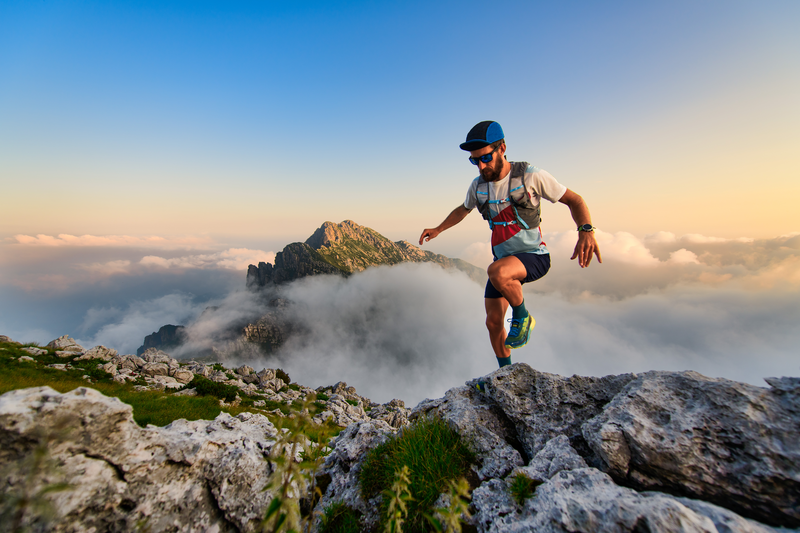
94% of researchers rate our articles as excellent or good
Learn more about the work of our research integrity team to safeguard the quality of each article we publish.
Find out more
MINI REVIEW article
Front. Oncol. , 25 November 2022
Sec. Cancer Genetics
Volume 12 - 2022 | https://doi.org/10.3389/fonc.2022.1019750
Alternative RNA splicing (ARS) is an essential and tightly regulated cellular process of post-transcriptional regulation of pre-mRNA. It produces multiple isoforms and may encode proteins with different or even opposite functions. The dysregulated ARS of pre-mRNA contributes to the development of many cancer types, including oral squamous cell carcinoma (OSCC), and may serve as a biomarker for the diagnosis and prognosis of OSCC and an attractive therapeutic target. ARS is mainly regulated by splicing factors, whose expression is also often dysregulated in OSCC and involved in tumorigenesis. This review focuses on the expression and roles of splicing factors in OSCC, the alternative RNA splicing events associated with OSCC, and recent advances in therapeutic approaches that target ARS.
Oral squamous cell carcinoma (OSCC) is among the most malignant cancer types worldwide (1), and its prognosis remains poor despite improvements in therapies in recent decades. Therefore, the molecular pathogenesis of the development of OSCC needs to be urgently determined. The dysregulated alternative RNA splicing (ARS) of pre-mRNA contributes to the development of many cancer types, including OSCC (2, 3). The alternative splicing of pre-mRNA in eukaryotes was first discovered in 1980 (4, 5), by which exons can be jointed in different ways to produce multiple mRNA isoforms, and then possibly encode different protein isoforms (6). As a complex biological process, ARS is tightly regulated via interactions between cis-elements and trans-acting splicing factors. The cis-elements include exon splice enhancer, exon splice silencer, intron splice enhancer, and intron splice silencer, while trans-elements include trans-acting splicing factors (7). The common types of alternative RNA splicing include exon skipping, mutually exclusive exons, alternative 5’ splice site, alternative 3’ splice site, and intron retention (Figure 1A). ARS occurs in more than 90% human genes and is precisely regulated in cells. This review focuses on the expression and roles of splicing factors in OSCC, the ARS events associated with OSCC, and recent advances in therapeutic approaches that target ARS.
Figure 1 The common types of alternative RNA splicing and the SF3b complex involved in alternative RNA splicing. (A) The different types of alternative RNA splicing and transcript variants (exon skipping, intron retention, mutually exclusive exons, alternative 5’ splice site, and alternative 3’ splice site). (B) Diagram of the components of spliceosome complex, especially SF3b complex, and SF3b1-specific inhibitors.
The ARS patterns of pre-mRNA are often altered in OSCC, mainly because of the dysregulated expression of splicing factors. Moreover, abnormal alternative RNA splicing can promote the development of OSCC by enhancing cancer cell proliferation, invasion, and metastasis.
The process of pre-mRNA alternative splicing is catalyzed by the spliceosomes, which are divided into two categories, namely, major spliceosomes (U1, U2, U4, U5, and U6 small nuclear ribonucleoproteins, snRNPs) and minor spliceosomes (U11, U12, U4atac, and U6atac snRNPs) (8) (Figure 1B). Spliceosome is a megadalton complex. The SF3b protein subcomplex is a component of the U2 snRNP and plays key roles in recognizing the branch point sequence during spliceosome assembly (9). SF3B1 is the largest protein in the SF3b complex and has been regarded as a therapeutic target because of its frequent mutations in both hematologic cancers and solid tumors (10–13). The high expression level of SF3B1 in hepatocellular carcinoma (HCC) has been correlated with increased tumor aggressiveness and shorter overall survival (OS) (14). SF3B1 is also upregulated in OSCC tissues (15).
The ARS of pre-mRNA is mainly regulated by splicing factors. Splicing factors include serine/arginine-rich protein (SR) family and heterogeneous nuclear ribonucleoproteins (hnRNPs) family, and other splicing factors.
SR proteins are an evolutionarily conserved family and consist of at least 12 members (SRSF1 to SRSF12). SR proteins contain N-terminal RNA recognition motifs and C-terminal serine and arginine-rich (RS) domains. SR proteins play important roles in the regulation of pre-mRNA splicing and ARS (16). Several SR family members participate in the tumorigenesis of OSCC.
SRSF1 is a well-known oncogenic splicing factor (17). SRSF1 is also overexpressed in OSCC and promotes cell proliferation, invasion, and epithelial-mesenchymal transition (EMT) by interacting with lncRNA LINC01296 (18).
Oncogene SRSF3 is overexpressed in many cancers, including OSCC (19–21). In OSCC, SRSF3 overexpression is positively associated with high-grade tumors and lymph node metastasis, indicating that SRSF3 may promote the development of OSCC. Mechanically, SRSF3 is required for the expression of Slug and N-cadherin genes (19), which can promote EMT and metastasis. Moreover, SRSF3 can inhibit autophagy by repressing the expression of p65 and FOXO1, and their downstream target, BECN1, which is a key factor of autophagy (22). In general, autophagy plays a tumor-suppressive function in the early stage of oncogenesis (23). SRSF3 may enhance the early development of OSCC by inhibiting autophagy. Importantly, SRSF3 has an autoregulatory mechanism to maintain its stable expression level in cells. SRSF3 exon 4 is an alternative exon with an in-frame pre-mature stop codon. SRSF3 inhibits its own expression by increasing exon 4 inclusion. In cancer cells, oncogenic PTBP1 and PTBP2 proteins impair SRSF3 autoregulation by repressing SRSF3 exon 4 inclusion and significantly enhancing SRSF3 expression (21).
SRSF5 is also overexpressed in OSCC and is essential for cell proliferation and tumor development. The downregulation of SRSF5 expression significantly inhibited cell growth and cell cycle progression, and tumor formation in vivo. Interestingly, SRSF5 also has an autoregulatory mechanism mediated by the selection of two alternative 3’ splice sites in exon 6. The usage of proximal 3’ splice site includes a pre-mature stop codon. SRSF3 inhibits the usage of proximal 3’ splice site and the autoregulation, thus remarkably enhancing SRSF5 expression in cancer cells (24).
Heterogeneous nuclear ribonucleoproteins (hnRNPs) represent a large family with multiple functions in regulating RNA metabolism, including ARS. The proteins in this family are named from A1 to U (25). Many hnRNP proteins are associated with tumorigenesis, and some of them have been demonstrated to be oncogenes (26). Some hnRNP proteins play oncogenic roles, while others play tumor-suppressive roles in OSCC.
HnRNP A1 is overexpressed in OSCC tissues and required for OSCC cell proliferation. HnRNP A1 is predominantly highly expressed in the G2/M cell cycle phase. A1 knockdown leads to cell cycle G2/M arrest in OSCC cells. In principle, A1 promotes alternative exon 5 inclusion of cyclin-dependent kinase 2 (CDK2) and produces full-length CDK2 protein, which participates in cell cycle regulation (27).
HnRNP C is also overexpressed in OSCC tissues. The high expression of hnRNP C is associated with advanced tumor stage, lymph node metastasis, and poor prognosis in patients with OSCC. HnRNP C promotes OSCC cell proliferation, migration, and invasion (28). In addition, hnRNP C can enhance the radioresistance of OSCC cells by interacting with lncRNA LINC00662 to stabilize adenylate kinase 4 (AK4) mRNA and increase the expression of AK4 protein, which is a key enzyme in cancer cell resistance to radiation (29).
The nuclear expression of hnRNP D is remarkably higher in oral dysplasia and OSCC tissues than in normal tissues and is positively correlated with OSCC tumor size, tumor stage, and poor patient survival (30). The overexpression of hnRNP D is mediated by NF-κB (RelA) binding to its promoter. HnRNP D expression is strongly correlated with NF-κB in OSCC (31).
HnRNP K mainly plays oncogenic roles in cancer (32). In comparison with normal oral mucosal tissues, hnRNP K is overexpressed in OSCC tissues (33). HnRNP K protein can be detected in both cytoplasm and nucleus. Both cytoplasm and nucleus hnRNP K expression gradually increases from normal tissue to OSCC, and is associated with poor OSCC prognosis (33, 34).
HnRNP L is overexpressed in OSCC tissues and required for OSCC cell growth, migration, and tumorigenesis (35). HnRNP L expression is also controlled by an autoregulatory mechanism. HnRNP L promotes the inclusion of its alternative exon 7, which includes a pre-mature stop codon. Only the transcripts without exon 7 can encode the full-length oncogenic hnRNP L protein. In OSCC cells, increased SRSF3 promotes exon 7 skipping and the expression of full-length hnRNP L protein, and then enhances tumorigenesis (36).
Most splicing factors are overexpressed and play oncogenic roles in OSCC. However, hnRNP E1, which is also called PCBP1, mainly functions as a tumor suppressor (37). HnRNP E1 overexpression significantly inhibited OSCC cell proliferation and tumor formation by inhibiting the usage of the proximal 5’ splice site of STAT3 exon 23 and the expression of oncogenic STAT3α isoform (38). Importantly, two leucine residues (Leu100 and Leu102) of PCBP1 protein are frequently mutated in cancers and are essential for PCBP1 function (39).
HnRNP G, which is also known as RBMX, is differentially expressed in various cancer types. For example, hepatocellular carcinoma has a high level of hnRNP G (40), whereas bladder cancer shows downregulated hnRNP G expression (41). In dysplastic or OSCC tissues, the expression levels of hnRNP G protein are remarkably lower than those in normal oral mucosal tissues. The ectopic expression of hnRNP G remarkably abolished the clonogenic capacity of OSCC cells in soft agar in vitro, suggesting that hnRNP G may be a tumor suppressor in OSCC (42). Interestingly, in the patients of TCGA head and neck squamous cell carcinoma (HNSCC), the lower transcriptional level of hnRNP G is significantly associated with favorable overall survival (43). Therefore, further study is required for the complete understanding of the expression and clinical effects of hnRNP G in OSCC.
In addition to SR and hnRNP protein families, some other splicing factors are also associated with OSCC.
SF3B1 is a component of SF3b complex and is involved in the recognition of branch point during spliceosome assembly. SF3B1 expression is significantly upregulated in OSCC tissues compared with normal adjacent tissues (15). Interestingly, SF3B1 is also identified in the cultured medium based on a secretome analysis of HNSCC cell lines (15). Moreover, SF3B3, another component of SF3b complex, is also significantly upregulated in OSCC (15) and may be mutated in some cases (44). Further study is needed for the complete understanding of roles of SF3B1 and SF3B3 in OSCC.
LSM12 is a member of Sm-like proteins and regulates pre-mRNA splicing. LSM12 is significantly upregulated in OSCC tissues of patients and animal models. LSM12 is required for OSCC cell proliferation, migration, and tumorigenesis (45).
Epithelial splicing regulatory protein 1 and 2 (ESRP1 and ESRP2), which are also known as RBM35A and RBM35B, were upregulated in OSCC compared with normal epithelium. However, they were downregulated in the invasion front. ESRP1 and ESRP2 do not affect cell proliferation but inhibit cancer cell migration (46). Mechanically, ESRP1 knockdown significantly increased RAC1 exon 3b inclusion and the expression level of RAC1b isoform, which can modulate actin and enhance cell migration (46).
Therefore, splicing factors play significant roles in OSCC progression. However, the underlying mechanisms in which splicing factors are mediated need to be further investigated. Table S1 summarizes the information related to splicing factors in OSCC.
In addition to splicing factors, many alternative RNA splicing events of cancer-related genes contribute to OSCC progression (Figure 2; Table S2).
Figure 2 Alternative RNA splicing and splicing factors regulate OSCC cell proliferation, invasion, and EMT.
Myeloid cell leukemia-1 (MCL1) belongs to the Bcl-2 family and is an anti-apoptotic regulator essential for cell proliferation. MCL1 has three splicing variants, including MCL-1L, MCL-1S, and MCL-1ES (47). MCL-1L contains all exons, whereas MCL-1S is a short isoform without exon 2 and encodes a pro-apoptotic protein. MCL-1ES is another short isoform with a truncated exon 1 and encodes a pro-apoptotic protein, which is expressed at a very low level in OSCC (48). OSCC tissues expressed significantly higher MCL-1L level than normal adjacent control tissues (49). The downregulation of MCL-1L could enhance the radio sensitivity of OSCC cells (48). High MCL-1L expression is positively associated with lymph node positivity, tumor size, and poor overall survival in patients with OSCC. MCL-1L is an independent prognostic factor for OSCC (50). Conversely, MCL-1S expression is much weaker than MCL-1L in OSCC cells and tissues. The ratio of MCL-1L/S was positively correlated with poor overall survival (50). Mechanically, the MCL-1S protein can dimerize with MCL-1L and neutralize its anti-apoptotic function (51).
Survivin is an anti-apoptosis protein and is encoded by BIRC5 (baculoviral inhibitor of apoptosis protein repeat-containing 5) gene. Six splicing variants have been reported, including survivin-wt, survivin-2B, survivin-ΔEx3, survivin-3B, survivin-2α, and survivin-3α. Survivin-wt is a conventional variant composed of exons 1 to 4. Survivin-2B contains part of the intron 2 sequence and encodes a bigger protein with markedly reduced anti-apoptosis capacity. Survivin-ΔEx3 is a short isoform caused by exon 3 skipping, but it shows anti-apoptosis capacity (52). Survivin-3B (53) and survivin-2α (54) comprise extra sequences from intron 2 and intron 3, respectively. Survivin-3B is anti-apoptotic, while survivin-2α is pro-apoptotic. In OSCC cells and tissues, survivin-wt, survivin-ΔEx3, and survivin-2B are the dominantly overexpressed isoforms (55, 56). The expression level of survivin-3B in OSCC is positively associated with poor differentiation and lymph node metastases (55, 56). However, pro-apoptotic survivin-2α and survivin-3α were also highly expressed in OSCC tissues (55). The roles and regulatory mechanisms of survivin isoforms in OSCC remain to be further explored.
Transcription factor STAT3 plays important roles in the initiation and development of tumors. STAT3 has two isoforms generated by the ARS of exon 23. STAT3α is the longer isoform and encodes the full-length oncogenic STAT3α protein. STAT3β is shorter and encodes the truncated and tumor-suppressive STAT3β protein. Splicing factor hnRNP E1 (PCBP1) can bind to an exonic splicing suppressor (ESS) in STAT3 exon 23 and repress the expression of STAT3α in OSCC cells (38, 39).
USO1 (vesicle transport factor), which is also known as P115/TAP, is a member of the tether protein family, and it plays oncogenic roles in multiple myeloma and colon cancer (57, 58). USO1 exon 15 is an alternative exon. Full-length USO1 isoform was positively associated with OSCC cell proliferation and enhanced tumorigenic capacity, while USO1 short isoform without exon 15 showed the opposite effects on OSCC cell proliferation and cell cycle progression. LSM12 promotes USO1 exon 15 inclusion (45).
H19 is an lncRNA that can function as an oncogene in OSCC and is required for OSCC cell proliferation, invasion, and migration (59). Interestingly, H19 exon 4 is an alternative exon. H19-S, a short transcript isoform of H19 lncRNA that lacks exon 4, was able to transform normal oral mucosal cells and promote OSCC cell growth. H19-S could also induce EMT and promote oral carcinogenesis by binding and stabilizing zinc-finger E-box binding homeobox 1 (ZEB1) mRNA and increasing ZEB1 expression compared with the full-length transcript H19-L (60).
P63 is a tumor suppressor gene and bears strong homology to p53 (61). However, the exact function of p63 in OSCC is quite complicated because of the multiple isoforms of p63 (62). Two promoters of p63 drive the expression of two types of isoforms, namely, TAp63 and ΔNp63 (63, 64). Each of these isoforms has at least three variants (α/β/γ), which encode proteins with different COOH-termini generated by ARS. TAp63 isoforms are generally tumor suppressors. ΔNp63α is the predominant splicing isoform expressed in OSCC cells and tissues. In OSCC cells, Snail repressed ΔNp63α expression by inhibiting the binding of C/EBP to the promoter of ΔNp63α, and then increased cellular epithelial-mesenchymal transition and invasive capacity (65). ΔNp63β overexpression reversed stromal cell-like to epithelial cells in OSCC (66). The stable overexpression of ΔNp63β inhibited OSCC cell invasion and metastasis through the downregulation of Wnt5A, Ror2, and MMP-2 (66, 67). ΔNp63β can upregulate miR-205, which downregulates zinc-finger E-box binding homeobox (ZEB) 1 and ZEB2 and then inhibits EMT (68). However, ΔNp63γ promotes EMT (69). Two additional isoforms without exon 4, Δ4Tap63 and ΔNp73L, were also reported. These two isoforms are more likely to occur in patients with metastases (63), but their function and regulatory mechanisms remain unclear.
Oral cancer overexpressed 1 (ORAOV1) is an oncogene that is required for OSCC cell proliferation, tumor growth, and tumor angiogenesis (70). ORAOV1-A, a splice variant of ORAOV1 without exon 3, encodes a truncated protein because of a pre-mature stop codon in exon 4. ORAOV1-A expression is associated with poor differentiation in OSCC (71). ORAOV1-B, another splice variant of ORAOV1 without exon 2 and exon 3, was validated as an lncRNA. ORAOV1-B is overexpressed in OSCC and positively related with invasion and metastasis. ORAOV1-B can enhance EMT by activating the NF-κB pathway (72). All ORAOV1 isoforms play positive roles in OSCC progression.
Some antisense oligonucleotides (ASOs) and small molecules have been developed to control alternative RNA splicing in OSCC. ASOs are usually used to target specific sequences in mRNA, whereas small molecules often target splicing factor proteins.
ASOs have been used for the treatment of diseases caused by abnormal alternative RNA splicing, such as spinal muscular atrophy (SMA) (73) and Duchenne muscular dystrophy (DMD) (74). ASOs can bind to the key regulatory elements of target RNA and control ARS. In our previous study, an ASO that targeted the exonic splicing suppressor (ESS) motif of splicing factor SRSF3 pre-mRNA promoted the SRSF3 exon 4 inclusion and reduced the expression level of full-length SRSR3 (75). OSCC cells treated with anti-SRSF3 ASO grew significantly slower compared with those treated with non-specific ASO. Furthermore, anti-SRSF3 ASO treatment significantly sensitized OSCC cells to the chemotherapy drug paclitaxel, thus improving the therapeutic effects of paclitaxel (76).
Small molecules are usually used to inhibit splicing factors. For example, a novel SRSF3 inhibitor SFI003 was developed by screening compounds based on the structure of SRSF3 protein and chemical optimization. SFI003 binds to SRSF3 protein and leads to its degradation. SFI003 induced apoptosis and exhibited potent antitumor efficacy in colorectal cancer cells (77).
The SF3b spliceosome complex is involved in RNA splice site selection, and SF3B1 is a core component of SF3b complex. Pladienolide B, FD-895, GEX1A, and sudemycin E (an analog of FR901464) are inhibitors of SF3B1, and these molecules interact with SF3B1 demonstrating significant induction of cell apoptosis in cancers (78–80). Besides, small-molecule inhibitors of SF3B1 altered MCL1 splicing, thus promoting the generation of the pro-apoptotic variant MCL1-S and diminishing the anti-apoptotic variant MCL1-L, such as spliceostatin A (a methyl ketal derivative of FR901464) in chronic lymphocytic leukemia cells (81), meayamycin B (an analog of FR901464) in non-small cell lung cancer cells (82), and head and neck cancer cells (83). The splice modulators used in different types of tumor cells are summarized in Table S3.
In conclusion, the dysregulation of ARS occurs universally in OSCC, and may serve as biological markers for the diagnosis and prognosis of OSCC. Oncogenic isoforms produced by ARS can promote the progression of OSCC. Therefore, correcting aberrant alternative RNA splicing and decreasing the expression levels of oncogenic isoforms may contribute to OSCC treatment. Targeting the overexpressed oncogenic splicing factors may also provide novel treatment opportunities in OSCC. However, the regulatory mechanisms of aberrant ARS in OSCC remain largely unclear, thus limiting the development of efficient treatment methods. The regulatory elements of ARS events and corresponding splicing factors need to be explored. Recently, neoantigens produced by ARS have attracted great attention in cancer immunotherapy (84). The modification of RNA splicing can produce bona fide neoantigens and induce anti-cancer immunity (85), which sheds light on immunotherapy for OSCC.
ML wrote the manuscript. JG and RJ edited the manuscript. All authors contributed to the article and approved the submitted version.
This work was supported by the Key Research and Development Program of Hubei Province (grant no. 2020BCB046).
The authors declare that the research was conducted in the absence of any commercial or financial relationships that could be construed as a potential conflict of interest.
All claims expressed in this article are solely those of the authors and do not necessarily represent those of their affiliated organizations, or those of the publisher, the editors and the reviewers. Any product that may be evaluated in this article, or claim that may be made by its manufacturer, is not guaranteed or endorsed by the publisher.
The Supplementary Material for this article can be found online at: https://www.frontiersin.org/articles/10.3389/fonc.2022.1019750/full#supplementary-material
1. Bray F, Ferlay J, Soerjomataram I, Siegel RL, Torre LA, Jemal A. Global cancer statistics 2018: Globocan estimates of incidence and mortality worldwide for 36 cancers in 185 countries. CA Cancer J Clin (2018) 68(6):394–424. doi: 10.3322/caac.21492
2. Zhang S, Wu X, Diao P, Wang C, Wang D, Li S, et al. Identification of a prognostic alternative splicing signature in oral squamous cell carcinoma. J Cell Physiol (2020) 235(5):4804–13. doi: 10.1002/jcp.29357
3. Bonnal SC, Lopez-Oreja I, Valcarcel J. Roles and mechanisms of alternative splicing in cancer - implications for care. Nat Rev Clin Oncol (2020) 17(8):457–74. doi: 10.1038/s41571-020-0350-x
4. Alt FW, Bothwell AL, Knapp M, Siden E, Mather E, Koshland M, et al. Synthesis of secreted and membrane-bound immunoglobulin mu heavy chains is directed by mrnas that differ at their 3' ends. Cell (1980) 20(2):293–301. doi: 10.1016/0092-8674(80)90615-7
5. Early P, Rogers J, Davis M, Calame K, Bond M, Wall R, et al. Two mrnas can be produced from a single immunoglobulin mu gene by alternative rna processing pathways. Cell (1980) 20(2):313–9. doi: 10.1016/0092-8674(80)90617-0
6. Black DL. Mechanisms of alternative pre-messenger rna splicing. Annu Rev Biochem (2003) 72:291–336. doi: 10.1146/annurev.biochem.72.121801.161720
7. Mao M, Hu Y, Yang Y, Qian Y, Wei H, Fan W, et al. Modeling and predicting the activities of trans-acting splicing factors with machine learning. Cell Syst (2018) 7(5):510–20.e4. doi: 10.1016/j.cels.2018.09.002
8. Patel AA, Steitz JA. Splicing double: Insights from the second spliceosome. Nat Rev Mol Cell Biol (2003) 4(12):960–70. doi: 10.1038/nrm1259
9. Sun C. The Sf3b complex: Splicing and beyond. Cell Mol Life Sci (2020) 77(18):3583–95. doi: 10.1007/s00018-020-03493-z
10. Yoshida K, Ogawa S. Splicing factor mutations and cancer. Wiley Interdiscip Rev RNA (2014) 5(4):445–59. doi: 10.1002/wrna.1222
11. Bejar R. Splicing factor mutations in cancer. Adv Exp Med Biol (2016) 907:215–28. doi: 10.1007/978-3-319-29073-7_9
12. Agrawal AA, Yu L, Smith PG, Buonamici S. Targeting splicing abnormalities in cancer. Curr Opin Genet Dev (2018) 48:67–74. doi: 10.1016/j.gde.2017.10.010
13. Tripathi G, Tripathi A, Johnson J, Kashyap MK. Role of rna splicing in regulation of cancer stem cell. Curr Stem Cell Res Ther (2021). doi: 10.2174/1574888X16666211207103628
14. Kashyap A, Tripathi G, Tripathi A, Rao R, Kashyap M, Bhat A, et al. Rna splicing: A dual-edged sword for hepatocellular carcinoma. Med Oncol (2022) 39(11):173. doi: 10.1007/s12032-022-01726-8
15. Mumtaz M, Bijnsdorp IV, Bottger F, Piersma SR, Pham TV, Mumtaz S, et al. Secreted protein markers in oral squamous cell carcinoma (Oscc). Clin Proteomics (2022) 19(1):4. doi: 10.1186/s12014-022-09341-5
16. Tacke R, Manley JL. Determinants of Sr protein specificity. Curr Opin Cell Biol (1999) 11(3):358–62. doi: 10.1016/S0955-0674(99)80050-7
17. Karni R, de Stanchina E, Lowe SW, Sinha R, Mu D, Krainer AR. The gene encoding the splicing factor Sf2/Asf is a proto-oncogene. Nat Struct Mol Biol (2007) 14(3):185–93. doi: 10.1038/nsmb1209
18. Zhang Y, Wang A, Zhang X, Wang X, Zhang J, Ma J. Lncrna Linc01296 promotes oral squamous cell carcinoma development by binding with Srsf1. BioMed Res Int (2021) 2021:6661520. doi: 10.1155/2021/6661520
19. Peiqi L, Zhaozhong G, Yaotian Y, Jun J, Jihua G, Rong J. Expression of Srsf3 is correlated with carcinogenesis and progression of oral squamous cell carcinoma. Int J Med Sci (2016) 13(7):533–9. doi: 10.7150/ijms.14871
20. Jia R, Li C, McCoy JP, Deng CX, Zheng ZM. Srp20 is a proto-oncogene critical for cell proliferation and tumor induction and maintenance. Int J Biol Sci (2010) 6(7):806–26. doi: 10.7150/ijbs.6.806
21. Guo J, Jia J, Jia R. Ptbp1 and Ptbp2 impaired autoregulation of Srsf3 in cancer cells. Sci Rep (2015) 5:14548. doi: 10.1038/srep14548
22. Zhou L, Guo J, Jia R. Oncogene Srsf3 suppresses autophagy Via inhibiting Becn1 expression. Biochem Biophys Res Commun (2019) 509(4):966–72. doi: 10.1016/j.bbrc.2019.01.048
23. Kimmelman AC, White E. Autophagy and tumor metabolism. Cell Metab (2017) 25(5):1037–43. doi: 10.1016/j.cmet.2017.04.004
24. Yang S, Jia R, Bian Z. Srsf5 functions as a novel oncogenic splicing factor and is upregulated by oncogene Srsf3 in oral squamous cell carcinoma. Biochim Biophys Acta Mol Cell Res (2018) 1865(9):1161–72. doi: 10.1016/j.bbamcr.2018.05.017
25. Pinol-Roma S, Choi YD, Matunis MJ, Dreyfuss G. Immunopurification of heterogeneous nuclear ribonucleoprotein particles reveals an assortment of rna-binding proteins. Genes Dev (1988) 2(2):215–27. doi: 10.1101/gad.2.2.215
26. Geuens T, Bouhy D, Timmerman V. The hnrnp family: Insights into their role in health and disease. Hum Genet (2016) 135(8):851–67. doi: 10.1007/s00439-016-1683-5
27. Yu C, Guo J, Liu Y, Jia J, Jia R, Fan M. Oral squamous cancer cell exploits hnrnp A1 to regulate cell cycle and proliferation. J Cell Physiol (2015) 230(9):2252–61. doi: 10.1002/jcp.24956
28. Huang GZ, Wu QQ, Zheng ZN, Shao TR, Chen YC, Zeng WS, et al. M6a-related bioinformatics analysis reveals that hnrnpc facilitates progression of oscc Via emt. Aging (2020) 12(12):11667–84. doi: 10.18632/aging.103333
29. Chen Y, Bao C, Zhang X, Lin X, Fu Y. Knockdown of Linc00662 represses Ak4 and attenuates radioresistance of oral squamous cell carcinoma. Cancer Cell Int (2020) 20:244. doi: 10.1186/s12935-020-01286-9
30. Kumar M, Matta A, Masui O, Srivastava G, Kaur J, Thakar A, et al. Nuclear heterogeneous nuclear ribonucleoprotein d is associated with poor prognosis and interactome analysis reveals its novel binding partners in oral cancer. J Transl Med (2015) 13:285. doi: 10.1186/s12967-015-0637-3
31. Kumar V, Kumar A, Kumar M, Lone MR, Mishra D, Chauhan SS. Nfkappab (Rela) mediates transactivation of hnrnpd in oral cancer cells. Sci Rep (2022) 12(1):5944. doi: 10.1038/s41598-022-09963-7
32. Barboro P, Ferrari N, Balbi C. Emerging roles of heterogeneous nuclear ribonucleoprotein K (Hnrnp K) in cancer progression. Cancer Lett (2014) 352(2):152–9. doi: 10.1016/j.canlet.2014.06.019
33. Wu CS, Chang KP, Chen LC, Chen CC, Liang Y, Hseuh C, et al. Heterogeneous ribonucleoprotein K and thymidine phosphorylase are independent prognostic and therapeutic markers for oral squamous cell carcinoma. Oral Oncol (2012) 48(6):516–22. doi: 10.1016/j.oraloncology.2012.01.005
34. Matta A, Tripathi SC, DeSouza LV, Grigull J, Kaur J, Chauhan SS, et al. Heterogeneous ribonucleoprotein K is a marker of oral leukoplakia and correlates with poor prognosis of squamous cell carcinoma. Int J Cancer (2009) 125(6):1398–406. doi: 10.1002/ijc.24517
35. Jia R, Zhang S, Liu M, Zhang Y, Liu Y, Fan M, et al. Hnrnp l is important for the expression of oncogene Srsf3 and oncogenic potential of oral squamous cell carcinoma cells. Sci Rep (2016) 6:35976. doi: 10.1038/srep35976
36. Xu L, Shen J, Jia J, Jia R. Inclusion of hnrnp l alternative exon 7 is associated with good prognosis and inhibited by oncogene Srsf3 in head and neck squamous cell carcinoma. BioMed Res Int (2019) 2019:9612425. doi: 10.1155/2019/9612425
37. Guo J, Jia R. Splicing factor Poly(Rc)-binding protein 1 is a novel and distinctive tumor suppressor. J Cell Physiol (2018) 234(1):33–41. doi: 10.1002/jcp.26873
38. Wang X, Guo J, Che X, Jia R. Pcbp1 inhibits the expression of oncogenic Stat3 isoform by targeting alternative splicing of Stat3 exon 23. Int J Biol Sci (2019) 15(6):1177–86. doi: 10.7150/ijbs.33103
39. Li Z, Wang X, Jia R. Poly(Rc) binding protein 1 represses the translation of Stat3 through 5' utr. Curr Gene Ther (2022) 22(5):397–405. doi: 10.2174/1566523222666220511162934
40. Song Y, He S, Ma X, Zhang M, Zhuang J, Wang G, et al. Rbmx contributes to hepatocellular carcinoma progression and sorafenib resistance by specifically binding and stabilizing Blacat1. Am J Cancer Res (2020) 10(11):3644–65.
41. Yan Q, Zeng P, Zhou X, Zhao X, Chen R, Qiao J, et al. Rbmx suppresses tumorigenicity and progression of bladder cancer by interacting with the hnrnp A1 protein to regulate pkm alternative splicing. Oncogene (2021) 40(15):2635–50. doi: 10.1038/s41388-021-01666-z
42. Shin KH, Kang MK, Kim RH, Christensen R, Park NH. Heterogeneous nuclear ribonucleoprotein G shows tumor suppressive effect against oral squamous cell carcinoma cells. Clin Cancer Res (2006) 12(10):3222–8. doi: 10.1158/1078-0432.Ccr-05-2656
43. Guo J, Wang X, Jia J, Jia R. Underexpression of Srsf3 and its target gene rbmx predicts good prognosis in patients with head and neck cancer. J Oral Sci (2020) 62(2):175–9. doi: 10.2334/josnusd.18-0485
44. Chang CC, Chang YS, Chan WL, Yeh KT, Wei RJ, Chang JG. Detection of Sf3b3 gene mutations in oral cancer by high resolution melting analysis. Clin Lab (2014) 60(12):2023–9. doi: 10.7754/clin.lab.2014.140409
45. Dong Y, Xue L, Zhang Y, Liu C, Zhang Y, Jiang N, et al. Identification of rna-splicing factor Lsm12 as a novel tumor-associated gene and a potent biomarker in oral squamous cell carcinoma (Oscc). J Exp Clin Cancer Res (2022) 41(1):150. doi: 10.1186/s13046-022-02355-9
46. Ishii H, Saitoh M, Sakamoto K, Kondo T, Katoh R, Tanaka S, et al. Epithelial splicing regulatory proteins 1 (Esrp1) and 2 (Esrp2) suppress cancer cell motility Via different mechanisms. J Biol Chem (2014) 289(40):27386–99. doi: 10.1074/jbc.M114.589432
47. Kim JH, Sim SH, Ha HJ, Ko JJ, Lee K, Bae J. Mcl-1es, a novel variant of mcl-1, associates with mcl-1l and induces mitochondrial cell death. FEBS Lett (2009) 583(17):2758–64. doi: 10.1016/j.febslet.2009.08.006
48. Palve VC, Teni TR. Association of anti-apoptotic mcl-1l isoform expression with radioresistance of oral squamous carcinoma cells. Radiat Oncol (2012) 7:135. doi: 10.1186/1748-717X-7-135
49. Mallick S, Patil R, Gyanchandani R, Pawar S, Palve V, Kannan S, et al. Human oral cancers have altered expression of bcl-2 family members and increased expression of the anti-apoptotic splice variant of mcl-1. J Pathol (2009) 217(3):398–407. doi: 10.1002/path.2459
50. Palve V, Mallick S, Ghaisas G, Kannan S, Teni T. Overexpression of mcl-1l splice variant is associated with poor prognosis and chemoresistance in oral cancers. PloS One (2014) 9(11):e111927. doi: 10.1371/journal.pone.0111927
51. Bae J, Leo CP, Hsu SY, Hsueh AJ. Mcl-1s, a splicing variant of the antiapoptotic bcl-2 family member mcl-1, encodes a proapoptotic protein possessing only the Bh3 domain. J Biol Chem (2000) 275(33):25255–61. doi: 10.1074/jbc.M909826199
52. Mahotka C, Wenzel M, Springer E, Gabbert HE, Gerharz CD. Survivin-Deltaex3 and survivin-2b: Two novel splice variants of the apoptosis inhibitor survivin with different antiapoptotic properties. Cancer Res (1999) 59(24):6097–102.
53. Badran A, Yoshida A, Ishikawa K, Goi T, Yamaguchi A, Ueda T, et al. Identification of a novel splice variant of the human anti-apoptopsis gene survivin. Biochem Biophys Res Commun (2004) 314(3):902–7. doi: 10.1016/j.bbrc.2003.12.178
54. Caldas H, Honsey LE, Altura RA. Survivin 2alpha: A novel survivin splice variant expressed in human malignancies. Mol Cancer (2005) 4(1):11. doi: 10.1186/1476-4598-4-11
55. Mishra R, Palve V, Kannan S, Pawar S, Teni T. High expression of survivin and its splice variants survivin Deltaex3 and survivin 2 b in oral cancers. Oral Surg Oral Med Oral Pathol Oral Radiol (2015) 120(4):497–507. doi: 10.1016/j.oooo.2015.06.027
56. De Maria S, Pannone G, Bufo P, Santoro A, Serpico R, Metafora S, et al. Survivin gene-expression and splicing isoforms in oral squamous cell carcinoma. J Cancer Res Clin Oncol (2009) 135(1):107–16. doi: 10.1007/s00432-008-0433-z
57. Jin Y, Dai Z. Uso1 promotes tumor progression Via activating erk pathway in multiple myeloma cells. BioMed Pharmacother (2016) 78:264–71. doi: 10.1016/j.biopha.2016.01.012
58. Sui J, Li X, Xing J, Cao F, Wang H, Gong H, et al. Lentivirus-mediated silencing of Uso1 inhibits cell proliferation and migration of human colon cancer cells. Med Oncol (2015) 32(8):218. doi: 10.1007/s12032-015-0658-z
59. Zhang DM, Lin ZY, Yang ZH, Wang YY, Wan D, Zhong JL, et al. Incrna H19 promotes tongue squamous cell carcinoma progression through beta-Catenin/Gsk3beta/Emt signaling Via association with Ezh2. Am J Transl Res (2017) 9(7):3474–86.
60. Zhou W, Wang XZ, Fang BM. A variant of H19 transcript regulates emt and oral cancer progression. Oral Dis (2022) 28(1):116–24. doi: 10.1111/odi.13739
61. Yang AN, Kaghad M, Wang YM, Gillett E, Fleming MD, Dotsch V, et al. P63, a P53 homolog at 3q27-29, encodes multiple products with transactivating, death-inducing, and dominant-negative activities. Mol Cell (1998) 2(3):305–16. doi: 10.1016/S1097-2765(00)80275-0
62. Romano RA, Solomon LW, Sinha S. Tp63 in oral development, neoplasia, and autoimmunity. J Dent Res (2012) 91(2):125–32. doi: 10.1177/0022034511411302
63. Foschini MP, Gaiba A, Cocchi R, Pennesi MG, Gatto MR, Frezza GP, et al. Pattern of P63 expression in squamous cell carcinoma of the oral cavity. Virchows Arch (2004) 444(4):332–9. doi: 10.1007/s00428-003-0969-x
64. Matsubara R, Kawano S, Kiyosue T, Goto Y, Hirano M, Jinno T, et al. Increased Deltanp63 expression is predictive of malignant transformation in oral epithelial dysplasia and poor prognosis in oral squamous cell carcinoma. Int J Oncol (2011) 39(6):1391–9. doi: 10.3892/ijo.2011.1151
65. Higashikawa K, Yoneda S, Tobiume K, Taki M, Shigeishi H, Kamata N. Snail-induced down-regulation of Deltanp63alpha acquires invasive phenotype of human squamous cell carcinoma. Cancer Res (2007) 67(19):9207–13. doi: 10.1158/0008-5472.CAN-07-0932
66. Goto Y, Kawano S, Matsubara R, Kiyosue T, Hirano M, Jinno T, et al. Possible involvement of Deltanp63 downregulation in the invasion and metastasis of oral squamous cell carcinoma Via induction of a mesenchymal phenotype. Clin Exp Metastasis (2014) 31(3):293–306. doi: 10.1007/s10585-013-9628-z
67. Sakamoto T, Kawano S, Matsubara R, Goto Y, Jinno T, Maruse Y, et al. Critical roles of Wnt5a-Ror2 signaling in aggressiveness of tongue squamous cell carcinoma and production of matrix metalloproteinase-2 Via Deltanp63beta-mediated epithelial-mesenchymal transition. Oral Oncol (2017) 69:15–25. doi: 10.1016/j.oraloncology.2017.03.019
68. Hashiguchi Y, Kawano S, Goto Y, Yasuda K, Kaneko N, Sakamoto T, et al. Tumor-suppressive roles of Deltanp63beta-Mir-205 axis in epithelial-mesenchymal transition of oral squamous cell carcinoma Via targeting Zeb1 and Zeb2. J Cell Physiol (2018) 233(10):6565–77. doi: 10.1002/jcp.26267
69. Srivastava K, Pickard A, Craig SG, Quinn GP, Lambe SM, James JA, et al. Deltanp63gamma/Src/Slug signaling axis promotes epithelial-to-Mesenchymal transition in squamous cancers. Clin Cancer Res (2018) 24(16):3917–27. doi: 10.1158/1078-0432.CCR-17-3775
70. Jiang L, Zeng X, Yang H, Wang Z, Shen J, Bai J, et al. Oral cancer overexpressed 1 (Oraov1): A regulator for the cell growth and tumor angiogenesis in oral squamous cell carcinoma. Int J Cancer (2008) 123(8):1779–86. doi: 10.1002/ijc.23734
71. Jiang L, Yang HS, Wang Z, Zhou Y, Zhou M, Zeng X, et al. Oraov1-a correlates with poor differentiation in oral cancer. J Dent Res (2009) 88(5):433–8. doi: 10.1177/0022034509336994
72. Luo X, Jiang Y, Chen F, Wei Z, Qiu Y, Xu H, et al. Oraov1-b promotes oscc metastasis Via the nf-Kb-Tnfα loop. J Dent Res (2021) 100(8):858–67. doi: 10.1177/0022034521996339
73. Hua Y, Vickers TA, Okunola HL, Bennett CF, Krainer AR. Antisense masking of an hnrnp A1/A2 intronic splicing silencer corrects Smn2 splicing in transgenic mice. Am J Hum Genet (2008) 82(4):834–48. doi: 10.1016/j.ajhg.2008.01.014
74. Cirak S, Arechavala-Gomeza V, Guglieri M, Feng L, Torelli S, Anthony K, et al. Exon skipping and dystrophin restoration in patients with duchenne muscular dystrophy after systemic phosphorodiamidate morpholino oligomer treatment: An open-label, phase 2, dose-escalation study. Lancet (2011) 378(9791):595–605. doi: 10.1016/S0140-6736(11)60756-3
75. Guo J, Che X, Wang X, Jia R. Inhibition of the expression of oncogene Srsf3 by blocking an exonic splicing suppressor with antisense oligonucleotides. RSC Adv (2018) 8(13):7159–63. doi: 10.1039/c7ra11267j
76. Sun Y, Yan L, Guo J, Shao J, Jia R. Downregulation of Srsf3 by antisense oligonucleotides sensitizes oral squamous cell carcinoma and breast cancer cells to paclitaxel treatment. Cancer Chemother Pharmacol (2019) 84(5):1133–43. doi: 10.1007/s00280-019-03945-9
77. Zhang Y, Wang M, Meng F, Yang M, Chen Y, Guo X, et al. A novel Srsf3 inhibitor, Sfi003, exerts anticancer activity against colorectal cancer by modulating the Srsf3/Dhcr24/Ros axis. Cell Death Discovery (2022) 8(1):238. doi: 10.1038/s41420-022-01039-9
78. Hasegawa M, Miura T, Kuzuya K, Inoue A, Won Ki S, Horinouchi S, et al. Identification of Sap155 as the target of Gex1a (Herboxidiene), an antitumor natural product. ACS Chem Biol (2011) 6(3):229–33. doi: 10.1021/cb100248e
79. Convertini P, Shen M, Potter PM, Palacios G, Lagisetti C, de la Grange P, et al. Sudemycin e influences alternative splicing and changes chromatin modifications. Nucleic Acids Res (2014) 42(8):4947–61. doi: 10.1093/nar/gku151
80. Kumar D, Kashyap MK, Yu Z, Spaanderman I, Villa R, Kipps TJ, et al. Modulation of rna splicing associated with wnt signaling pathway using fd-895 and pladienolide b. Aging-Us (2022) 14(5):2081–100. doi: 10.18632/aging.203924
81. Larrayoz M, Blakemore SJ, Dobson RC, Blunt MD, Rose-Zerilli MJ, Walewska R, et al. The Sf3b1 inhibitor spliceostatin a (Ssa) elicits apoptosis in chronic lymphocytic leukaemia cells through downregulation of mcl-1. Leukemia (2016) 30(2):351–60. doi: 10.1038/leu.2015.286
82. Gao Y, Koide K. Chemical perturbation of mcl-1 pre-mrna splicing to induce apoptosis in cancer cells. ACS Chem Biol (2013) 8(5):895–900. doi: 10.1021/cb300602j
83. Gao Y, Trivedi S, Ferris RL, Koide K. Regulation of Hpv16 E6 and Mcl1 by Sf3b1 inhibitor in head and neck cancer cells. Sci Rep (2014) 4:6098. doi: 10.1038/srep06098
84. Cheng R, Xu Z, Luo M, Wang P, Cao H, Jin X, et al. Identification of alternative splicing-derived cancer neoantigens for mrna vaccine development. Brief Bioinform (2022) 23(2):bbab553. doi: 10.1093/bib/bbab553
Keywords: alternative splicing, OSCC, splicing factor, tumor progression, therapeutic targets
Citation: Liu M, Guo J and Jia R (2022) Emerging roles of alternative RNA splicing in oral squamous cell carcinoma. Front. Oncol. 12:1019750. doi: 10.3389/fonc.2022.1019750
Received: 15 August 2022; Accepted: 14 November 2022;
Published: 25 November 2022.
Edited by:
Xiao Zhu, Hangzhou Medical College, ChinaReviewed by:
Manoj Kumar Kashyap, Amity University Gurgaon, IndiaCopyright © 2022 Liu, Guo and Jia. This is an open-access article distributed under the terms of the Creative Commons Attribution License (CC BY). The use, distribution or reproduction in other forums is permitted, provided the original author(s) and the copyright owner(s) are credited and that the original publication in this journal is cited, in accordance with accepted academic practice. No use, distribution or reproduction is permitted which does not comply with these terms.
*Correspondence: Jihua Guo, amlodWFndW9Ad2h1LmVkdS5jbg==; Rong Jia, amlhcm9uZ0B3aHUuZWR1LmNu
Disclaimer: All claims expressed in this article are solely those of the authors and do not necessarily represent those of their affiliated organizations, or those of the publisher, the editors and the reviewers. Any product that may be evaluated in this article or claim that may be made by its manufacturer is not guaranteed or endorsed by the publisher.
Research integrity at Frontiers
Learn more about the work of our research integrity team to safeguard the quality of each article we publish.