- 1Department of Gastrointestinal Surgery, Shunde Hospital, Southern Medical University (The First People’s Hospital of Shunde Foshan), Foshan, Guangdong, China
- 2The Second School of Clinical Medicine, Southern Medical University, Guangzhou, Guangdong, China
- 3Guangdong Country Garden School, Shunde, Foshan, Guangdong, China
Copper is an essential trace element in an organism, and changes in copper levels in vivo often indicate a diseased state. Copper and immunity have been discussed since the last century, with copper deficiency significantly affecting the development and function of the immune system, such as increased host susceptibility to various pathogens, decreased number and impaired function of neutrophils, reduced antibacterial activity of macrophages, decreased proliferation of splenocytes, impaired B cell ability to produce antibodies and impaired function of cytotoxic T lymphocyte and helper T cells. In the past 20 years, some studies have shown that copper ions are related to the development of many tumors, including lung cancer, acute lymphoid leukaemia, multiple myeloma and other tumors, wherein copper ion levels were significantly elevated, and current studies reveal that copper ions are involved in the development, growth and metastasis of tumors through various pathways. Moreover, recent studies have shown that copper ions can regulate the expression of PD-L1, thus, attention should be paid to the important role of copper in tumor immunity. By exploring and studying copper ions and tumor immunity, new insights into tumor immunity could be generated and novel therapeutic approaches to improve the clinical prognosis of patients can be provided.
Introduction
Copper is an essential trace element in an organism. The redox properties of copper enable it to act as a catalytic cofactor for various enzymes, allowing for protein binding through complexation with cysteine, histidine and methionine, and binding to lower molecular compounds (1, 2). It participates in various redox reactions such as energy metabolism, mitochondrial respiration (cytochrome oxidase (COX)), antioxidation (zinc and copper superoxide dismutase (SOD)), collagen cross-linking (lysine oxidase (LOX)), pigmentation (tyrosinase) and catecholamine biosynthesis (dopamine-b-monooxygenase) (3).
Tumorigenesis is inextricably linked to abnormalities of the immune system, and copper ions are considered to be an indispensable trace element in the immune homeostasis of the body. Since the middle of the last century, copper deficiency has been found to significantly affect the development and function of the body’s immune system. Initially, copper deficiency was found to decrease the number of blood neutrophils (4) and impair their anti-bacterial function via reducing the production of superoxide anions (5). Subsequently, copper deficiency in various animal models has been found to cause immunosuppression (6–8), leading to the impaired function of immune cells such as B (9) and T cells (10) (Figure 1; Table 1). Additionally, copper deficiency also affects the development of the immune system in mice (10, 11). These data suggest that copper ions are essential for the proper functioning of the immune system, thereby maybe influencing tumorigenesis and development through immune-related pathways.
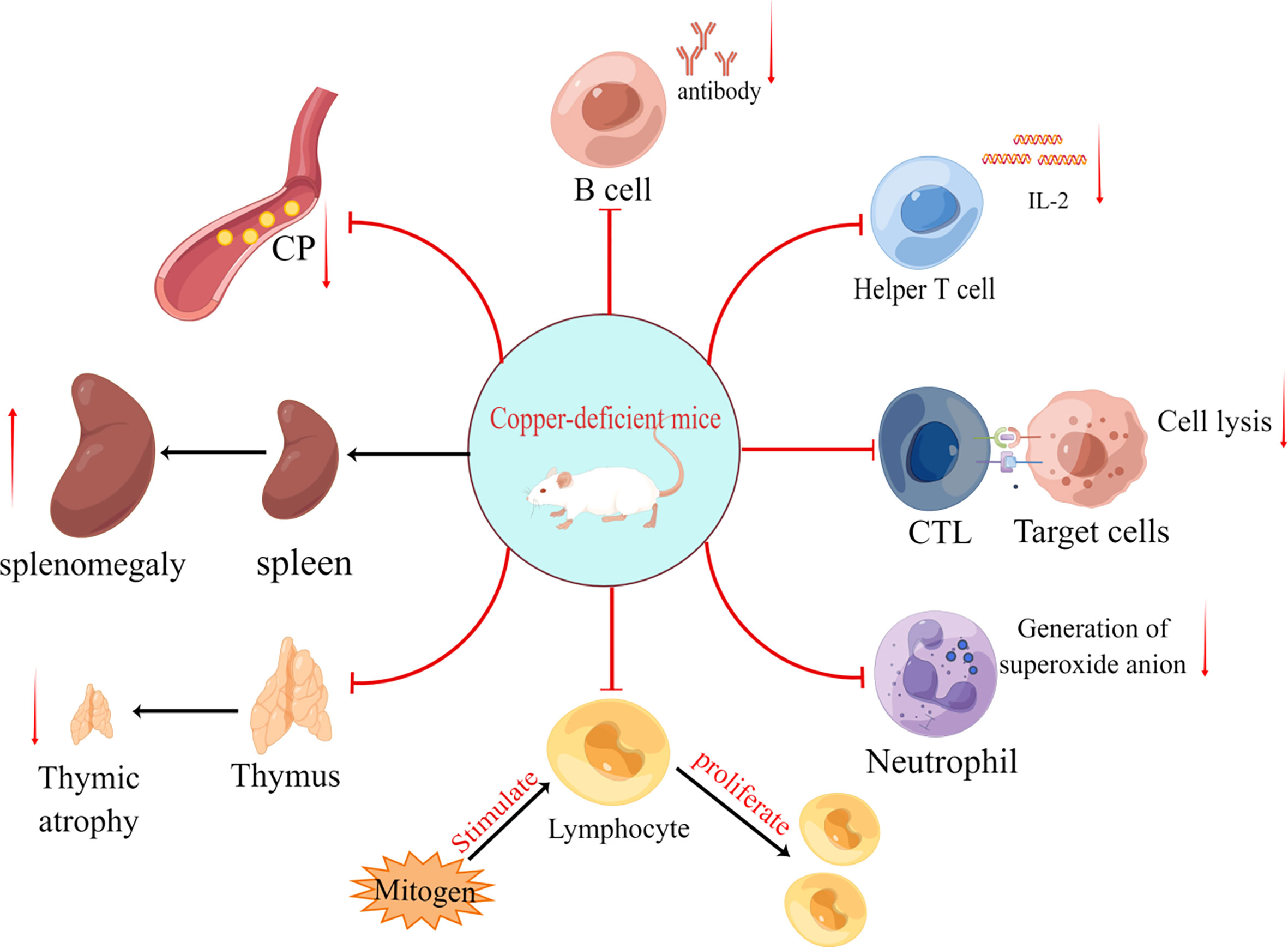
Figure 1 The effects of copper deficiency on mice are divided into eight primary aspects: (i) reduced antibody secretion by B cells; (ii) reduced IL-2 secretion by helper T cells; (iii) reduced target cell killing capacity of cytotoxic T lymphocyte (CTL); (iv) reduced number of neutrophils and production of superoxide anion (O2-); (v) reduced responsiveness of lymphocytes to mitogen stimulation; (vi) thymic atrophy; (vii) splenomegaly and (viii) decreased ceruloplasmin(CP).
Alterations in copper levels are often associated with diseases such as Menkes disease and Wilson disease (hepatolenticular degeneration) (24–26), which are characterized by a deficiency or an excess of copper in the body, respectively. The initial studies of Menkes and Wilson diseases laid the foundation for the understanding of intracellular transport and distribution of copper and determining the role of copper and copper metabolism proteins in cell signaling, gene expression and cancer cell proliferation (27). With scientific advances, the abnormal accumulation of copper in cancer cells was found to be an important feature in differentiating them from normal cells. Relevant experimental data showed that several cancer cells maintained trace elements, such as zinc, selenium or iron, at normal levels, but copper levels were significantly elevated (28), such as in stage I multiple myeloma (29), lung cancer (30) and acute lymphoblastic leukaemia (31).
With increasing studies, there is now a certain understanding of the molecular mechanisms of copper involvement in tumor development. Currently, copper has been found to play an important role in the high-affinity copper transporter (CTR-1) (32), LOX (33), hypoxia-inducible factor (HIF-1α) (34), antioxidant protein-1(ATOX-1) (35, 36), RAS-RAF-MEK-ERK signaling pathway (37) (38, 39) and PD-L1 (40), which are associated with tumorigenesis, adaptation to hypoxic environment (41), tumor extracellular matrix construction(ECM), neovascularization, epithelial to mesenchymal tissue transition (EMT) and tumor metastasis. Current studies of these enzymes and pathways identify other gene and protein targets that could be used for cancer therapy.
Current therapeutic strategies using traditional copper-binding molecules, such as copper chelators (ammonium tetrathiomolybdate(TTM)) and copper ionophores (disulfiram (DSF)) (42) (43, 44), have been proposed for cancer treatment and used in clinical trials. Meanwhile, novel copper-binding molecules (e.g., plant-derived copper-binders, autophagy inhibitors, proteasome inhibitors) have gradually become popular research topics due to their antitumor activity and low side effects. Although some of these copper-binding molecules have achieved a certain level of clinical efficacy, certain limitations remain. For example, DSF has not achieved satisfactory results in clinical trials because of its metabolism to diethyldithiocarbamate with low bioavailability in vivo (45). Therefore, further clinical trials are needed to prove its efficacy and safety. Owing to the close relationship between copper, immunity and tumor, this review provides new ideas on the use of copper in tumor immunotherapy and the development of better and more effective treatments to improve the prognosis of patients with tumors.
Relationship between copper and immunity
The recommended daily dietary requirement of copper for a healthy adult is 0.9 mg. Copper is absorbed mainly through the small intestine, enters the liver through the portal vein and is partially excreted through the bile and mostly transported to different tissues and organs by binding to albumin and ceruloplasmin in the blood (3). In the 1950s, researchers found that copper deficiency causes hypocopperemia, hypoceruloplasminemia and neutropenia in infants (4, 6, 46–48) (Figure 1). Following this, it was found that copper deficiency leads to impaired superoxide anion production by neutrophils, which kills bacteria (5). Since then, the effect of copper on the immune system has gained increasing research attention.
Macrophages are an important component of the innate immune system and play a key role in the activation and regulation of specific immunological responses through antigen presentation and secretion of cytokines (49, 50), in addition to phagocytosis. In 1991, Bala et al. found that although copper deficiency caused a much larger percentage of macrophages in the spleen of mice than in controls, the absolute number was reduced (19). And Babu et al. discovered that copper deficiency dramatically reduced the production of superoxide anion in macrophages, which in turn affected the ability to kill yeast, Candida, etc., making the organism more susceptible to infection (51). However, studies has been demonstrated that excessive copper supplementation can inhibit macrophage function (52), and so, maintaining copper homeostasis inside and outside the cell is one of the prerequisites for proper macrophage function. While White et al. in 2009 discovered that the effect of copper ions on macrophages appears to be related to the expression of ATP7A in macrophages, they also discovered that pro-inflammatory agents such as LPS or IFN-γ promote copper uptake by macrophages and increase the expression of CTR1 and ATP7A proteins, and demonstrated that the ability of phagocytes to kill pathogens was positively correlated with the expression of ATP7A (53) (54), and consistent with this hypothesis, researchers have identified the process of copper efflux from bacteria via copA protein as a defensive mechanism against macrophage killing (55). Tumor-associated macrophages (TAMs) are subtypes of macrophages, which differ from general macrophages in that they exert immunosuppressive effects in the tumor microenvironment by secreting immunosuppressive cytokines (e.g., TGF-β, IL-10) and, while also promote tumor progression and metastasis by secreting growth factors and angiogenic factors (56). Studies targeting TAMs have found that CuNG (a copper chelate) can promote reprogramming of TAMs, promote the secretion of IL-12 and reduce the secretion of TGF-β and IL-10, thus altering their immunosuppressive properties and reactivating the immune response of T cells against tumor cells (57). Although not much research has been done on copper and macrophages, together with the above investigations, we believe that further research and uncovering the mechanism of action between copper and macrophages are feasible and significant for alleviating the immunosuppressive state of TME and enhancing the efficacy of immunotherapy.
In addition to innate immunity, the effect of copper deficiency on acquired immunity has been demonstrated. In 1981, Prohaska et al. observed a reduction in the number of antibody-producing plasma cells in severe and borderline copper-deficient mice, and the extent of this impairment was highly correlated with the degree of hypoceruloplasminemia (9). In the same year, Flynn and Yen demonstrated experimentally that CTL in copper-deficient mice has a reduced ability to target cytolytic cells stimulated by alloantigens and that this defect seems to be associated with the dysfunction of helper T cells (5) (Figure 1; Table 1). Lukasewycz et al. found that the initiation and maintenance of cell-mediated immunity against leukemic cells were severely impaired in copper-deficient animals (58). Additionally, copper deficiency was also reported to lead to reduced numbers and impaired function of T cells in vitro (59) (Table 1). Thus, it is clear that copper deficiency affects both humoral and cellular immunity.
The proliferative activity and composition of immune cells are altered in response to copper deficiency. Prohaska et al. demonstrated the reduced responsiveness of splenic lymphocytes in copper-deficient mice models to mitogens such as lipopolysaccharide, phytohemagglutinin and Concanamycin A (16) (Figure 1; Table 1). The composition of lymphocytes was changed in copper-deficient mice. Furthermore, Lukasewycz et al. (17) analyzed the surface determinant clusters of splenocytes in copper-deficient C58 mice and found that the absolute number and relative percentage of B cells were significantly higher than that in copper-supplemented controls, whereas the relative percentage of Thy 1.2-positive T cells decreased most significantly in the Lyt 1 positive cell (helper T cells) subpopulation (Table 1). Additionally, Bala et al. (19) reported a decrease in total splenic monocyte production and the relative and absolute numbers of CD4+ and CD8+ T subpopulations in copper-deficient male rats, however, copper deficiency only increased the relative percentage of splenic B cells and not the absolute numbers. This contrast with the above-mentioned results of Lukasewycz’s experiment could be attributed to the animal model used for the experiment, the experimental method or the apparatus. Another study by Bala et al. demonstrated that dietary supplementation with copper restored the number and function of helper T cells (60). In 1981, Flynn et al. showed that CTL in a copper-deficient medium failed to produce the specific killing of target cells (Figure 1; Table 1), and which are fully recovered after the addition of T-cell replacement factor (TRF), thus demonstrating the suppressive effect of copper deficiency on helper T cells (10). These experiments demonstrate that copper deficiency leads to a decrease in the number and function of helper T cells. Therefore, copper deficiency has the potential to affect T cells, especially helper T cells, more than B cells.
Copper deficiency affects the production and secretion of immunoreactive substances. In 1981, Prohaska et al. demonstrated that antibody production by splenic plasma cells against sheep erythrocyte antigens was significantly reduced when copper was deficient in cells (9) (Table 1). Hamilton et al. also demonstrated that antibody production against sheep erythrocytes was inhibited in copper-deficient mice (P < 0.0001). Contrastingly, antibody production against dinitrophenyl-phenanthroline was not altered by copper deficiency (P = 0.90) (61). In the same year, Koller et al. also demonstrated that copper deficiency affected antibody secretion, resulting in a decrease in plasma antibody titers (18). However, the normal functioning of helper T cells is indispensable for the production of antibodies. Moreover, in Menkes disease, the production of T cells was found to be affected (62). Many of the above experiments have also demonstrated that copper deficiency affects the number and function of helper T cells in experimental animals (10, 17, 19). Furthermore, Lukasewycz et al. (20) found that copper deficiency seemed to have different effects on different cytokines of T cells, such as the overproduction of IL-1 and underproduction of IL-2 (Figure 1; Table 1). However, the mechanism of copper regulating these cytokines remains unclear. Hopkins et al. in 1997 found that copper deficiency reversibly decreased the amount of IL-2 and IL-2 mRNA in human T lymphocytes (21). In 1999, Hopkins et al. further showed that copper deficiency in human Jurkat T lymphocytes impaired the transcriptional regulation of IL-2 gene expression, resulting in reduced IL-2 production (22) (Table 1). Furthermore, in 1992, Bala et al. showed that copper deficiency reversibly restricted DNA synthesis in T lymphocytes by decreasing IL-2 activity, leading to T cell dysfunction (23). Additionally, the reduction in IL-2 synthesis appears to be associated with reduced splenocyte proliferative activity (63). Given these results, it can be speculated that copper deficiency reduces IL-2 synthesis by decreasing IL-2 mRNA synthesis, leading to impaired functions, such as the regulation of other immune cells by helper T cells, the killing capacity of CTL and antibody secretion by B cells.
Interestingly, the effects of copper deficiency on the immune function also vary with gender (64, 65), with male mice appearing to be more susceptible to the effects of copper deficiency (14). However, the mechanisms responsible for this gender difference are unclear.
The occurrence of severe copper deficiency in humans is rare, with borderline copper deficiency state being the most common. Moreover, studies on the effects of copper deficiency on immunity in humans are very few. In 1985, Heresi et al. studied immunoglobulin and neutrophil phagocytosis in children with copper deficiency and found that copper deficiency reversibly impaired neutrophil phagocytosis (66). In 1995, Kelley et al. found that the proliferative capacity of human peripheral blood mononuclear cells and the concentration of IL-2 decreased with a copper-deficient diet, but there was no effect on the peripheral blood cell counts of leukocytes, monocytes, neutrophils, lymphocytes or natural killer cells (67). This result contradicts the significant difference observed in the number and function of neutrophil and lymphocyte subpopulations in animal experiments, which could be attributed to biological differences between animals and humans and the different levels of copper deficiency to which both are subjected. Therefore, the effect of copper deficiency on the human immune system needs further exploration.
The relationship between copper and tumors
The association between copper and tumors began with the finding that copper levels are elevated in many tumors. In 1965, de Jorge et al. demonstrated an 11-fold increase in copper levels in brain cancer, which was the first study linking tumors to copper levels (68). Subsequently, in 1975, Schwartz highlighted the potential role of copper as a carcinogenic and diagnostic/prognostic marker (69). Meanwhile, studies found elevated copper serum concentrations in various tumors (28), such as stage I multiple myeloma (29), reticulocyte sarcoma, bronchial and laryngeal squamous cell carcinoma, lung cancer (31), leukaemia, lymphoma (70), cervical cancer, breast cancer and gastric cancer (71, 72). In addition to elevated serum copper levels, elevated copper concentrations were reported in the nails and/or hair of patients with tumors, such as breast, prostate and cervical cancers (73). Furthermore, copper deposition in the eye is associated with lung adenocarcinoma (74), multiple myeloma (75) and chronic lymphocytic leukaemia (76). In a clinical study of hematologic malignancies, Kaiafa et al. found that serum copper levels appeared to correlate with disease remission and recurrence (70). Current studies report that elevated copper concentrations in tumor cells are associated with a high expression of CTR-1, however, further investigation is required to determine if there are other factors influencing these copper levels.
Although the specific mechanism of copper involvement in tumor development requires further study, previous reports have identified some of the possible mechanisms of copper involvement in tumor development. CTR-1 is the main transporter protein for the cellular uptake of copper, and researchers have found elevated intracellular copper in various tumors, exhibiting a high expression state of CTR-1. Thus, the hypothesis of CTR-1 promoting tumor development gained attention. Guo et al. (77) in 2021 showed that copper can promote tumorigenesis by activating the phosphatidylinositol 3-kinase (PI3K)-protein kinase B (PKB, also known as AKT) oncogenic signaling pathway and reported that blocking CTR-1 and reducing intracellular copper can inhibit the tumor-promoting effects of this signaling pathway (Figure 3). The RAS-RAF-MEK-ERK signaling pathway is another signaling pathway required for cancer formation (37), wherein copper plays a promotional role (38). Copper promotes ERK phosphorylation by binding to MEK1 (copper-binding protein), which leads to RAS/MAPK signaling activation and subsequently to cancer development. Similarly, researchers have found that blocking CTR-1 leads to impaired RAS/MAPK signaling activation, which hinders the oncogenic effects of BRAF/V600E mutation (38, 39) (Figure 3). Thus, CTR-1 plays an important role in tumorigenesis, and CTR-1 inhibitors have good therapeutic potential. In addition to participating in tumorigenesis, copper also has a facilitative role in tumor development. Copper promotes the adaptation of cancer cells to the hypoxic environment by reducing degradation (41) and increasing HIF-1α stability (34). It also promotes the construction of the ECM by regulating the activity of LOX (33). HIF-1α and LOX promote tumor development in coordination with each other (78, 79). Furthermore, Eva et al. in 2021 summarized the relationship between copper and tumors and proposed the concept of “cuproplasia”, which is defined as cell proliferation that is regulated by copper (80). It is implicated in many cellular functions, such as mitochondrial respiration, antioxidative defense mechanisms, kinase signaling, autophagy and protein control.
Many tumors stimulate tumor growth through neovascularization (81), which is regulated by angiogenesis-stimulating factors (angiogenin, vascular endothelial growth factor (VEGF), basic fibroblast growth factor (bFGF) and transforming growth factor β (TGFβ)), cytokines (IL-1, -6 and -8)) and angiogenesis-inhibiting factors (vasopressors and endothelial inhibitors) (3). Notably, the activity of many angiogenic factors (calcium-dependent protease (eNOS), angiogenin, platelet-derived growth factor (PDGF)) and their expression (VEGF, fibroblast growth factor (FGF-1) and copper cyanobacteria) are regulated by copper. For example, 1) copper upregulates the expression of several angiogenic genes (e.g., ceruloplasmin, VEGF, FGF-1) (34, 82) by regulating the activation of HIF-1α; 2) copper promotes NO pathway-induced vasodilation by enhancing the enzymatic activity of eNOS (83); 3) copper enhances the function of PDGF and thus promotes smooth muscle cell migration and LOX secretion (84); 4) copper promotes FGF-1 synthesis, thereby promoting vascular endothelial proliferation and ECM formation (83) (Table 2). Therefore, copper plays an essential cofactor role in the whole angiogenic signaling cascade and hence copper deficiency can hinder neovascularization (89). Currently, copper chelators are used as neovascularization inhibitors in the clinical treatment of tumors.
Tumor metastasis is an important factor indicating poor patient prognosis. Studies suggest that the two key pathways in tumor metastasis, ECM construction and EMT, are regulated by copper ions (33, 78, 90–92) (Figure 2). Cancer cells secrete LOX to promote the cross-linking of collagen and elastin in the ECM (33) and to prepare a suitable environment for the metastasis of tumor cells (93). LOX is a copper-dependent enzyme, and copper ions can induce the secretion of LOX by activating HIF-1α. Moreover, LOX can also promote the synthesis of HIF-1α protein by positive feedback, and the two factors synergize and regulate each other to promote tumor progression (78) (79) (Figure 2). LOX also promotes EMT development by stimulating Twist transcription (91). It is also speculated to be an inter-responder of MEMO1, a protein involved in cell migration by regulating the cytoskeleton and forming adhesion sites (94). MEMO1 is a copper-dependent oxidoreductase whose main roles are to produce reactive oxygen species (ROS) that control the redox state of cells and regulate EMT-related transcriptional pathways (95). Copper also contributes to EMT development by activating the interaction between HIF-1α and HRE (96) and the HIF1-α-Snail/Twist signaling pathway (92) to promote the regulation of EMT (Figure 2). Some studies have found that the dysregulation of Amyloid Precursor Protein (APP) expression, which contains an extracellular copper-binding domain at the N-terminal end, is also involved in the development of EMT in tumor cells (97–99). Therefore, copper promotes tumor development through multiple pathways and their inhibition is crucial to avoiding poor prognosis due to tumor metastasis.
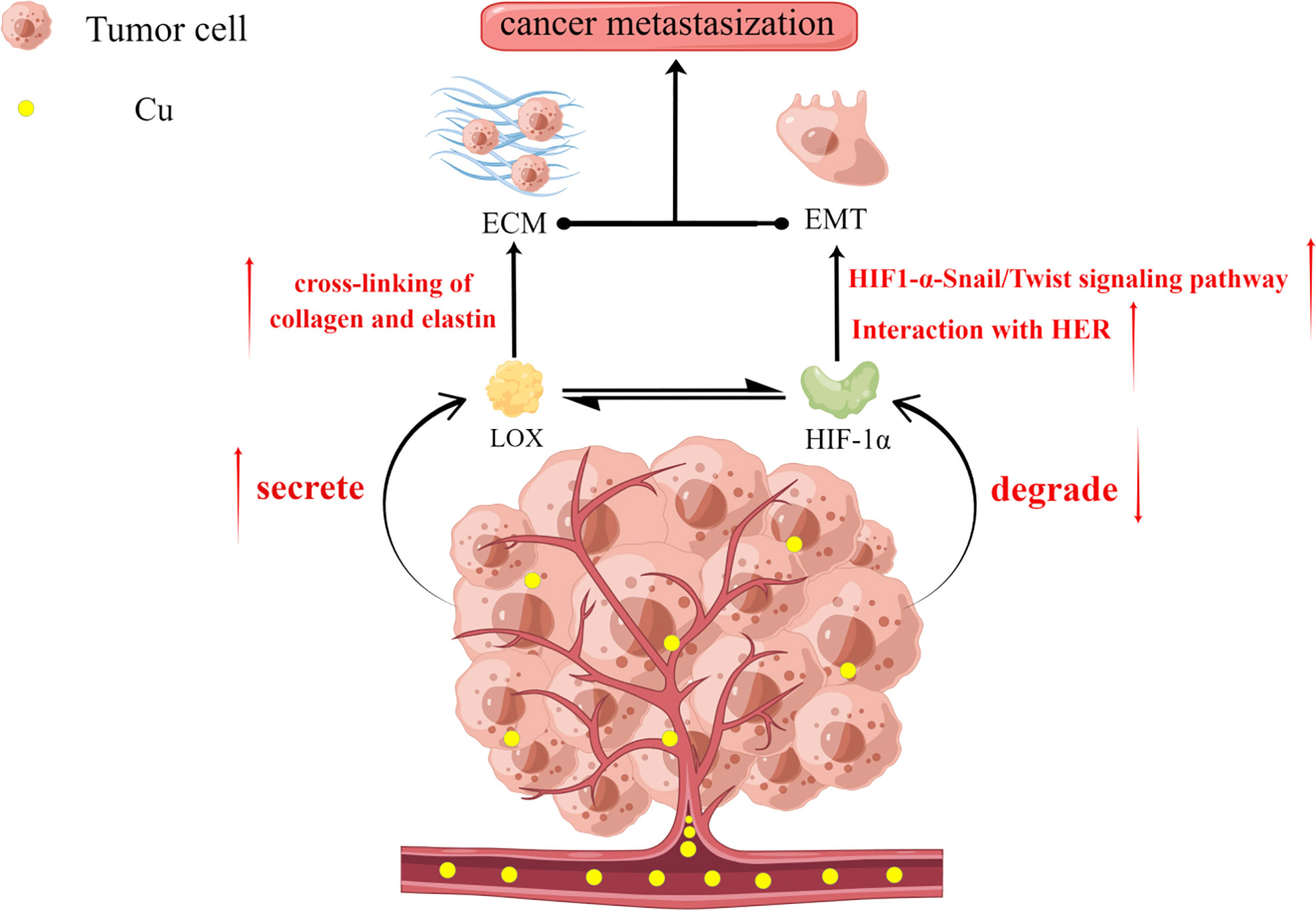
Figure 2 Copper plays an important role in extracellular matrix (ECM) construction and epithelial-mesenchymal transition (EMT), which are two key aspects of tumor metastasis. Copper promotes the secretion of LOX by tumor cells but inhibits the degradation of the structural stability of HIF-1α. There also exists a reciprocal relationship between LOX and HIF-1α. LOX promotes ECM formation by facilitating the cross-linking of collagen and elastin. Increased HIF-1α promotes the adaptation of tumor cells to the hypoxic environment while promoting EMT through interactions with the hypoxia response element (HRE) and the HIF1-α-Snail/Twist signaling pathway. Together, these factors promote the development of tumor metastasis.
Current studies have shown that copper is implicated in several key aspects of tumor development. (1) Copper promotes the adaptation of cancer cells to the hypoxic environment by increasing the level of HIF-1α; (2) Tumor angiogenesis is necessary for tumor growth progression, wherein copper is involved in tumor neovascularization by directly interacting with angiogenic factors (VEGF and FGF) (86, 100)and regulating the synthesis of angiogenic molecules (101, 102); (3) Tumor metastasis often implies poor prognosis, and copper promotes tumor metastasis by promoting ECM construction and EMT. Thus, a gradual elucidation of the interrelationship between copper and tumors has been provided, which has therapeutic potential for patients with cancer.
Copper and tumor immunity-related studies
Copper, immunity and tumors are closely related. Therefore, copper could have a potential regulatory role in tumor immunity. Some studies have reported on the relationship between copper and tumor immunity, such as the reduction of copper in mouse mesothelioma associated with CD4+ T cell infiltration (103), the effect of anti-tumor drug Dp44mT on T cell activity through a copper-related mechanism (104, 105), the apoptosis of bone marrow-derived suppressor cells (MDSCs) and the enhancement of anti-tumor immune responses through selective copper chelation in a drug-resistant tumor model (106) and the downregulation of ACO3 (copper-containing amine oxidase) in lung cancer leading to reduced adherent aggregation of CD4+ cells (107). In a phase II study of high-risk and triple-negative patients with breast cancer, tetrathiomolybdate (TTM) reduced collagen deposition, decreased MDSCs levels and increased CD4+ T cell infiltration in the treated mice (108). In 2020, Voli (40) was the first to propose that copper ions could regulate the expression of the immune checkpoint PD-L1, opening up the possibility and potential of copper ions to participate in tumor therapy by interfering with tumor immunity.
Tumor immune escape is a protective mechanism by which tumor cells protect themselves from the immune system, mainly through the binding of PD-L1, which is overexpressed by tumor cells, to PD-1, which is expressed by lymphocytes, and thereby exerting a negative regulatory effect on T lymphocytes and resulting in diminished cytokine production and cytotoxic effects of immune cells on tumor cells. Through this mechanism, tumor cells can proliferate in vivo without the control of the immune system (109). Therapeutic agents targeting this immune checkpoint are currently in clinical application and have greatly contributed to the prognosis of tumor treatment. However, the application of this therapy is currently limited due to the varying reactivity of different tumor cells and the side effects associated with the treatment.
On examining biopsies from 90 patients with neuroblastoma and 90 patients with a brain tumor (including GBM), Voli et al. demonstrated that the three indicators, CTR1/PD-L1/MT1X, have a positive correlation with each other. However, the positive correlation between PD-L1 and CTR1 was limited to tumor tissues. On further exploring how intracellular copper regulates PD-L1 expression, the authors found that Cu and IFN-γ share similar signaling pathways in the regulation of PD-L1 expression and tumor immune response in tumor cells using transcriptome and gene set enrichment analysis. Contrastingly, experiments with copper chelators (DC/TEPA) revealed that DC and TEPA down-regulated PD-L1 expression by inhibiting EGFR signaling and transcriptional activator protein (STAT) phosphorylation signaling pathways (Figure 3). Furthermore, they confirmed through several experiments that reducing intracellular copper can inhibit PD-L1 expression at two levels: (1) at the transcriptional level by downregulating the JAK/STAT cellular pathway and reducing PD-L1 mRNA production; (2) at the translational level by promoting the ubiquitination of PD-L1 and facilitating its degradation to downregulate PD-L1 (Figure 3). In in vivo experiments validated by flow cytometry analysis, the authors confirmed that TEPA and DC increased CD8+/CD4+ T lymphocytes and CD244+ NK cell infiltration. Whole-transcript sequencing of tumor tissues demonstrated that TEPA increased the number of macrophages, CD4+, CD8+ T lymphocytes and NK cells. Furthermore, DC and TEPA reduced IFN-γ (from activated NK cells) mediated PD-L1 upregulation and enhanced NK cell-mediated tumor lysis.
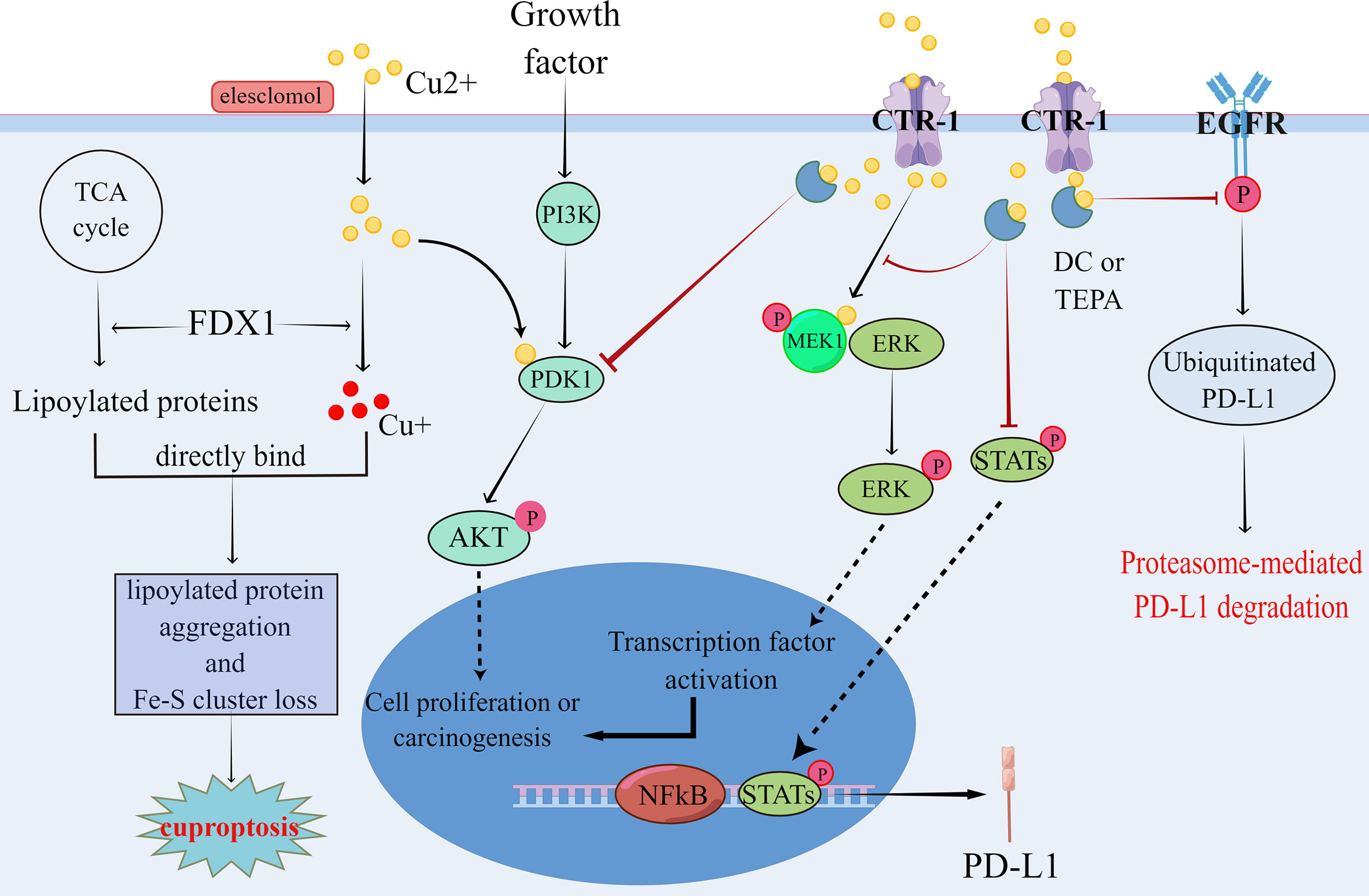
Figure 3 Copper has been shown to promote tumor formation by affecting the regulation of phosphorylation in the PI3K-AKT and RAS-RAF-MEK-ERK signaling pathways, which in turn promotes tumor formation. Meanwhile, copper can regulate PD-L1 expression by both regulating the proteasome-mediated degradation of PD-L1 and affecting the transcription of PD-L1, which leads to tumor immune escape. In contrast, copper chelators can inhibit the aforementioned signaling pathways, thereby suppressing pro-tumor signaling and ameliorating tumor immune escape. cuprotosis is a recently identified copper-dependent mode of cell death, mainly through direct binding of copper to lipid acylated components of the TCA cycle, leading to lipid acyl protein aggregation and loss of iron-sulfur cluster proteins, which in turn leads to cuprotosis due to proteotoxic stress. These theoretical results have contributed to the understanding of the relationship between copper and tumors.
Although several studies and clinical trials have demonstrated that copper chelators are effective in inhibiting tumor growth and angiogenesis, Voli et al. demonstrated for the first time that chelation therapy reduced PD-L1 expression, enhanced anti-tumor immune responses and can be repurposed for immune checkpoint inhibition. Therefore, copper ion-related drugs have great therapeutic potential in tumor treatment.
Copper related medication
The classical anti-cancer copper-binding molecules are mainly divided into two categories: copper chelators and copper ionophores. Copper chelators act mainly by reducing intracellular copper (110), whereas copper ionophores act by increasing intracellular copper ions, which causes cytotoxic effects through various pathways (111). Furthermore, novel copper-based anti-cancer agents (plant-derived copper-binding molecules, autophagy inhibitors and proteasome inhibitors) are also beginning to emerge (3).
Copper chelators were initially used for the treatment of Wilson’s disease (112). Later, they were tested in tumor treatment and were found to inhibit tumorigenesis, angiogenesis, tumor metastasis and other effects. Common copper chelators include TTM and D-penicillamine (D-pen). TTM is a selective copper chelator that depletes bioavailable intracellular copper and exerts anticancer effects through four pathways: (1) inhibiting angiogenesis through the activation of transcription factors (NF-kB) (113); (2) inducing anti-metastatic activity via the inhibition of bone marrow-derived endothelial progenitor cell (EPC) recruitment (114, 115); (3) promoting anti-tumor proliferation activity by reducing ATP production through the inhibition of cytochrome C oxidase function (116); (4) reducing tumor growth in BRAF/V600E transformed cells by decreasing MEK1/2 kinase activity (39). Moreover, TTM is well tolerated in oncology treatment and the resulting side effects such as anemia and neutropenia are reversible (117–119). D-pen was initially a reducing and chelating agent used in the treatment of rheumatoid arthritis, and researchers studying the therapeutic effects of D-pen on arthritis found that it could lead to the reduced chemotaxis of polymorphonuclear leukocytes (120), cause a decrease in antibody titers (121–123) and cause reduced levels of circulating immune complexes (124–126). These results suggest that D-pen could exert immunosuppressive effects. Subsequent experiments have demonstrated that copper salts and ceruloplasmin enhance this immunosuppressive effect, and D-pen was found to inhibit helper T-cell function and antibody production (127–131), which is closely related to the peroxidase enzyme of monocytes (130). D-pen has been found to inhibit LOX secretion and impair collagen cross-linking in tumor therapy (132). It also inhibits tumor angiogenesis by reducing VEGF expression (103). However, some studies have reported serious adverse effects (133). Moreover, D-pen did not improve the survival rate of patients with brain tumors in phase II clinical trials(NCT00003751) (134) (Table 3).
Excessive intracellular concentrations of copper ions are cytotoxic because it leads to the excessive production of ROS and substitution of other metals from binding sites within key proteins (73), causing cytotoxicity. Tsvetkov et al. (140) demonstrated a novel copper-dependent cell death described as the binding of copper ions to the lipid acyl component of the tricarboxylic acid cycle (TCA) in mitochondrial respiration, leading to lipid acylated protein aggregation and iron-sulfur cluster protein downregulation. These result in proteotoxic stress and ultimately cell death, and this copper-dependent cell death is known as “cuproptosis” (Figure 3). Although copper ionophores have shown selective anti-cancer activity in vitro and mouse models (32, 141, 142), the mechanism of this selectivity remains unclear. Currently, common copper ionophores drugs include DSF, Chloroquine (CQ) and Elesclomol (STA-4783). Increasing the level of intracellular bioavailable copper is a common feature of copper carrier drugs. Many studies have shown that DSF can be used for treating bone metastases in breast cancer (143), ocular melanoma whit liver metastases (144) and non-small cell lung cancer(NCT00312819) (136) (Table 3). Additionally, DSF inhibits the proliferation, migration and invasion of hepatocellular carcinoma, especially the nuclear translocation of NF-κB subunit and the expression of Smad4 and leads to the downregulation of Snail and Slug, which inhibit EMT development and hinder tumor metastasis (145). CQ, a derivative of chloroquine, was originally synthesized as an antibacterial agent, but it was found to induce cell death by activating multiple apoptotic pathways in cancer cells (146). The efficacy of CQ was positively correlated with extracellular copper levels (147). Moreover, the results of clinical trials with Elesclomol as a potential copper ionophore-anti-cancer drug were unsatisfactory(NCT00522834, NCT00888615) (138, 139) (Table 3). However, subsequent analyses showed that Elesclomol affected tumors that depended on mitochondrial energy production and that FDX1, the gene encoding the target protein of Elesclomol, promotes cuproptosis (148–150). Thus, the identification of the mechanism of cuproptosis could provide more theoretical support for the application of Elesclomol.
Novel copper-based anti-cancer agents have received increasing attention over the past few years. Plant-derived copper-binding molecules (curcumin, Oleuropein-Cu complexes and resveratrol-copper complexes) have been reported to exert anti-cancer effects and increase the anti-tumor activity of known anti-cancer drugs with low side effects. These compounds act as antioxidants, but in the presence of metals such as copper, they act as pro-oxidants that catalyse ROS formation and DNA degradation. For example, curcumin has been experimentally shown to inhibit tumor growth and reduce angiogenesis, and resveratrol-copper complexes have been reported to cause DNA breakage (151). The ubiquitin proteasome pathway (UPP) is responsible for protein targeting and proteolytic degradation and plays a central role in the regulation of cell cycle progression, signal transduction, differentiation, proliferation and apoptosis (152). When traditional copper-binding compounds (i.e. CQ, DSF and TTM) enter cancer cells, they form proteasome inhibitor complexes to affect the proteasome pathway (153). Copper-based compounds have also been developed to target the autophagic process, and the inhibition of autophagic signaling has been demonstrated in human malignant glioma (154) and hepatocellular carcinoma (155).
The deletion of CTR-1 has also been found to be related to platinum anti-cancer drug resistance in tumor cells (156, 157). This could be a breakthrough in tumor resistance to platinum anti-cancer drugs. In human ovarian tumor grafts, the resistance to cisplatin and carboplatin was overcome by co-treatment with selenite, a drug used as a chemotherapeutic adjuvant (158). One possible mechanism to explain this effect could be attributed to the fact that selenite increases the expression of the antioxidant enzyme glutoxigenin 1 (Grx1), which in turn promotes an increase in CTR-1 (159). However, subsequent studies did not support this mechanism, indicating the need for further experimentation.
Copper-related anti-tumor drugs are already used clinically; however, reports on drugs related to copper and tumor immunity remain scarce. Recent studies have demonstrated that copper regulates PD-L1 expression, which could inspire the development of drugs that target tumor immunity.
Discussion
Tumors are a major class of diseases that endanger human health. In the process of fighting with tumors, human beings have discovered more and more weapons, such as surgical resection, chemotherapy, radiotherapy, immune-targeted therapy, and so on. However, it seems that tumors are also evolving, such as resistance to chemotherapy drugs, post-operative recurrence, immunotherapy side effects and a series of other problems, forcing us to discover more ways to treat tumors. And we believe that there is a close relationship between ion metabolism and tumors, especially the close relationship between copper ions and tumors and immunity, which makes one speculate that new research fields can be opened up for tumor treatment through the study of copper ions and tumor immunity.
Current studies have shown that abnormal levels of copper in the body often indicate a state of disease. And copper deficiency significantly affects the development of the immune system and normal immune function. However, the mechanism of how copper is involved in the regulation of immunity has not been elucidated and further studies are needed. Also copper ions are involved in the regulation of three important tumor properties, namely, infinite proliferation, angiogenesis and metastasis. Excitingly, the finding that copper ions can regulate the expression of the immune checkpoint PD-L1 and increase the tumor infiltration of T and NK cells, as well as cuproptosis, has raised new expectations about the possibility and great potential of copper ions to participate in tumor therapy by intervening in tumor immunity. However, while copper deficiency in normal organisms leads to immunosuppression as previously described, in tumor cells, copper chelators can increase immune cell infiltration and inhibit immune escape by decreasing copper levels. While IL-2 biphasic (160) finding, i.e., it can promote immunity and suppress tumorigenesis in early tumor stages, but in mid- to late-tumor stages, IL-2 signaling promotes 5-hydroxytryptophan (5-HTP) production via the STAT5-TPH1 pathway, leading to CD8+ T-cell depletion. Combined with the aforementioned studies showing that copper deficiency inhibits IL-2 production, this seems to explain the paradox to some extent, but the complete answer requires further study.
Author contributions
MO and YJ are responsible for the concept and design of this study. Material preparation was done by KW. FC and GP drafted the article and QJ and YL revised it critically for important intellectual content. FC and GP retrieved the data and revised the article. FC and GP participated in manuscript writing and editing, who contributed equally to this work. The final manuscript was read and approved by all authors.
Funding
This study was funded by the Natural Science Foundation of Guangdong Province, China (No. 2022A1515012315), the Beijing Science and Technology Medical Development Foundation (No. KC2021-JX-0186-94),the 2021 Special Innovation Project of Guangdong Provincial Department of Education (No. 2021KTSCX015), In-depth promotion of the innovation-driven assistance project in Foshan City (No. 2021043), the 2018 Foshan City Outstanding Young Medical Talent Training Project (No. 600009), 2020 Shunde District Competition Support Talent Project (no serial number), Southern Medical University Shunde Hospital Scientific Research Startup Plan (No. SRSP2018001), Guangdong Medical Science and Technology Research Fund Project (No. A2019302), the Science and Technology Plan Project of Foshan Science and Technology Bureau (No. 2018AB000683), National Natural Science Foundation of China, National Natural Science Youth Fund Project (No. 81802879), Southern Medical University Scientific Research Startup Plan (No. PY2018N110) and Foshan City’s 13th Five-Year Key Specialty Project (FSGSP2D135051).
Acknowledgments
We thank Figdraw (www.figdraw.com) for assistance in the pattern drawing.
Conflict of interest
The authors declare that the research was conducted in the absence of any commercial or financial relationships that could be construed as a potential conflict of interest.
Publisher’s note
All claims expressed in this article are solely those of the authors and do not necessarily represent those of their affiliated organizations, or those of the publisher, the editors and the reviewers. Any product that may be evaluated in this article, or claim that may be made by its manufacturer, is not guaranteed or endorsed by the publisher.
References
1. Arredondo M, Núñez MT. Iron and copper metabolism. Mol Aspects Med (2005) 26:313–27. doi: 10.1016/j.mam.2005.07.010
2. Robinson NJ, Winge DR. Copper metallochaperones. Annu Rev Biochem (2010) 79:537–62. doi: 10.1146/annurev-biochem-030409-143539
3. De Luca A, Barile A, Arciello M, Rossi L. Copper homeostasis as target of both consolidated and innovative strategies of anti-tumor therapy. J Trace Elem Med Biol Organ Soc Miner Trace Elem GMS (2019) 55:204–13. doi: 10.1016/j.jtemb.2019.06.008
4. Cordano A, Placko RP, Graham GG. Hypocupremia and neutropenia in copper deficiency. Blood (1966) 28:280–3. doi: 10.1182/blood.V28.2.280.280
5. Boyne R, Arthur JR. Effects of selenium and copper deficiency on neutrophil function in cattle. J Comp Pathol (1981) 91:271–6. doi: 10.1016/0021-9975(81)90032-3
6. Dunlap WM, James GW, Hume DM. Anemia and neutropenia caused by copper deficiency. Ann Intern Med (1974) 80:470–6. doi: 10.7326/0003-4819-80-4-470
7. Jones DG, Suttle NF. The effect of copper deficiency on the resistance of mice to infection with pasteurella haemolytica. J Comp Pathol (1983) 93:143–9. doi: 10.1016/0021-9975(83)90052-x
8. Newberne PM, Hunt CE, Young VR. The role of diet and the reticuloendothelial system in the response of rats to salmonella typhilmurium infection. Br J Exp Pathol (1968) 49:448–57.
9. Prohaska JR, Lukasewycz OA. Copper deficiency suppresses the immune response of mice. Science (1981) 213:559–61. doi: 10.1126/science.7244654
10. Flynn A, Yen BR. Mineral deficiency effects on the generation of cytotoxic T-cells and T-helper cell factors in vitro. J Nutr (1981) 111:907–13. doi: 10.1093/jn/111.5.907
11. Prohaska JR, Lukasewycz OA. Copper deficiency during perinatal development: effects on the immune response of mice. J Nutr (1989) 119:922–31. doi: 10.1093/jn/119.6.922
12. Prohaska JR, Downing SW, Lukasewycz OA. Chronic dietary copper deficiency alters biochemical and morphological properties of mouse lymphoid tissues. J Nutr (1983) 113:1583–90. doi: 10.1093/jn/113.8.1583
13. Vyas D, Chandra RK. Thymic factor activity, lymphocyte stimulation response and antibody producing cells in copper deficiency. Nutr Res (1983) 3:343–9. doi: 10.1016/S0271-5317(83)80084-0
14. Mulhern SA, Koller LD. Severe or marginal copper deficiency results in a graded reduction in immune status in mice. J Nutr (1988) 118:1041–7. doi: 10.1093/jn/118.8.1041
15. Jones DG. Effects of dietary copper depletion on acute and delayed inflammatory responses in mice. Res Vet Sci (1984) 37:205–10. doi: 10.1016/S0034-5288(18)31906-4
16. Lukasewycz O, Prohaska J. Lymphocytes from copper-deficient mice exhibit decreased mitogen reactivity. Nutr Res (1983). 3:335–41. doi: 10.1016/S0271-5317(83)80083-9
17. Lukasewycz OA, Prohaska JR, Meyer SG, Schmidtke JR, Hatfield SM, Marder P. Alterations in lymphocyte subpopulations in copper-deficient mice. Infect Immun (1985) 48:644–7. doi: 10.1128/iai.48.3.644-647.1985
18. Koller LD, Mulhern SA, Frankel NC, Steven MG, Williams JR. Immune dysfunction in rats fed a diet deficient in copper. Am J Clin Nutr (1987) 45:997–1006. doi: 10.1093/ajcn/45.5.997
19. Bala S, Bala ML JK. Alterations in splenic lymphoid cell subsets and activation antigens in copper-deficient rats. J Nutr (1991) 121:745–53. doi: 10.1093/jn/121.5.745
20. Lukasewycz OA, Prohaska JR. The immune response in copper deficiency. Ann N Y Acad Sci (1990) 587:147–59. doi: 10.1111/j.1749-6632.1990.tb00142.x
21. Hopkins RG, Failla ML. Copper deficiency reduces interleukin-2 (IL-2) production and IL-2 mRNA in human T-lymphocytes. J Nutr (1997) 127:257–62. doi: 10.1093/jn/127.2.257
22. Hopkins RG, Failla ML. Transcriptional regulation of interleukin-2 gene expression is impaired by copper deficiency in jurkat human T lymphocytes. J Nutr (1999) 129:596–601. doi: 10.1093/jn/129.3.596
23. Bala S, Failla ML. Copper deficiency reversibly impairs DNA synthesis in activated T lymphocytes by limiting interleukin 2 activity. Proc Natl Acad Sci U A (1992) 89:6794–7. doi: 10.1073/pnas.89.15.6794
24. Członkowska A, Litwin T, Dusek P, Ferenci P, Lutsenko S, Medici V, et al. Wilson Disease. Nat Rev Dis Primer (2018) 4:21. doi: 10.1038/s41572-018-0018-3
25. Bandmann O, Weiss KH, Kaler SG. Wilson’s disease and other neurological copper disorders. Lancet Neurol (2015) 14:103–13. doi: 10.1016/S1474-4422(14)70190-5
26. de Bie P, Muller P, Wijmenga C, Klomp LWJ. Molecular pathogenesis of Wilson and menkes disease: correlation of mutations with molecular defects and disease phenotypes. J Med Genet (2007) 44:673–88. doi: 10.1136/jmg.2007.052746
27. Turski ML, Thiele DJ. New roles for copper metabolism in cell proliferation, signaling, and disease. J Biol Chem (2009) 284:717–21. doi: 10.1074/jbc.R800055200
28. Ebadi M, Swanson S. The status of zinc, copper, and metallothionein in cancer patients. Prog Clin Biol Res (1988) 259:161–75.
29. Khadem-Ansari M-H, Asoudeh M, Gheshlaghi HFK, Nozari S, Zarringol M, Maroufi NF, et al. Copper and zinc in stage I multiple myeloma: relation with ceruloplasmin, lipid peroxidation, and superoxide dismutase activity. Horm Mol Biol Clin Investig (2018) 37(3):n. pag. doi: 10.1515/hmbci-2018-0055
30. Zhang L, Shao J, Tan S-W, Ye H-P, Shan X-Y. Association between serum copper/zinc ratio and lung cancer: A systematic review with meta-analysis. J Trace Elem Med Biol Organ Soc Miner Trace Elem GMS (2022) 74:127061. doi: 10.1016/j.jtemb.2022.127061
31. Akhgarjand C, Djafarian K, Rezvani H, Azargashb E, Vafa M. Comparing serum levels of zinc, copper, certain antioxidant vitamins and dietary intakes in acute lymphoblastic leukemia (ALL) patients before and after chemotherapy. Am J Blood Res (2018) 8:21–8.
32. Safi R, Nelson ER, Chitneni SK, Franz KJ, George DJ, Zalutsky MR, et al. Copper signaling axis as a target for prostate cancer therapeutics. Cancer Res (2014) 74:5819–31. doi: 10.1158/0008-5472.CAN-13-3527
33. Xiao Q, Ge G. Lysyl oxidase, extracellular matrix remodeling and cancer metastasis. Cancer Microenviron Off J Int Cancer Microenviron Soc (2012) 5:261–73. doi: 10.1007/s12307-012-0105-z
34. Martin F, Linden T, Katschinski DM, Oehme F, Flamme I, Mukhopadhyay CK, et al. Copper-dependent activation of hypoxia-inducible factor (HIF)-1: implications for ceruloplasmin regulation. Blood (2005) 105:4613–9. doi: 10.1182/blood-2004-10-3980
35. Itoh S, Kim HW, Nakagawa O, Ozumi K, Lessner S, Aoki H, et al. Novel role of antioxidant-1 (Atox1) as a copper-dependent transcription factor involved in cell proliferation*. J Biol Chem (2008) 283:9157–67. doi: 10.1074/jbc.M709463200
36. Chen G-F, Sudhahar V, Youn S-W, Das A, Cho J, Kamiya T, et al. Copper transport protein antioxidant-1 promotes inflammatory neovascularization via chaperone and transcription factor function. Sci Rep (2015) 5:14780. doi: 10.1038/srep14780
37. Dankner M, Rose AAN, Rajkumar S, Siegel PM, Watson IR. Classifying BRAF alterations in cancer: new rational therapeutic strategies for actionable mutations. Oncogene (2018) 37:3183–99. doi: 10.1038/s41388-018-0171-x
38. Turski ML, Brady DC, Kim HJ, Kim B-E, Nose Y, Counter CM, et al. A novel role for copper in ras/mitogen-activated protein kinase signaling. Mol Cell Biol (2012) 32:1284–95. doi: 10.1128/MCB.05722-11
39. Brady DC, Crowe MS, Turski ML, Hobbs GA, Yao X, Chaikuad A, et al. Copper is required for oncogenic BRAF signalling and tumorigenesis. Nature (2014) 509:492–6. doi: 10.1038/nature13180
40. Voli F, Valli E, Lerra L, Kimpton K, Saletta F, Giorgi FM, et al. Intratumoral copper modulates PD-L1 expression and influences tumor immune evasion. Cancer Res (2020) 80:4129–44. doi: 10.1158/0008-5472.CAN-20-0471
41. Rigiracciolo DC, Scarpelli A, Lappano R, Pisano A, Santolla MF, De Marco P, et al. Copper activates HIF-1α/GPER/VEGF signalling in cancer cells. Oncotarget (2015) 6:34158–77. doi: 10.18632/oncotarget.5779
42. Babak MV, Ahn D. Modulation of intracellular copper levels as the mechanism of action of anticancer copper complexes: Clinical relevance. Biomedicines (2021) 9:852. doi: 10.3390/biomedicines9080852
43. da Silva DA, De Luca A, Squitti R, Rongioletti M, Rossi L, Machado CML, et al. Copper in tumors and the use of copper-based compounds in cancer treatment. J Inorg Biochem (2022) 226:111634. doi: 10.1016/j.jinorgbio.2021.111634
44. Leliévre P, Sancey L, Coll J, Deniaud A, Busser B. The multifaceted roles of copper in cancer: A trace metal element with dysregulated metabolism, but also a target or a bullet for therapy. Cancers (2020) 12:E3594. doi: 10.3390/cancers12123594
45. Denoyer D, Clatworthy SAS, Cater MA. Copper complexes in cancer therapy. Met Ions Life Sci (2018) 18:n. pag. doi: 10.1515/9783110470734-022
46. Brubaker C, Sturgeon P. Copper deficiency in infants; a syndrome characterized by hypocupremia, iron deficiency anemia, and hypoproteinemia. AMA J Dis Child (1956) 92:254–65. doi: 10.1001/ARCHPEDI.1956.02060030248002
47. Cordano A, Baertl J, Graham G. Copper deficiency in infancy. Pediatrics (1964) 34:324–36. doi: 10.1007/BF02761022
48. Ashkenazi A, Levin S, Djaldetti M, Fishel E, Benvenisti D. The syndrome of neonatal copper deficiency. Pediatrics (1973) 52:525–33. doi: 10.1542/peds.52.4.525
49. Parkin J, Cohen B. An overview of the immune system. Lancet Lond Engl (2001) 357:1777–89. doi: 10.1016/S0140-6736(00)04904-7
50. Iwasaki A, Medzhitov R. Control of adaptive immunity by the innate immune system. Nat Immunol (2015) 16:343–53. doi: 10.1038/ni.3123
51. Babu U, Failla ML. Respiratory burst and candidacidal activity of peritoneal macrophages are impaired in copper-deficient rats. J Nutr (1990) 120:1692–9. doi: 10.1093/jn/120.12.1692
52. Zhao H-J, Zhao X-H. Modulatory effect of the supplemented copper ion on In vitro activity of bovine lactoferrin to murine splenocytes and RAW264.7 macrophages. Biol Trace Elem Res (2019) 189:519–28. doi: 10.1007/s12011-018-1472-1
53. White C, Lee J, Kambe T, Fritsche K, Petris MJ. A role for the ATP7A copper-transporting ATPase in macrophage bactericidal activity. J Biol Chem (2009) 284:33949–56. doi: 10.1074/jbc.M109.070201
54. Stafford SL, Bokil NJ, Achard MES, Kapetanovic R, Schembri MA, McEwan AG, et al. Metal ions in macrophage antimicrobial pathways: emerging roles for zinc and copper. Biosci Rep (2013) 33:e00049. doi: 10.1042/BSR20130014
55. Johnson MDL, Kehl-Fie TE, Klein R, Kelly J, Burnham C, Mann B, et al. Role of copper efflux in pneumococcal pathogenesis and resistance to macrophage-mediated immune clearance. Infect Immun (2015) 83:1684–94. doi: 10.1128/IAI.03015-14
56. Mantovani A, Marchesi F, Malesci A, Laghi L, Allavena P. Tumour-associated macrophages as treatment targets in oncology. Nat Rev Clin Oncol (2017) 14:399–416. doi: 10.1038/nrclinonc.2016.217
57. Chatterjee S, Mookerjee A, Basu JM, Chakraborty P, Ganguly A, Adhikary A, et al. A novel copper chelate modulates tumor associated macrophages to promote anti-tumor response of T cells. PloS One (2009) 4:e7048. doi: 10.1371/journal.pone.0007048
58. Lukasewycz OA, Prohaska JR. Immunization against transplantable leukemia impaired in copper-deficient mice. J Natl Cancer Inst (1982) 69:489–93. doi: 10.1093/jnci/69.2.489
59. McMurray DN. Cell-mediated immunity in nutritional deficiency. Prog Food Nutr Sci (1984) 8:193–228.
60. Bala S, Failla ML. Copper repletion restores the number and function of CD4 cells in copper-deficient rats. J Nutr (1993) 123:991–6. doi: 10.1093/jn/123.6.991
61. Blakley BR, Hamilton DL. The effect of copper deficiency on the immune response in mice. Drug Nutr Interact (1987) 5:103–11.
62. Pedroni E, Bianchi E, Ugazio AG, Burgio GR. Letter: Immunodeficiency and steely hair. Lancet Lond Engl (1975) 1:1303–4. doi: 10.1016/s0140-6736(75)92598-2
63. Percival SS. Copper and immunity. Am J Clin Nutr (1998) 67:1064S–8S. doi: 10.1093/ajcn/67.5.1064S
64. Fields M, Lewis CG, Beal T, Scholfield D, Patterson K, Smith JC, et al. Sexual differences in the expression of copper deficiency in rats. Proc Soc Exp Biol Med Soc Exp Biol Med N Y N (1987) 186:183–7. doi: 10.3181/00379727-186-42600
65. Gender differences in immune competence during copper deficiency. Nutr Rev (1992) 50:114–6. doi: 10.1111/j.1753-4887.1992.tb01300.x
66. Heresi G, Castillo-Durán C, Muñoz C, Arevalo M, Schlesinger L. Phagocytosis and immunoglobulin levels in hypocupremic infants. Nutr Res (1985) 5:1327–34. doi: 10.1016/S0271-5317(85)80043-9
67. Kelley DS, Daudu PA, Taylor PC, Mackey BE, Turnlund JR. Effects of low-copper diets on human immune response. Am J Clin Nutr (1995) 62:412–6. doi: 10.1093/ajcn/62.2.412
68. de Jorge FB, Canato C, Delascio D. [Biochemical studies on fibroleiomyoma]. Matern E Infancia Arq Medicos-Sociais (1965) 24:649–54.
69. Schwartz MK, Schwartz MK. Role of trace elements in cancer. Cancer Res (1975) 35:3481–7. doi: 10.2307/3960603
70. Kaiafa GD, Saouli Z, Diamantidis MD, Kontoninas Z, Voulgaridou V, Raptaki M, et al. Copper levels in patients with hematological malignancies. Eur J Intern Med (2012) 23:738–41. doi: 10.1016/j.ejim.2012.07.009
72. Gupte A, Mumper RJ. Elevated copper and oxidative stress in cancer cells as a target for cancer treatment. Cancer Treat Rev (2009) 35:32–46. doi: 10.1016/j.ctrv.2008.07.004
73. Denoyer D, Masaldan S, La Fontaine S, Cater MA. Targeting copper in cancer therapy: ‘Copper that cancer.’. Metallomics (2015) 7:1459–76. doi: 10.1039/C5MT00149H
74. Martin NF, Kincaid MC, Stark WJ, Petty BG, Surer JL, Hirst LW, et al. Ocular copper deposition associated with pulmonary carcinoma, IgG monoclonal gammopathy and hypercupremia. a clinicopathologic correlation. Ophthalmology (1983) 90:110–6. doi: 10.1016/s0161-6420(83)34599-1
75. Hawkins AS, Stein RM, Gaines BI, Deutsch TA. Ocular deposition of copper associated with multiple myeloma. Am J Ophthalmol (2001) 131:257–9. doi: 10.1016/s0002-9394(00)00657-7
76. Aldave AJ, King JA, Kim BT, Hopp L. Corneal copper deposition associated with chronic lymphocytic leukemia. Am J Ophthalmol (2006) 142:174–6. doi: 10.1016/j.ajo.2006.01.078
77. Guo J, Cheng J, Zheng N, Zhang X, Dai X, Zhang L, et al. Copper promotes tumorigenesis by activating the PDK1-AKT oncogenic pathway in a copper transporter 1 dependent manner. Adv Sci Weinh Baden-Wurtt Ger (2021) 8:e2004303. doi: 10.1002/advs.202004303
78. Wong CC-L, Gilkes DM, Zhang H, Chen J, Wei H, Chaturvedi P, et al. Hypoxia-inducible factor 1 is a master regulator of breast cancer metastatic niche formation. Proc Natl Acad Sci U.S.A. (2011) 108:16369–74. doi: 10.1073/pnas.1113483108
79. Pez F, Dayan F, Durivault J, Kaniewski B, Aimond G, Le Provost GS, et al. The HIF-1-inducible lysyl oxidase activates HIF-1 via the akt pathway in a positive regulation loop and synergizes with HIF-1 in promoting tumor cell growth. Cancer Res (2011) 71:1647–57. doi: 10.1158/0008-5472.CAN-10-1516
80. Ge EJ, Bush AI, Casini A, Cobine PA, Cross JR, DeNicola GM, et al. Connecting copper and cancer: from transition metal signalling to metalloplasia. Nat Rev Cancer (2022) 22:102–13. doi: 10.1038/s41568-021-00417-2
81. Zhao Y, Adjei AA. Targeting angiogenesis in cancer therapy: Moving beyond vascular endothelial growth factor. Oncologist (2015) 20:660–73. doi: 10.1634/theoncologist.2014-0465
82. Sen CK, Khanna S, Venojarvi M, Trikha P, Ellison EC, Hunt TK, et al. Copper-induced vascular endothelial growth factor expression and wound healing. Am J Physiol Heart Circ Physiol (2002) 282:H1821–1827. doi: 10.1152/ajpheart.01015.2001
83. Demura Y, Ameshima S, Ishizaki T, Okamura S, Miyamori I, Matsukawa S. The activation of eNOS by copper ion (Cu2+) in human pulmonary arterial endothelial cells (HPAEC). Free Radic Biol Med (1998) 25:314–20. doi: 10.1016/s0891-5849(98)00056-2
84. Ashino T, Sudhahar V, Urao N, Oshikawa J, Chen G-F, Wang H, et al. Unexpected role of the copper transporter ATP7A in PDGF-induced vascular smooth muscle cell migration. Circ Res (2010) 107:787–99. doi: 10.1161/CIRCRESAHA.110.225334
85. La Mendola D, Farkas D, Bellia F, Magrì A, Travaglia A, Hansson Ö, et al. Probing the copper(II) binding features of angiogenin. similarities and differences between a n-terminus peptide fragment and the recombinant human protein. Inorg Chem (2012) 51:128–41. doi: 10.1021/ic201300e
86. Soncin F, Guitton JD, Cartwright T, Badet J. Interaction of human angiogenin with copper modulates angiogenin binding to endothelial cells. Biochem Biophys Res Commun (1997) 236:604–10. doi: 10.1006/bbrc.1997.7018
87. Kagan HM, Li W. Lysyl oxidase: properties, specificity, and biological roles inside and outside of the cell. J Cell Biochem (2003) 88:660–72. doi: 10.1002/jcb.10413
88. Zimnicka AM, Tang H, Guo Q, Kuhr FK, Oh M-J, Wan J, et al. Upregulated copper transporters in hypoxia-induced pulmonary hypertension. PloS One (2014) 9:e90544. doi: 10.1371/journal.pone.0090544
89. Urso E, Maffia M. Behind the link between copper and angiogenesis: Established mechanisms and an overview on the role of vascular copper transport systems. J Vasc Res (2015) 52:172–96. doi: 10.1159/000438485
90. Dongre A, Weinberg RA. New insights into the mechanisms of epithelial-mesenchymal transition and implications for cancer. Nat Rev Mol Cell Biol (2019) 20:69–84. doi: 10.1038/s41580-018-0080-4
91. El-Haibi CP, Bell GW, Zhang J, Collmann AY, Wood D, Scherber CM, et al. Critical role for lysyl oxidase in mesenchymal stem cell-driven breast cancer malignancy. Proc Natl Acad Sci U.S.A. (2012) 109:17460–5. doi: 10.1073/pnas.1206653109
92. Li S, Zhang J, Yang H, Wu C, Dang X, Liu Y. Copper depletion inhibits CoCl2-induced aggressive phenotype of MCF-7 cells via downregulation of HIF-1 and inhibition of Snail/Twist-mediated epithelial-mesenchymal transition. Sci Rep (2015) 5:12410. doi: 10.1038/srep12410
93. Erler JT, Bennewith KL, Cox TR, Lang G, Bird D, Koong A, et al. Hypoxia-induced lysyl oxidase is a critical mediator of bone marrow cell recruitment to form the premetastatic niche. Cancer Cell (2009) 15:35–44. doi: 10.1016/j.ccr.2008.11.012
94. Okkelman IA, Sukaeva AZ, Kirukhina EV, Korneenko TV, Pestov NB. Nuclear translocation of lysyl oxidase is promoted by interaction with transcription repressor p66β. Cell Tissue Res (2014) 358:481–9. doi: 10.1007/s00441-014-1972-z
95. MacDonald G, Nalvarte I, Smirnova T, Vecchi M, Aceto N, Dolemeyer A, et al. Memo is a copper-dependent redox protein with an essential role in migration and metastasis. Sci Signal (2014) 7:ra56. doi: 10.1126/scisignal.2004870
96. Feng W, Ye F, Xue W, Zhou Z, Kang YJ. Copper regulation of hypoxia-inducible factor-1 activity. Mol Pharmacol (2009) 75:174–82. doi: 10.1124/mol.108.051516
97. Armendariz AD, Gonzalez M, Loguinov AV, Vulpe CD. Gene expression profiling in chronic copper overload reveals upregulation of prnp and app. Physiol Genomics (2004) 20:45–54. doi: 10.1152/physiolgenomics.00196.2003
98. Acevedo KM, Hung YH, Dalziel AH, Li Q-X, Laughton K, Wikhe K, et al. Copper promotes the trafficking of the amyloid precursor protein. J Biol Chem (2011) 286:8252–62. doi: 10.1074/jbc.M110.128512
99. Gough M, Blanthorn-Hazell S, Delury C, Parkin E. The E1 copper binding domain of full-length amyloid precursor protein mitigates copper-induced growth inhibition in brain metastatic prostate cancer DU145 cells. Biochem Biophys Res Commun (2014) 453:741–7. doi: 10.1016/j.bbrc.2014.10.004
100. Badet J, Soncin F, Guitton JD, Lamare O, Cartwright T, Barritault D. Specific binding of angiogenin to calf pulmonary artery endothelial cells. Proc Natl Acad Sci U.S.A. (1989) 86:8427–31. doi: 10.1073/pnas.86.21.8427
101. Mandinov L, Mandinova A, Kyurkchiev S, Kyurkchiev D, Kehayov I, Kolev V, et al. Copper chelation represses the vascular response to injury. Proc Natl Acad Sci U.S.A. (2003) 100:6700–5. doi: 10.1073/pnas.1231994100
102. Prudovsky I, Bagala C, Tarantini F, Mandinova A, Soldi R, Bellum S, et al. The intracellular translocation of the components of the fibroblast growth factor 1 release complex precedes their assembly prior to export. J Cell Biol (2002) 158:201–8. doi: 10.1083/jcb.200203084
103. Crowe A, Jackaman C, Beddoes KM, Ricciardo B, Nelson DJ. Rapid copper acquisition by developing murine mesothelioma: decreasing bioavailable copper slows tumor growth, normalizes vessels and promotes T cell infiltration. PloS One (2013) 8:e73684. doi: 10.1371/journal.pone.0073684
104. Gundelach JH, Madhavan AA, Wettstein PJ, Bram RJ. The anticancer drug Dp44mT inhibits T-cell activation and CD25 through a copper-dependent mechanism. FASEB J Off Publ Fed Am Soc Exp Biol (2013) 27:782–92. doi: 10.1096/fj.12-215756
105. Kalinowski DS, Jansson PJ, Kovacevic Z, Richardson DR. The redox-active, anti-cancer drug Dp44mT inhibits T-cell activation and CD25 through a copper-dependent mechanism. Redox Rep Commun Free Radic Res (2013) 18:48–50. doi: 10.1179/1351000213Y.0000000041
106. Chakraborty P, Das S, Banerjee K, Sinha A, Roy S, Chatterjee M, et al. A copper chelate selectively triggers apoptosis in myeloid-derived suppressor cells in a drug-resistant tumor model and enhances antitumor immune response. Immunopharmacol Immunotoxicol (2014) 36:165–75. doi: 10.3109/08923973.2014.897727
107. Chang C-Y, Wu K-L, Chang Y-Y, Tsai P-H, Hung J-Y, Chang W-A, et al. Amine oxidase, copper containing 3 exerts anti−mesenchymal transformation and enhances CD4 t−cell recruitment to prolong survival in lung cancer. Oncol Rep (2021) 46:103. doi: 10.3892/or.2021.8154
108. Liu YL, Bager CL, Willumsen N, Ramchandani D, Kornhauser N, Ling L, et al. Tetrathiomolybdate (TM)-associated copper depletion influences collagen remodeling and immune response in the pre-metastatic niche of breast cancer. NPJ Breast Cancer (2021) 7:108. doi: 10.1038/s41523-021-00313-w
109. Iwai Y, Ishida M, Tanaka Y, Okazaki T, Honjo T, Minato N. Involvement of PD-L1 on tumor cells in the escape from host immune system and tumor immunotherapy by PD-L1 blockade. Proc Natl Acad Sci (2002) 99:12293–7. doi: 10.1073/pnas.192461099
110. Sammons S, Brady D, Vahdat L, Salama AK. Copper suppression as cancer therapy: the rationale for copper chelating agents in BRAFV600 mutated melanoma. Melanoma Manag (2016) 3:207–16. doi: 10.2217/mmt-2015-0005
111. Weekley CM, He C. Developing drugs targeting transition metal homeostasis. Curr Opin Chem Biol (2017) 37:26–32. doi: 10.1016/j.cbpa.2016.12.011
112. European Association for Study of Liver. EASL clinical practice guidelines: Wilson’s disease. J Hepatol (2012) 56:671–85. doi: 10.1016/j.jhep.2011.11.007
113. Pan Q, Bao LW, Merajver SD. Tetrathiomolybdate inhibits angiogenesis and metastasis through suppression of the NFkappaB signaling cascade. Mol Cancer Res MCR (2003) 1:701–6.
114. Doñate F, Juarez JC, Burnett ME, Manuia MM, Guan X, Shaw DE, et al. Identification of biomarkers for the antiangiogenic and antitumour activity of the superoxide dismutase 1 (SOD1) inhibitor tetrathiomolybdate (ATN-224). Br J Cancer (2008) 98:776–83. doi: 10.1038/sj.bjc.6604226
115. Gao D, Nolan D, McDonnell K, Vahdat L, Benezra R, Altorki N, et al. Bone marrow-derived endothelial progenitor cells contribute to the angiogenic switch in tumor growth and metastatic progression. Biochim Biophys Acta (2009) 1796:33–40. doi: 10.1016/j.bbcan.2009.05.001
116. Ishida S, Andreux P, Poitry-Yamate C, Auwerx J, Hanahan D. Bioavailable copper modulates oxidative phosphorylation and growth of tumors. Proc Natl Acad Sci U.S.A. (2013) 110:19507–12. doi: 10.1073/pnas.1318431110
117. Brewer GJ, Dick RD, Grover DK, LeClaire V, Tseng M, Wicha M, et al. Treatment of metastatic cancer with tetrathiomolybdate, an anticopper, antiangiogenic agent: Phase I study. Clin Cancer Res Off J Am Assoc Cancer Res (2000) 6:1–10.
118. Jain S, Cohen J, Ward MM, Kornhauser N, Chuang E, Cigler T, et al. Tetrathiomolybdate-associated copper depletion decreases circulating endothelial progenitor cells in women with breast cancer at high risk of relapse. Ann Oncol Off J Eur Soc Med Oncol (2013) 24:1491–8. doi: 10.1093/annonc/mds654
119. Redman BG, Esper P, Pan Q, Dunn RL, Hussain HK, Chenevert T, et al. Phase II trial of tetrathiomolybdate in patients with advanced kidney cancer. Clin Cancer Res Off J Am Assoc Cancer Res (2003) 9:1666–72.
120. Chwalinska-Sadowska H, Baum J. The effect of d-penicillamine on polymorphonuclear leukocyte function. J Clin Invest (1976) 58:871–9. doi: 10.1172/JCI108540
121. Jaffe IA. Comparison of the effect of plasmapheresis and penicillamine on the level of circulating rheumatoid factor. Ann Rheum Dis (1963) 22:71–6. doi: 10.1136/ard.22.2.71
122. Multicentre Trial Group. Controlled trial of d(-)penicillamine in severe rheumatoid arthritis. Lancet Lond Engl (1973) 1:275–80. doi: 10.1016/S0140-6736(73)91536-5
123. Jaffe IA. The effect of penicillamine on the laboratory parameters in rheumatoid arthritis. Arthritis Rheum (1965) 8:1064–79. doi: 10.1002/art.1780080606
124. Jaffe IA. Penicillamine treatment of rheumatoid arthritis: effect on immune complexes. Ann N Y Acad Sci (1975) 256:330–7. doi: 10.1111/j.1749-6632.1975.tb36059.x
125. Mohammed I, Barraclough D, Holborow EJ, Ansell BM. Effect of penicillamine therapy on circulating immune complexes in rheumatoid arthritis. Ann Rheum Dis (1975) 35:458–62. doi: 10.1136/ard.35.5.458
126. Epstein O, De Villiers D, Jain S, Potter BJ, Thomas HC, Sherlock S. Reduction of immune complexes and immunoglobulins induced by d-penicillamine in primary biliary cirrhosis. N Engl J Med (1979) 300:274–8. doi: 10.1056/NEJM197902083000602
127. Lipsky PE, Ziff M. Inhibition of human helper T cell function in vitro by d-penicillamine and CuSO4. J Clin Invest (1980) 65:1069–76. doi: 10.1172/JCI109759
128. Lewins EG, Cripps AW, Clancy RL, Major GA. Penicillamine-induced immunosuppression: in vitro studies of inhibition of immunoglobulin synthesis. J Rheumatol (1982) 9:677–84.
129. Lipsky PE. Modulation of human antibody production in vitro by d-penicillamine and CuSO4: inhibition of helper T cell function. J Rheumatol Suppl (1981) 7:69–73.
130. Lipsky PE. Immunosuppression by d-penicillamine in vitro. inhibition of human T lymphocyte proliferation by copper- or ceruloplasmin-dependent generation of hydrogen peroxide and protection by monocytes. J Clin Invest (1984) 73:53–65. doi: 10.1172/JCI111207
131. Lewins EG, Clancy RL, Cripps AW, Glass JS, Major GA. D-penicillamine induced suppression of b cell function: in vivo effect of d-penicillamine. J Rheumatol (1985) 12:685–91.
132. Mammoto T, Jiang A, Jiang E, Panigrahy D, Kieran MW, Mammoto A. Role of collagen matrix in tumor angiogenesis and glioblastoma multiforme progression. Am J Pathol (2013) 183:1293–305. doi: 10.1016/j.ajpath.2013.06.026
133. Goodman VL, Brewer GJ, Merajver SD. Copper deficiency as an anti-cancer strategy. Endocr Relat Cancer (2004) 11:255–63. doi: 10.1677/erc.0.0110255
134. Brem S, Grossman SA, Carson KA, New P, Phuphanich S, Alavi JB, et al. Phase 2 trial of copper depletion and penicillamine as antiangiogenesis therapy of glioblastoma. Neuro-Oncol (2005) 7:246–53. doi: 10.1215/S1152851704000869
135. Huang J, Chaudhary R, Cohen AL, Fink K, Goldlust S, Boockvar J, et al. A multicenter phase II study of temozolomide plus disulfiram and copper for recurrent temozolomide-resistant glioblastoma. J Neurooncol (2019) 142:537–44. doi: 10.1007/s11060-019-03125-y
136. Nechushtan H, Hamamreh Y, Nidal S, Gotfried M, Baron A, Shalev YI, et al. A phase IIb trial assessing the addition of disulfiram to chemotherapy for the treatment of metastatic non-small cell lung cancer. Oncologist (2015) 20:366–7. doi: 10.1634/theoncologist.2014-0424
137. Ramchandani D, Berisa M, Tavarez DA, Li Z, Miele M, Bai Y, et al. Copper depletion modulates mitochondrial oxidative phosphorylation to impair triple negative breast cancer metastasis. Nat Commun (2021) 12:7311. doi: 10.1038/s41467-021-27559-z
138. O’Day SJ, Eggermont AMM, Chiarion-Sileni V, Kefford R, Grob JJ, Mortier L, et al. Final results of phase III SYMMETRY study: randomized, double-blind trial of elesclomol plus paclitaxel versus paclitaxel alone as treatment for chemotherapy-naive patients with advanced melanoma. J Clin Oncol Off J Am Soc Clin Oncol (2013) 31:1211–8. doi: 10.1200/JCO.2012.44.5585
139. Monk BJ, Kauderer JT, Moxley KM, Bonebrake AJ, Dewdney SB, Secord AA, et al. A phase II evaluation of elesclomol sodium and weekly paclitaxel in the treatment of recurrent or persistent platinum-resistant ovarian, fallopian tube or primary peritoneal cancer: An NRG oncology/gynecologic oncology group study. Gynecol Oncol (2018) 151:422–7. doi: 10.1016/j.ygyno.2018.10.001
140. Tsvetkov P, Coy S, Petrova B, Dreishpoon M, Verma A, Abdusamad M, et al. Copper induces cell death by targeting lipoylated TCA cycle proteins. Science (2022) 375:1254–61. doi: 10.1126/science.abf0529
141. Cater MA, Pearson HB, Wolyniec K, Klaver P, Bilandzic M, Paterson BM, et al. Increasing intracellular bioavailable copper selectively targets prostate cancer cells. ACS Chem Biol (2013) 8:1621–31. doi: 10.1021/cb400198p
142. Duan L, Shen H, Zhao G, Yang R, Cai X, Zhang L, et al. Inhibitory effect of disulfiram/copper complex on non-small cell lung cancer cells. Biochem Biophys Res Commun (2014) 446:1010–6. doi: 10.1016/j.bbrc.2014.03.047
144. Brar SS, Grigg C, Wilson KS, Holder WD, Dreau D, Austin C, et al. Disulfiram inhibits activating transcription factor/cyclic AMP-responsive element binding protein and human melanoma growth in a metal-dependent manner in vitro, in mice and in a patient with metastatic disease. Mol Cancer Ther (2004) 3:1049–60. doi: 10.1158/1535-7163.1049.3.9
145. Li Y, Wang L-H, Zhang H-T, Wang Y-T, Liu S, Zhou W-L, et al. Disulfiram combined with copper inhibits metastasis and epithelial-mesenchymal transition in hepatocellular carcinoma through the NF-κB and TGF-β pathways. J Cell Mol Med (2018) 22:439–51. doi: 10.1111/jcmm.13334
146. Ding W-Q, Liu B, Vaught JL, Yamauchi H, Lind SE. Anticancer activity of the antibiotic clioquinol. Cancer Res (2005) 65:3389–95. doi: 10.1158/0008-5472.CAN-04-3577
147. Cater MA, Haupt Y. Clioquinol induces cytoplasmic clearance of the X-linked inhibitor of apoptosis protein (XIAP): therapeutic indication for prostate cancer. Biochem J (2011) 436:481–91. doi: 10.1042/BJ20110123
148. Tsvetkov P, Detappe A, Cai K, Keys HR, Brune Z, Ying W, et al. Mitochondrial metabolism promotes adaptation to proteotoxic stress. Nat Chem Biol (2019) 15:681–9. doi: 10.1038/s41589-019-0291-9
149. Soma S, Latimer AJ, Chun H, Vicary AC, Timbalia SA, Boulet A, et al. Elesclomol restores mitochondrial function in genetic models of copper deficiency. Proc Natl Acad Sci U.S.A. (2018) 115:8161–6. doi: 10.1073/pnas.1806296115
150. Nagai M, Vo NH, Shin Ogawa L, Chimmanamada D, Inoue T, Chu J, et al. The oncology drug elesclomol selectively transports copper to the mitochondria to induce oxidative stress in cancer cells. Free Radic Biol Med (2012) 52:2142–50. doi: 10.1016/j.freeradbiomed.2012.03.017
151. Zhang W, Chen C, Shi H, Yang M, Liu Y, Ji P, et al. Curcumin is a biologically active copper chelator with antitumor activity. Phytomed Int J Phytother Phytopharm (2016) 23:1–8. doi: 10.1016/j.phymed.2015.11.005
152. Nalepa G, Rolfe M, Harper JW. Drug discovery in the ubiquitin-proteasome system. Nat Rev Drug Discovery (2006) 5:596–613. doi: 10.1038/nrd2056
153. Zhang Z, Wang H, Yan M, Wang H, Zhang C. Novel copper complexes as potential proteasome inhibitors for cancer treatment (Review). Mol Med Rep (2017) 15:3–11. doi: 10.3892/mmr.2016.6022
154. Trejo-Solís C, Jimenez-Farfan D, Rodriguez-Enriquez S, Fernandez-Valverde F, Cruz-Salgado A, Ruiz-Azuara L, et al. Copper compound induces autophagy and apoptosis of glioma cells by reactive oxygen species and JNK activation. BMC Cancer (2012) 12:156. doi: 10.1186/1471-2407-12-156
155. Wang T, Liu Y, Fu Y, Huang T, Yang Y, Li S, et al. Antiproliferative activity of di-2-pyridylhydrazone dithiocarbamate acetate partly involved in p53 mediated apoptosis and autophagy. Int J Oncol (2017) 51:1909–19. doi: 10.3892/ijo.2017.4149
156. Holzer AK, Samimi G, Katano K, Naerdemann W, Lin X, Safaei R, et al. The copper influx transporter human copper transport protein 1 regulates the uptake of cisplatin in human ovarian carcinoma cells. Mol Pharmacol (2004) 66:817–23. doi: 10.1124/mol.104.001198
157. Noordhuis P, Laan AC, van de Born K, Losekoot N, Kathmann I, Peters GJ. Oxaliplatin activity in selected and unselected human ovarian and colorectal cancer cell lines. Biochem Pharmacol (2008) 76:53–61. doi: 10.1016/j.bcp.2008.04.007
158. Caffrey PB, Frenkel GD. Prevention of carboplatin-induced resistance in human ovarian tumor xenografts by selenite. Anticancer Res (2013) 33:4249–54.
159. Wallenberg M, Olm E, Hebert C, Björnstedt M, Fernandes AP. Selenium compounds are substrates for glutaredoxins: a novel pathway for selenium metabolism and a potential mechanism for selenium-mediated cytotoxicity. Biochem J (2010) 429:85–93. doi: 10.1042/BJ20100368
Keywords: copper deficiency, CTR-1, IL-2, PD-L1, copper ionophores, copper chelators, tumor immunity
Citation: Cheng F, Peng G, Lu Y, Wang K, Ju Q, Ju Y and Ouyang M (2022) Relationship between copper and immunity: The potential role of copper in tumor immunity. Front. Oncol. 12:1019153. doi: 10.3389/fonc.2022.1019153
Received: 14 August 2022; Accepted: 17 October 2022;
Published: 07 November 2022.
Edited by:
Massimo Broggini, Mario Negri Pharmacological Research Institute (IRCCS), ItalyCopyright © 2022 Cheng, Peng, Lu, Wang, Ju, Ju and Ouyang. This is an open-access article distributed under the terms of the Creative Commons Attribution License (CC BY). The use, distribution or reproduction in other forums is permitted, provided the original author(s) and the copyright owner(s) are credited and that the original publication in this journal is cited, in accordance with accepted academic practice. No use, distribution or reproduction is permitted which does not comply with these terms.
*Correspondence: Manzhao Ouyang, ouyangmanzhao@163.com
†These authors have contributed equally to this work