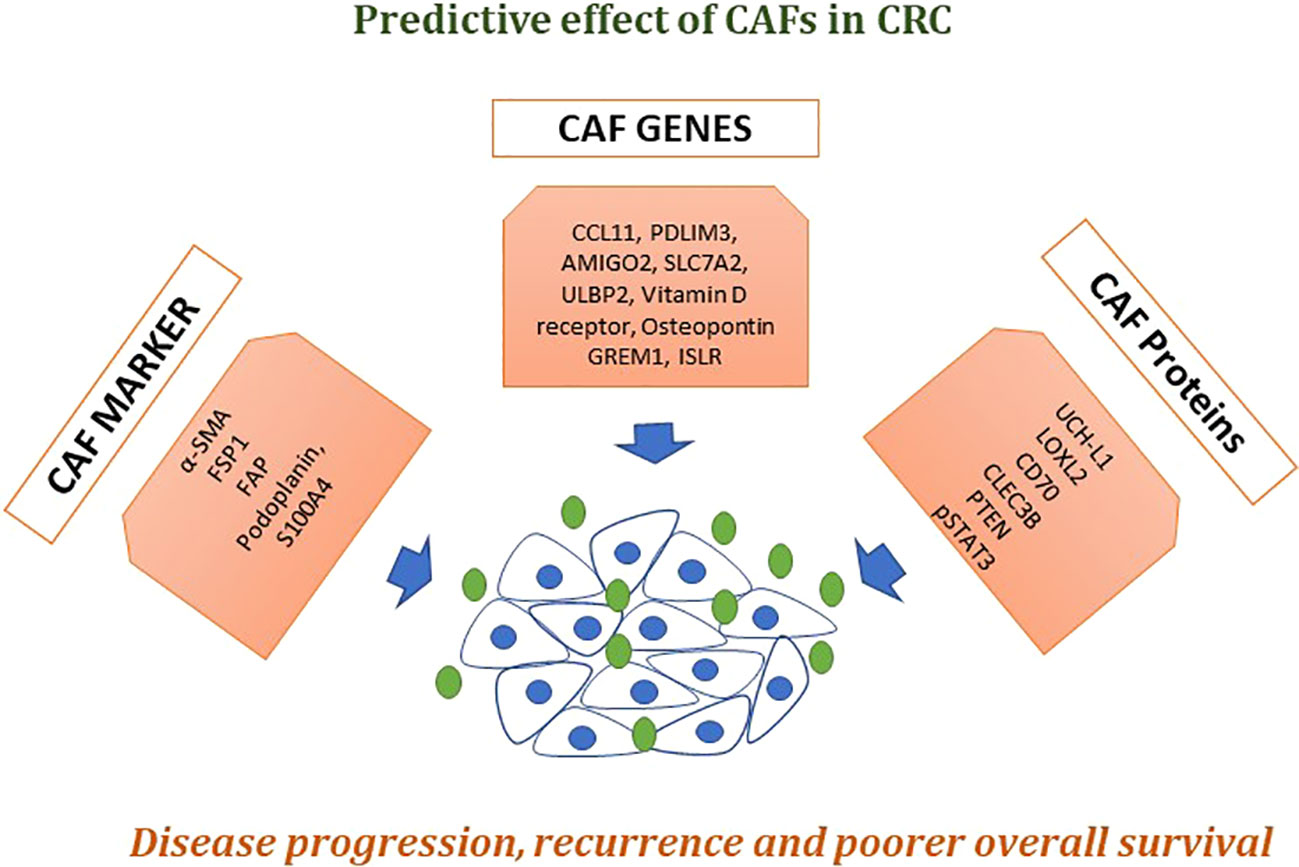
Figure 4 Effects of CAFs in CRC. A numeral of biomarkers that are extremely expressed in CAFs, like α-SMA, fibroblast activation protein alpha (FAP), fibroblast-specific protein 1 (FSP-1), platelet-derived growth factor receptor-α (PDGFRα) and PDGFRβ have now been commonly used to classify or isolate CAFs from the pool of fibroblasts present in the whole body. Described genes and proteins showed poorer disease progression, recurrence-free survival, and overall survival. Taken together, these markers could be used as liquid biopsy approach for early detection and treatment prognosis in CRC patients.
Exosomal miRNAs/ctDNA/cfDNA
A stimulating realm of tumor research has advanced over the past decade by concentrating on extracellular vesicles (EVs), known as exosomes, to answer pivotal challenges around therapeutics, diagnosis, and prevention. Exosomes are known as vesicles formed via the endocytic pathway and ranging in size from 30-140nm in diameter. As a new significant focus on the enigma of cancer, exosomes signify a noteworthy characteristic of biological signaling between cells and are also used as novel biomarker identification strategies (89–91). In addition, numerous studies have discovered that body fluids harbor abundant quantities of EVs, the constituent’s quantity of which varies based on the physiological or pathological state of an individual (92, 93). These diverse populations of extracellular? vehicles transfer detailed cargo such as miRNA, proteins, and lipids from one cell to another to stimulate a specific response. Exosomes can be found in all body fluids and can be detected in liquid biopsies (94). This section focuses primarily on exosomes containing miRNAs, proteins, and mRNA that appear to be consistently altered in patients with CRC. To date, there are only a minority of publications aimed at understanding exosomes in relation to CRC. EVs released from CRC cells can reveal vital evidence about significant molecules and signaling pathways involved in the growth and development of CRC (95, 96). Thus, the existence of tumor-derived EVs in circulating body fluids makes them prospective innovative biomarkers for early prognosis, diagnosis, and prediction of CRC cancer.
Exosomes have a prominent role in cell proliferation, metastasis, and epithelial-to-mesenchymal transition (EMT), as well as by supporting the angiogenic switch and the remodeling of the extracellular matrix (ECM) in CRC (97, 98) (Figure 5). In recent research, it was observed that CRC cells released more exosomes in hypoxic conditions (99, 100). Besides, these exosomes encourage cell proliferation via shortening the mitosis period and triggering STAT3 signaling in CRC (95, 101). Furthermore, Mulvey et al. demonstrated that co-culture of the CRC HCT116 cell line exosomes with normal colon cells can increase its clonogenicity (102). Numerous cellular components in exosomes have been reported that could contribute to CRC metastasis through various molecular mechanisms. A recent research report suggested that glycoprotein A repetition-dominant (GARP) knockdown Mesenchymal stroma/stem-like cells prevent the cell proliferation and invasion of mouse colorectal cancer cells through exosomes (103).
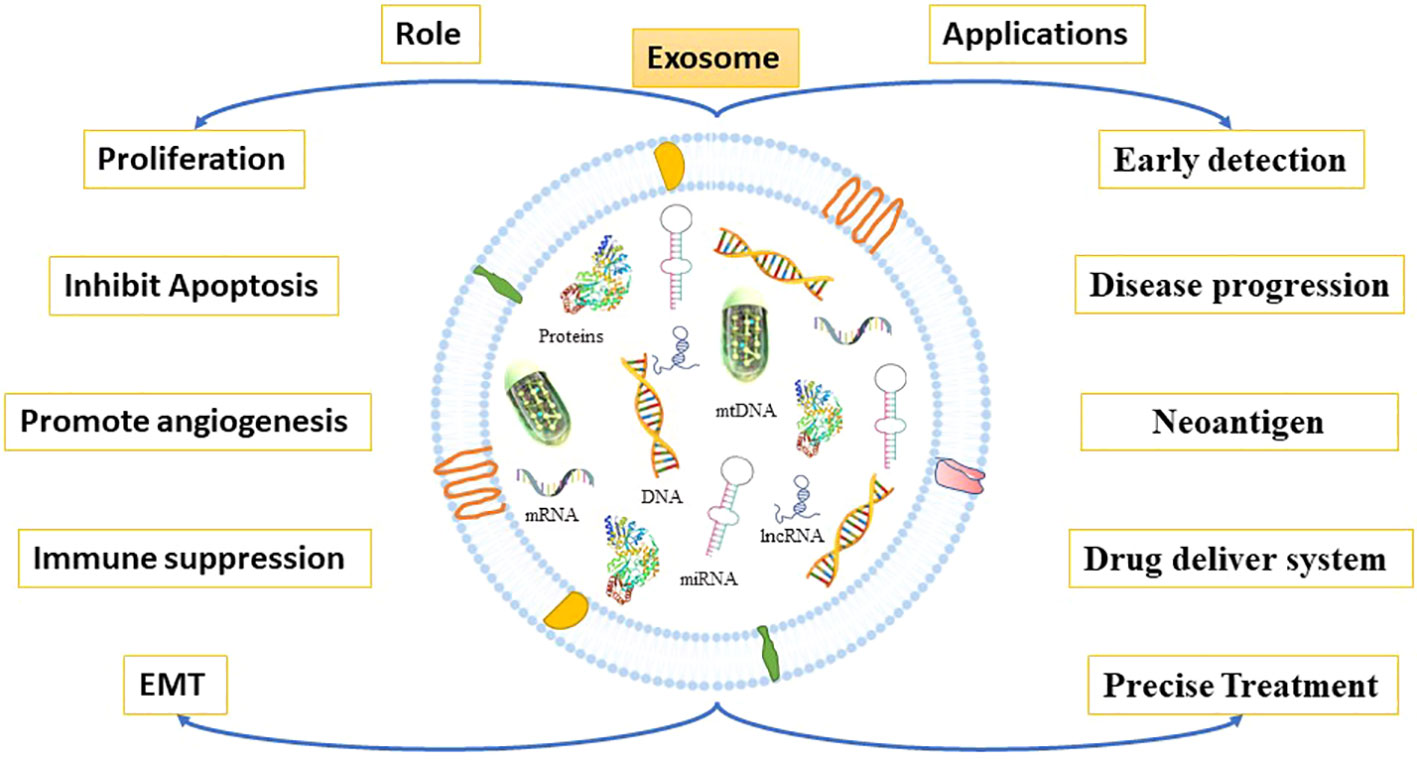
Figure 5 Roles and application of exosomes. Tumor-derived exosomes promote cancer growth and metastasis. Through multiple mechanisms, they participate in cancer growth and metastasis by reshaping TME resulting into EMT, cell proliferation, apoptosis inhibition, immunosuppression, and angiogenesis. Exosomes derived from cancer cells are enriched with proteins, mRNA, miRNA, lncRNA, DNA etc. that are more abundant in cancer cells than in normal cells. Thus, exosomes may be used as biomarkers for cancer diagnosis, prediction, and treatment.
Exosomal miRNAs have been significantly concerned in several exosome-mediated biological functions in cell-cell communication in numerous cancers including CRC (104, 105). MiR-21-5p and miR-155-5p have been revealed to be highly expressed in macrophage-derived M2 exosomes, which facilitated the migration and invasion of CRC (106, 107). In addition to this, it also observed that exosomes from bone marrow-derived mesenchymal stem cells (BMSCs) can inspire stem cell-like features of colorectal cancer through miR-142-3p (108). In addition, CAFs, TAM, and MSC exosome proteins are also significant mediators of cancer and TME regulation. Gang, N, and his team used proteomic analysis of CAFs and serum-derived exosomes that have recognized QSOX1 as a biomarker for the early prediction and detection of CRC non-invasively (109). Current research also described novel types of RNAs, such as Piwi-interacting RNA (piRNA) and tRNA-derived small RNA (tsRNA), along with miRNA, lncRNA, and cicrRNA (110, 111). There has only been limited research into the current existence and role of these types of non-coding RNAs in CRC exosomes. Thus, even though the therapeutic approach of exosomes has revealed countless application scenarios in colorectal cancers, many problems remain before we can routinely use exosomes in the clinical treatment of CRC.
In most solid tumors, CAFs are the significant cellular components of the TME (112). CAF-derived exosomes could stimulate neoplastic angiogenesis and cancer cell growth in CRC. Furthermore, these can also activate cancer cell dedifferentiation through the Wnt signaling pathway, therefore increasing the chemical resistance of CRC (95, 113). Compared to RNA and protein, there is little research on exosomal DNA. In previous research, it was observed that the gDNA from exosomes is widely used in liquid biopsy, and it has a great impact on tumor immunity and metabolism (114–118). The KRAS and BRAF mutation was identified in serum exosomes of patients with CRC with greater sensitivity and specificity (119, 120). Furthermore, it was also revealed that exosome gDNA plays a significant role in immunity in CRC patients (117). Current research studies have revealed that the number of exosomes in the body fluid of CRC patients is markedly higher compared to healthy controls (98). Hence, the studies on CRC exosomes must be encouraged due to this increased presence of CRC exosomes that can likely be used during cancer treatment.
Another promising biomarker that has established noteworthy consideration in the current era is circulating tumor DNA (ctDNA). ctDNA comprises DNA fragments that are released by fragmenting tumor cells into the blood circulation and in principle should have genetic and epigenetic alterations identical to the cancer cells they initiated from (121, 122). Numerous types of DNA modifications have been noticed with adaptable frequency in the ctDNA of patients with CRC. The revealing of mutant DNA in plasma or serum from a CRC patient has been associated with diagnosis, prognosis, and therapeutic response in numerous reports (123). Furthermore, in CRC patients KRAS mutations in ctDNA have been identified in different stages, with the highest level found in the more advanced stage (124–126). Furthermore, recent studies found that ctDNA was detected postoperatively in approximately 5% to 30% of patients with stage II to III colon cancer and has established a strong prognostic capacity in numerous observational studies (127). Since the last decade, in CRC, the introduction of next-generation sequencing (NGS) technology has led to the discovery of ctDNA in plasma, which is an encouraging practice (128, 128). Some research reports revealed that ctDNA methylation has a notable sensitivity compared to traditional serum tumor markers in patients with initial-stage CRC and is a significant biomarker for the diagnosis of CRC (129–131). Currently, personalized immunotherapy based on neoantigens requires tissue samples to obtain accurate evidence of somatic genomic modifications in individual cancer patients. Although it is from time to time problematic to obtain many tumor tissues; consequently, the development of ctDNA analysis could be significant in the enlargement of neoantigen-based treatment, even though it is still puzzling. Together, current potential clinical trials with ctDNA focus on the diagnosis, surveillance, and prognosis of CRC. With the rapid progress of research technology, liquid biopsies will play a crucial role in the diagnosis and treatment of CRC. In Tables 2, 3 (132–179), we have listed circulating miRNAs, lncRNAs, circ-RNAs and proteins as diagnostic biomarkers in CRC patients.
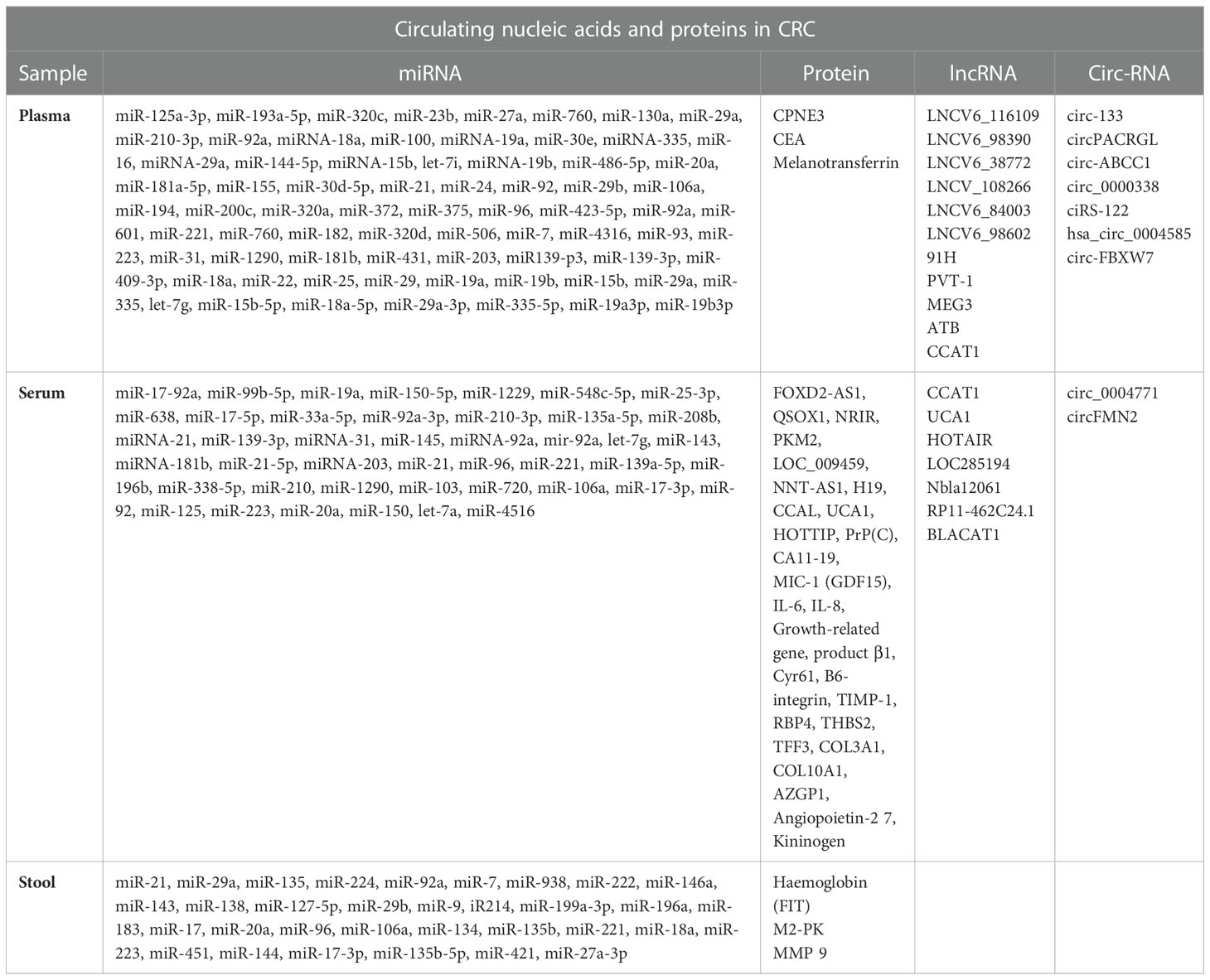
Table 2 Non-invasive biomarkers (miRNA, Proteins, lnc RNA and circ-RNA) used for CRC detection till date.
Mitochondrial DNA: Unexplored arena
In the recent era, the standard for the molecular profile of colorectal cancer (CRC) is tissue biopsy. However, they are inadequate concerning about sampling rate, illustration of tumor heterogeneity, and sampling can expose patients to antagonistic side effects. To study cell-free DNA (cfDNA) from the various body fluids, this being a component of a liquid biopsy, is relatively invasive, but highly significant to discover all tumor-specific mutations. Furthermore, mitochondria have their circular genome and therefore contribute to the total cfDNA content in the blood. MtDNA plays an essential role in mitochondrial biogenesis and regulates mitochondrial function and the regulation of apoptosis (180–182). A single cell comprises up to several thousand copies of mitochondrial DNA (mtDNA) contrasting to two copies of nuclear DNA (nDNA). Therefore, investigating hypothetically cell-free mitochondrial DNA (cf-mtDNA) could give an advanced level of understanding, rather than the examination of cell-free nuclear DNA (cf-nDNA). Furthermore, it was reported that mtDNA has a high mutation frequency and in CRC and other cancers fundamental molecular modifications (183, 184). Based on reported research literature, assessment of cf-mtDNA as a significant biomarker is stimulating for liquid biopsies and as a neoantigen due to high copy number might enable discovery of even minor quantities of ctDNA and their molecular modifications. Besides, earlier research has exposed that cf-mtDNA content and fragmentation design distinguish between cancer patients and healthy individuals, therefore also potentially serving as an indicative marker of disease (185–188). Though cf-mtDNA has been not completely categorized yet and an efficient method for comprehensive examination is still missing.
Rigorous research has been done to understand the hereditary risk issues of CRC. Thus far, over 40 nuclear genome alternatives significantly related to CRC risk have been recognized, counting SNP rs10911251, rs1321311, rs1035209, and so on (189, 190). But such loci account for only about 8%–16% of CRC cases, signifying that additional genetic risk factors of CRC still possibly need to be discovered.
Remarkably, numerous somatic mtDNA mutations and copy number alterations have also been commonly recognized in a wide variety of malignancies, including CRC (191). In CRC it was observed that mtDNA copy number is increased during the cancer process. Previous studies by Guo et al. have described that the reduction of mtDNA made by the mitochondrial transcription factor A (TFAM) mutation plays a potential role in cancer progression and resistance to cisplatin in MSI CRC (192). In addition to this, a report from China investigated 104 colorectal cancer patients and found that the percentage of mtDNA deletion of 4977 bp of mtDNA in CRC tissues was significantly reduced (193). Furthermore, recent research revealed that mitochondrial cfDNA had a surprisingly higher plasma copy number in healthy subjects than in CRC patients (188). Though, today the possible contribution of germline mtDNA differences in CRC expansion is a smaller amount of knowledge available including liquid biopsy. Together, we are confident that liquid biopsy is likely to be a substitute standard approach for monitoring the advanced development of genomic changes during cancer progression. Liquid biopsy has revealed remarkable effectiveness in a variety of applications and will contribute to personalized oncology.
Organoids
Tumor organoids were reviewed by Tatullo et al. with 77 references (194). To date, scientific cancer research has been conducted in in vitro experiments, performed on tissue culture plates and two-dimensional (2D) samples. In this framework, the development of colonies and spheroids has been determined as morphological indicators of cancer and stemness of cancer cells (195). In the current research scenario, 3D cultures systems have significantly enhanced in-vitro tumor models based on new biological mediums that mimic the extracellular environments. Organoids have been described more extensively in many reports in the scientific research literature. The overview of patient-derived organoids (PDO) has allowed for more representative cancer modeling, highlighting their excessive significance in biomedical applications, translational medicine, and personalized therapy approaches (196, 197). Furthermore, patient-derived organoids have certain advantages such as stable morphology, gene expression, and cell signaling, heterogeneity with cancer cells in the tumor, significant drug screening, low cost, and being easily generated “in a dish” (198). The application of the organoid culture method to liquid biopsy is a promising approach that combines the advantages of organoid cultures with the boundless potential of the liquid biopsy component for precision oncology.
Sato and their team first developed an organoid model from mice in the CRC research field and later they also developed an organoid culture protocol that is acceptable and also suitable for colon epithelial cell culture (199). In CRC, PDO developed from metastases taken by serial biopsies at various time points, and various counties of severely pretreated CRC patients were taken as preclinical models in clinical trials studies (200, 201). Those organoids were further treated with anti-cancer drugs, and the outcomes were associated with patients’ responses in clinical trial studies. The outcome suggested the ability of PDO to mimic TME in vivo, notable molecular and functional levels, and the most important aspect being to predict patient treatment response (202). Clinically active KRAS signaling suppressors and various drug groupings were observed against non-cancerous colon and CRC organoids (203). In recent research Zhao et al. used the organoid culture approach to identify the metabolic phenotype in cancer stem cells and differentiated cancer cells in CRC (204). To date, only one study has been done on organoids derived from CTCs and it revealed that CTC-derived organoids were more sensitive than Xenograft-derived organoids, to drugs affecting the Survivin pathway, which significantly decreased the levels of Survivin and X-linked inhibitor of apoptosis protein (XIAP), that induce CTC derived organoid death. Based on this first study, future use of the organoid approach to CTCs may open new viewpoints by providing extraordinary visions of the cancer growth and metastatic process, by allowing the discovery of novel CTC markers, beneficial treatment targets, and chemoresistance mechanisms (205).
Notwithstanding organoid significant advantages, patient-derived organoid (PDO) also possesses certain limitations such as abnormalities, noise during drug screening, development and standardization of organoid culture, and lack of major TME components (206–208). Based on the published literature, PDO is a fascinating in vitro model for the development of preclinical drugs in CRC, because of its ability to mimic human physiopathology. Taken together, the potential of the organoid approach for basic and clinical studies of CRC is greater than the treatment of patients with CRC in the new time of personalized medicine. Furthermore, it will open a new door for the liquid biopsy approach using CTCs and/or CTSCs to generate organoid models.
Liquid biopsies and immunotherapy
In the research of CRC treatment, diagnostic and chemotherapy have developed curiously in the last two eras. Still, it is problematic to find minimal residual disease (MRD) essential for primary detection of recurrence of tumors and give suitable drugs timely prior cancer becomes multi-drug-resistant and more aggressive. However, the most thrilling example of change in cancer therapy in the current era has been immunotherapy. Subsequently, with its early approval for the treatment of melanoma, it has become the standard of care for various other tumors. Immunotherapy has also established promising abilities and good tolerance in gastrointestinal (GI)-related cancers (209). All the research conferred so far in CRC are focused either on the association between ctDNA existence and tumor burden or the recognition of molecular modifications that predict response or resistance to targeted agents. The burden of tumor mutations is currently being argued in CRC and various solid tumors were given its association with response to immunotherapy and the current approval of the Food and Drug Administration (FDA) as an agnostic biomarker to access cancer immunotherapy with pembrolizumab or dostarlimab (210, 211). On the other hand, MSI is currently the most applicable potential biomarker for immunotherapy sensitivity in CRC, characteristically measured in solid tissue samples (212). Additional growing manipulation of liquid biopsy in CRC is the examination of methylation biomarkers, which is rapidly developing as an influential approach to early diagnosis and prognosis (213).
MSI colorectal cancer
Microsatellite instability (MSI), also known as a hypermutable phenotype, occurs because of a defective mismatch repair system (dMMR) in approximately 15% of colorectal cancer patients (CRC) (214–216). MSI CRC is most often associated with the proximal colon, increased immunogenicity, and a good prognosis, in contrast to CRC of chromosomal instability (CIN) (also known as stable/low-level microsatellite stable/MSI-low-level [MSS/MSI-L]) CRC which is more commonly found in the distal colon with increased immune tolerance and a poor prognosis (215, 217). Many studies have shown the advantages in detecting MSI status, including prognosis and specific treatment benefits associated with this molecular subtype, with increased survival rates of up to 15% in CRC patients (218, 219). A few studies thus far have illustrated MSI to be a rare occurrence in rectal cancer, and linked to a poorer prognosis with a higher risk of dying (220–222). Better results are observed in locally advanced (stage II/III) MSI CRC compared to CIN CRC, with the recently added benefit of oFDA-approved immunotherapy (i.e. pembrolizumab, nivolumab, and combination nivolumab/ipilimumab) in the treatment of unresectable or metastatic resistant MSI CRC in conventional regimens (223–226). To date, the conventional treatment regimen for rectal cancer continues to be resection surgery, chemoradiation (preoperative), and chemotherapy, with the intolerant response that do not have alternative approved treatment strategies available (227, 228). MSI CRC is known to have a poor response to 5-fluorouracil (5-FU), which is a fluoropyrimidine drug used in the conventional adjuvant treatment regimen of CRC (229). Adverse effects include nausea, diarrhoea, mucositis, neuropathy, neutropenia and more serious complications leading to death have been reported in 1% of patients. Therefore, it is imperative to implement a reliable diagnostic methodology for accurate diagnosis of MSI. Mononucleotide markers have been well described as the most reliable markers for MSI panels, without the need for di-nucleotide markers and matched normal tissue testing (230, 231). Ethnic polymorphisms have also been described in certain markers (eg. African polymorphisms in BAT25 and BAT26) and should therefore be considered when deciding on the implementation of diagnostic markers panel in certain geographical settings (231–233). If instability is required in 30% of markers used in the panel for a diagnosis of MSI, it is important to establish that the markers included are nonpolymorphic in the general population. Additional testing to confirm MSI status is to assess the expression profiles of mismatch repair (MMR) proteins through immunohistochemistry (IHC) (234–236). This is a more cost-effective approach and in addition provides information on the deficient dMMR protein, gaining insight into the possible mechanism of the disease, whether likely sporadic (associated with MLH1 protein loss through MLH1 promoter methylation, and BRAFV600E pathogenic variants) or due to hereditary Lynch syndrome pathogenic variants (MSH2, MSH6, MLH1, or PMS2) (237–239).
MSI CRC is known to have a better response to immunotherapy, and this is due to the active innate inflammatory tumor microenvironment, as a response to the hypermutated phenotype of these tumors (240). The TCGA study revealed that hypermethylated and hypermutated cancers were more commonly associated with the proximal colon and distinct at the genomic level compared to distal colon and rectum cancer (217). This could potentially be due to the difference in the originator cells of the right colon (developed from the midgut) compared to the distal colon (originated from the hindgut) (241, 242). To date, few clinical trials have also begun exploring combination radioimmune therapy, with promising toxicity reports indicating hope for patients with rectal cancer (243). Another remarkable study of a PD-1 blockade (dostarlimab) in the treatment of MSI rectal cancer indicated high sensitivity and 100% complete response rates with no severe adverse events (244). This illustrates the need for more clinical trials in immunotherapy and neoadjuvant therapy with a focus on rectal cancer to be conducted, to provide more effective predictive therapy for the better management and increased survival of these patients. Besides this, the neoantigens currently appear in MSI-H CRC, which is related to a higher tumor mutation burden, so it has potential as neoantigens in the immunotherapeutic strategy for the treatment of various types of CRC. But a liquid biopsy-based examination to assess MSI can successfully assess an extensive subclass of CRC patients, including those with inadequate tissue samples or when protection concerns about invasive surgery arise.
MSS colorectal cancer
Tumors in the distal colon display lower mutational burdens and are less immunologically active, with little to no CD8+ T lymphocyte localization or infiltration. This type is generally referred to as a “cold-tumor” (245). Cold-tumors represent the majority of CRC and mostly do not benefit from immune checkpoint inhibitor (ICI) therapy. Improvement in immune therapeutic strategies includes transitioning “cold-” into immune infiltrated “hot-tumors”, and once infiltrated, ensuring an effective inhibitory response on tumor cell activity is attained (245). This is achieved by controlling tumor immunogenicity and the TME by directing the immune system in targeting tumor cells specifically (246). ICIs are designed to inhibit certain receptors such as Programmed-death-1 (PD-1) on T-cells that are controlled by cancer cells to evade immune attack. Monoclonal antibody (mAb) treatment, chimeric antigen receptor (CAR)-T cell therapy, and ICIs are key immunotherapies currently being used against many cancers (247). mAb therapy against the receptor Programmed death ligand-1 (PD-L1) on cancer cells, to block its communication with PD-1 and increase T cell immune response has shown effective in many solid tumors. Adoptive cell transfer (ACT), such as chimeric antigen receptor (CAR) T-cell therapy involving the patient’s own T-cells has also gained increased recognition (248). These cells are genetically engineered to include the new CAR, and then re-administered to the patient (247) The CAR increases-affinity and binding of T-cells to target antigens, without the need of the major histocompatibility complex (MHC) receptor. CAR-T therapy has had fewer success rates in solid tumors, mainly due to a suppressive TME (increased cytokine and dense stromal network) (248). Enzymes targeting and degrading stromal matrices (eg. heparanase) have been employed to overcome this hurdle and increase infiltration of CAR T-cells in solid tumors (249, 250). Cancer vaccines have also been introduced as novel immunotherapeutic approaches to target antigens uniquely expressed on tumor cells, thus inducing an anti-tumor immune response in patients (251). In addition, oncolytic viruses destroying cancer cells but non-virulent to normal cells is another immunotherapy strategy (247). Certain virus, have natural tropism to infect certain cells, for example, hepatitis B virus for hepatocytes and parvovirus B19 for human erythroid progenitor cells, and this mechanism has been used to direct virus-mediated cytotoxicity in tumor cells (252). To address effective immunotherapeutic strategies in MSS CRC in future, combination therapy involving two or more approaches would need to be implemented, involving chemotherapy, radiotherapy, mAb, ICI targeted therapy, stromal matrix degradation, oncolytic viral therapy, CAR-T therapy and cancer vaccines (247).
Neoantigen: An emerging concept
Neoantigens have potential high specificity and targeted but are mainly patient-specific and, consequently, are difficult to classify for utility and are mostly remarkable procedures in a cancer patient population. Currently, immunotherapy, inclusive of immune checkpoint inhibitors (ICIs), tumor-specific vaccines, and tumor-infiltrating lymphocytes (TILs) based on neoantigens, has a progressively significant role in cancer treatment (253). The conventional significant cDNA library screening method is labor-intensive, low-throughput, and unable of classifying some altered antigens consequent from GC-rich transcripts and low-expression transcripts (254). However, current scientific developments in next-generation sequencing and a notable improvement in bioinformatics analysis have provided a robust groundwork on which to build these significant efforts. A peptide-based identification method connecting whole-exome sequencing (WES) and MHC-peptide binding prediction algorithms has been effective in identifying neoantigens recognized by TILs in patients with melanoma (255). Neuropeptides are expressed in tumor cells, while healthy cells will not present such antigens. Earlier research on CRC genomics mostly focused on the mechanism of tumor development and progression, with a lower inclusion of neoantigens and neoantigen-based immunotherapy (256). In research, it was observed that certain CRC patients with high microsatellite instability (MSI-H) might benefit from ICI treatment due to the presence of high neoantigens (256). However, not all patients with MSI-H CRC show medical efficacy in ICI treatment. Neoantigen-based immunotherapy is synchronizing with ICI since it does not need a detailed analysis of the patient’s MSI status or tumor mutation burden (TMB) (257). The tumor-specific landscape of neoantigens makes them significant perfect targets for antitumor immunotherapy and has been investigated for the treatment of CRC in a variety of basic and clinical immunotherapy studies. The average TMB of CRC was classified seventh among 30 of the most common categories of tumors. A previous study by Aleksandrov L. B observed that approximately 16% of CRCs have a TMB of >12 mutations per 106 base pairs, which are identified as extremely mutated tumors (258). Patients with higher TMB might have more potent neoantigens that can be used for the clinical approach in CRC. For MSI-H CRC, frameshift mutations generally instigated by INDELs can lead to the creation of novel frameshift peptides (FSP), which are the key cause of neoantigens in CRC. Frameshift mutations can be frequently initiated in DNA segments or genes with a significant biological role in maximum MSI-H CRC. These genes play a vital role in epigenetic regulation, DNA repair, signal transduction, cell apoptosis, and miRNA processing. Besides frameshift mutation currently, it has been described that single-nucleotide variants (SNVs) in genes like KRAS, PIK3CA, PCBP1, and CHEK2, are related to the creation of the 10 most frequent neoantigens. In Table 4, we have listed the mutated antigens that were studied in CRC tissue (259).
One main hurdle for personalized neoantigen-based immunotherapy is the availability of tumor biopsies. To date, neoantigens are usually recognized from genomic profiling of various tumor biopsies (260). Although this predictable approach is time-consuming, invasive, with a low positivity rate, and in the most challenging case where repeated sampling is mandatory or there is an inadequate sample, it is more common with frequent and metastatic cancers. Specifically, at the top immune checkpoint, significant inhibitors can be more effective in the presence of natural neoantigens (261, 262). Based on the current scenario, liquid biopsies can be a good replacement for determining potential neoantigens as budding targets for immunotherapy in numerous cancers. Although there is a certain restriction in the detection of genomic mutations with very low allele occurrence in the plasma sample, the dependability of genetic information has been described concerning the use of liquid biopsy (263). Thus, based on current research on liquid biopsies, valuable visions could be served for making treatment choices using neoantigen.
Immune cells
The TME generates a potential protective shell in which cancer cells easily and rapidly gather gene alterations and immune escape. Generally, this process occurs in the early stage of cancer, the immune response created by immune cells in the TME has antitumoral properties (264). Collectively, evidence has revealed that TME contains NK cells, CD8+ cytotoxic T cells, M1 macrophages, T helper-1 cells, and antigen-presenting cells (APCs) which act as tumor foes and suppress tumor development. Neutrophils, tumor-associated macrophages (TAMs), CD4+ T helper-2 cells, and regulatory T cells (Tregs) are crucial components for reducing the immune suppression environment, inhibiting cancer cell survival and progression, in addition to helping to avoid immune devastation (265) (Figure 6). In metastatic CRC, it has been confirmed that tumor behavior with the lowest tumoricidal immune infiltrates shows a higher risk of tumor replacement (266). CD8+ T and CD4+ T cells are the utmost powerful cytolytic cell subcategory. Cytotoxic processes are supported by some constituents shaped by CD8+ T cells, such as granzymes, perforin, Fas ligand (FasL), and TNF-α (267). Recent research established that patients with promising CRC regularly have tumor immune cell infiltrates with higher cytolytic events (268). But, the percentage of cytotoxic T cells number decreases as TNM-stage increases in CRC (269).
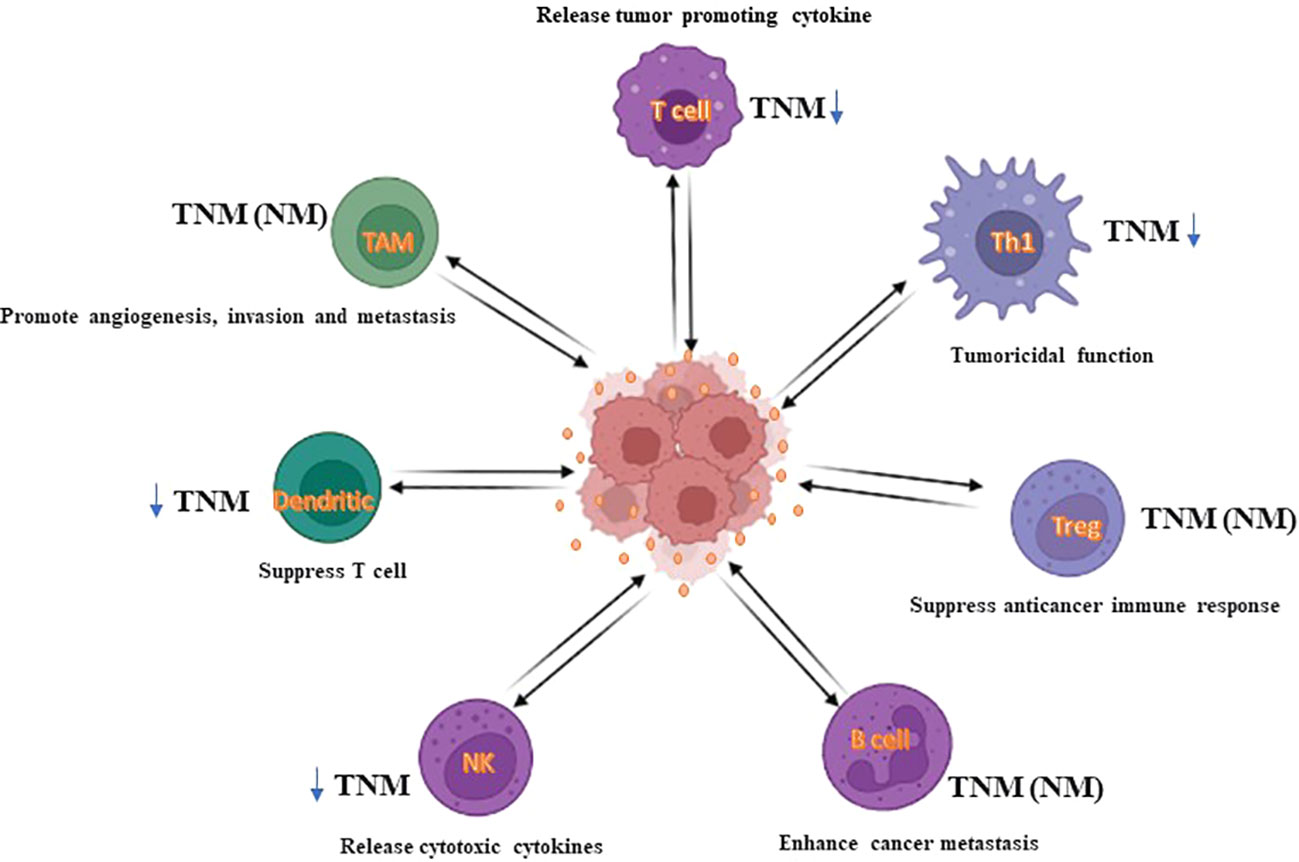
Figure 6 The impact of immune infiltrates on CRC. In CRC, immune infiltrates can impact CRC cell death, either directly or via tumoricidal T cells (TCT), and consequently affect tumor progression. For example, cytotoxic T cells, macrophages, and NK cells can exert a cytolytic effect on CRC cells. For other populations of cells, such as Treg, B cells, dendritic cells, or M2-like macrophages, generally impact CRC cell death by mediating the tumoricidal activity of TCT cells. Herein, Treg, regulatory B cells, immature dendritic cells, and macrophages enable TCT cells to be exhausted, thus causing substantial progression in CRC tumors. Accordingly, immunoscore system using immune cells could deliver insights into a novel liquid biopsy approach as a diagnostic tool.
In humans, Treg cells are the main source of IL10. IL10 has numerous effects on immune cells, including decreasing the cytotoxic activity of CD8+ T cells, down-regulating MHC-II-restricted antigens, preventing the synthesis of IFN-γ or TNF-α, and hindering the effector roles of dendritic cells and other CD4+ T cell subsets (Th1, Th2, or Th17 cells) (270, 271). Based on the results of numerous reviews, there is still support that tumor infiltration of Treg cells possibly extends the survival of CRC patients (272). In concept, Treg cells are susceptible to apoptosis in CRC tumors that could negatively regulate the expression of IFN-γ, TNF-α, and IL-2 by tumoricidal T cells (273). Collectively this suggested that the concentration of Treg cells along with their connected cytokine profiles in cancer should be determined together in a liquid biopsy-based approach to increase the use of Treg cells in predicting CRC prognosis.
B cells consist of diverse subcategories and govern antibody production, antigen appearance, and immunosuppression (274). A currently published study on colon cancer has reported that a high concentration of tumor B cells may provide for promising clinical outcomes only in patients with right-sided colon cancer (275). Furthermore, the higher expression of CXCL9 and CXCL10 in CRC tumors can also attract regulatory B cells (Breg), although such chemoattractants are also effective in employing tumoricidal T cell functions (276). Assessing the concentrations of tumoricidal T cells, Treg cells, and B cells together could significantly improve the prediction of the prognosis of CRC. In addition to this component, natural killer cells (NK) also play a cytolytic role in TME. In CRC, it was found that alteration of MHC-I functions, resulting in NK cells, will reduce its development and decrease the production of IFN-γ, GZMB, and perforin production (277). Surprisingly, in CRC metastasis, it was observed that the number of tumor-formed NKT cells was markedly decreased compared to normal tissue (278). However, it is at minimum knowledge that NK cell infiltration into CRC at progressive disease phases is challenging. In TME, one of the most significant components of dendritic cells (DCs) is specialized antigen-presenting cells in the human body. Previous data suggested that in CRC tumor infiltration of DCs is negatively related to tumor phases because this growth of DC cells with various phenotypes will result in a poor prognosis of CRC (279). Fundamentally, it is indicated that mature or immature DC could have various effects on CRC development. Lastly, the major component of Tumor-associated macrophages (TAMs) are dangerous immune infiltrates in cancer phenotype. In CRC, numerous studies have shown that a high number of CD68+ macrophages in tumor IM expect a promising prognosis (280, 281). Furthermore, Itatani et al. observed that by improving the production of metalloproteinase-9, CCR1+ macrophages support the invasion of CRC (282). Similarly, to CCL2 and CCL15, CCL5 helps as another significant chemokine that controls the development of CRC (283). Besides, in CCL5-deficient mice, xenografted CRC tumors show a high amount of tumoral CD8+ T cells, signifying that CCL5 at minimum influences T cell infiltration (284).
The Immunoscore system delivers insights into a novel approach for consistently predicting CRC diagnosis, particularly since this tool has the potential to screen immunotherapy components. On the other hand, Immunoscore combined with diagnostic tools such as liquid biopsy, and a neoantigen-based approach provides for better CRC treatment, especially for immunotherapy.
Future perspective and conclusion
The prognosis of individuals with CRC has substantially improved in the current era due to the significant improvement and expansion in diagnostic and therapeutic approaches. However, early prediction, diagnosis, and treatment monitoring of CRC have various lacunae; due to this, many patients die each year. In recent years, the field of liquid biopsy has grown rapidly because it is noninvasive, overcomes tumor heterogeneity, and can allow real-time intensive care of tumor development, recurrence, or therapeutic response (285). This is the reason that recently there are numerous ongoing clinical trials from the US National Laboratory of Medicine (NIH) on liquid biopsy-based approaches to detect CRC. Presently, numerous efforts have been made utilizing CTCs, CAFs, exosomes, immune cells, neoantigens, mtDNA, and ctDNA isolation and characterization-based approaches to detect and treat CRC; and which have shown to be highly sensitive and effective. In addition, genes and proteins expressed by these components can also be used for early CRC detection and therapy. However, a CTC end point value for the clinical evaluation of CRC patients’ progression and prognosis is still not adequately developed owing to sampling issues, storage conditions and timing of biopsy; and most importantly enrichment procedures (286, 287). Therefore, it is important to develop a CTC capturing platform that is more precise and effective. Additionally, recent studies on CTCs/cCAFs clusters open a new path for developing an additional personalized and detailed treatment plan for each cancer patient. But there are still several lacunae on the biology of CTCs clusters, and specifically on the heterotypic CTCs-CAFs clusters, that need to be investigated to recognize the mechanism of cellular aggregates and their role in metastasis. Furthermore, it is also important to see which of the CAF-derived signals improve CTC survival and cancer cell growth, besides to govern the efficient alterations between homotypic CTCs clusters and heterotypic CTCs-CAFs clusters. Another significant component of liquid biopsy is the exosome, that has a potential role in tumor initiation, development and metastasis, including EMT, tumor angiogenesis, extracellular matrix remodeling, organ-specific metastasis, and immune evasion. The advantage of exosomes is that they are easier to isolate than CTCs and cfDNA in tumors; a current era is improved and more research will be focused on exosomes in the diagnosis of cancers at an early stage in the future. But there is still uncertainty in clinical approaches due to low effectiveness and informal phagocytosis by the immune system. So, based on evidence, indepth research should be undertaken to solve this hindrance and develop precise clinical applications of exosomes. Furthermore, analysis of ctDNA is a most promising component of liquid biopsy that can play a critical role in numerous characteristics in the clinical management of patients with CRC (288). Furthermore, TMB in ctDNA and immune check point proteins in CTCs show significant roles in tumor immunotherapy. However, due to inadequate and partial knowledge of molecular mechanisms, ctDNA as liquid biopsy has not yet been applied in immune-oncology in the clinic; however, promising available data and advanced noteworthy technologies and methods recommend that this approach certainly has a plausible role in CRC patient therapy. Based on our review, we found that a higher copy number of mtDNA significantly promotes cell proliferation, apoptosis resistance, and CRC metastasis, thus also providing a novel indication for this process as a drug target and prediction of neoantigens in CRC treatment (188). Existing genomic research has revealed that there are many hotspot mutations in significant driver genes; and the neoantigen epitopes made by these mutations are vital “public” immunotherapy targets as a liquid biopsy approach. More recently, liquid biopsy-based neoantigens are a new immunotherapeutic approach for the treatment of various types of CRC. Though, there are still numerous challenges such as tissue biopsy and identification, which still require further research as explored form of liquid biopsy (Table 5). The worldwide replacement of tumor biopsies with liquid biopsies appears idealistic; however, with a range of approaches using CTCs, CAFs, ctDNA, exosomes, mtDNA and neoantigen, it seems highly likely that useful tools will be developed for CRC with applications in early detection, postoperative monitoring, treatment response and therapeutic resistance. In summary, liquid biopsy is an important part of precision medicine and is held to be a clinical reality soon.
Author contributions
SM and CP conceptualized and designed the manuscript. SM, KB and MM contributed in literature review and drafted manuscript. MJM created all figures. SM, KB, RS and CP contributed to critical review and finalized the manuscript. All authors contributed to manuscript and approved the submission.
Acknowledgments
Wits/MRC Common Epithelial Cancer Research Centre (CECRC), South Africa.
Conflict of interest
The authors declare that the research was conducted in the absence of any commercial or financial relationships that could be construed as a potential conflict of interest.
Publisher’s note
All claims expressed in this article are solely those of the authors and do not necessarily represent those of their affiliated organizations, or those of the publisher, the editors and the reviewers. Any product that may be evaluated in this article, or claim that may be made by its manufacturer, is not guaranteed or endorsed by the publisher.
References
1. Sawicki T, Ruszkowska M, Danielewicz A, Niedźwiedzka E, Arłukowicz T, rzybyłowicz KE. A review of colorectal cancer in terms of epidemiology, risk factors, development, symptoms and diagnosis. Cancers (Basel) (2021) 13(9):2025. doi: 10.3390/cancers13092025
2. Sung H, Ferlay J, Siegel RL, Laversanne M, Soerjomataram I, Jemal A, et al. Global cancer statistics 2020: GLOBOCAN estimates of incidence and mortality worldwide for 36 cancers in 185 countries. CA Cancer J Clin (2021) 71(3):209–49. doi: 10.3322/caac.21660
3. Siegel RL, Miller KD, Fuchs HE, Jemal A. Cancer statistics, 2021. CA Cancer J Clin (2021) 71:7–33. doi: 10.3322/caac.21654
4. Douaiher J, Ravipati A, Grams B, Chowdhury S, Alatise O, Are C. Colorectal cancer-global burden, trends, and geographical variations. J Surg Oncol (2017) 115(5):619–30. doi: 10.1002/jso.24578
5. Xi Y, Xu P. Global colorectal cancer burden in 2020 and projections to 2040. Transl Oncol (2021) 14(10):101174. doi: 10.1016/j.tranon.2021.101174
6. Dekker E, Tanis PJ, Vleugels JLA, Kasi PM, Wallace MB. Colorectal cancer. Lancet (2019) 394:1467–80. doi: 10.1016/S0140-6736(19)32319-0
7. Guren MG. The global challenge of colorectal cancer. Lancet Gastroenterol Hepatol (2019) 4:894–5. doi: 10.1016/S2468-1253(19)30329-2
8. Baxter N, Rabeneck L. New findings about the risks and limitations of colonoscopy used in the early detection of colorectal cancer. Healthc Q (2009) 12(2):24–5. doi: 10.12927/hcq.2009.20675
9. Mazouji O, Ouhajjou A, Incitti R, Mansour H. Updates on clinical use of liquid biopsy in colorectal cancer screening, diagnosis, follow-up, and treatment guidance. Front Cell Dev Biol (2021) 9:660924. doi: 10.3389/fcell.2021.660924
10. Fernández-Lázaro D, García Hernández JL, García AC, Córdova Martínez A, Mielgo-Ayuso J, Cruz-Hernández JJ. Liquid biopsy as novel tool in precision medicine: Origins, properties, identification and clinical perspective of cancer's biomarkers. Diagn (Basel) (2020) 10(4):215. doi: 10.3390/diagnostics10040215
11. Raza A, Khan AQ, Inchakalody VP, Mestiri S, Yoosuf ZSKM, Bedhiafi T, et al. Dynamic liquid biopsy components as predictive and prognostic biomarkers in colorectal cancer. J Exp Clin Cancer Res (2022) 41(1):99. doi: 10.1186/s13046-022-02318-0
12. Michela B. Liquid biopsy: A family of possible diagnostic tools. Diagn (Basel) (2021) 11(8):1391. doi: 10.3390/diagnostics11081391
13. Peng M, Chen C, Hulbert A, Brock MV, Yu F. Non-blood circulating tumor DNA detection in cancer. Oncotarget (2017) 8(40):69162–73. doi: 10.18632/oncotarget.19942
14. Wills B, Gorse E, Lee V. Role of liquid biopsies in colorectal cancer. Curr Probl Cancer (2018) 42(6):593–600. doi: 10.1016/j.currproblcancer.2018.08.004
15. Yamada T, Matsuda A, Koizumi M, Shinji S, Takahashi G, Iwai T, et al. Liquid biopsy for the management of patients with colorectal cancer. Digestion (2019) 99(1):39–45. doi: 10.1159/000494411
16. Werner B, Warton K, Ford CE. Transcending blood-opportunities for alternate liquid biopsies in oncology. Cancers (Basel) (2022) 14(5):1309. doi: 10.3390/cancers14051309
17. Siravegna G, Mussolin B, Venesio T, Marsoni S, Seoane J, Dive C, et al. How liquid biopsies can change clinical practice in oncology. Ann Oncol (2019) 30(10):1580–90. doi: 10.1093/annonc/mdz227
18. Vacante M, Ciuni R, Basile F, Biondi A. The liquid biopsy in the management of colorectal cancer: An overview. Biomedicines (2020) 8(9):308. doi: 10.3390/biomedicines8090308
19. Mauri G, Vitiello PP, Sogari A, Crisafulli G, Sartore-Bianchi A, Marsoni S, et al. Liquid biopsies to monitor and direct cancer treatment in colorectal cancer. Br J Cancer (2022) 127:394–407. doi: 10.1038/s41416-022-01769-8
20. Siravegna G, Marsoni S, Siena S, Bardelli A. Integrating liquid biopsies into the management of cancer. Nat Rev Clin Oncol (2017) 14:531–48. doi: 10.1038/nrclinonc.2017.14
21. Crisafulli G, Mussolin B, Cassingena A, Montone M, Bartolini A, Barault L, et al. Whole exome sequencing analysis of urine trans-renal tumour DNA in metastatic colorectal cancer patients. ESMO Open (2019) 4:e000572. doi: 10.1136/esmoopen-2019-000572
22. Jiang J, Gao J, Wang G, Lv J, Chen W, Ben J, et al. Case report: Vemurafenib treatment in brain metastases of BRAFS365L -mutant lung papillary cancer by genetic sequencing of cerebrospinal fluid circulating tumor DNA detection. Front Oncol (2021) 11:688200. doi: 10.3389/fonc.2021.688200
23. Ding Y, Li W, Wang K, Xu C, Hao M, Ding L. Perspectives of the application of liquid biopsy in colorectal cancer. BioMed Res Int (2020) 2020:6843180. doi: 10.1155/2020/6843180
24. Vymetalkova V, Cervena K, Bartu L, Vodicka P. Circulating cell-free DNA and colorectal cancer: A systematic review. Int J Mol Sci (2018) 19(11):3356. doi: 10.3390/ijms19113356
25. Eslami-S Z, Cortés-Hernández LE, Cayrefourcq L, Alix-Panabières C. The different facets of liquid biopsy: A kaleidoscopic view. Cold Spring Harb Perspect Med (2020) 10(6):a037333. doi: 10.1101/cshperspect.a037333
26. Jia S, Zhang R, Li Z, Li J. Clinical and biological significance of circulating tumor cells, circulating tumor DNA, and exosomes as biomarkers in colorectal cancer. Oncotarget (2017) 8(33):55632–45. doi: 10.18632/oncotarget.17184
27. Heitzer E, Ulz P, Geigl JB. Circulating tumor DNA as a liquid biopsy for cancer. Clin Chem (2015) 61(1):112–23. doi: 10.1373/clinchem.2014.222679
28. Kowalik A, Kowalewska M, Góźdź S. Current approaches for avoiding the limitations of circulating tumor cells detection methods-implications for diagnosis and treatment of patients with solid tumors. Transl Res (2017) 185:58–84.e15. doi: 10.1016/j.trsl.2017.04.002
29. Krog BL, Henry MD. Biomechanics of the circulating tumor cell microenvironment. Biomech Oncol (2018) 1092:209–33. doi: 10.1007/978-3-319-95294-9_11
30. Sistigu A, Musella M, Galassi C, Vitale I, De Maria R. Tuning cancer fate: Tumor microenvironment's role in cancer stem cell quiescence and reawakening. Front Immunol (2020) 11:2166. doi: 10.3389/fimmu.2020.02166
31. Quail DF, Joyce JA. Microenvironmental regulation of tumor progression and metastasis. Nat Med (2013) 19(11):1423–37. doi: 10.1038/nm.3394
32. Dzobo K. Taking a full snapshot of cancer biology: Deciphering the tumor microenvironment for effective cancer therapy in the oncology clinic. OMICS (2020) 24(4):175–9. doi: 10.1089/omi.2020.0019
33. Baranyi M, Lippai M, Szatmári Z. A stroma szerepe a tumorok kialakulásában és progressziójában [Role of the stroma in the initiation and progression of tumors]. Orv Hetil (2015) 156(45):1816–23. doi: 10.1556/650.2015.30294
34. Lyssiotis CA, Kimmelman AC. Metabolic interactions in the tumor microenvironment. Trends Cell Biol (2017) 27(11):863–75. doi: 10.1016/j.tcb.2017.06.003
35. Fridman WH, Pagès F, Sautès-Fridman C, Galon J. The immune contexture in human tumours: impact on clinical outcome. Nat Rev Cancer (2012) 12(4):298–306. doi: 10.1038/nrc3245
36. Taube JM, Klein A, Brahmer JR, Xu H, Pan X, Kim JH, et al. Association of PD-1, PD-1 ligands, and other features of the tumor immune microenvironment with response to anti-PD-1 therapy. Clin Cancer Res (2014) 20(19):5064–74. doi: 10.1158/1078-0432.CCR-13-3271
37. Andre F, Pusztai L. Molecular classification of breast cancer: implications for selection of adjuvant chemotherapy. Nat Clin Pract Oncol (2006) 3(11):621–32. doi: 10.1038/ncponc0636
38. Li X, Gao Y, Xu Z, Zhang Z, Zheng Y, Qi F. Identification of prognostic genes in adrenocortical carcinoma microenvironment based on bioinformatic methods. Cancer Med (2020) 9(3):1161–72. doi: 10.1002/cam4.2774
39. Liu Z, Zhu Y, Xu L, Zhang J, Xie H, Fu H, et al. Tumor stroma-infiltrating mast cells predict prognosis and adjuvant chemotherapeutic benefits in patients with muscle invasive bladder cancer. Oncoimmunology (2018) 7(9):e1474317. doi: 10.1080/2162402X.2018.1474317
40. Zhang S, Zhang E, Long J, Hu Z, Peng J, Liu L, et al. Immune infiltration in renal cell carcinoma. Cancer Sci (2019) 110(5):1564–72. doi: 10.1111/cas.13996
41. Leman JKH, Munoz-Erazo L, Kemp RA. The intestinal tumour microenvironment. Adv Exp Med Biol (2020) 1226:1–22. doi: 10.1007/978-3-030-36214-0_1
42. Galindo-Pumariño C, Collado M, Herrera M, Peña C. Tumor microenvironment in metastatic colorectal cancer: The arbitrator in patients' outcome. Cancers (Basel) (2021) 13(5):1130. doi: 10.3390/cancers13051130
43. Wozniakova M, Skarda J, Raska M. The role of tumor microenvironment and immune response in colorectal cancer development and prognosis. Pathol Oncol Res (2022) 28:1610502. doi: 10.3389/pore.2022.1610502
44. Vellinga TT, den Uil S, Rinkes IH, Marvin D, Ponsioen B, Alvarez-Varela A, et al. Collagen-rich stroma in aggressive colon tumors induces mesenchymal gene expression and tumor cell invasion. Oncogene (2016) 35(40):5263–71. doi: 10.1038/onc.2016.60
45. Calon A, Lonardo E, Berenguer-Llergo A, Espinet E, Hernando-Momblona X, Iglesias M, et al. Stromal gene expression defines poor-prognosis subtypes in colorectal cancer. Nat Genet (2015) 47(4):320–9. doi: 10.1038/ng.3225
46. Ferreira MM, Ramani VC, Jeffrey SS. Circulating tumor cell technologies. Mol Oncol (2016) 10(3):374–94. doi: 10.1016/j.molonc.2016.01.007
47. Alix-Panabières C, Pantel K. Circulating tumor cells: liquid biopsy of cancer. Clin Chem (2013) 59(1):110–8. doi: 10.1373/clinchem.2012.194258
48. Hu M, Wang Z, Wu Z, Ding P, Pei R, Wang Q, et al. Circulating tumor cells in colorectal cancer in the era of precision medicine. J Mol Med (Berl) (2022) 100(2):197–213. doi: 10.1007/s00109-021-02162-3
49. Tsai WS, You JF, Hung HY, Hsieh PS, Hsieh B, Lenz HJ, et al. Novel circulating tumor cell assay for detection of colorectal adenomas and cancer. Clin Transl Gastroenterol (2019) 10(10):e00088. doi: 10.14309/ctg.0000000000000088
50. Baek DH, Kim GH, Song GA, Han IS, Park EY, Kim HS, et al. Clinical potential of circulating tumor cells in colorectal cancer: A prospective study. Clin Transl Gastroenterol (2019) 10(7):e00055. doi: 10.14309/ctg.0000000000000055
51. Yang C, Zou K, Yuan Z, Guo T, Xiong B. Prognostic value of circulating tumor cells detected with the CellSearch system in patients with gastric cancer: evidence from a meta-analysis. Onco Targets Ther (2018) 11:1013–23. doi: 10.2147/OTT.S154114
52. Wang PP, Liu SH, Chen CT, Lv L, Li D, Liu QY, et al. Circulating tumor cells as a new predictive and prognostic factor in patients with small cell lung cancer. J Cancer (2020) 11(8):2113–22. doi: 10.7150/jca.35308
53. Delgado-Ureña M, Ortega FG, de Miguel-Pérez D, Rodriguez-Martínez A, García-Puche JL, Ilyine H, et al. Circulating tumor cells criteria (CyCAR) versus standard RECIST criteria for treatment response assessment in metastatic colorectal cancer patients. J Transl Med (2018) 16(1):251. doi: 10.1186/s12967-018-1624-2
54. Krebs MG, Hou JM, Ward TH, Blackhall FH, Dive C. Circulating tumour cells: their utility in cancer management and predicting outcomes. Ther Adv Med Oncol (2010) 2(6):351–65. doi: 10.1177/1758834010378414
55. Lin D, Shen L, Luo M, Zhang K, Li J, Yang Q, et al. Circulating tumor cells: biology and clinical significance. Signal Transduct Target Ther (2021) 6(1):404. doi: 10.1038/s41392-021-00817-8
56. Jiang M, Jin S, Han J, Li T, Shi J, Zhong Q, et al. Detection and clinical significance of circulating tumor cells in colorectal cancer. biomark Res (2021) 9:85. doi: 10.1186/s40364-021-00326-4
57. Masuda T, Hayashi N, Iguchi T, Ito S, Eguchi H, Mimori K. Clinical and biological significance of circulating tumor cells in cancer. Mol Oncol (2016) 10(3):408–17. doi: 10.1016/j.molonc.2016.01.010
58. Rossi E, Fabbri F. CTCs 2020: Great expectations or unreasonable dreams. Cells (2019) 8(9):989. doi: 10.3390/cells8090989
59. Chelakkot C, Yang H, Shin YK. Relevance of circulating tumor cells as predictive markers for cancer incidence and relapse. Pharm (Basel) (2022) 15(1):75. doi: 10.3390/ph15010075
60. Alix-Panabières C, Pantel K. Liquid biopsy: From discovery to clinical application. Cancer Discovery (2021) 11(4):858–73. doi: 10.1158/2159-8290.CD-20-1311
61. Alix-Panabières C, Pantel K. Challenges in circulating tumour cell research. Nat Rev Cancer (2014) 14(9):623–31. doi: 10.1038/nrc3820
62. Au SH, Storey BD, Moore JC, Tang Q, Chen YL, Javaid S, et al. Clusters of circulating tumor cells traverse capillary-sized vessels. Proc Natl Acad Sci U S A (2016) 113(18):4947–52. doi: 10.1073/pnas.1524448113
63. Murlidhar V, Reddy RM, Fouladdel S, Zhao L, Ishikawa MK, Grabauskiene S, et al. Poor prognosis indicated by venous circulating tumor cell clusters in early-stage lung cancers. Cancer Res (2017) 77(18):5194–206. doi: 10.1158/0008-5472.CAN-16-2072
64. Micalizzi DS, Maheswaran S, Haber DA. A conduit to metastasis: circulating tumor cell biology. Genes Dev (2017) 31(18):1827–40. doi: 10.1101/gad.305805.117
65. Cima I, Kong SL, Sengupta D, Tan IB, Phyo WM, Lee D, et al. Tumor-derived circulating endothelial cell clusters in colorectal cancer. Sci Transl Med (2016) 8(345):345ra89. doi: 10.1126/scitranslmed.aad7369
66. Xing F, Saidou J, Watabe K. Cancer associated fibroblasts (CAFs) in tumor microenvironment. Front Biosci (Landmark Ed) (2010) 15(1):166–79. doi: 10.2741/3613
67. Tommelein J, Verset L, Boterberg T, Demetter P, Bracke M, De Wever O. Cancer-associated fibroblasts connect metastasis-promoting communication in colorectal cancer. Front Oncol (2015) 5:63. doi: 10.3389/fonc.2015.00063
68. Deng L, Jiang N, Zeng J, Wang Y, Cui H. The versatile roles of cancer-associated fibroblasts in colorectal cancer and therapeutic implications. Front Cell Dev Biol (2021) 9:733270. doi: 10.3389/fcell.2021.733270
69. Chen YF, Yu ZL, Lv MY, Cai ZR, Zou YF, Lan P, et al. Cancer-associated fibroblasts impact the clinical outcome and treatment response in colorectal cancer via immune system modulation: a comprehensive genome-wide analysis. Mol Med (2021) 27(1):139. doi: 10.1186/s10020-021-00402-3
70. Paget S. The distribution of secondary growths in cancer of the breast. 1889 Cancer Metastasis Rev (1989) 8(2):98–101.
71. Danese E, Montagnana M, Lippi G. Circulating molecular biomarkers for screening or early diagnosis of colorectal cancer: Which is ready for prime time? Ann Transl Med (2019) 7(21):610. doi: 10.21037/atm.2019.08.97
72. Cayrefourcq L, Mazard T, Joosse S, Solassol J, Ramos J, Assenat E, et al. Establishment and characterization of a cell line from human circulating colon cancer cells. Cancer Res (2015) 75(5):892–901. doi: 10.1158/0008-5472.CAN-14-2613
73. Soler A, Cayrefourcq L, Mazard T, Babayan A, Lamy PJ, Assou S, et al. Autologous cell lines from circulating colon cancer cells captured from sequential liquid biopsies as model to study therapy-driven tumor changes. Sci Rep (2018) 8(1):15931. doi: 10.1038/s41598-018-34365-z
74. Grillet F, Bayet E, Villeronce O, Zappia L, Lagerqvist EL, Lunke S, et al. Circulating tumour cells from patients with colorectal cancer have cancer stem cell hallmarks in ex vivo culture. Gut (2017) 66(10):1802–10. doi: 10.1136/gutjnl-2016-311447
75. Chauhan A, Kaur R, Ghoshal S, Pal A. Exploration of circulating tumour cell (CTC) biology: A paradigm shift in liquid biopsy. Indian J Clin Biochem (2021) 36(2):131–42. doi: 10.1007/s12291-020-00923-4
76. Yang C, Xia BR, Jin WL, Lou G. Circulating tumor cells in precision oncology: clinical applications in liquid biopsy and 3D organoid model. Cancer Cell Int (2019) 19:341. doi: 10.1186/s12935-019-1067-8
77. Agarwal A, Balic M, El-Ashry D, Cote RJ. Circulating tumor cells: Strategies for capture, analyses, and propagation. Cancer J (2018) 24(2):70–7. doi: 10.1097/PPO.0000000000000310
78. Yadav A, Kumar A, Siddiqui MH. Detection of circulating tumour cells in colorectal cancer: Emerging techniques and clinical implications. World J Clin Oncol (2021) 12(12):1169–81. doi: 10.5306/wjco.v12.i12.1169
79. Maestro LM, Sastre J, Rafael SB, Veganzones SB, Vidaurreta M, Martín M, et al. Circulating tumor cells in solid tumor in metastatic and localized stages. Anticancer Res (2009) 29(11):4839–43.
80. Chiu TK, Chou WP, Huang SB, Wang HM, Lin YC, Hsieh CH, et al. Application of optically-induced-dielectrophoresis in microfluidic system for purification of circulating tumour cells for gene expression analysis- cancer cell line model. Sci Rep (2016) 6:32851. doi: 10.1038/srep32851
81. Yu H, Ma L, Zhu Y, Li W, Ding L, Gao H. Significant diagnostic value of circulating tumour cells in colorectal cancer. Oncol Lett (2020) 20:317–25. doi: 10.3892/ol.2020.11537
82. Wang L, Zhou S, Zhang W, Wang J, Wang M, Hu X, et al. Circulating tumor cells as an independent prognostic factor in advanced colorectal cancer: a retrospective study in 121 patients. Int J Colorectal Dis (2019) 34:589–97. doi: 10.1007/s00384-018-03223-9
83. Bahnassy AA, Salem SE, Mohanad M, Abulezz NZ, Abdellateif MS, Hussein M, et al. Prognostic significance of circulating tumor cells (CTCs) in Egyptian non-metastatic colorectal cancer patients: A comparative study for four different techniques of detection (Flowcytometry, CellSearch, quantitative real-time PCR and cytomorphology). Exp Mol Pathol (2019) 106:90–101. doi: 10.1016/j.yexmp.2018.12.006
84. Gazzaniga P, Gradilone A, Petracca A, Nicolazzo C, Raimondi C, Iacovelli R, et al. Molecular markers in circulating tumour cells from metastatic colorectal cancer patients. J Cell Mol Med (2010) 14:2073–7. doi: 10.1111/j.1582-4934.2010.01117.x
85. Buim ME, Fanelli MF, Souza VS, Romero J, Abdallah EA, Mello CA, et al. Detection of KRAS mutations in circulating tumor cells from patients with metastatic colorectal cancer. Cancer Biol Ther (2015) 16:1289–95. doi: 10.1080/15384047.2015.1070991
86. Deng G, Krishnakumar S, Powell AA, Zhang H, Mindrinos MN, Telli ML, et al. Single cell mutational analysis of PIK3CA in circulating tumor cells and metastases in breast cancer reveals heterogeneity, discordance, and mutation persistence in cultured disseminated tumor cells from bone marrow. BMC Cancer (2014) 14:456. doi: 10.1186/1471-2407-14-456
87. Smit DJ, Cayrefourcq L, Haider MT, Hinz N, Pantel K, Alix-Panabières C, et al. High sensitivity of circulating tumor cells derived from a colorectal cancer patient for dual inhibition with AKT and mTOR inhibitors. Cells (2020) 9(9):2129. doi: 10.3390/cells9092129
88. Zhao R, Cai Z, Li S, Cheng Y, Gao H, Liu F, et al. Expression and clinical relevance of epithelial and mesenchymal markers in circulating tumor cells from colorectal cancer. Oncotarget (2017) 8(6):9293–302. doi: 10.18632/oncotarget.14065
89. Raposo G, Stoorvogel W. Extracellular vesicles: exosomes, microvesicles, and friends. J Cell Biol (2013) 200:373–83. doi: 10.1083/jcb.201211138
90. He M, Zeng Y. Microfluidic exosome analysis toward liquid biopsy for cancer. J Lab Autom (2016) 21:599–608. doi: 10.1177/2211068216651035
91. Andaloussi S EL, Mäger I, Breakefield XO, Wood MJ. Extracellular vesicles: biology and emerging therapeutic opportunities. Nat Rev Drug Discov (2013) 12(5):347–57. doi: 10.1038/nrd3978
92. Kalluri R, LeBleu VS. The biology, function, and biomedical applications of exosomes. Science (2020) 367(6478):eaau6977. doi: 10.1126/science.aau6977
93. Mosquera-Heredia MI, Morales LC, Vidal OM, Barceló E, Silvera-Redondo C, Vélez JI, et al. Exosomes: Potential disease biomarkers and new therapeutic targets. Biomedicines (2021) 9(8):1061. doi: 10.3390/biomedicines9081061
94. Brinton LT, Sloane HS, Kester M, Kelly KA. Formation and role of exosomes in cancer. Cell Mol Life Sci (2015) 72(4):659–71. doi: 10.1007/s00018-014-1764-3
95. Umwali Y, Yue CB, Gabriel ANA, Zhang Y, Zhang X. Roles of exosomes in diagnosis and treatment of colorectal cancer. World J Clin Cases (2021) 9(18):4467–79. doi: 10.12998/wjcc.v9.i18.4467
96. Zhou J, Li XL, Chen ZR, Chng WJ. Tumor-derived exosomes in colorectal cancer progression and their clinical applications. Oncotarget (2017) 8(59):100781–90. doi: 10.18632/oncotarget.20117
97. Dohmen J, Semaan A, Kobilay M, Zaleski M, Branchi V, Schlierf A, et al. Diagnostic potential of exosomal microRNAs in colorectal cancer. Diagn (Basel) (2022) 12(6):1413. doi: 10.3390/diagnostics12061413
98. Li X, Wang Q, Wang R. Roles of exosome genomic DNA in colorectal cancer. Front Pharmacol (2022) 13:923232. doi: 10.3389/fphar.2022.923232
99. Huang Z, Feng Y. Exosomes derived from hypoxic colorectal cancer cells promote angiogenesis through Wnt4-induced β-catenin signaling in endothelial cells. Oncol Res (2017) 25(5):651–61. doi: 10.3727/096504016X14752792816791
100. Hu X, Mu Y, Liu J, Mu X, Gao F, Chen L, et al. Exosomes derived from hypoxic colorectal cancer cells transfer miR-410-3p to regulate tumor progression. J Cancer (2020) 11(16):4724–35. doi: 10.7150/jca.33232
101. Ren R, Sun H, Ma C, Liu J, Wang H. Colon cancer cells secrete exosomes to promote self-proliferation by shortening mitosis duration and activation of STAT3 in a hypoxic environment. Cell Biosci (2019) 9:62. doi: 10.1186/s13578-019-0325-8
102. Mulvey HE, Chang A, Adler J, Del Tatto M, Perez K, Quesenberry PJ, et al. Extracellular vesicle-mediated phenotype switching in malignant and non-malignant colon cells. BMC Cancer (2015) 15:571. doi: 10.1186/s12885-015-1568-3
103. Xing H, Liang C, Xu X, Sun H, Ma X, Jiang Z. Mesenchymal stroma/stem-like cells of GARP knockdown inhibits cell proliferation and invasion of mouse colon cancer cells (MC38) through exosomes. J Cell Mol Med (2020) 24(23):13984–90. doi: 10.1111/jcmm.16008
104. Vautrot V, Chanteloup G, Elmallah M, Cordonnier M, Aubin F, Garrido C, et al. Exosomal miRNA: Small molecules, big impact in colorectal cancer. J Oncol (2019) 2019:8585276. doi: 10.1155/2019/8585276
105. Valadi H, Ekström K, Bossios A, Sjöstrand M, Lee JJ, Lötvall JO. Exosome-mediated transfer of mRNAs and microRNAs is a novel mechanism of genetic exchange between cells. Nat Cell Biol (2007) 9(6):654–9. doi: 10.1038/ncb1596
106. Lan J, Sun L, Xu F, Liu L, Hu F, Song D, et al. M2 macrophage-derived exosomes promote cell migration and invasion in colon cancer. Cancer Res (2019) 79(1):146–58. doi: 10.1158/0008-5472.CAN-18-0014
107. Ma YS, Wu TM, Ling CC, Yu F, Zhang J, Cao PS, et al. M2 macrophage-derived exosomal microRNA-155-5p promotes the immune escape of colon cancer by downregulating ZC3H12B. Mol Ther Oncolyt (2021) 20:484–98. doi: 10.1016/j.omto.2021.02.005
108. Li H, Li F. Exosomes from BM-MSCs increase the population of CSCs via transfer of miR-142-3p. Br J Cancer (2018) 119(6):744–55. doi: 10.1038/s41416-018-0254-z
109. Ganig N, Baenke F, Thepkaysone ML, Lin K, Rao VS, Wong FC, et al. Proteomic analyses of fibroblast- and serum-derived exosomes identify QSOX1 as a marker for non-invasive detection of colorectal cancer. Cancers (Basel) (2021) 13(6):1351. doi: 10.3390/cancers13061351
110. Slack FJ, Chinnaiyan AM. The role of non-coding RNAs in oncology. Cell (2019) 179(5):1033–55. doi: 10.1016/j.cell.2019.10.017
111. Weng M, Wu D, Yang C, Peng H, Wang G, Wang T, et al. Noncoding RNAs in the development, diagnosis, and prognosis of colorectal cancer. Transl Res (2017) 181:108–20. doi: 10.1016/j.trsl.2016.10.001
112. Zhao H, Yang L, Baddour J, Achreja A, Bernard V, Moss T, et al. Tumor microenvironment derived exosomes pleiotropically modulate cancer cell metabolism. Elife (2016) 5:e10250. doi: 10.7554/eLife.10250
113. Hu YB, Yan C, Mu L, Mi YL, Zhao H, Hu H, et al. Correction: Exosomal wnt-induced dedifferentiation of colorectal cancer cells contributes to chemotherapy resistance. Oncogene (2019) 38(35):6319–21. doi: 10.1038/s41388-019-0863-x
114. Lian Q, Xu J, Yan S, Huang M, Ding H, Sun X, et al. Chemotherapy-induced intestinal inflammatory responses are mediated by exosome secretion of double-strand DNA via AIM2 inflammasome activation. Cell Res (2017) 27(6):784–800. doi: 10.1038/cr.2017.54
115. Vanpouille-Box C, Alard A, Aryankalayil MJ, Sarfraz Y, Diamond JM, Schneider RJ, et al. DNA Exonuclease Trex1 regulates radiotherapy-induced tumour immunogenicity. Nat Commun (2017) 8:15618. doi: 10.1038/ncomms15618
116. Zhang Q, Jeppesen DK, Higginbotham JN, Demory Beckler M, Poulin EJ, Walsh AJ, et al. Mutant KRAS exosomes alter the metabolic state of recipient colonic epithelial cells. Cell Mol Gastroenterol Hepatol (2018) 5(4):627–629.e6. doi: 10.1016/j.jcmgh.2018.01.013
117. Zhao F, Zheng T, Gong W, Wu J, Xie H, Li W, et al. Extracellular vesicles package dsDNA to aggravate crohn's disease by activating the STING pathway. Cell Death Dis (2021) 12(9):815. doi: 10.1038/s41419-021-04101-z
118. Sharma A, Johnson A. Exosome DNA: Critical regulator of tumor immunity and a diagnostic biomarker. J Cell Physiol (2020) 235(3):1921–32. doi: 10.1002/jcp.29153
119. Hao YX, Li YM, Ye M, Guo YY, Li QW, Peng XM, et al. KRAS and BRAF mutations in serum exosomes from patients with colorectal cancer in a Chinese population. Oncol Lett (2017) 13(5):3608–16. doi: 10.3892/ol.2017.5889
120. Lucchetti D, Zurlo IV, Colella F, Ricciardi-Tenore C, Di Salvatore M, Tortora G, et al. Mutational status of plasma exosomal KRAS predicts outcome in patients with metastatic colorectal cancer. Sci Rep (2021) 11(1):22686. doi: 10.1038/s41598-021-01668-7
121. Wan JCM, Massie C, Garcia-Corbacho J, Mouliere F, Brenton JD, Caldas C, et al. Liquid biopsies come of age: towards implementation of circulating tumour DNA. Nat Rev Cancer (2017) 17(4):223–38. doi: 10.1038/nrc.2017.7
122. Leon SA, Shapiro B, Sklaroff DM, Yaros MJ. Free DNA in the serum of cancer patients and the effect of therapy. Cancer Res (1977) 37(3):646–50.
123. Hsieh JS, Lin SR, Chang MY, Chen FM, Lu CY, Huang TJ, et al. K-Ras, and p53 gene mutations in colorectal cancer patients: correlation to clinicopathologic features and postoperative surveillance. Am Surg (2005) 71(4):336–43. doi: 10.1177/000313480507100413
124. Sclafani F, Chau I, Cunningham D, Hahne JC, Vlachogiannis G, Eltahir Z, et al. KRAS and BRAF mutations in circulating tumour DNA from locally advanced rectal cancer. Sci Rep (2018) 8(1):1445. doi: 10.1038/s41598-018-19212-5
125. Nakamura Y, Yokoyama S, Matsuda K, Tamura K, Mitani Y, Iwamoto H, et al. Preoperative detection of KRAS mutated circulating tumor DNA is an independent risk factor for recurrence in colorectal cancer. Sci Rep (2021) 11(1):441. doi: 10.1038/s41598-020-79909-4
126. Perdyan A, Spychalski P, Kacperczyk J, Rostkowska O, Kobiela J. Circulating tumor DNA in KRAS positive colorectal cancer patients as a prognostic factor - a systematic review and meta-analysis. Crit Rev Oncol Hematol (2020) 154:103065. doi: 10.1016/j.critrevonc.2020.103065
127. Naidoo M, Gibbs P, Tie J. ctDNA and adjuvant therapy for colorectal cancer: Time to re-invent our treatment paradigm. Cancers (Basel) (2021) 13(2):346. doi: 10.3390/cancers13020346
128. Ginghina O, Hudita A, Zamfir M, Spanu A, Mardare M, Bondoc I, et al. Liquid biopsy and artificial intelligence as tools to detect signatures of colorectal malignancies: A modern approach in patient's stratification. Front Oncol (2022) 12:856575. doi: 10.3389/fonc.2022.856575
129. Petit J, Carroll G, Gould T, Pockney P, Dun M, Scott RJ. Cell-free DNA as a diagnostic blood-based biomarker for colorectal cancer: a systematic review. J Surg Res (2019) 236:184–97. doi: 10.1016/j.jss.2018.11.029
130. Sun J, Fei F, Zhang M, Li Y, Zhang X, Zhu S, et al. The role of SEPT9 in screening, diagnosis, and recurrence monitoring of colorectal cancer. BMC Cancer (2019) 19:450. doi: 10.1186/s12885-019-5663-8
131. Bi F, Wang Q, Dong Q, Wang Y, Zhang L, Zhang J. Circulating tumor DNA in colorectal cancer: opportunities and challenges. Am J Transl Res (2020) 12(3):1044–55.
132. Zygulska AL, Pierzchalski P. Novel diagnostic biomarkers in colorectal cancer. Int J Mol Sci (2022) 23(2):852. doi: 10.3390/ijms23020852
133. Loktionov A. Biomarkers for detecting colorectal cancer non-invasively: DNA, RNA or proteins? World J Gastrointest Oncol (2020) 12(2):124–48. doi: 10.4251/wjgo.v12.i2.124
134. Kanaan Z, Roberts H, Eichenberger MR, Billeter A, Ocheretner G, Pan J, et al. A plasma microRNA panel for detection of colorectal adenomas: A step toward more precise screening for colorectal cancer. Ann Surg (2013) 258:400–8. doi: 10.1097/SLA.0b013e3182a15bcc
135. Giráldez MD, Lozano JJ, Ramírez G, Hijona E, Bujanda L, Castells A, et al. Circulating microRNAs as biomarkers of colorectal cancer: Results from a genome-wide profiling and validation study. Clin Gastroenterol Hepatol (2013) 11:681–8. doi: 10.1016/j.cgh.2012.12.009
136. Wang R, Wen H, Xu Y, Chen Q, Luo Y, Lin Y, et al. Circulating microRNAs as a novel class of diagnostic biomarkers in gastrointestinal tumors detection: A meta-analysis based on 42 articles. PloS One (2014) 9:e113401. doi: 10.1371/journal.pone.0113401
137. Slaby O. Non-coding RNAs as biomarkers for colorectal cancer screening and early detection. Adv Exp Med Biol (2016) 937:153–70. doi: 10.1007/978-3-319-42059-2_8
138. Carter JV, Galbraith NJ, Yang D, Burton JF, Walker SP, Galandiuk S. Blood-based microRNAs as biomarkers for the diagnosis of colorectal cancer: A systematic review and meta-analysis. Br J Cancer (2017) 116:762–74. doi: 10.1038/bjc.2017.12
139. Chen B, Xia Z, Deng YN, Yang Y, Zhang P, Zhu H, et al. Emerging microRNA biomarkers for colorectal cancer diagnosis and prognosis. Open Biol (2019) 9:180212. doi: 10.1098/rsob.180212
140. Sabry D, El-Deek SEM, Maher M, El-Baz MAH, El-Bader HM, Amer E, et al. Role of miRNA-210, miRNA-21 and miRNA-126 as diagnostic biomarkers in colorectal carcinoma: Impact of HIF-1α-VEGF signaling pathway. Mol Cell Biochem (2019) 454:177–89. doi: 10.1007/s11010-018-3462-1
141. Ng L, Wan TM, Man JH, Chow AK, Iyer D, Chen G, et al. Identification of serum miR-139-3p as a non-invasive biomarker for colorectal cancer. Oncotarget (2017) 8:27393–400. doi: 10.18632/oncotarget.16171
142. Wang P, He X. Current research on circular RNAs associated with colorectal cancer. Scand J Gastroenterol (2017) 52:1203–10. doi: 10.1080/00365521.2017.1365168
143. Liu X, Xu T, Hu X, Chen X, Zeng K, Sun L, et al. Elevated circulating miR-182 acts as a diagnostic biomarker for early colorectal cancer. Cancer Manage Res (2018) 10:857–65. doi: 10.2147/CMAR.S158016
144. Bilegsaikham E, Liu HN, Shen XZ, Liu TT. Circulating miR-338-5p is a potential diagnostic biomarker in colorectal cancer. J Dig Dis (2018) 19:404–10. doi: 10.1111/1751-2980.12643
145. Krawczyk P, Powrózek T, Olesi ´nski T, Dmitruk A, Dziwota J, Kowalski D, et al. Evaluation of miR-506 and miR-4316 expression in early and non-invasive diagnosis of colorectal cancer. Int J Color Dis (2017) 32:1057–60. doi: 10.1007/s00384-017-2814-8
146. Nonaka R, Miyake Y, Hata T, Kagawa Y, Kato T, Osawa H, et al. Circulating miR-103 and miR-720 as novel serum biomarkers for patients with colorectal cancer. Int J Oncol (2015) 47:1097–102. doi: 10.3892/ijo.2015.3064
147. Sarlinova ,M, Halasa M, Mistuna D, Musak L, Iliev R, Slaby O, et al. miR-21, miR-221 and miR-150 are deregulated in peripheral blood of patients with colorectal cancer. Anticancer Res (2016) 36:5449–54. doi: 10.21873/anticanres.11124
148. Chang PY, Chen CC, Chang YS, Tsai WS, You JF, Lin GP, et al. MicroRNA-223 and microRNA-92a in stool and plasma samples act as complementary biomarkers to increase colorectal cancer detection. Oncotarget (2016) 7:10663–75. doi: 10.18632/oncotarget.7119
149. Nikolaou S, Qiu S, Fiorentino F, Rasheed S, Tekkis P, Kontovounisios C. Systematic review of blood diagnostic markers in colorectal cancer. Tech Coloproctol (2018) 22:481–98. doi: 10.1007/s10151-018-1820-3
150. Liu X, Xu X, Pan B, He B, Chen X, Zeng K, et al. Circulating miR-1290 and miR-320d as novel diagnostic biomarkers of human colorectal cancer. J Cancer (2019) 10:43–50. doi: 10.7150/jca.26723
151. Wikberg ML, Myte R, Palmqvist R, van Guelpen B, Ljuslinder I. Plasma miRNA can detect colorectal cancer, but how early? Cancer Med (2018) 7:1697–705. doi: 10.1002/cam4.1398
152. Herreros-Villanueva M, Duran-Sanchon S, Martin AC, Perez-Palacios R, Vila-Navarro E, Marcuello M, et al. Plasma microRNA signature validation for early detection of colorectal cancer. Clin Transl Gastroenterol (2019) 10:e00003. doi: 10.14309/ctg.0000000000000003
153. Zhang H, Zhu M, Shan X, Zhou X, Wang T, Zhang J, et al. A panel of seven-miRNA signature in plasma as potential biomarker for colorectal cancer diagnosis. Gene (2019) 687:246–54. doi: 10.1016/j.gene.2018.11.055
154. Tian J, Xi X, Wang J, Yu J, Huang Q, Ma R, et al. CircRNA hsa_circ_0004585 as a potential biomarker for colorectal cancer. Cancer Manage Res (2019) 11:5413–23. doi: 10.2147/CMAR.S199436
155. Karimi N, Hosseinpour Feizi MA, Safarazadeh R, Hashemzadeh S, Baradaran B, Shokouhi B, et al. Serum overexpression of miR-301a and miR-23a in patients with colorectal cancer. J Chin Med Assoc (2019) 82:215–20. doi: 10.1097/JCMA.0000000000000031
156. Tan Y, Lin JJ, Yang XF, Gou DM, Wu FL, Li FR, et al. A panel of three plasma microRNAs for colorectal cancer diagnosis. Cancer Epidemiol (2019) 60:67–76. doi: 10.1016/j.canep.2019.01.015
157. Maminezdah H, Ghanadian S, Pakravan K, Razmara E, Rouhollah F, Mossahebi-Mohammadi M, et al. A panel of six-circulating miRNA signature in serum and its potential diagnostic value in colorectal cancer. Life Sci (2020) 258:118226. doi: 10.1016/j.lfs.2020.118226
158. Liu W, Yang D, Chen L, Liu Q, Wang W, Yang Z, et al. Plasma exosomal miRNA-139-3p is a novel biomarker of colorectal cancer. J Cancer (2020) 11:4899–906. doi: 10.7150/jca.45548
159. Jin XH, Lu S, Wang AF. Expression and clinical significance of miR-4516 and miR-21-5p in serum of patients with colorectal cancer. BMC Cancer (2020) 20:241. doi: 10.1186/s12885-020-06715-6
160. Zhao W, Song M, Zhang J, Kuerban M, Wang H. Combined identification of long non-coding RNA CCAT1 and HOTAIR in serum as an effective screening for colorectal carcinoma. Int J Clin Exp Pathol (2015) 8:14131–40.
161. Wang C, Yu J, Han Y, Li L, Li J, Li T, et al. Long non-coding RNAs LOC285194, RP11-462C24.1 and Nbla12061 in serum provide a new approach for distinguishing patients with colorectal cancer from healthy controls. Oncotarget (2016) 7:70769–78. doi: 10.18632/oncotarget.12220
162. Dai M, Chen X, Mo S, Li J, Huang Z, Huang S, et al. Meta-signature LncRNAs serve as novel biomarkers for colorectal cancer: Integrated bioinformatics analysis, experimental validation and diagnostic evaluation. Sci Rep (2017) 7:46572. doi: 10.1038/srep46572
163. Barbagallo C, Brex D, Caponnetto A, Cirnigliaro M, Scalia M, Magnano A, et al. LncRNA UCA1, upregulated in CRC biopsies and downregulated in serum exosomes, controls mRNA expression by RNA-RNA interactions. Mol Ther Nucleic Acids (2018) 12:229–41. doi: 10.1016/j.omtn.2018.05.009
164. Liu H, Ye D, Chen A, Tan D, Zhang W, Jiang W, et al. A pilot study of new promising non-coding RNA diagnostic biomarkers for early-stage colorectal cancers. Clin Chem Lab Med (2019) 57:1073–83. doi: 10.1515/cclm-2019-0052
165. Dong L, Lin W, Qi P, Xu MD, Wu X, Ni S, et al. Circulating long RNAs in serum extracellular vesicles: Their characterization and potential application as biomarkers for diagnosis of colorectal cancer. Cancer Epidemiol biomark Prev (2016) 25:1158–66. doi: 10.1158/1055-9965.EPI-16-0006
166. Koga Y, Yasunaga M, Takahashi A, Kuroda J, Moriya Y, Akasu T, et al. MicroRNA expression profiling of exfoliated colonocytes isolated from feces for colorectal cancer screening. Cancer Prev Res (2010) 3:1435–42. doi: 10.1158/1940-6207.CAPR-10-0036
167. Kalimutho M, Del Vecchio Blano G, Di Cecilia S, Sileri P, Cretella M, Pallone F, et al. Differential expression of miR-144* as a novel fecal-based diagnostic marker for colorectal cancer. J Gastroenterol (2011) 46:1391–402. doi: 10.1007/s00535-011-0456-0
168. Ahmed FE, Ahmed NC, Vos PW, Bonnerup C, Atkins JN, Casey M, et al. Diagnostic microRNA markers to screen for sporadic human colon cancer in stool: I. proof of principle. Cancer Genom Proteomics (2013) 10:93–113.
169. Wu CW, Ng SS, Dong YJ, Ng SC, Leung WW, Lee CW, et al. Detection of miR-92a and miR-21 in stool samples as potential screening biomarkers for colorectal cancer and polyps. Gut (2012) 61:739–45. doi: 10.1136/gut.2011.239236
170. Wu CW, Ng SC, Dong Y, Tian L, Ng SS, Leung WW, et al. Identification of microRNA-135b in stool as a potential noninvasive biomarker for colorectal cancer and adenoma. Clin Cancer Res (2014) 20:2994–3002. doi: 10.1158/1078-0432.CCR-13-1750
171. Yau TO, Wu CW, Dong Y, Tang CM, Ng SSM, Chan FKL, et al. microRNA-221 and microRNA-18a identification in stool as potential biomarkers for the non-invasive diagnosis of colorectal carcinoma. Br J Cancer (2014) 111:1765–71. doi: 10.1038/bjc.2014.484
172. Phua LC, Chue XP, Koh PK, Cheah PY, Chan EC, Ho HK. Global fecal microRNA profiling in the identification of biomarkers for colorectal cancer screening among asians. Oncol Rep (2014) 32:97–104. doi: 10.3892/or.2014.3193
173. Zhu Y, Xu A, Li J, Fu J, Wang G, Yang Y, et al. Fecal miR-29a and miR-224 as the noninvasive biomarkers for colorectal cancer. Cancer biomark (2016) 16:259–64. doi: 10.3233/CBM-150563
174. Bastaminejad S, Taherikalani M, Ghanbari R, Akbari A, Shabab N, Saidijam M. Investigation of microRNA-21 expression levels in serum and stool as a potential non-invasive biomarker for diagnosis of colorectal cancer. Iran Biomed J (2017) 21:106–13. doi: 10.18869/acadpub.ibj.21.2.106
175. Choi HH, Cho YS, Choi H, Kim HK, Lim SS, Chae HS. Stool-based miR-92a and miR-144* as noninvasive biomarkers for colorectal cancer screening. Oncology (2019) 97:173–9. doi: 10.1159/000500639
176. Li L, Wang A, Cai M, Tong M, Chen F, Huang L. Identification of stool miR-135b-5p as a non-invasive diaognostic biomarker in later tumor stage of colorectal cancer. Life Sci (2020) 260:118417. doi: 10.1016/j.lfs.2020.118417
177. Duran-Sanchon S, Moreno L, Auge JM, Serra-Burrel M, Cuatrecasas M, Moreira L, et al. Identification and validation of microRNA profiles in fecal samples for detection of colorectal cancer. Gastroenterology (2020) 158:947–54. doi: 10.1053/j.gastro.2019.10.005
178. Wang J, Yan F, Zhao Q, Zhan F, Wang R, Wang L, et al. Circulating exosomal miR-125a-3p as a novel biomarker for early-stage colon cancer. Sci Rep (2017) 7:4150. doi: 10.1038/s41598-017-04386-1
179. Zhou H, Zhu L, Song J, Wang G, Li P, Li W, et al. Liquid biopsy at the frontier of detection, prognosis and progression monitoring in colorectal cancer. Mol Cancer (2022) 21(1):86. doi: 10.1186/s12943-022-01556-2
180. Clay Montier LL, Deng JJ, Bai Y. Number matters: control of mammalian mitochondrial DNA copy number. J Genet Genomics (2009) 36(3):125–31. doi: 10.1016/S1673-8527(08)60099-5
181. Chen D, Yu Z, Zhu Z, Lopez CD. The p53 pathway promotes efficient mitochondrial DNA base excision repair in colorectal cancer cells. Cancer Res (2006) 66(7):3485–94. doi: 10.1158/0008-5472.CAN-05-4103
182. Wallace DC. A mitochondrial paradigm of metabolic and degenerative diseases, aging, and cancer: a dawn for evolutionary medicine. Annu Rev Genet (2005) 39:359–407. doi: 10.1146/annurev.genet.39.110304.095751
183. Hertweck KL, Dasgupta S. The landscape of mtDNA modifications in cancer: A tale of two cities. Front Oncol (2017) 7:262. doi: 10.3389/fonc.2017.00262
184. van Osch FH, Voets AM, Schouten LJ, Gottschalk RW, Simons CC, van Engeland M, et al. Mitochondrial DNA copy number in colorectal cancer: between tissue comparisons, clinicopathological characteristics and survival. Carcinogenesis (2015) 36(12):1502–10. doi: 10.1093/carcin/bgv151
185. Lo YM, Chan KC, Sun H, Chen EZ, Jiang P, Lun FM, et al. Maternal plasma DNA sequencing reveals the genome-wide genetic and mutational profile of the fetus. Sci Transl Med (2010) 2:61ra91. doi: 10.1126/scitranslmed.3001720
186. An Q, Hu Y, Li Q, Chen X, Huang J, Pellegrini M, et al. The size of cell-free mitochondrial DNA in blood is inversely correlated with tumor burden in cancer patients. Precis Clin Med (2019) 2:131–9. doi: 10.1093/pcmedi/pbz014
187. Mouliere F, Rosenfeld N. Circulating tumor-derived DNA is shorter than somatic DNA in plasma. Proc Natl Acad Sci USA (2015) 112:3178–9. doi: 10.1073/pnas.1501321112
188. Haupts A, Vogel A, Foersch S, Hartmann M, Maderer A, Wachter N, et al. Comparative analysis of nuclear and mitochondrial DNA from tissue and liquid biopsies of colorectal cancer patients. Sci Rep (2021) 11(1):16745. doi: 10.1038/s41598-021-95006-6
189. Mik M, Dziki L, Malinowska K, Trzcinski R, Majsterek I, Dziki A. Polymorphism of MSH2 Gly322Asp and MLH1 -93G>A in non-familial colon cancer - a case-controlled study. Arch Med Sci (2017) 13(6):1295–302. doi: 10.5114/aoms.2017.67024
190. Sheng WY, Yong Z, Yun Z, Hong H, Hai LL. Toll-like receptor 4 gene polymorphisms and susceptibility to colorectal cancer: a meta-analysis and review. Arch Med Sci (2015) 11(4):699–707. doi: 10.5114/aoms.2015.53288
191. Lee HC, Chang CM, Chi CW. Somatic mutations of mitochondrial DNA in aging and cancer progression. Ageing Res Rev (2010) 9 Suppl 1:S47–58. doi: 10.1016/j.arr.2010.08.009
192. Guo J, Zheng L, Liu W, Wang X, Wang Z, Wang Z, et al. Frequent truncating mutation of TFAM induces mitochondrial DNA depletion and apoptotic resistance in microsatellite-unstable colorectal cancer. Cancer Res (2011) 71(8):2978–87. doi: 10.1158/0008-5472.CAN-10-3482
193. Chen T, He J, Shen L, Fang H, Nie H, Jin T, et al. The mitochondrial DNA 4,977-bp deletion and its implication in copy number alteration in colorectal cancer. BMC Med Genet (2011) 12:8. doi: 10.1186/1471-2350-12-8
194. Tatullo M, Marrelli B, Benincasa C, Aiello E, Makeeva I, Zavan B, et al. Organoids in translational oncology. J Clin Med (2020) 9(9):2774. doi: 10.3390/jcm9092774
195. Sogawa C, Eguchi T, Tran MT, Ishige M, Trin K, Okusha Y, et al. Antiparkinson drug benztropine suppresses tumor growth, circulating tumor cells, and metastasis by acting on SLC6A3/DAT and reducing STAT3. Cancers (Basel) (2020) 12(2):523. doi: 10.3390/cancers12020523
196. Drost J, Clevers H. Organoids in cancer research. Nat Rev Cancer (2018) 18(7):407–18. doi: 10.1038/s41568-018-0007-6
197. Dutta D, Heo I, Clevers H. Disease modeling in stem cell-derived 3D organoid systems. Trends Mol Med (2017) 23(5):393–410. doi: 10.1016/j.molmed.2017.02.007
198. Lou YR, Leung AW. Next generation organoids for biomedical research and applications. Biotechnol Adv (2018) 36(1):132–49. doi: 10.1016/j.biotechadv.2017.10.005
199. Sato T, Vries RG, Snippert HJ, van de Wetering M, Barker N, Stange DE, et al. Single Lgr5 stem cells build crypt-villus structures in vitro without a mesenchymal niche. Nature (2009) 459(7244):262–5. doi: 10.1038/nature07935
200. Nardella C, Lunardi A, Patnaik A, Cantley LC, Pandolfi PP. The APL paradigm and the "co-clinical trial" project. Cancer Discov (2011) 1(2):108–16. doi: 10.1158/2159-8290.CD-11-0061
201. Li X, Ootani A, Kuo C. An air-liquid interface culture system for 3D organoid culture of diverse primary gastrointestinal tissues. Methods Mol Biol (2016) 1422:33–40. doi: 10.1007/978-1-4939-3603-8_4
202. Vlachogiannis G, Hedayat S, Vatsiou A, Jamin Y, Fernández-Mateos J, Khan K, et al. Patient-derived organoids model treatment response of metastatic gastrointestinal cancers. Science (2018) 359(6378):920–6. doi: 10.1126/science.aao2774
203. Verissimo CS, Overmeer RM, Ponsioen B, Drost J, Mertens S, Verlaan-Klink I, et al. Targeting mutant RAS in patient-derived colorectal cancer organoids by combinatorial drug screening. Elife (2016) 5:e18489. doi: 10.7554/eLife.18489.041
204. Zhao H, Yan C, Hu Y, Mu L, Liu S, Huang K, et al. Differentiated cancer cell-originated lactate promotes the self-renewal of cancer stem cells in patient-derived colorectal cancer organoids. Cancer Lett (2020) 493:236–44. doi: 10.1016/j.canlet.2020.08.044
205. De Angelis ML, Francescangeli F, Nicolazzo C, Signore M, Giuliani A, Colace L, et al. An organoid model of colorectal circulating tumor cells with stem cell features, hybrid EMT state and distinctive therapy response profile. J Exp Clin Cancer Res (2022) 41(1):86. doi: 10.1186/s13046-022-02263-y
206. Lancaster MA, Corsini NS, Wolfinger S, Gustafson EH, Phillips AW, Burkard TR, et al. Guided self-organization and cortical plate formation in human brain organoids. Nat Biotechnol (2017) 35(7):659–66. doi: 10.1038/nbt.3906
207. Urbischek M, Rannikmae H, Foets T, Ravn K, Hyvönen M, de la Roche M. Organoid culture media formulated with growth factors of defined cellular activity. Sci Rep (2019) 9(1):6193. doi: 10.1038/s41598-019-42604-0
208. Lancaster MA, Huch M. Disease modelling in human organoids. Dis Model Mech (2019) 12(7):dmm039347. doi: 10.1242/dmm.039347
209. Stein A, Moehler M, Trojan J, Goekkurt E, Vogel A. Immuno-oncology in GI tumours: Clinical evidence and emerging trials of PD-1/PD-L1 antagonists. Crit Rev Oncol Hematol (2018) 130:13–26. doi: 10.1016/j.critrevonc.2018.07.001
210. Passaro A, Stenzinger A, Peters S. Tumor mutational burden as a pan-cancer biomarker for immunotherapy: The limits and potential for convergence. Cancer Cell (2020) 38(5):624–5. doi: 10.1016/j.ccell.2020.10.019
211. Sha D, Jin Z, Budczies J, Kluck K, Stenzinger A, Sinicrope FA. Tumor mutational burden as a predictive biomarker in solid tumors. Cancer Discov (2020) 10(12):1808–25. doi: 10.1158/2159-8290.CD-20-0522
212. Yoshino T, Arnold D, Taniguchi H, Pentheroudakis G, Yamazaki K, Xu RH, et al. Pan-Asian adapted ESMO consensus guidelines for the management of patients with metastatic colorectal cancer: a JSMO-ESMO initiative endorsed by CSCO, KACO, MOS, SSO and TOS. Ann Oncol (2018) 29(1):44–70. doi: 10.1093/annonc/mdx738
213. Di Nicolantonio F, Vitiello PP, Marsoni S, Siena S, Tabernero J, Trusolino L, et al. Precision oncology in metastatic colorectal cancer - from biology to medicine. Nat Rev Clin Oncol (2021) 18(8):506–25. doi: 10.1038/s41571-021-00495-z
214. Boland C, Goel A. Microsatellite instability in colorectal cancer. J Gastroenterol (2010) 138:2073–87. doi: 10.1053/j.gastro.2009.12.064
215. De Smedt L, Lemahieu J, Palmans S, Govaere O, Tousseyn T, Van Cutsem E, et al. Microsatellite instable vs stable colon carcinomas: Analysis of tumour heterogeneity, inflammation and angiogenesis. Br J Cancer (2015) 113:500–9. doi: 10.1038/bjc.2015.213
216. Nojadeh J, Sharif S, Sakhinia E. Microsatellite instability in colorectal cancer. EXCLI J (2018) 17:159–68. doi: 10.17179/excli2017-948
217. TCGA. Comprehensive molecular characterization of human colon and rectal cancer. Nature (2012) 487:330–7. doi: 10.1038/nature11252
218. Benatti P, Gafà R, Barana D, Marino M, Scarselli A, Pedroni M, et al. Microsatellite instability and colorectal cancer prognosis. Clin Cancer Res (2005) 11:8332–40. doi: 10.1158/1078-0432.CCR-05-1030
219. Bae JM, Kim JH, Kang GH. Molecular subtypes of colorectal cancer and their clinicopathologic features, with an emphasis on the serrated neoplasia pathway. Arch Pathol Lab Med (2016) 140:406–12. doi: 10.5858/arpa.2015-0310-RA
220. Devaraj B, Lee A, Cabrera BL, Miyai K, Luo L, Ramamoorthy S, et al. Relationship of EMAST and microsatellite instability among patients with rectal cancer. J Gastrointest Surg (2010) 14:1521–8. doi: 10.1007/s11605-010-1340-6
221. Hoogerbrugge N, Willems R, Van Krieken HJ, Kiemeney LA, Weijmans M, Nagengast FM, et al. Very low incidence of microsatellite instability in rectal cancers from families at risk for HNPCC. Clin Genet (2003) 63:64–70. doi: 10.1034/j.1399-0004.2003.630110.x
222. Samowitz WS, Curtin K, Wolff RK, Tripp SR, Caan BJ, Slattery ML. Microsatellite instability and survival in rectal cancer. Cancer Causes Control (2009) 20:1763–8. doi: 10.1007/s10552-009-9410-3
223. Kolligs FT. Diagnostics and epidemiology of colorectal cancer. Visc Med (2016) 32:158–64. doi: 10.1159/000446488
224. Casak SJ, Marcus L, Fashoyin-Aje L, Mushti SL, Cheng J, Shen YL, et al. FDA Approval summary: Pembrolizumab for the first-line treatment of patients with MSI-H/dMMR advanced unresectable or metastatic colorectal carcinoma. Clin Cancer Res (2021) 27:4680–4. doi: 10.1158/1078-0432.CCR-21-0557
225. Overman MJ, Lonardi S, Wong KYM, Lenz HJ, Gelsomino F, Aglietta M, et al. Durable clinical benefit with nivolumab plus ipilimumab in DNA mismatch repair-deficient/microsatellite instability-high metastatic colorectal cancer. J Clin Oncol (2018) 36:773–9. doi: 10.1200/JCO.2017.76.9901
226. Zhang X, Wu T, Cai X, Dong J, Xia C, Zhou Y, et al. Neoadjuvant immunotherapy for MSI-H/dMMR locally advanced colorectal cancer: New strategies and unveiled opportunities. Front Immunol (2022) 13. doi: 10.3389/fimmu.2022.795972
227. Minsky BD. Unique considerations in the patient with rectal cancer. Semin Oncol (2011) 38:542–51. doi: 10.1053/j.seminoncol.2011.05.008
228. Pérez-Ruiz E, Berraondo P. Immunological landscape and clinical management of rectal cancer. Front Immunol (2016) 7:1–6. doi: 10.3389/fimmu.2016.00061
229. Sargent DJ, Marsoni S, Monges G, Thibodeau SN, Labianca R, Hamilton SR, et al. Defective mismatch repair as a predictive marker for lack of efficacy of fluorouracil-based adjuvant therapy in colon cancer. J Clin Oncol (2010) 28:3219–26. doi: 10.1200/JCO.2009.27.1825
230. Goel A, Nagasaka T, Hamelin R, Boland CR. An optimized pentaplex PCR for detecting DNA mismatch repair-deficient colorectal cancers. PloS One (2010) 5. doi: 10.1371/annotation/572bb6d3-0315-40b1-a6d7-ce818809b5ea
231. Shemirani AI, Haghighi MM, Zadeh SM, Fatemi SR, Taleghani MY, Zali N, et al. Simplified MSI marker panel for diagnosis of colorectal cancer. Asian Pac J Cancer Prev (2011) 12:2101–4.
232. Brennetot C, Buhard O, Jourdan F, Flejou JF, Duval A, Hamelin R. Mononucleotide repeats BAT-26 and BAT-25 accurately detect MSI-h tumors and predict tumor content: Implications for population screening. Int J Cancer (2005) 113:446–50. doi: 10.1002/ijc.20586
233. Buhard O, Cattaneo F, Wong YF, Yim SF, Friedman E, Flejou JF, et al. Multipopulation analysis of polymorphisms in five mononucleotide repeats used to determine the microsatellite instability status of human tumors. J Clin Oncol (2005) 24:241–51. doi: 10.1200/JCO.2005.02.7227
234. Cicek MS, Lindor NM, Gallinger S, Bapat B, Hopper JL, Jenkins MA, et al. Quality assessment and correlation of microsatellite instability and immunohistochemical markers among population- and clinic-based colorectal tumors: Results from the colon cancer family registry. J Mol Diagn (2011) 13:271–81. doi: 10.1016/j.jmoldx.2010.12.004
235. Vorajee N. Epidermal growth factor receptor and anaplastic lymphoma kinase mutations detected by immunohistochemistry in lung adenocarcinoma in patients from Johannesburg. South Africa: University of the Witwatersrand (2017).
236. Zhang X, Li J. Era of universal testing of microsatellite instability in colorectal cancer. World J Gastrointest Oncol (2013) 5:12. doi: 10.4251/wjgo.v5.i2.12
237. Kumar A, Jain M, Yadav A, Kumari N, Krishnani N. Pattern of mismatch repair protein loss and its clinicopathological correlation in colorectal cancer in north India. South Afr J Surg (2018) 56:25–9. doi: 10.17159/2078-5151/2018/v56n1a2285
238. Chang DT, Pai RK, Rybicki LA, Dimaio MA, Limaye M, Jayachandran P, et al. Clinicopathologic and molecular features of sporadic early-onset colorectal adenocarcinoma: An adenocarcinoma with frequent signet ring cell differentiation, rectal and sigmoid involvement, and adverse morphologic features. Mod Pathol (2012) 25:1128–39. doi: 10.1038/modpathol.2012.61
239. Sehgal R, Sheahan K, O'Connell PR, Hanly AM, Martin ST, Winter DC, et al. Lynch syndrome: An updated review. Genes (Basel) (2014) 5:497–507. doi: 10.3390/genes5030497
240. Lin A, Zhang J, Luo P. Crosstalk between the MSI status and tumor microenvironment in colorectal cancer. Front Immunol (2020) 11:1–13. doi: 10.3389/fimmu.2020.02039
241. Mik M, Berut M, Dziki L, Trzcinski R, Dziki A. Right-and left-sided colon cancer-clinical and pathological differences of the disease entity in one organ. Arch Med Sci (2017) 13:157–62. doi: 10.5114/aoms.2016.58596
242. Takahashi Y, Sugai T, Habano W, Ishida K, Eizuka M, Otsuka K, et al. Molecular differences in the microsatellite stable phenotype between left-sided and right-sided colorectal cancer. Int J Cancer (2016) 139:2493–501. doi: 10.1002/ijc.30377
243. Demisse R, Damle N, Kim E, Gong J, Fakih M, Eng C, et al. Neoadjuvant immunotherapy-based systemic treatment in MMR-deficient or MSI-high rectal cancer: Case series. JNCCN J Natl Compr Cancer Netw (2020) 18:798–804. doi: 10.6004/jnccn.2020.7558
244. Cercek A, Lumish M, Sinopoli J, Weiss J, Shia J, Lamendola-Essel M, et al. PD-1 blockade in mismatch repair–deficient, locally advanced rectal cancer. N Engl J Med (2022) 386:2363–76. doi: 10.1056/NEJMoa2201445
245. Bonaventura P, Shekarian T, Alcazer V, Valladeau-Guilemond J, Valsesia-Wittmann S, Amigorena S, et al. Cold tumors: A therapeutic challenge for mmunotherapy. Front Immunol (2019) 10:168. doi: 10.3389/fimmu.2019.00168
246. Vafaei S, Zekiy AO, Khanamir RA, Zaman BA, Ghayourvahdat A, Azimizonuzi H, et al. Combination therapy with immune checkpoint inhibitors (ICIs); a new frontier. Cancer Cell Int (2022) 22:1–27. doi: 10.1186/s12935-021-02407-8
247. Gupta SL, Basu S, Soni V, Jaiswal RK. Immunotherapy: an alternative promising therapeutic approach against cancers. Mol Biol Rep (2022) 49:9903–13. doi: 10.1007/s11033-022-07525-8
248. Grosser R, Cherkassky L, Chintala N, Adusumilli PS. Combination immunotherapy with CAR T cells and checkpoint blockade for the treatment of solid tumors. Cancer Cell (2019) 36(5):471–82. doi: 10.1016/j.ccell.2019.09.006
249. Caruana I, Savoldo B, Hoyos V, Weber G, Liu H, Kim ES, et al. Heparanase promotes tumor infiltration and antitumor activity of CAR-redirected T lymphocytes. Nat Med (2015) 21(5):524–9. doi: 10.1038/nm.3833
250. Madsen DH, Bugge TH. The source of matrix-degrading enzymes in human cancer: Problems of research reproducibility and possible solutions. J Cell Biol (2015) 209(2):195–8. doi: 10.1083/jcb.201501034
252. Russell SJ, Peng KW, Bell JC. Oncolytic virotherapy. Nat Biotechnol (2012) 30(7):658–70. doi: 10.1038/nbt.2287
253. Klebanoff CA, Rosenberg SA, Restifo NP. Prospects for gene-engineered T cell immunotherapy for solid cancers. Nat Med (2016) 22(1):26–36. doi: 10.1038/nm.4015
254. Lu YC, Yao X, Li YF, El-Gamil M, Dudley ME, Yang JC, et al. Mutated PPP1R3B is recognized by T cells used to treat a melanoma patient who experienced a durable complete tumor regression. J Immunol (2013) 190(12):6034–42. doi: 10.4049/jimmunol.1202830
255. Robbins PF, Lu YC, El-Gamil M, Li YF, Gross C, Gartner J, et al. Mining exomic sequencing data to identify mutated antigens recognized by adoptively transferred tumor-reactive T cells. Nat Med. (2013) 19:747–52. doi: 10.1038/nm.3161
256. Dudley JC, Lin MT, Le DT, Eshleman JR. Microsatellite instability as a biomarker for PD-1 blockade. Clin Cancer Res (2016) 22(4):813–20. doi: 10.1158/1078-0432.CCR-15-1678
257. Malekzadeh P, Pasetto A, Robbins PF, Parkhurst MR, Paria BC, Jia L, et al. Neoantigen screening identifies broad TP53 mutant immunogenicity in patients with epithelial cancers. J Clin Invest (2019) 129(3):1109–14. doi: 10.1172/JCI123791
258. Alexandrov LB, Nik-Zainal S, Wedge DC, Aparicio SA, Behjati S, Biankin AV, et al. Signatures of mutational processes in human cancer. Nature (2013) 500(7463):415–21. doi: 10.1038/nature12477
259. Zheng Y, Fu Y, Wang PP, Ding ZY. Neoantigen: A promising target for the immunotherapy of colorectal cancer. Dis Markers (2022) 2022:8270305. doi: 10.1155/2022/8270305
260. Yarchoan M, Johnson BA 3rd, Lutz ER, Laheru DA, Jaffee EM. Targeting neoantigens to augment antitumour immunity. Nat Rev Cancer (2017) 17(4):209–22. doi: 10.1038/nrc.2016.154
261. Le DT, Durham JN, Smith KN, Wang H, Bartlett BR, Aulakh LK, et al. Mismatch repair deficiency predicts response of solid tumors to PD-1 blockade. Science (2017) 357(6349):409–13. doi: 10.1126/science.aan6733
262. Kreiter S, Vormehr M, van de Roemer N, Diken M, Löwer M, Diekmann J, et al. Mutant MHC class II epitopes drive therapeutic immune responses to cancer. Nature (2015) 520(7549):692–6. doi: 10.1038/nature14426
263. Parkhurst MR, Robbins PF, Tran E, Prickett TD, Gartner JJ, Jia L, et al. Unique neoantigens arise from somatic mutations in patients with gastrointestinal cancers. Cancer Discovery (2019) 9(8):1022–35. doi: 10.1158/2159-8290.CD-18-1494
264. Helfen A, Roth J, Ng T, Eisenblaetter M. In vivo imaging of pro- and antitumoral cellular components of the tumor microenvironment. J Nucl Med (2018) 59(2):183–8. doi: 10.2967/jnumed.117.198952
265. Wang M, Zhao J, Zhang L, Wei F, Lian Y, Wu Y, et al. Role of tumor microenvironment in tumorigenesis. J Cancer (2017) 8(5):761–73. doi: 10.7150/jca.17648
266. Van den Eynde M, Mlecnik B, Bindea G, Fredriksen T, Church SE, Lafontaine L, et al. The link between the multiverse of immune microenvironments in metastases and the survival of colorectal cancer patients. Cancer Cell (2018) 34(6):1012–26.e3. doi: 10.1016/j.ccell.2018.11.003
267. Russell JH, Ley TJ. Lymphocyte-mediated cytotoxicity. Annu Rev Immunol (2002) 20:323–70. doi: 10.1146/annurev.immunol.20.100201.131730
268. Narayanan S, Kawaguchi T, Yan L, Peng X, Qi Q, Takabe K. Cytolytic activity score to assess anticancer immunity in colorectal cancer. Ann Surg Oncol (2018) 25(8):2323–31. doi: 10.1245/s10434-018-6506-6
269. Mlecnik B, Tosolini M, Kirilovsky A, Berger A, Bindea G, Meatchi T, et al. Histopathologic-based prognostic factors of colorectal cancers are associated with the state of the local immune reaction. J Clin Oncol (2011) 29(6):610–8. doi: 10.1200/JCO.2010.30.5425
270. Moore KW, de Waal Malefyt R, Coffman RL, O'Garra A. Interleukin-10 and the interleukin-10 receptor. Annu Rev Immunol (2001) 19:683–765. doi: 10.1146/annurev.immunol.19.1.683
271. Jones LL, Alli R, Li B, Geiger TL. Differential T cell cytokine receptivity and not signal quality distinguishes IL-6 and IL-10 signaling during Th17 differentiation. J Immunol (2016) 196(7):2973–85. doi: 10.4049/jimmunol.1402953
272. Mei Z, Liu Y, Liu C, Cui A, Liang Z, Wang G, et al. Tumour-infiltrating inflammation and prognosis in colorectal cancer: systematic review and meta-analysis. Br J Cancer (2014) 110(6):1595–605. doi: 10.1038/bjc.2014.46
273. Maj T, Wang W, Crespo J, Zhang H, Wang W, Wei S, et al. Oxidative stress controls regulatory T cell apoptosis and suppressor activity and PD-L1-blockade resistance in tumor. Nat Immunol (2017) 18(12):1332–41. doi: 10.1038/ni.3868
274. Deng J, Galipeau J. B cells for cancer immunotherapy. Oncoimmunology (2014) 3(10):e955702. doi: 10.4161/21624011.2014.955702
275. Berntsson J, Nodin B, Eberhard J, Micke P, Jirström K. Prognostic impact of tumour-infiltrating b cells and plasma cells in colorectal cancer. Int J Cancer (2016) 139(5):1129–39. doi: 10.1002/ijc.30138
276. Liu R, Lu Z, Gu J, Liu J, Huang E, Liu X, et al. MicroRNAs 15A and 16-1 activate signaling pathways that mediate chemotaxis of immune regulatory b cells to colorectal tumors. Gastroenterology (2018) 154(3):637–651.e7. doi: 10.1053/j.gastro.2017.09.045
277. Seo H, Jeon I, Kim BS, Park M, Bae EA, Song B, et al. IL-21-mediated reversal of NK cell exhaustion facilitates anti-tumour immunity in MHC class I-deficient tumours. Nat Commun (2017) 8:15776. doi: 10.1038/ncomms15776
278. Pugh SA, Harrison RJ, Primrose JN, Khakoo SI. T Cells but not NK cells are associated with a favourable outcome for resected colorectal liver metastases. BMC Cancer (2014) 14:180. doi: 10.1186/1471-2407-14-180
279. Malietzis G, Lee GH, Jenkins JT, Bernardo D, Moorghen M, Knight SC, et al. Prognostic value of the tumour-infiltrating dendritic cells in colorectal cancer: A systematic review. Cell Commun Adhes (2015) 22(1):9–14. doi: 10.3109/15419061.2015.1036859
280. Li S, Xu F, Zhang J, Wang L, Zheng Y, Wu X, et al. Tumor-associated macrophages remodeling EMT and predicting survival in colorectal carcinoma. Oncoimmunology (2017) 7(2):e1380765. doi: 10.1080/2162402X.2017.1380765
281. Koelzer VH, Canonica K, Dawson H, Sokol L, Karamitopoulou-Diamantis E, Lugli A, et al. Phenotyping of tumor-associated macrophages in colorectal cancer: Impact on single cell invasion (tumor budding) and clinicopathological outcome. Oncoimmunology (2015) 5(4):e1106677. doi: 10.1080/2162402X.2015.1106677
282. Itatani Y, Kawada K, Fujishita T, Kakizaki F, Hirai H, Matsumoto T, et al. Loss of SMAD4 from colorectal cancer cells promotes CCL15 expression to recruit CCR1+ myeloid cells and facilitate liver metastasis. Gastroenterology (2013) 145(5):1064–75. doi: 10.1053/j.gastro.2013.07.033
283. Mantovani A, Marchesi F, Malesci A, Laghi L, Allavena P. Tumour-associated macrophages as treatment targets in oncology. Nat Rev Clin Oncol (2017) 14(7):399–416. doi: 10.1038/nrclinonc.2016.217
284. Zhang S, Zhong M, Wang C, Xu Y, Gao WQ, Zhang Y. CCL5-deficiency enhances intratumoral infiltration of CD8+ T cells in colorectal cancer. Cell Death Dis (2018) 9(7):766. doi: 10.1038/s41419-018-0796-2
285. Heidrich I, Ackar L, Mohammadi PM, Pantel K. Liquid biopsies: potential and challenges. Int J Cancer (2021) 148:528–45. doi: 10.1002/ijc.33217
286. Kilgour E, Rothwell DG, Brady G, Dive C. Liquid biopsy-based biomarkers of treatment response and resistance. Cancer Cell (2020) 37:485–95. doi: 10.1016/j.ccell.2020.03.012
287. Biswas D, Ganeshalingam J, Wan JCM. The future of liquid biopsy. Lancet Oncol (2020) 21:e550. doi: 10.1016/S1470-2045(20)30687-2
Keywords: liquid biopsy, neoantigen, immune cells, exosomes, colorectal cancer, mitochondrial DNA, circulating cancer associated fibroblasts (cCAFs)
Citation: Mirza S, Bhadresha K, Mughal MJ, McCabe M, Shahbazi R, Ruff P and Penny C (2023) Liquid biopsy approaches and immunotherapy in colorectal cancer for precision medicine: Are we there yet? Front. Oncol. 12:1023565. doi: 10.3389/fonc.2022.1023565
Received: 19 August 2022; Accepted: 08 December 2022;
Published: 06 January 2023.
Edited by:
Francesca Negri, University Hospital of Parma, ItalyReviewed by:
Shuiling Jin, First Affiliated Hospital of Zhengzhou University, ChinaZhigang Bai, Beijing Friendship Hospital, China
Copyright © 2023 Mirza, Bhadresha, Mughal, McCabe, Shahbazi, Ruff and Penny. This is an open-access article distributed under the terms of the Creative Commons Attribution License (CC BY). The use, distribution or reproduction in other forums is permitted, provided the original author(s) and the copyright owner(s) are credited and that the original publication in this journal is cited, in accordance with accepted academic practice. No use, distribution or reproduction is permitted which does not comply with these terms.
*Correspondence: Clement Penny, Clement.penny@wits.ac.za
†These authors have contributed equally to this work