- Medical Oncology, IRCCS Azienda Ospedaliero-Universitaria di Bologna, Bologna, Italy
Hepatocellular carcinoma (HCC) remains the sixth most commonly diagnosed malignancy worldwide, still representing an important cause of cancer-related death. Over the next few years, novel systemic treatment options have emerged. Among these, immune checkpoint inhibitors (ICIs) have been widely evaluated and are under assessment, as monotherapy or in combination with other anticancer agents in treatment-naïve and previously treated patients. In particular, the approval of the PD-L1 inhibitor atezolizumab plus the antiangiogenic agent bevacizumab as front-line treatment for advanced HCC has led to the adoption of this combination in this setting, and the IMbrave 150 phase III trial has established a novel standard of care. However, several questions remain unanswered, including the identification of reliable predictors of response to ICIs in HCC patients. In the current paper, we will provide an updated overview of potentially useful predictive biomarkers of response to immunotherapy in advanced HCC. A literature search was conducted in September 2021 of Pubmed/Medline, Cochrane library and Scopus databases.
Introduction
Hepatocellular carcinoma (HCC) remains a lethal tumor with a 5-year survival rate of less than 20% for patients at all stages, accounting for more than 75% of all primary liver malignancies and resulting in 83 million deaths in 2020 (1, 2). A number of liver diseases has been historically associated with the onset of HCC, including liver cirrhosis, hepatitis virus infection, and non-alcoholic liver disease (NAFLD), with some of these etiologies that have been suggested to play a role in modifying responses to systemic treatments (3, 4). Potentially curative treatment options for patients with limited disease [encompassing early-stage Barcelona Clinic Liver Cancer (BCLC) 0/A and intermediate-stage BCLC B HCC] include radical surgery, liver transplantation, and locoregional approaches such as chemoembolization and radiofrequency ablation, while BCLC C patients usually receive systemic therapies (5–9). We have recently witnessed the emerging and advent of immune checkpoint inhibitors (ICIs) in a wide number of hematological and solid tumors, and these agents have caused unprecedented treatment paradigm shifts in a relatively short period of time (10–12). ICIs are able to contrast immune checkpoint-related molecules - including programmed cell death-1 (PD-1), cytotoxic T-lymphocyte-associated antigen 4 (CTLA-4), lymphocyte-activation gene 3 (LAG-3) (13, 14) – and these agents have been recently tested and are under evaluation in HCC patients. While results of ICI monotherapy have been disappointing so far (15–17), the recently presented and published phase III IMbrave150 study comparing the PD-L1 inhibitor atezolizumab plus bevacizumab versus sorafenib monotherapy as front-line treatment approach for advanced HCC patients has established a new standard of care (18–20). In particular, patients receiving the atezolizumab plus bevacizumab combination showed impressive benefits in terms of several endpoints, such as progression-free survival (PFS), overall survival (OS), objective response rate (ORR) and complete response (CR) rate, as also confirmed by the updated results presented by Finn and colleagues reporting a median OS of 19.2 months in the experimental arm (21, 22). These results are destined to mark a new era in this setting, and ICIs seem to have found their role in advanced HCC patients in combinatorial strategies (23); however, the use of immunotherapy in HCC has been associated with several limitations in this setting (24). In particular, the identification of predictive biomarkers is urgently needed to guide the therapeutic process since approximately half of advanced HCC patients treated with ICIs do not benefit from this therapeutic approach (25–27). Thus, a detailed discussion and comprehension of this issue is of pivotal importance, and several potential predictors such as programmed death ligand 1 (PD-L1), tumor mutational burden (TMB), gut microbiota and several others are under assessment (28–30). We performed a research on Pubmed/Medline, Cochrane library and Scopus using the following keywords “HCC” OR “hepatocellular carcinoma” OR “liver cancer” AND “PD-L1” OR “TMB” OR “MSI” OR “MMR” OR “DDR” OR “gut microbiome” OR “predictive biomarkers” OR “predictors of response” AND “pembrolizumab” OR “PD-L1” OR “immune checkpoint inhibitors” OR “immunotherapy” OR “PD-1 inhibitor” OR “atezolizumab” OR “durvalumab” OR “tremelimumab” OR “nivolumab”.
PD-L1
As a matter of fact, we have recently witnessed the approval of several ICIs according to PD-L1 expression in different malignancies, as in the case of the PD-1 inhibitor pembrolizumab in previously untreated patients with advanced non-small cell lung cancer (NSCLC) with PD-L1 Tumor Proportion Score (TPS) of 50% or more (31–33). However, PD-L1 assessment presents several issues, such as the use of different antibody clones as well as distinct cut-off thresholds (34, 35); in addition, PD-L1 is determined on tumor cells or immune infiltrating cells, something that further limits the standardization of this biomarker across different tumor types (36, 37).
As regards the field of HCC, around 10% of tumor cells have been suggested to express PD-L1, with this biomarker reporting a putative prognostic value (38); in particular, PD-L1 positive HCCs have been associated with shorter survival and recurrence-free survival, with recent retrospective trials reporting a possible link between macrovascular invasion, poorly differentiated or undifferentiated disease, high serum alfa-fetoprotein (AFP), and PD-L1 expression (39). In terms of predictive value, few data are available so far regarding the role of PD-L1 status in advanced HCC patients receiving ICIs as monotherapy or part of combinatorial strategies; in addition, not only these data are sparse, but are also conflicting. For example, the phase III CheckMate 459 trial comparing nivolumab monotherapy versus sorafenib as front-line treatment showed higher response rate in PD-L1 positive advanced HCCs treated with immunotherapy (40); similarly, the KEYNOTE-224 study on pembrolizumab observed that PD-L1 positive expression was associated with improved response in patients receiving ICI monotherapy (41). On the other hand, in the phase I/II CheckMate 040 study including 174 advanced HCCs with available PD-L1 status, a non-statistically significant difference was reported between PD-L1 positive and PD-L1 negative patients treated with nivolumab (26% versus 19% in PD-L1 positive and PD-L1 negative HCCs, respectively, by using a cut-off value of 1% of tumor cells) (42). These controversial results observed across different trials on ICIs are not particularly surprising since all these studies were not adequately powered to differentiate responders and non-responders according to PD-L1 expression. In addition, and similar to other solid tumors, the current lack of validated and standardized methodologies and cut-off values clearly evaluating PD-L1 status further complicates this scenario, also considering the dynamic nature of PD-L1 due to its high spatial and temporal heterogeneity (43–45). Lastly, responses to ICIs have been observed in PD-L1 positive and PD-L1 negative patients, with this evidence calling into question the effective utility of this biomarker in this setting.
TMB and MMR/MSI
The term tumor mutational burden (TMB) is commonly used to quantify the number of nonsynonymous mutations observed in the tumoral genome (46, 47), and tumors with higher number of mutations have been associated with the production of higher levels of neoantigens and enhanced immune response (48, 49). Recent years have witnessed the presentation and publication of several trials specifically focused on the association between high TMB and response to immunotherapy, with the former suggested to be a predictive biomarker in this setting (50, 51). However, TMB has not been validated so far and its use remains investigational.
As regards immunotherapy in HCC, the role of high TMB in modifying and determining response to ICIs as monotherapy or in combination with other anticancer agents remains to be elucidated (Figure 1) (47, 52). Of note, some recent studies have reported a median of 4-5 mutations per megabase in HCC patients (53); in particular, Ang and colleagues recently investigated the prevalence of TMB across 755 HCC patients, and only 0.8% of included subjects presented high TMB, with the same trial reporting no association between high TMB and response to ICIs (54). Another interesting research avenue regards the suggested differences in terms of TMB across geographical areas and according to ethnicity; in fact, in a study by Tang and colleagues the authors reported that Chinese patients with HCC presented higher median TMB compared with patients from Western areas (9.3% and 1%, respectively) (55). Another recent study by Wong et al. performed targeted Next Generation Sequencing (NGS) on archival and fresh samples of 29 HCCs (56); of note, the trial highlighted lower TMB in fresh samples, with a median of 958.39 mutations per megabase in archival samples versus 2.51 mutations per megabase in fresh samples. Therefore, the authors suggested that fresh HCC samples could represent a better source of tumor DNA compared with archival samples (56).
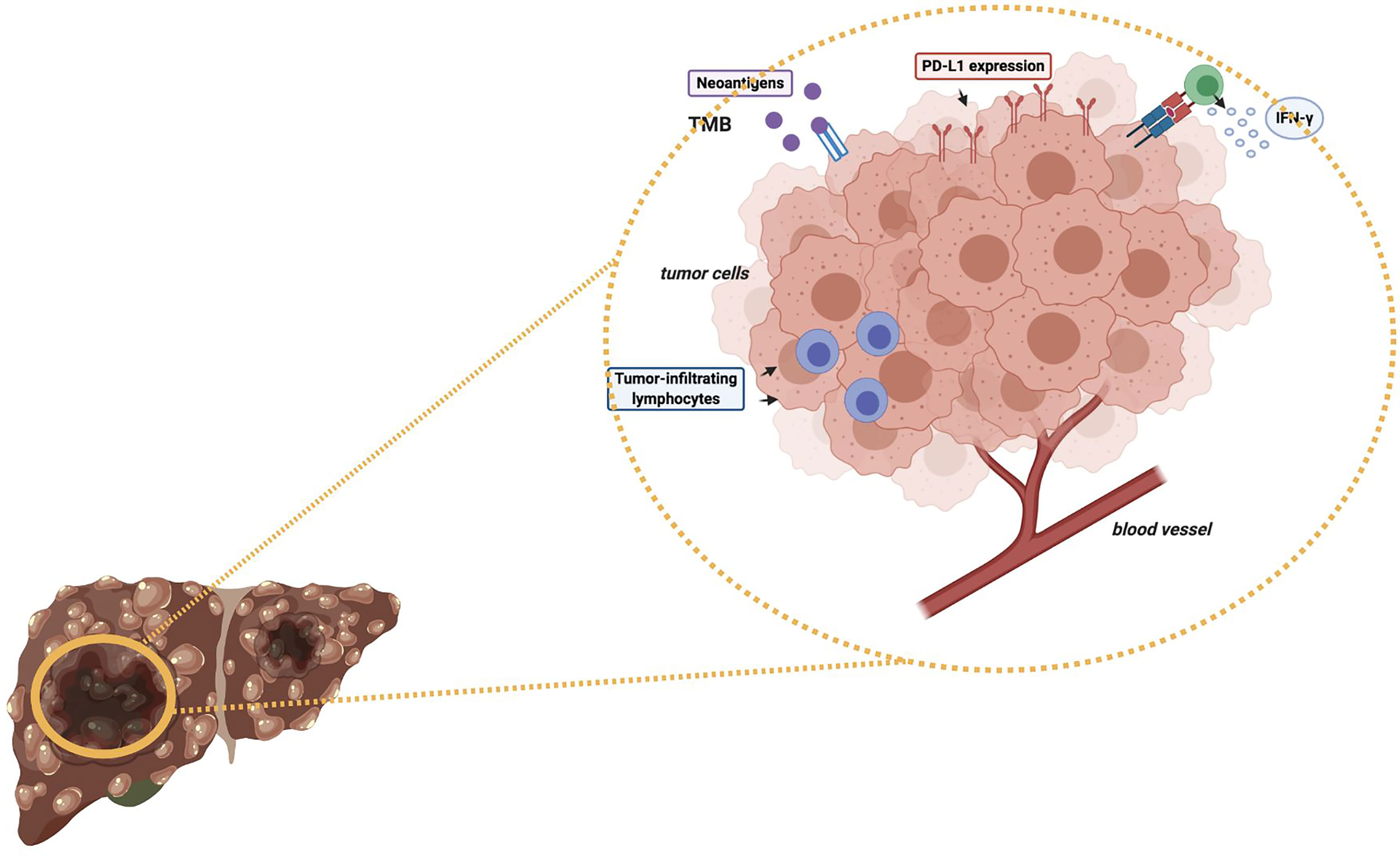
Figure 1 Schematic figure representing some potential predictive biomarkers of response to immunotherapy in HCC patients. TMB, Tumor Mutational Burden; PD-L1, Programmed Death-Ligand 1; IFN-γ, Interferon-gamma.
Another potentially meaningful predictor under assessment in cancer immunotherapy is microsatellite instability (MSI) status, as witnessed by landmark studies published over the last decade (57, 58). In fact, several trials have reported that deficiencies in DNA mismatch repair (MMR) may cause a “hypermutator” phenotype commonly defined as microsatellite instability (MSI) (59, 60); in particular, loss of mutations in MMR-related genes including MLH1, MSH2, MSH6, and PMS2 impair the MMR system (61, 62). In addition, high mutation rates in somatic cells have been suggested to boost the neoantigen load, resulting in lymphocyte activation and consequent increase of the efficacy of cancer immunotherapy. Despite the use of MSI has entered into the clinical practice leading to the use of ICIs as first-line (e.g., colorectal cancer) or in second- and later-line, available literature regarding the role of MSI as a predictive biomarker in HCC patients receiving ICIs is mainly based on case reports (63). Of note, MSI-H is deemed to be quite a rare finding, with retrospective studies reporting a proportion of approximately 3% of cases (64, 65). In the previously cited study, Ang and colleagues highlighted that only 0.2% of included HCC patients were MSI-H and had high TMB, suggesting that “hypermutated phenotypes” seem to be a rare evidence in this primary liver tumor (54). The role of MSI-H in this setting remains to be fully elucidated.
Gene Mutations
The advent of molecular profiling has produced notable paradigm shifts in the management of several hematological and solid tumors (57, 66). Of note, not only several genetic mutations have been suggested to represent a therapeutic target and to identify “oncogene-addicted disease”, but some of these aberrations have been also associated with response to ICIs (67, 68).
As regards HCC, a recent study by Harding et al. performed NGS to evaluate the association between systemic treatments (such as PD-1 inhibitors, CTLA-4 inhibitors, anti-LAG3, etc.) and genetic aberrations including Wnt/β-catenin mutations (69). Interestingly, in 31 patients with advanced HCC receiving heterogeneous schedules and types of ICIs, Wnt/β-catenin mutated HCCs reported a statistically significant association with resistance to immunotherapy and shorter median OS and PFS compared with wild-type patients, with a median OS of 9.1 and 15.2 months, and a median PFS of 2.0 months and 7.4 months, respectively (69). In addition, around half of wild-type HCCs reported a response to ICIs, while no responses were reported in patients with Wnt/β-catenin mutations (69). This evidence has been also confirmed by other trials on this topic, with malignancies harboring β-catenin aberrations reporting a putative resistance to immunotherapy in mouse models (70, 71).
Among the most commonly highlighted genetic mutations in HCC, TP53 gene mutations are mutually exclusive with CTNBB1 mutations and HCCs with these aberrations have been suggested to have specific features in terms of tumor microenvironment (TME) (72); in fact, according to recent studies these malignancies report higher Foxp3+ Treg infiltration and lower CD8+ T cell infiltration (73). At the same time, it is worth noting that these results remain preliminary, with other trials reporting better clinical outcomes and higher tumor-infiltrating lymphocytes (TILs) in HCC patients with TP53 mutations (74, 75).
DNA damage repair (DDR) gene alterations have recently emerged as potential predictive biomarkers of response to immunotherapy due to the genomic instability associated with these aberrations (76, 77). In fact, alterations in DDR genes have been involved in the impairment of DNA repair processes, hesitating in accumulation of DNA damages due to genomic stability loss (78, 79). Despite several preclinical trials have highlighted a synergistic effect for PARP inhibitors plus immunotherapy in cancer patients with these aberrations, no data are available regarding this approach in advanced HCC so far (80, 81).
Immune-related long noncoding RNAs (lncRNAs) represents another emerging line of research in the field of predictors of response to immunotherapy (82, 83). Interestingly, a trial by Peng et al. suggested an association between PD-L1 and CTLA-4 with the lncRNA MIR155 host gene, and this gene seems to appear as a promising predictive biomarker of response to ICIs (84). Moreover, recent trials have highlighted that HCC patients with specific immune-related lncRNAs signatures may present enhanced immune cell infiltration and high PD-L1, PD-L2, and IDO1 expression (85). Despite the use of lncRNAs seems promising, the application of these potential predictors remains far from everyday clinical practice.
Gut Microbiota
Several novel focuses of research are under active development, including gut microbiome (86–89); for example, Zheng et al. recently suggested dynamic changes occurring in the gut microbiome during immunotherapy in HCC patients (90). In this interesting report, fecal samples from patients responding to ICIS showed higher taxa richness compared to fecal samples of non-responders, suggesting that human microbiota could have a notable impact on responses to immunotherapy in HCC patients (90). Despite the application of gut microbiota in modulating response to immunotherapy remains far from clinical practice, the landmark role of gut microbiota in modulating innate and adaptive immunity supports makes this research avenue particularly promising (91–96).
Future Perspectives
Recent years have seen the advent of immunotherapy, and the identification of specific histological and molecular predictors of response to ICIs represents one of the current and future challenges in several tumor types, including HCC (97). In fact, only a proportion of HCC patients seems to benefit from immunotherapy, highlighting the need for a deeper understanding of predictors of response. In the near future, the HCC medical community is called to more efforts aimed at evaluating novel biomarkers of response to ICIs, considering tumor-intrinsic (e.g., PD-L1 expression, TMB, MSI status, etc.), immune-specific, and combinatorial biomarkers. In fact, a combination of PD-L1 with other biomarkers would probably be more impactful compared to a single predictor of response.
The identification of predictive biomarkers of response to immunotherapy remains a priority in HCC, especially considering that the number of indications and patients receiving ICIs is supposed to further increase in the near future. Thus, the question whether PD-L1 expression, TMB and other potential predictors could be applied to select the right patients to receive immunotherapy and to provide useful information for disease-monitoring and treatment-decision making remains critical. Further investigations are warranted in this direction.
Author Contributions
All authors contributed to the article and approved the submitted version.
Conflict of Interest
The authors declare that the research was conducted in the absence of any commercial or financial relationships that could be construed as a potential conflict of interest.
Publisher’s Note
All claims expressed in this article are solely those of the authors and do not necessarily represent those of their affiliated organizations, or those of the publisher, the editors and the reviewers. Any product that may be evaluated in this article, or claim that may be made by its manufacturer, is not guaranteed or endorsed by the publisher.
References
1. Forner A, Reig M and Bruix J. Hepatocellular Carcinoma. Lancet (2018) 391(10127):1301–14. doi: 10.1016/S0140-6736(18)30010-2
2. Villanueva A. Hepatocellular Carcinoma. N Engl J Med (2019) 380(15):1450–62. doi: 10.1056/NEJMra1713263
3. Pfister D, Núñez NG, Pinyol R, Govaere O, Pinter M, Szydlowska M, et al. NASH Limits Anti-Tumour Surveillance in Immunotherapy-Treated HCC. Nat (2021) 592(7854):450–6. doi: 10.1038/s41586-021-03362-0
4. Waidmann O, Trojan J. Novel Drugs in Clinical Development for Hepatocellular Carcinoma. Expert Opin Investig Drugs (2015) 24(8):1075–82. doi: 10.1517/13543784.2015.1058776
5. Noonan A, Pawlik TM. Hepatocellular Carcinoma: An Update on Investigational Drugs in Phase I and II Clinical Trials. Expert Opin Investig Drugs (2019) 28(11):941–9. doi: 10.1080/13543784.2019.1677606
6. Faivre S, Rimassa L, Finn RS. Molecular Therapies for HCC: Looking Outside the Box. J Hepatol (2020) 72(2):342–52. doi: 10.1016/j.jhep.2019.09.010
7. Rizzo A, Brandi G. Biochemical Predictors of Response to Immune Checkpoint Inhibitors in Unresectable Hepatocellular Carcinoma. Cancer Treat Res Commun (2021) 27:100328. doi: 10.1016/j.ctarc.2021.100328
8. Llovet JM, Montal R, Sia D, Finn RS. Molecular Therapies and Precision Medicine for Hepatocellular Carcinoma. Nat Rev Clin Oncol (2018) 15(10):599–616. doi: 10.1038/s41571-018-0073-4
9. Rizzo A, Ricci AD, Brandi G. Immune-Based Combinations for Advanced Hepatocellular Carcinoma: Shaping the Direction of First-Line Therapy. Future Oncol (2021) 17(7):755–7. doi: 10.2217/fon-2020-0986
10. Reck M. Pembrolizumab as First-Line Therapy for Metastatic Non-Small-Cell Lung Cancer. Immunother (2018) 10(2):93–105. doi: 10.2217/imt-2017-0121
11. Reck M, Rodríguez-Abreu D, Robinson AG, Hui R, Csoszi T, Fülöp A, et al. Updated Analysis of KEYNOTE-024: Pembrolizumab Versus Platinum-Based Chemotherapy for Advanced Non-Small-Cell Lung Cancer With PD-L1 Tumor Proportion Score of 50% or Greater. J Clin Oncol (2019) 37(7):537–46. doi: 10.1200/JCO.18.00149
12. Paz-Ares L, Spira A, Raben D, Planchard D, Cho BC, Özgüroglu M, et al. Outcomes With Durvalumab by Tumour PD-L1 Expression in Unresectable, Stage III Non-Small-Cell Lung Cancer in the PACIFIC Trial. Ann Oncol (2020) 31(6):798–806. doi: 10.1016/j.annonc.2020.03.287
13. Ingles Garces AH, Au L, Mason R, Thomas J, Larkin J. Building on the Anti-PD1/PD-L1 Backbone: Combination Immunotherapy for Cancer. Expert Opin Investig Drugs (2019) 28(8):695–708. doi: 10.1080/13543784.2019.1649657
14. Alsaab HO, Sau S, Alzhrani R, Tatiparti K, Bhise K, Kashaw SK, et al. PD-1 and PD-L1 Checkpoint Signaling Inhibition for Cancer Immunotherapy: Mechanism, Combinations, and Clinical Outcome. Front Pharmacol (2017) 8:561. doi: 10.3389/fphar.2017.00561
15. Cheng AL, Hsu C, Chan SL, Choo SP, Kudo M. Challenges of Combination Therapy With Immune Checkpoint Inhibitors for Hepatocellular Carcinoma. J Hepatol (2020) 72(2):307–19. doi: 10.1016/j.jhep.2019.09.025
16. Finkelmeier F, Waidmann O, Trojan J. Nivolumab for the Treatment of Hepatocellular Carcinoma. Expert Rev Anticancer Ther (2018) 18(12):1169–75. doi: 10.1080/14737140.2018.1535315
17. Pinter M, Jain RK, Duda DG. The Current Landscape of Immune Checkpoint Blockade in Hepatocellular Carcinoma: A Review. JAMA Oncol (2021) 7(1):113–23. doi: 10.1001/jamaoncol.2020.3381
18. Kudo M. Scientific Rationale for Combined Immunotherapy With PD-1/PD-L1 Antibodies and VEGF Inhibitors in Advanced Hepatocellular Carcinoma. Cancers (Basel) (2020) 12(5):1089. doi: 10.3390/cancers12051089
19. Finn RS, Qin S, Ikeda M, Galle PR, Ducreux M, Kim TY, et al. IMbrave150 Investigators. Atezolizumab Plus Bevacizumab in Unresectable Hepatocellular Carcinoma. N Engl J Med (2020) 382(20):1894–905. doi: 10.1056/NEJMoa1915745
20. Kelley RK. Atezolizumab Plus Bevacizumab - A Landmark in Liver Cancer. N Engl J Med (2020) 382(20):1953–5. doi: 10.1056/NEJMe2004851
21. Rizzo A, Ricci AD, Brandi G. Atezolizumab in Advanced Hepatocellular Carcinoma: Good Things Come to Those Who Wait. Immunother (2021) 13(8):637–44. doi: 10.2217/imt-2021-0026
22. Finn RS, Qin S, Ikeda M, Galle PR, Ducreux M, Kim T-Y, et al. IMbrave150: Updated Overall Survival (OS) Data From a Global, Randomized, Open-Label Phase III Study of Atezolizumab (Atezo) + Bevacizumab (Bev) Versus Sorafenib (Sor) in Patients (Pts) With Unresectable Hepatocellular Carcinoma (HCC). J Clin Oncol (2021) 3_suppl, 267-267:39. doi: 10.1200/JCO.2021.39.3_suppl.267
23. Kelley RK, Sangro B, Harris W, Ikeda M, Okusaka T, Kang YK, et al. Safety, Efficacy, and Pharmacodynamics of Tremelimumab Plus Durvalumab for Patients With Unresectable Hepatocellular Carcinoma: Randomized Expansion of a Phase I/II Study. J Clin Oncol (2021) 39(27):2991–3001. doi: 10.1200/JCO.20.03555
24. Park R, Eshrat F, Al-Jumayli M, Saeed A, Saeed A. Immuno-Oncotherapeutic Approaches in Advanced Hepatocellular Carcinoma. Vaccines (Basel) (2020) 8(3):447. doi: 10.3390/vaccines8030447
25. Pinato DJ, Guerra N, Fessas P, Murphy R, Mineo T, Mauri FA, et al. Immune-Based Therapies for Hepatocellular Carcinoma. Oncogene (2020) 39(18):3620–37. doi: 10.1038/s41388-020-1249-9
26. Boilève A, Hilmi M, Delaye M, Tijeras-Raballand A, Neuzillet C. Biomarkers in Hepatobiliary Cancers: What is Useful in Clinical Practice? Cancers (Basel) (2021) 13(11):2708. doi: 10.3390/cancers13112708
27. Rizzo A, Dadduzio V, Ricci AD, Massari F, Di Federico A, Gadaleta-Caldarola G, et al. Lenvatinib Plus Pembrolizumab: The Next Frontier for the Treatment of Hepatocellular Carcinoma? Expert Opin Investig Drugs (2021) 30:1–8. doi: 10.1080/13543784.2021.1948532
28. Zhang T, Merle P, Wang H, Zhao H, Kudo M. Combination Therapy for Advanced Hepatocellular Carcinoma: Do We See the Light at the End of the Tunnel? Hepatobiliary Surg Nutr (2021) 10(2):180–92. doi: 10.21037/hbsn-2021-7
29. Zayac A, Almhanna K. Hepatobiliary Cancers and Immunotherapy: Where are We Now and Where are We Heading? Transl Gastroenterol Hepatol (2020) 5:8. doi: 10.21037/tgh.2019.09.07
30. Sangro B, Sarobe P, Hervás-Stubbs S, Melero I. Advances in Immunotherapy for Hepatocellular Carcinoma. Nat Rev Gastroenterol Hepatol (2021) 13:1–19. doi: 10.1038/s41575-021-00438-0
31. Houssaini MS, Damou M, Ismaili N. Advances in the Management of Non-Small Cell Lung Cancer (NSCLC): A New Practice Changing Data From Asco 2020 Annual Meeting. Cancer Treat Res Commun (2020) 25:100239. doi: 10.1016/j.ctarc.2020.100239
32. Ghanem S, Valecha GK, Hossri S, Ibrahim U, Atallah JP. Investigational PD-1 Inhibitors for Advanced Non-Small Lung Cancer: New Players in a Shifting Paradigm. Expert Opin Investig Drugs (2017) 26(12):1317–9. doi: 10.1080/13543784.2017.1395019
33. Rocco D, Gregorc V, Della Gravara L, Lazzari C, Palazzolo G, Gridelli C. New Immunotherapeutic Drugs in Advanced Non-Small Cell Lung Cancer (NSCLC): From Preclinical to Phase I Clinical Trials. Expert Opin Investig Drugs (2020) 29(9):1005–23. doi: 10.1080/13543784.2020.1793956
34. Naso JR, Banyi N, Al-Hashami Z, Zhu J, Wang G, Ionescu DN, et al. Discordance in PD-L1 Scores on Repeat Testing of Non-Small Cell Lung Carcinomas. Cancer Treat Res Commun (2021) 27:100353. doi: 10.1016/j.ctarc.2021.100353
35. Heimes AS, Schmidt M. Atezolizumab for the Treatment of Triple-Negative Breast Cancer. Expert Opin Investig Drugs (2019) 28(1):1–5. doi: 10.1080/13543784.2019.1552255
36. Ilie M, Hofman V, Dietel M, Soria JC, Hofman P. Assessment of the PD-L1 Status by Immunohistochemistry: Challenges and Perspectives for Therapeutic Strategies in Lung Cancer Patients. Virchows Arch (2016) 468(5):511–25. doi: 10.1007/s00428-016-1910-4
37. Zhang S, Bai X, Shan F. The Progress and Confusion of Anti-PD1/PD-L1 Immunotherapy for Patients With Advanced Non-Small Cell Lung Cancer. Int Immunopharmacol (2020) 80:106247. doi: 10.1016/j.intimp.2020.106247
38. Lei J, Zhang D, Yao C, Ding S, Lu Z. Development of a Predictive Immune-Related Gene Signature Associated With Hepatocellular Carcinoma Patient Prognosis. Cancer Control (2020) 27(1):1073274820977114. doi: 10.1177/1073274820977114
39. Calderaro J, Rousseau B, Amaddeo G, Mercey M, Charpy C, Costentin C, et al. Programmed Death Ligand 1 Expression in Hepatocellular Carcinoma: Relationship With Clinical and Pathological Features. Hepatology (2016) 64(6):2038–46. doi: 10.1002/hep.28710
40. Yau T, Park J, Finn R, Cheng AL, Mathurin P, Edeline J, et al. CheckMate 459, A Randomized, Multi-Center Phase III Study of Nivolumab (NIVO) vs Sorafenib (SOR) as First-Line (1L) Treatment in Patients (Pts) With Advanced Hepatocellular Carcinoma (aHCC). Ann Oncol (2019) 30:v874–5. doi: 10.1093/annonc/mdz394.029
41. Zhu AX, Finn RS, Edeline J, Cattan S, Ogasawara S, Palmer D, et al. KEYNOTE-224 investigators. Pembrolizumab in Patients With Advanced Hepatocellular Carcinoma Previously Treated With Sorafenib (KEYNOTE-224): A Non-Randomised, Open-Label Phase 2 Trial. Lancet Oncol (2018) 19(7):940–52. doi: 10.1016/S1470-2045(18)30351-6
42. El-Khoueiry AB, Sangro B, Yau T, Crocenzi TS, Kudo M, Hsu C, et al. Nivolumab in Patients With Advanced Hepatocellular Carcinoma (CheckMate 040): An Open-Label, Non-Comparative, Phase 1/2 Dose Escalation and Expansion Trial. Lancet (2017) 24389(10088):2492–502. doi: 10.1016/S0140-6736(17)31046-2
43. Macek Jilkova Z, Aspord C, Decaens T. Predictive Factors for Response to PD-1/PD-L1 Checkpoint Inhibition in the Field of Hepatocellular Carcinoma: Current Status and Challenges. Cancers (Basel) (2019) 11(10):1554. doi: 10.3390/cancers11101554
44. McLaughlin J, Han G, Schalper KA, Carvajal-Hausdorf D, Pelekanou V, Rehman J, et al. Quantitative Assessment of the Heterogeneity of PD-L1 Expression in Non-Small-Cell Lung Cancer. JAMA Oncol (2016) 2(1):46–54. doi: 10.1001/jamaoncol.2015.3638
45. Dodson A, Parry S, Lissenberg-Witte B, Haragan A, Allen D, O'Grady A, et al. External Quality Assessment Demonstrates That PD-L1 22C3 and SP263 Assays are Systematically Different. J Pathol Clin Res (2020) 6(2):138–45. doi: 10.1002/cjp2.153
46. Uson Junior PLS, Nagalo BM, Ahn DH, Bekaii-Saab T, Borad MJ. Combination Immunotherapy for Hepatocellular Carcinoma: Where Are We Currently? Semin Liver Dis (2021) 41(2):136–41. doi: 10.1055/s-0040-1722646
47. Samstein RM, Lee CH, Shoushtari AN, Hellmann MD, Shen R, Janjigian YY, et al. Tumor Mutational Load Predicts Survival After Immunotherapy Across Multiple Cancer Types. Nat Genet (2019) 51(2):202–6. doi: 10.1038/s41588-018-0312-8
48. Chan TA, Yarchoan M, Jaffee E, Swanton C, Quezada SA, Stenzinger A, et al. Development of Tumor Mutation Burden as an Immunotherapy Biomarker: Utility for the Oncology Clinic. Ann Oncol (2019) 30(1):44–56. doi: 10.1093/annonc/mdy495
49. van den Bulk J, Verdegaal EM, de Miranda NF. Cancer Immunotherapy: Broadening the Scope of Targetable Tumours. Open Biol (2018) 8(6):180037. doi: 10.1098/rsob.180037
50. Pardoll DM. The Blockade of Immune Checkpoints in Cancer Immunotherapy. Nat Rev Cancer (2012) 12(4):252–64. doi: 10.1038/nrc3239
51. Merino DM, McShane LM, Fabrizio D, Funari V, Chen SJ, White JR, et al. TMB Harmonization Consortium; Establishing Guidelines to Harmonize Tumor Mutational Burden (TMB): In Silico Assessment of Variation in TMB Quantification Across Diagnostic Platforms: Phase I of the Friends of Cancer Research TMB Harmonization Project. J Immunother Cancer (2020) 8(1):e000147. doi: 10.1136/jitc-2019-000147
52. Shrestha R, Prithviraj P, Anaka M, Bridle KR, Crawford DHG, Dhungel B, et al. Monitoring Immune Checkpoint Regulators as Predictive Biomarkers in Hepatocellular Carcinoma. Front Oncol (2018) 8:269. doi: 10.3389/fonc.2018.00269
53. Liu L, Bai X, Wang J, Tang X-R, Wu D-H, Du S-S, et al. Combination of TMB and CNA Stratifies Prognostic and Predictive Responses to Immunotherapy Across Metastatic Cancer. Clin Cancer Res (2019) 25(24):7413–23. doi: 10.1158/1078-0432.CCR-19-0558
54. Ang C, Klempner SJ, Ali SM, Madison R, Ross JS, Severson EA, et al. Prevalence of Established and Emerging Biomarkers of Immune Checkpoint Inhibitor Response in Advanced Hepatocellular Carcinoma. Oncotarget (2019) 10(40):4018–25. doi: 10.18632/oncotarget.26998
55. Tang X, Fan L, Chen G, Guo W, Qiu F, Wang J, et al. Higher Level of Tumor Mutational Burden and 11q13 Amplification in Chinese Hepatocellular Carcinoma Patients. Cancer Res (2018) 78(13 Supplement):4349. doi: 10.1158/1538-7445.Am2018-4349
56. Wong CN, Fessas P, Dominy K, Mauri FA, Kaneko T, Du P, et al. Qualification of Tumour Mutational Burden by Targeted Next-Generation Sequencing as a Biomarker in Hepatocellular Carcinoma. Liver Int (2020) 41(1):192–203. doi: 10.1111/liv.14706
57. Slatko BE, Gardner AF, Ausubel FM. Overview of Next-Generation Sequencing Technologies. Curr Protoc Mol Biol (2018) 122(1):e59. doi: 10.1002/cpmb.59
58. Baretti M, Le DT. DNA Mismatch Repair in Cancer. Pharmacol Ther (2018) 189:45–62. doi: 10.1016/j.pharmthera.2018.04.004
59. Chang L, Chang M, Chang HM, Chang F. Microsatellite Instability: A Predictive Biomarker for Cancer Immunotherapy. Appl Immunohistochem Mol Morphol (2018) 26(2):e15–21. doi: 10.1097/PAI.0000000000000575
60. Hause RJ, Pritchard CC, Shendure J, Salipante SJ. Classification and Characterization of Microsatellite Instability Across 18 Cancer Types. Nat Med (2016) 22(11):1342–50. doi: 10.1038/nm.4191
61. Rotte A. Combination of CTLA-4 and PD-1 Blockers for Treatment of Cancer. J Exp Clin Cancer Res (2019) 38(1):255. doi: 10.1186/s13046-019-1259-z
62. Svrcek M, Lascols O, Cohen R, Collura A, Jonchère V, Fléjou J-F, et al. MSI/MMR-Deficient Tumor Diagnosis: Which Standard for Screening and for Diagnosis? Diagnostic Modalities for the Colon and Other Sites: Differences Between Tumors. Bull Cancer (2019) 106(2):119–28. doi: 10.1016/j.bulcan.2018.12.008
63. André T, Shiu KK, Kim TW, Jensen BV, Jensen LH, Punt C, et al. Pembrolizumab in Microsatellite-Instability-High Advanced Colorectal Cancer. N Engl J Med (2020) 383(23):2207–18. doi: 10.1056/NEJMoa2017699
64. Togni R, Bagla N, Muiesan P, Miquel R, O'Grady J, Heaton N, et al. Microsatellite Instability in Hepatocellular Carcinoma in Non-Cirrhotic Liver in Patients Older Than 60 Years. Hepatol Res (2009) 39(3):266–73. doi: 10.1111/j.1872-034X.2008.00455.x
65. Goumard C, Desbois-Mouthon C, Wendum D, Calmel C, Merabtene F, Scatton O, et al. Low Levels of Microsatellite Instability at Simple Repeated Sequences Commonly Occur in Human Hepatocellular Carcinoma. Cancer Genomics Proteomics (2017) 14(5):329–39. doi: 10.21873/cgp.20043
66. Levy SE, Myers RM. Advancements in Next-Generation Sequencing. Annu Rev Genomics Hum Genet (2016) 17:95–115. doi: 10.1146/annurev-genom-083115-022413
67. Stratton MR, Campbell PJ, Futreal PA. The Cancer Genome. Nat (2009) 458(7239):719–24. doi: 10.1038/nature07943
68. Berger MF, Mardis ER. The Emerging Clinical Relevance of Genomics in Cancer Medicine. Nat Rev Clin Oncol (2018) 15(6):353–65. doi: 10.1038/s41571-018-0002-6
69. Harding JJ, Nandakumar S, Armenia J, Khalil DN, Albano M, Ly M, et al. Prospective Genotyping of Hepatocellular Carcinoma: Clinical Implications of Next-Generation Sequencing for Matching Patients to Targeted and Immune Therapies. Clin Cancer Res (2019) 25(7):2116–26. doi: 10.1158/1078-0432.CCR-18-2293
70. Ruiz de Galarreta M, Bresnahan E, Molina-Sánchez P, Lindblad KE, Maier B, Sia D, et al. β-Catenin Activation Promotes Immune Escape and Resistance to Anti-PD-1 Therapy in Hepatocellular Carcinoma. Cancer Discov (2019) 9(8):1124–41. doi: 10.1158/2159-8290.CD-19-0074
71. Spranger S, Bao R, Gajewski TF. Melanoma-Intrinsic β-Catenin Signalling Prevents Anti-Tumour Immunity. Nat (2015) 523(7559):231–5. doi: 10.1038/nature14404
72. von Felden J, Craig AJ, Garcia-Lezana T, Labgaa I, Haber PK, D'Avola D, et al. Mutations in Circulating Tumor DNA Predict Primary Resistance to Systemic Therapies in Advanced Hepatocellular Carcinoma. Oncogene (2021) 40(1):140–51. doi: 10.1038/s41388-020-01519-1
73. Long J, Wang A, Bai Y, Lin J, Yang X, Wang D, et al. Development and Validation of a TP53-Associated Immune Prognostic Model for Hepatocellular Carcinoma. EBioMed (2019) 42:363–74. doi: 10.1016/j.ebiom.2019.03.022
74. Hu ZQ, Xin HY, Luo CB, Li J, Zhou ZJ, Zou JX, et al. Associations Among the Mutational Landscape, Immune Microenvironment, and Prognosis in Chinese Patients With Hepatocellular Carcinoma. Cancer Immunol Immunother (2021) 70(2):377–89. doi: 10.1007/s00262-020-02685-7
75. Yang H, Sun L, Guan A, Yin H, Liu M, Mao X, et al. Unique TP53 Neoantigen and the Immune Microenvironment in Long-Term Survivors of Hepatocellular Carcinoma. Cancer Immunol Immunother (2021) 70(3):667–77. doi: 10.1007/s00262-020-02711-8
76. O'Connor MJ. Targeting the DNA Damage Response in Cancer. Mol Cell (2015) 60(4):547–60. doi: 10.1016/j.molcel.2015.10.040
77. Brandsma I, Fleuren EDG, Williamson CT, Lord CJ. Directing the Use of DDR Kinase Inhibitors in Cancer Treatment. Expert Opin Investig Drugs (2017) 26(12):1341–55. doi: 10.1080/13543784.2017.1389895
78. Arora S, Velichinskii R, Lesh RW, Ali U, Kubiak M, Bansal P, et al. Existing and Emerging Biomarkers for Immune Checkpoint Immunotherapy in Solid Tumors. Adv Ther (2019) 36(10):2638–78. doi: 10.1007/s12325-019-01051-z
79. Jeggo PA, Pearl LH, Carr AM. DNA Repair, Genome Stability and Cancer: A Historical Perspective. Nat Rev Cancer (2016) 16(1):35–42. doi: 10.1038/nrc.2015.4
80. Pilié PG, Tang C, Mills GB, Yap TA. State-Of-the-Art Strategies for Targeting the DNA Damage Response in Cancer. Nat Rev Clin Oncol (2019) 16(2):81–104. doi: 10.1038/s41571-018-0114-z
81. Reisländer T, Groelly FJ, Tarsounas M. DNA Damage And Cancer Immunotherapy: A STING in the Tale. Mol Cell (2020) 80(1):21–8. doi: 10.1016/j.molcel.2020.07.026
82. Zhang Y, Zhang L, Xu Y, Wu X, Zhou Y, Mo J. Immune-Related Long Noncoding RNA Signature for Predicting Survival and Immune Checkpoint Blockade in Hepatocellular Carcinoma. J Cell Physiol (2020) 235(12):9304–16. doi: 10.1002/jcp.29730
83. Yuan M, Wang Y, Sun Q, Liu S, Xian S, Dai F, et al. Identification of a Nine Immune-Related lncRNA Signature as a Novel Diagnostic Biomarker for Hepatocellular Carcinoma. BioMed Res Int (2021) 2021:9798231. doi: 10.1155/2021/9798231
84. Peng L, Chen Z, Chen Y, Wang X, Tang N. MIR155HG is a Prognostic Biomarker and Associated With Immune Infiltration and Immune Checkpoint Molecules Expression in Multiple Cancers. Cancer Med (2019) 8(17):7161–73. doi: 10.1002/cam4.2583
85. Xu Q, Wang Y, Huang W. Identification of Immune-Related lncRNA Signature for Predicting Immune Checkpoint Blockade and Prognosis in Hepatocellular Carcinoma. Int Immunopharmacol (2021) 92:107333. doi: 10.1016/j.intimp.2020.107333
86. Matson V, Fessler J, Bao R, Chongsuwat T, Zha Y, Alegre ML, et al. The Commensal Microbiome Is Associated With Anti-PD-1 Efficacy in Metastatic Melanoma Patients. Sci (2018) 359(6371):104–8. doi: 10.1126/science.aao3290
87. Gopalakrishnan V, Spencer CN, Nezi L, Reuben A, Andrews MC, Karpinets TV, et al. Gut Microbiome Modulates Response to Anti-PD-1 Immunotherapy in Melanoma Patients. Sci (2018) 359(6371):97–103. doi: 10.1126/science.aan4236
88. Lambring CB, Siraj S, Patel K, Sankpal UT, Mathew S, Basha R. Impact of the Microbiome on the Immune System. Crit Rev Immunol (2019) 39(5):313–28. doi: 10.1615/CritRevImmunol.2019033233
89. Gharaibeh RZ, Jobin C. Microbiota and Cancer Immunotherapy: In Search of Microbial Signals. Gut (2019) 68(3):385–8. doi: 10.1136/gutjnl-2018-317220
90. Zheng Y, Wang T, Tu X, Huang Y, Zhang H, Tan DF, et al. Gut Microbiome Affects the Response to Anti-PD-1 Immunotherapy in Patients With Hepatocellular Carcinoma. J Immunother Cancer (2019) 7(1):193. doi: 10.1186/s40425-019-0650-9
91. Fessler J, Matson V, Gajewski TF. Exploring the Emerging Role of the Microbiome in Cancer Immunotherapy. J Immunother Cancer (2019) 7(1):108. doi: 10.1186/s40425-019-0574-4
92. Makuku R, Khalili N, Razi S, Keshavarz-Fathi M, Rezaei N. Current and Future Perspectives of PD-1/PDL-1 Blockade in Cancer Immunotherapy. J Immunol Res (2021) 2021:6661406. doi: 10.1155/2021/6661406
93. Rizzo A, Ricci AD. PD-L1, TMB, and Other Potential Predictors of Response to Immunotherapy for Hepatocellular Carcinoma: How can They Assist Drug Clinical Trials? Expert Opin Investig Drugs (2021) 1–9. doi: 10.1080/13543784.2021.1972969
94. Hayase E, Jenq RR. Role of the Intestinal Microbiome and Microbial-Derived Metabolites in Immune Checkpoint Blockade Immunotherapy of Cancer. Genome Med (2021) 13(1):107. doi: 10.1186/s13073-021-00923-w
95. Baruch EN, Wang J, Wargo JA. Gut Microbiota and Antitumor Immunity: Potential Mechanisms for Clinical Effect. Cancer Immunol Res (2021) 9(4):365–70. doi: 10.1158/2326-6066.CIR-20-0877
96. Kim E, Ahn H, Park H. A Review on the Role of Gut Microbiota in Immune Checkpoint Blockade Therapy for Cancer. Mamm Genome (2021) 32(4):223–31. doi: 10.1007/s00335-021-09867-3
Keywords: hepatocellular carcinoma, HCC, immunotherapy, biomarkers, PD-L1
Citation: Rizzo A, Ricci AD, Di Federico A, Frega G, Palloni A, Tavolari S and Brandi G (2021) Predictive Biomarkers for Checkpoint Inhibitor-Based Immunotherapy in Hepatocellular Carcinoma: Where Do We Stand? Front. Oncol. 11:803133. doi: 10.3389/fonc.2021.803133
Received: 27 October 2021; Accepted: 01 December 2021;
Published: 17 December 2021.
Edited by:
Hongsong Chen, Peking University People’s Hospital, ChinaReviewed by:
Mehmet Akce, Emory University, United StatesCopyright © 2021 Rizzo, Ricci, Di Federico, Frega, Palloni, Tavolari and Brandi. This is an open-access article distributed under the terms of the Creative Commons Attribution License (CC BY). The use, distribution or reproduction in other forums is permitted, provided the original author(s) and the copyright owner(s) are credited and that the original publication in this journal is cited, in accordance with accepted academic practice. No use, distribution or reproduction is permitted which does not comply with these terms.
*Correspondence: Giovanni Brandi, giovanni.brandi@unibo.it