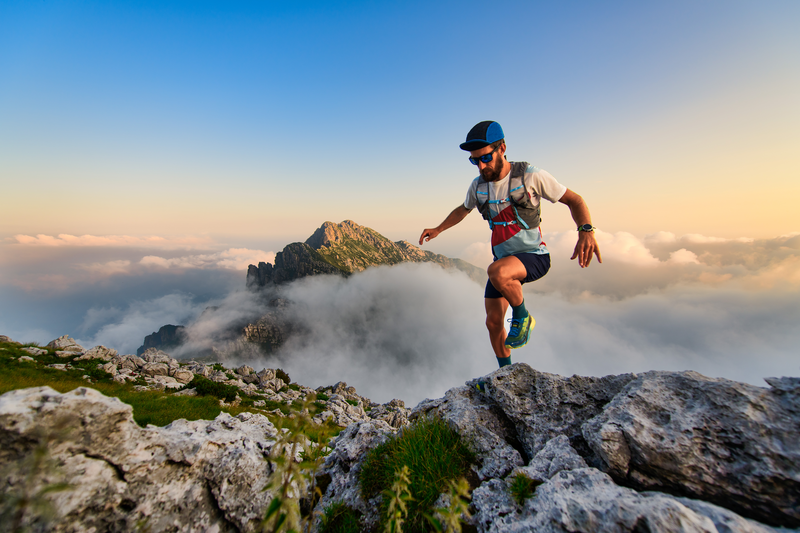
95% of researchers rate our articles as excellent or good
Learn more about the work of our research integrity team to safeguard the quality of each article we publish.
Find out more
EDITORIAL article
Front. Oncol. , 23 December 2021
Sec. Head and Neck Cancer
Volume 11 - 2021 | https://doi.org/10.3389/fonc.2021.785001
This article is part of the Research Topic Non-coding RNAs in Head and Neck Squamous Cell Carcinoma: Functional and Clinical Implications. View all 26 articles
Editorial on the Research Topic
Non-Coding RNAs in Head and Neck Squamous Cell Carcinoma: Functional and Clinical Implications
Head and Neck Squamous Cell Carcinoma (HNSCC) is one of the most commonly reported malignant tumors in human beings, representing 5.3% of all cancers (1). In the past several years, there has been a lot of progress in revealing the mutational landscape of HNSCC. For example, a series of hotspot mutational genes such as tumor protein p53 (TP53), cyclin dependent kinase inhibitor 2A (CDKN2A), phosphatase and tensin homolog (PTEN), phosphatidylinositol-4,5-bisphosphate 3-kinase catalytic subunit alpha (PIK3CA), and notch receptor 1 (NOTCH1) were identified and tumors can be now classified based on genomic profiling (2, 3). In addition, epigenetic profiling revealed non-coding RNAs play a regulatory role in HNSCC (4, 5, Shao et al.). Non–coding RNAs including microRNAs (miRNAs), long non–coding RNAs (lncRNAs) and circular RNAs (circRNAs) have been found to be closely involved in the development and progression of HNSCC and partially associated with clinical significance of HNSCC patients.
MicroRNAs are non–coding RNAs with about 22 nucleotides long that participate in the post–transcriptional regulation of gene expression involved in the proliferation, migration, metastasis and angiogenesis of cancer (6). For instant, microRNA–125a is responsible for the increased proliferation and migration of cancer cells by inhibiting the expression of the p53 protein (7). MicroRNA–363 promotes increased invasion and metastasis of HNSCC cells by targeting podoplanin protein (8). MicroRNA–300 influences epithelial to mesenchymal transition and metastasis by inhibiting the expression of the Twist (9). Paired box 4 (PAX4)–regulated microRNA–144/451 modulates invasion and metastasis by suppressing disintegrin and metalloproteinases (ADAMs) expression (10). MicroRNA–30e–5p inhibits angiogenesis and metastasis of cancer cells through directly targeting astrocyte elevated gene–1 (AEG–1) in HNSCC (11). In addition, a part of miRNAs derived from serum and salivary were found to be linked to the non–invasive diagnosis and prognosis of HNSCC patients. Serum exosomal microRNA–491–5p and microRNA–941 serve as the promising diagnostic biomarkers for HNSCC, respectively (12, 13). The three miRNA signature (microRNA–383, microRNA–615, and microRNA–877) or two–miRNA signature (miR–626 and miR–5100) act as the diagnostic and prognostic predictors for HNSCC patients, respectively (14, 15). Salivary miR–423–5p,miR–let–7a–5p,miR–3928 and a three–miRNA panel (miR–9, miR–134 and miR–191) could be used as novel non–invasive diagnostic biomarkers for HNSCC (16–18). More and more novel researches continue to strengthen the position of miRNAs as important regulator and useful biomarker in HNSCC as time goes on.
LncRNAs also belong to a subgroup of non–coding RNAs that are at least 200 nucleotides long and the majority of them do not have proteincoding ability (19). lncRNAs can be detected both in the nucleus and cytoplasm, and their different locations mean different functions that were involved in regulation of gene expression, e.g., chromatin modification, interaction with transcriptional factors, mRNA processing, cell metabolism, proliferation, apoptosis, acting as “molecular sponge” and creating ribonucleoprotein complexes (19–22). For example, Metastasis–associated lung adenocarcinoma transcript 1 (MALAT1) has one of the most conserved primary and secondary structures of all lncRNAs (23). Knockdown of MALAT–1 led to impaired migration and proliferation ability in vitro and fewer metastases in vivo in HNSCC cells (24). MALAT–1 transcriptionally activated by signal transducer and activator of transcription 3 (STAT3) induces epithelial–to–mesenchymal transition (EMT) and accelerates HNSCC metastasis by interacting reciprocally with miR–30a (25). HOX transcript antisense RNA (HOTAIR) is expressed from locus homeobox C cluster (HOXC) and lncRNA HOTAIR transcriptionally activated by STAT3 interact with pEZH2–S21 resulting in proliferation and the growth of HNSCC xenograft tumors in vivo (26). Besides, some of lncRNAs functionally act as tumor suppressors, e.g., lincRNA–p21 (27) or LINC02487(Feng et al.), long non–coding RNA Fer–1–like protein 4 (FER1L4) (28), and growth arrest specific 5 (GAS5) (29), but others play an oncogenic role, e.g., ring finger and CCCH–type domains 2 (RC3H2) (30), FOXD2 adjacent opposite strand RNA 1(FOXD2–AS1) (31), KTN1 antisense RNA 1(KTN1–AS1) (32), LINC00460 (33), H19 imprinted maternally expressed transcript (H19) (34), small nucleolar RNA host gene 6 (SNHG6) (35), urothelial cancer associated 1 (UCA1) (36), or ZNFX1 antisense RNA 1 (ZFAS1) (37), and can be used as therapeutic targets in the future. More and more evidences show that multiple lncRNAs–based signatures could be diagnostic and prognostic predictors of HNSCC patients by mathematical modeling. A nomogram based on an 8–lncRNA signature was identified as a novel diagnostic biomarker for HNSCC (38). A three–lncRNA signature was screened and identified to well predict the survival of HNSCC patients (39). Immune–related and autophagy–related lncRNA signatures were respectively developed into prognostic indicator for HNSCC (40, Guo et al).
circRNAs are a novel subclass of non–coding RNAs, which are produced by reverse splicing and are characterized by a closed single–stranded structure and lack of 5′cap and 3′polyadenylation [poly(A)] tail (41). Currently, the most established function of circRNAs is that circRNAs can act as miRNA sponges resulting in the initiation and progression of cancer and play a regulatory role in the tumor microenvironment (TME) (42, 43). For example, circRNA Pvt1 oncogene (circPVT1) transcriptionally enhanced by the mut–p53/Yes1 associated transcriptional regulator (YAP)/TEAD complex promotes the proliferation of HNSCC cells by modulating the expression of miR–497–5p (44). CircRNA_ 036186 likely regulates 14–3–3ζ expression by functioning as a ceRNA in the development and progression of HNSCC (45). And the function as a ceRNA was also found to be in circRNA_100533 (46), circRNA myosin light chain kinase (circMYLK) (47), circRNA_0042666 (48), circRNA_100290 (49), circRNA_0000140 (50), circRNA Matrin 3(circMATR3) (51), circRNA_0000495 (52),circRNA coronin 1C (circCORO1C) (53), circRNA_0036722 (54), circRNA_0000218 (55), circRNA epithelial stromal interaction 1(circEPSTI1) (56), circRNA_103862 (57), circRNA_009755 (58), circRNA septin 9 (circSEPT9) (59), circRNA par–3 family cell polarity regulator (circPARD3) (60), circRNA BICD cargo adaptor 2 (circBICD2) (61), circKIAA0907 (62), circRNA_0023028 (63), circRNA_0000700 (64), circRNA ATP binding cassette subfamily B member 10 (circABCB10) (65), circRNA_0042823 (66), circBCL11B (67), circRNA fibronectin type III domain containing 3B (circFNDC3B) (68), and circRNA PTPRF interacting protein alpha 1(circPPFIA1) (69). In addition, a few circRNAs can directly interact with proteins and enzymes as protein scaffolds. For instance, circRNA G protein subunit gamma 7 (circGNG7) inhibits HNSCC cell proliferation by binding to serine residues 78 and 82 of Heat shock protein 27 (HSP27) (70). CircRNA FAT atypical cadherin 1(circFAT1) prevents STAT3 dephosphorylation by binding to STAT3, resulting in HNSCC stemness and immune evasion (71). Due to more stable than lncRNA and miRNA, circRNAs are regarded as an ideal diagnostic predictor tool in clinical practice. In HNSCC, circRNA_0003829 and circRNA_0036722 serve as diagnostic predictors with AUC=0.81 and AUC=0.83, respectively (54, 72). Plasma–derived the three circRNA panel well predicts the occurrence of HNSCC (73).
This Research Topic “Non–coding RNAs in Head and Neck Squamous Cell Carcinoma: Functional and Clinical Implications” includes 21 original articles and 3 review articles, which highlight the regulatory mechanism and clinical relevance of non–coding RNAs in HNSCC. For example, Wan et al. identifies a super–enhancer regulatory model of miR–21–5p by FOS like 1 (FOSL1), promoting malignant progression of HNSCC. The article by Li et al. discuss LINC02195 as a regulator of MHC I and the number of CD8+ and CD4+ T cells in the tumor microenvironment. Moreover, Wang et al. summarizes the crosstalk between lncRNAs and microRNAs as well as the detailed regulatory mechanism of the interaction. Additionally, Luo et al. identifies miRNAs signatures respectively for HPV+ and HPV− HNSCC, which are of great significance in evaluating patient survival. All of other articles mainly discuss the potential function of non–coding RNA as a ceRNA in HNSCC.
All authors listed have made a substantial, direct and intellectual contribution to the work, and approved it for publication.
WC was supported by grants from the National Natural Science Foundation of China (81972589).
The authors declare that the research was conducted in the absence of any commercial or financial relationships that could be construed as a potential conflict of interest.
All claims expressed in this article are solely those of the authors and do not necessarily represent those of their affiliated organizations, or those of the publisher, the editors and the reviewers. Any product that may be evaluated in this article, or claim that may be made by its manufacturer, is not guaranteed or endorsed by the publisher.
1. Aupérin A. Epidemiology of Head and Neck Cancers: An Update. Curr Opin Oncol (2020) 32(3):178–86. doi: 10.1097/CCO.0000000000000629
2. Stransky N, Egloff AM, Tward AD, Kostic AD, Cibulskis K, Sivachenko A, et al. The Mutational Landscape of Head and Neck Squamous Cell Carcinoma. Science (2011) 333:1157–60. doi: 10.1126/science.1208130
3. Agrawal N, Frederick MJ, Pickering CR, Bettegowda C, Chang K, Li RJ, et al. Exome Sequencing of Head and Neck Squamous Cell Carcinoma Reveals Inactivating Mutations in NOTCH1. Science (2011) 333:1154–7. doi: 10.1126/science.1206923
4. Karatas OF, Oner M, Abay A, Diyapoglu A. MicroRNAs in Human Tongue Squamous Cell Carcinoma: From Pathogenesis to Therapeutic Implications. Oral Oncol (2017) 67:124–30. doi: 10.1016/j.oraloncology.2017.02.015
5. Nohata N, Abba MC, Gutkind JS. Unraveling the Oral Cancer Lncrnaome: Identification of Novel lncRNAs Associated With Malignant Progression and HPV Infection. Oral Oncol (2016) 59:58–66. doi: 10.1016/j.oraloncology.2016.05.014
6. Nowicka Z, Stawiski K, Tomasik B, Fendler W. Extracellular miRNAs as Biomarkers of Head and Neck Cancer Progression and Metastasis. Int J Mol Sci (2019) 20:4799. doi: 10.3390/ijms20194799
7. Peng SY, Tu HF, Yang CC, Wu CH, Liu CJ, Chang KW, et al. miR–134 Targets PDCD7 to Reduce E–Cadherin Expression and Enhance Oral Cancer Progression. Int J Cancer (2018) 143(11):2892–904. doi: 10.1002/ijc.31638
8. Sun Q, Zhang J, Cao W, Wang X, Xu Q, Yan M, et al. Dysregulated miR–363 Affects Head and Neck Cancer Invasion and Metastasis by Targeting Podoplanin. Int J Biochem Cell Biol (2013) 45(3):513–20. doi: 10.1016/j.biocel.2012.12.004
9. Yu J, Xie F, Bao X, Chen W, Xu Q. miR–300 Inhibits Epithelial to Mesenchymal Transition and Metastasis by Targeting Twist in Human Epithelial Cancer. Mol Cancer (2014) 13:121. doi: 10.1186/1476–4598–13–121
10. Zhang J, Qin X, Sun Q, Guo H, Wu X, Xie F, et al. Transcriptional Control of PAX4–Regulated miR–144/451 Modulates Metastasis by Suppressing ADAMs Expression. Oncogene (2015) 34(25):3283–95. doi: 10.1038/onc.2014.259
11. Zhang S, Li G, Liu C, Lu S, Jing Q, Chen X, et al. miR–30e–5p Represses Angiogenesis and Metastasis by Directly Targeting AEG–1 in Squamous Cell Carcinoma of the Head and Neck. Cancer Sci (2020) 111(2):356–68. doi: 10.1111/cas.14259
12. Panvongsa W, Siripoon T, Worakitchanon W, Arsa L, Trachu N, Jinawath N, et al. Plasma Extracellular Vesicle microRNA–491–5p as Diagnostic and Prognostic Marker for Head and Neck Squamous Cell Carcinoma. Cancer Sci (2021) 112(10):4257–69. doi: 10.1111/cas.15067
13. Zhao Q, Zheng X, Guo H, Xue X, Zhang Y, Niu M, et al. Serum Exosomal miR–941 as a Promising Oncogenic Biomarker for Laryngeal Squamous Cell Carcinoma. J Cancer (2020) 11(18):5329–44. doi: 10.7150/jca.45394
14. Liu C, Yu Z, Huang S, Zhao Q, Sun Z, Fletcher C, et al. Combined Identification of Three miRNAs in Serum as Effective Diagnostic Biomarkers for HNSCC. EBioMedicine (2019) 50:135–43. doi: 10.1016/j.ebiom.2019.11.016
15. Shi J, Bao X, Liu Z, Zhang Z, Chen W, Xu Q. Serum miR–626 and miR–5100 are Promising Prognosis Predictors for Oral Squamous Cell Carcinoma. Theranostics (2019) 9(4):920–31. doi: 10.7150/thno.30339
16. Romani C, Salviato E, Paderno A, Zanotti L, Ravaggi A, Deganello A, et al. Genome–Wide Study of Salivary miRNAs Identifies miR–423–5p as Promising Diagnostic and Prognostic Biomarker in Oral Squamous Cell Carcinoma. Theranostics (2021) 11(6):2987–99. doi: 10.7150/thno.45157
17. Fadhil RS, Wei MQ, Nikolarakos D, Good D, Nair RG. Salivary microRNA miR–Let–7a–5p and miR–3928 Could Be Used as Potential Diagnostic Bio–Markers for Head and Neck Squamous Cell Carcinoma. PloS One (2020) 15(3):e0221779. doi: 10.1371/journal.pone.0221779
18. Salazar C, Nagadia R, Pandit P, Cooper–White J, Banerjee N, Dimitrova N, et al. A Novel Saliva–Based microRNA Biomarker Panel to Detect Head and Neck Cancers. Cell Oncol (Dordr) (2014) 37(5):331–8. doi: 10.1007/s13402–014–0188–2
19. Mercer TR, Dinger ME, Mattick JS. Long non–Coding RNAs: Insights Into Functions. Nat Rev Genet (2009) 10(3):155–9. doi: 10.1038/nrg2521
20. Prensner JR, Chinnaiyan AM. The Emergence of lncRNAs in Cancer Biology. Cancer Discovery (2011) 1(5):391–407. doi: 10.1158/2159–8290.CD–11–0209
21. Schmitt AM, Chang HY. Long Noncoding RNAs in Cancer Pathways. Cancer Cell (2016) 29(4):452–63. doi: 10.1016/j.ccell.2016.03.010
22. Jiang C, Li X, Zhao H, Liu H. Long Non–Coding RNAs: Potential New Biomarkers for Predicting Tumor Invasion and Metastasis. Mol Cancer (2016) 15(1):62. doi: 10.1186/s12943–016–0545–z
23. Tripathi V, Ellis JD, Shen Z, Song DY, Pan Q, Watt AT, et al. The Nuclear–Retained Noncoding RNA MALAT1 Regulates Alternative Splicing by Modulating SR Splicing Factor Phosphorylation. Mol Cell (2010) 39(6):925–38. doi: 10.1016/j.molcel.2010.08.011
24. Fang Z, Zhang S, Wang Y, Shen S, Wang F, Hao Y, et al. Long non–Coding RNA MALAT–1 Modulates Metastatic Potential of Tongue Squamous Cell Carcinomas Partially Through the Regulation of Small Proline Rich Proteins. BMC Cancer (2016) 16(1):706. doi: 10.1186/s12885–016–2735–x
25. Wang Y, Wu C, Zhang C, Li Z, Zhu T, Chen J, et al. TGF–β–Induced STAT3 Overexpression Promotes Human Head and Neck Squamous Cell Carcinoma Invasion and Metastasis Through Malat1/miR–30a Interactions. Cancer Lett (2018) 436:52–62. doi: 10.1016/j.canlet.2018.08.009
26. Sun S, Wu Y, Guo W, Yu F, Kong L, Ren Y, et al. STAT3/HOTAIR Signaling Axis Regulates HNSCC Growth in an EZH2–Dependent Manner. Clin Cancer Res (2018) 24(11):2665–77. doi: 10.1158/1078–0432.CCR–16–2248
27. Jin S, Yang X, Li J, Yang W, Ma H, Zhang Z. P53–Targeted lincRNA–P21 Acts as a Tumor Suppressor by Inhibiting JAK2/STAT3 Signaling Pathways in Head and Neck Squamous Cell Carcinoma. Mol Cancer (2019) 18(1):38. doi: 10.1186/s12943–019–0993–3
28. Jiao L, Liu S, Liu L, Hao P, Gong Z, Yan Z, et al. Long non−Coding RNA Fer−1−Like Family Member 4 Serves as a Tumor Suppressor in Laryngeal Squamous Cell Carcinoma Cells via Regulating the AKT/ERK Signaling Pathway. Mol Med Rep (2020) 22(6):5304–12. doi: 10.3892/mmr.2020.11598
29. Wang J, Zhu Y, Ni S, Liu S. LncRNA GAS5 Suppressed Proliferation and Promoted Apoptosis in Laryngeal Squamous Cell Carcinoma by Targeting MiR–26a–5p and Modifying Ulk2. Cancer Manag Res (2021) 13:871–87. doi: 10.2147/CMAR.S250778
30. Wu K, Jiang Y, Zhou W, Zhang B, Li Y, Xie F, et al. Long Noncoding RNA RC3H2 Facilitates Cell Proliferation and Invasion by Targeting MicroRNA–101–3p/EZH2 Axis in OSCC. Mol Ther Nucleic Acids (2020) 20:97–110. doi: 10.1016/j.omtn.2020.02.006
31. Liu Z, Zhou W, Lin C, Wang X, Zhang X, Zhang Y, et al. Dysregulation of FOXD2–AS1 Promotes Cell Proliferation and Migration and Predicts Poor Prognosis in Oral Squamous Cell Carcinoma: A Study Based on TCGA Data. Aging (Albany NY) (2020) 13(2):2379–96. doi: 10.18632/aging.202268
32. Jiang Y, Wu K, Cao W, Xu Q, Wang X, Qin X, et al. Long Noncoding RNA KTN1–AS1 Promotes Head and Neck Squamous Cell Carcinoma Cell Epithelial–Mesenchymal Transition by Targeting miR–153–3p. Epigenomics (2020) 12(6):487–505. doi: 10.2217/epi–2019–0173
33. Jiang Y, Cao W, Wu K, Qin X, Wang X, Li Y, et al. LncRNA LINC00460 Promotes EMT in Head and Neck Squamous Cell Carcinoma by Facilitating Peroxiredoxin–1 Into the Nucleus. J Exp Clin Cancer Res (2019) 38(1):365. doi: 10.1186/s13046–019–1364–z
34. Guan GF, Zhang DJ, Wen LJ, Xin D, Liu Y, Yu DJ, et al. Overexpression of lncRNA H19/miR–675 Promotes Tumorigenesis in Head and Neck Squamous Cell Carcinoma. Int J Med Sci (2016) 13(12):914–22. doi: 10.7150/ijms.16571
35. Guo T, Zambo KDA, Zamuner FT, Ou T, Hopkins C, Kelley DZ, et al. Chromatin Structure Regulates Cancer–Specific Alternative Splicing Events in Primary HPV–Related Oropharyngeal Squamous Cell Carcinoma. Epigenetics (2020) 15(9):959–71. doi: 10.1080/15592294.2020.1741757
36. Qian Y, Liu D, Cao S, Tao Y, Wei D, Li W, et al. Upregulation of the Long Noncoding RNA UCA1 Affects the Proliferation, Invasion, and Survival of Hypopharyngeal Carcinoma. Mol Cancer (2017) 16(1):68. doi: 10.1186/s12943–017–0635–6
37. Kolenda T, Guglas K, Kopczyńska M, Teresiak A, Bliźniak R, Mackiewicz A, et al. Oncogenic Role of ZFAS1 lncRNA in Head and Neck Squamous Cell Carcinomas. Cells (2019) 8(4):366. doi: 10.3390/cells8040366
38. Mao R, Chen Y, Xiong L, Liu Y, Zhang T. Identification of a Nomogram Based on an 8–lncRNA Signature as a Novel Diagnostic Biomarker for Head and Neck Squamous Cell Carcinoma. Aging (Albany NY) (2020) 12(20):20778–800. doi: 10.18632/aging.104014
39. Cao W, Liu JN, Liu Z, Wang X, Han ZG, Ji T, et al. A three–lncRNA Signature Derived From the Atlas of ncRNA in Cancer (TANRIC) Database Predicts the Survival of Patients With Head and Neck Squamous Cell Carcinoma. Oral Oncol (2017) 65:94–101. doi: 10.1016/j.oraloncology.2016.12.017
40. Chen Y, Luo TQ, Xu SS, Chen CY, Sun Y, Lin L, et al. An Immune–Related seven–lncRNA Signature for Head and Neck Squamous Cell Carcinoma. Cancer Med (2021) 10(7):2268–85. doi: 10.1002/cam4.3756
41. Meng S, Zhou H, Feng Z, Xu Z, Tang Y, Li P, et al. CircRNA: Functions and Properties of a Novel Potential Biomarker for Cancer. Mol Cancer (2017) 16(1):94. doi: 10.1186/s12943–017–0663–2
42. He J, Xie Q, Xu H, Li J, Li Y. Circular RNAs and Cancer. Cancer Lett (2017) 396:138–44. doi: 10.1016/j.canlet.2017.03.027
43. Ma Z, Shuai Y, Gao X, Wen X, Ji J. Circular RNAs in the Tumour Microenvironment. Mol Cancer (2020) 19(1):8. doi: 10.1186/s12943–019–1113–0
44. Verduci L, Ferraiuolo M, Sacconi A, Ganci F, Vitale J, Colombo T, et al. The Oncogenic Role of Circpvt1 in Head and Neck Squamous Cell Carcinoma is Mediated Through the Mutant P53/YAP/TEAD Transcription–Competent Complex. Genome Biol (2017) 18(1):237. doi: 10.1186/s13059–017–1368–y
45. Wang WL, Yang Z, Zhang YJ, Lu P, Ni YK, Sun CF, et al. Competing Endogenous RNA Analysis Reveals the Regulatory Potency of circRNA_036186 in HNSCC. Int J Oncol (2018) 53(4):1529–43. doi: 10.3892/ijo.2018.4499
46. Zhu X, Shao P, Tang Y, Shu M, Hu WW, Zhang Y. Hsa_circRNA_100533 Regulates GNAS by Sponging Hsa_Mir_933 to Prevent Oral Squamous Cell Carcinoma. J Cell Biochem (2019) 120(11):19159–71. doi: 10.1002/jcb.29245
47. Duan X, Shen N, Chen J, Wang J, Zhu Q, Zhai Z. Circular RNA MYLK Serves as an Oncogene to Promote Cancer Progression via microRNA–195/Cyclin D1 Axis in Laryngeal Squamous Cell Carcinoma. Biosci Rep (2019) 39(9):BSR20190227. doi: 10.1042/BSR20190227
48. Wei Z, Chang K, Fan C. Hsa_circ_0042666 Inhibits Proliferation and Invasion via Regulating miR–223/TGFBR3 Axis in Laryngeal Squamous Cell Carcinoma. BioMed Pharmacother (2019) 119:109365. doi: 10.1016/j.biopha.2019.109365
49. Wang Z, Huang C, Zhang A, Lu C, Liu L. Overexpression of circRNA_100290 Promotes the Progression of Laryngeal Squamous Cell Carcinoma Through the miR–136–5p/RAP2C Axis. BioMed Pharmacother (2020) 125:109874. doi: 10.1016/j.biopha.2020.109874
50. Peng QS, Cheng YN, Zhang WB, Fan H, Mao QH, Xu P. circRNA_0000140 Suppresses Oral Squamous Cell Carcinoma Growth and Metastasis by Targeting miR–31 to Inhibit Hippo Signaling Pathway. Cell Death Dis (2020) 11(2):112. doi: 10.1038/s41419–020–2273–y
51. Wang Z, Wei P, Wei D, Cao S, Liu H, Chen L, et al. Effect of Up–Regulation of Circmatr3 on the Proliferation, Metastasis, Progression and Survival of Hypopharyngeal Carcinoma. J Cell Mol Med (2020) 24(8):4687–97. doi: 10.1111/jcmm.15134
52. Hao Y, Zhang D, Guo Y, Fu Z, Yu D, Guan G. miR–488–3p Sponged by Circ–0000495 and Mediated Upregulation of TROP2 in Head and Neck Squamous Cell Carcinoma Development. J Cancer (2020) 11(11):3375–86. doi: 10.7150/jca.40339
53. Wu Y, Zhang Y, Zheng X, Dai F, Lu Y, Dai L, et al. Circular RNA Circcoro1c Promotes Laryngeal Squamous Cell Carcinoma Progression by Modulating the Let–7c–5p/PBX3 Axis. Mol Cancer (2020) 19(1):99. doi: 10.1186/s12943–020–01215–4
54. Guo Y, Huang Q, Zheng J, Hsueh CY, Yuan X, Heng Y, et al. Diagnostic Role of Dysregulated Circular RNA Hsa_Circ_0036722 in Laryngeal Squamous Cell Carcinoma. Onco Targets Ther (2020) 13:5709–19. doi: 10.2147/OTT.S231076
55. Bai Y, Hou J, Wang X, Geng L, Jia X, Xiang L, et al. Circ_0000218 Plays a Carcinogenic Role in Laryngeal Cancer Through Regulating microRNA–139–3p/Smad3 Axis. Pathol Res Pract (2020) 216(9):153103. doi: 10.1016/j.prp.2020.153103
56. Wang J, Jiang C, Li N, Wang F, Xu Y, Shen Z, et al. The Circepsti1/Mir–942–5p/LTBP2 Axis Regulates the Progression of OSCC in the Background of OSF via EMT and the PI3K/Akt/mTOR Pathway. Cell Death Dis (2020) 11(8):682. doi: 10.1038/s41419–020–02851–w
57. Wang X, Wu T, Wang P, Yang L, Li Q, Wang J, et al. Circular RNA 103862 Promotes Proliferation and Invasion of Laryngeal Squamous Cell Carcinoma Cells Through the miR–493–5p/GOLM1 Axis. Front Oncol (2020) 10:1064. doi: 10.3389/fonc.2020.01064
58. Zhang B, Wang Z, Shen Y, Yang H. Silencing Circular RNA Hsa_Circ_009755 Promotes Growth and Metastasis of Oral Squamous Cell Carcinoma. Genomics (2020) 112(6):5275–81. doi: 10.1016/j.ygeno.2020.09.035
59. Ai Y, Tang Z, Zou C, Wei H, Wu S, Huang D. Circ_SEPT9, a Newly Identified Circular RNA, Promotes Oral Squamous Cell Carcinoma Progression Through miR–1225/PKN2 Axis. J Cell Mol Med (2020) 24(22):13266–77. doi: 10.1111/jcmm.15943
60. Gao W, Guo H, Niu M, Zheng X, Zhang Y, Xue X, et al. Circpard3 Drives Malignant Progression and Chemoresistance of Laryngeal Squamous Cell Carcinoma by Inhibiting Autophagy Through the PRKCI–Akt–mTOR Pathway. Mol Cancer (2020) 19(1):166. doi: 10.1186/s12943–020–01279–2
61. Qiu L, Zheng L, Gan C, Deng W, Sun Y, Wang T. Circbicd2 Targets miR–149–5p/IGF2BP1 Axis to Regulate Oral Squamous Cell Carcinoma Progression. J Oral Pathol Med (2021) 50(7):668–80. doi: 10.1111/jop.13156
62. Dong W, Zhao L, Zhang S, Zhang S, Si H. Circ–KIAA0907 Inhibits the Progression of Oral Squamous Cell Carcinoma by Regulating the miR–96–5p/UNC13C Axis. World J Surg Oncol (2021) 19(1):75. doi: 10.1186/s12957–021–02184–8
63. Zheng Y, Duan L, Yang Y, Luo D, Yan B. Circ_0023028 Contributes to the Progression of Laryngeal Squamous Cell Carcinoma by Upregulating LASP1 Through miR–486–3p. Mol Cell Biochem (2021) 476(8):2951–61. doi: 10.1007/s11010–021–04129–x
64. Fang J, Ji WH, Wang FZ, Xie TM, Wang L, Fu ZF, et al. Circular RNA Hsa_Circ_0000700 Promotes Cell Proliferation and Migration in Esophageal Squamous Cell Carcinoma by Sponging miR–1229. J Cancer (2021) 12(9):2610–23. doi: 10.7150/jca.47112
65. Zhao J, Li XD, Wang M, Song LN, Zhao MJ. Circular RNA ABCB10 Contributes to Laryngeal Squamous Cell Carcinoma (LSCC) Progression by Modulating the miR–588/CXCR4 Axis. Aging (Albany NY) (2021) 13(10):14078–87. doi: 10.18632/aging.203025
66. Wu T, Sun Y, Sun Z, Li S, Wang W, Yu B, et al. Hsa_circ_0042823 Accelerates Cancer Progression via miR–877–5p/FOXM1 Axis in Laryngeal Squamous Cell Carcinoma. Ann Med (2021) 53(1):960–70. doi: 10.1080/07853890.2021.1934725
67. Zeng W, Guo M, Yao L, Deng Z. Circular RNA Hsa_Circ_0033144 (CircBCL11B) Regulates Oral Squamous Cell Carcinoma Progression via the miR–579/LASP1 Axis. Bioengineered (2021) 12(1):4111–22. doi: 10.1080/21655979.2021.1953214
68. Yang J, Cao XH, Luan KF, Huang YD. Circular RNA FNDC3B Protects Oral Squamous Cell Carcinoma Cells From Ferroptosis and Contributes to the Malignant Progression by Regulating miR–520d–5p/SLC7A11 Axis. Front Oncol (2021) 11:672724. doi: 10.3389/fonc.2021.672724
69. Shuang Y, Liu J, Niu J, Guo W, Li C. A Novel Circular RNA Circppfia1 Promotes Laryngeal Squamous Cell Carcinoma Progression Through Sponging miR–340–3p and Regulating ELK1 Expression. Bioengineered (2021) 12(1):5220–30. doi: 10.1080/21655979.2021.1959866
70. Ju H, Hu Z, Wei D, Huang J, Zhang X, Rui M, et al. A Novel Intronic Circular RNA, Circgng7, Inhibits Head and Neck Squamous Cell Carcinoma Progression by Blocking the Phosphorylation of Heat Shock Protein 27 at Ser78 and Ser82. Cancer Commun (Lond) (2021) 41(11):1152–72. doi: 10.1002/cac2.12213
71. Jia L, Wang Y, Wang CY. Circfat1 Promotes Cancer Stemness and Immune Evasion by Promoting STAT3 Activation. Adv Sci (Weinh) (2021) 8(13):2003376. doi: 10.1002/advs.202003376
72. Zhang H, Shen Y, Zhang B, Qian M, Zhang Y, Yang H. Hsa_circ_0003829 Serves as a Potential Diagnostic Predictor for Oral Squamous Cell Carcinoma. J Int Med Res (2020) 48(9):300060520936880. doi: 10.1177/0300060520936880
Keywords: non-coding RNAs, microRNAs, circular RNAs, long non-coding RNAs, head and neck squamous cell carcinoma
Citation: Cao W, Shen Q and Lim MY (2021) Editorial: “Non-Coding RNAs in Head and Neck Squamous Cell Carcinoma”. Front. Oncol. 11:785001. doi: 10.3389/fonc.2021.785001
Received: 28 September 2021; Accepted: 09 December 2021;
Published: 23 December 2021.
Edited and reviewed by:
Jan Baptist Vermorken, University of Antwerp, BelgiumCopyright © 2021 Cao, Shen and Lim. This is an open-access article distributed under the terms of the Creative Commons Attribution License (CC BY). The use, distribution or reproduction in other forums is permitted, provided the original author(s) and the copyright owner(s) are credited and that the original publication in this journal is cited, in accordance with accepted academic practice. No use, distribution or reproduction is permitted which does not comply with these terms.
*Correspondence: Wei Cao, Y2Fvd2VpNTYxNTIxQGhvdG1haWwuY29t
Disclaimer: All claims expressed in this article are solely those of the authors and do not necessarily represent those of their affiliated organizations, or those of the publisher, the editors and the reviewers. Any product that may be evaluated in this article or claim that may be made by its manufacturer is not guaranteed or endorsed by the publisher.
Research integrity at Frontiers
Learn more about the work of our research integrity team to safeguard the quality of each article we publish.