- 1Laboratory of Cancer Biology, Key Laboratory of Biotherapy of Zhejiang Province, Sir Run Run Shaw Hospital, Zhejiang University School of Medicine, Hangzhou, China
- 2State Key Laboratory for Diagnosis and Treatment of Infectious Diseases, Collaborative Innovation Center for Diagnosis and Treatment of Infectious Disease, The First Affiliated Hospital, Zhejiang University School of Medicine, Hangzhou, China
- 3Cancer Center, Zhejiang University, Hangzhou, China
Golgi phosphoprotein 73 (GP73, also termed as GOLM1 or GOLPH2) is a glycosylated protein residing on cis-Golgi cisternae and highly expressed in various types of cancer tissues. Since GP73 is a secretory protein and detectable in serum derived from cancer patients, it has been regarded as a novel serum biomarker for the diagnosis of different cancers, especially hepatocellular carcinoma (HCC). However, the functional roles of GP73 in cancer development are still poorly understood. In recent years, it has been discovered that GP73 acts as a multifunctional protein-facilitating cancer progression, and strikingly, it has been identified as a leading factor promoting epithelial-mesenchymal transition (EMT) of cancer cells and causing cancer metastasis. In this review, we have overviewed the latest findings of the functional roles of GP73 in elevating cancer progression, especially in facilitating EMT and cancer metastasis through modulating expression, transactivation, and trafficking of EMT-related proteins. In addition, unsolved research fields of GP73 have been lightened, which might be helpful to elucidate the regulatory mechanisms of GP73 on EMT and provide potential approaches in therapeutics against cancer metastasis.
Introduction
In the past decades, cancer has been ranked as the primary cause of death and the largest health problem worldwide (1). The incidence and mortality of cancer are rapidly rising because of aging, growth of the population, environmental pollution, as well as other social problems (1–3). Metastasis and recurrence are the main causes of cancer-related deaths (4). A hypoxic tumor microenvironment is created since a large amount of oxygen is consumed in the metabolism of cancer cells, which challenges the survival of cancer cells. As a result, cancer cells have to shift to places with high oxygen concentrations to promise cell metabolism and resist cell death, the process of which is named cancer metastasis (5). Cancer progression towards metastasis is often depicted as a multistage process and cancer cells achieve metastasis through epithelial-mesenchymal transition (EMT) during the process (6–8). When cancer cells demonstrate a shift towards the mesenchymal state, expression, and modifications of EMT-related molecules are changed, which shape cells into spindle, then, migratory and invasive behaviors of cancer cells are facilitated (9). Some EMT-like cancer cells named as circulating tumor cells (CTCs) invade into blood vessels and migrate with the bloodstream. CTCs can be clustered to evade immune defense and enhance survival of cancer cells in the blood, moreover, high expression of CD44, the cancer-specific surface antigen, facilitates heterotypic adhesion of CTCs, which promises the distant metastasis of CTCs and strives for more nutrients (10, 11).
EMT-associated transcription factors (TFs), such as ZEB1, SNAIL1, and TWIST1 transactivate EMT factors associated with cell adhesion, migration, and invasion (12–14). The repression of such transactivation was proposed as a rational strategy to reverse EMT. However, these well-known EMT-associated TFs are differentially expressed in various cancer types. Therefore, it is valuable to discover a protein regulating most of EMT-related factors, thus allowing a specific small-molecule inhibitor to potentially target EMT of cancer cells.
Characteristics and Structure of GP73
In 2000, a novel protein named Golgi phosphoprotein 73 (GP73, also termed as GOLM1 or GOLPH2) was identified and isolated from the liver of a patient who suffered from adult giant-cell hepatitis (GCH), a rare form of hepatitis with presumed viral etiology (15). GP73 is encoded by GOLM1, and the open reading frame comprises two regions encoding products containing 392 and 401 amino acids (aa) (16). GP73 resides in cis-Golgi cisternae, and it contains a transmembrane domain (TMD) at the N-terminal region (13–35aa) and two α-helixes at the C-terminal region (56–205 and 206–401aa) (16, 17). The cytoplasmic region of GP73 is formed by 1–12aa and, remarkably, GP73 interacts with its substrates via this domain and involves in the vesicular trafficking of these proteins (17–19). Also, three N-linked glycosylated sites (N109, N144, and N398) and two phosphorylated sites (S187 and S309) have been detected using liquid chromatography and high-throughput mass spectrometry (LC-MS/MS), but the exact functions of these modifications remain poorly understood (Figure 1) (20–23). Intracellular vesicles engage in the trafficking process of GP73 from the Golgi apparatus to cell surface, and the secretion of GP73 from the cell surface to extracellular spaces is exosome dependent (18). Furin has been identified as a proteinase to exclusively cleave GP73 at R55 on the intracellular side of the cell surface, which permits the remaining part of GP73 (56–401aa) covered by exosomes and secreted into extracellular spaces via exosome-dependent secretion (24). Therefore, 56–401aa residue of GP73 is detectable in extracellular spaces and potentially used as a serum biomarker for the diagnosis of cancers (25).
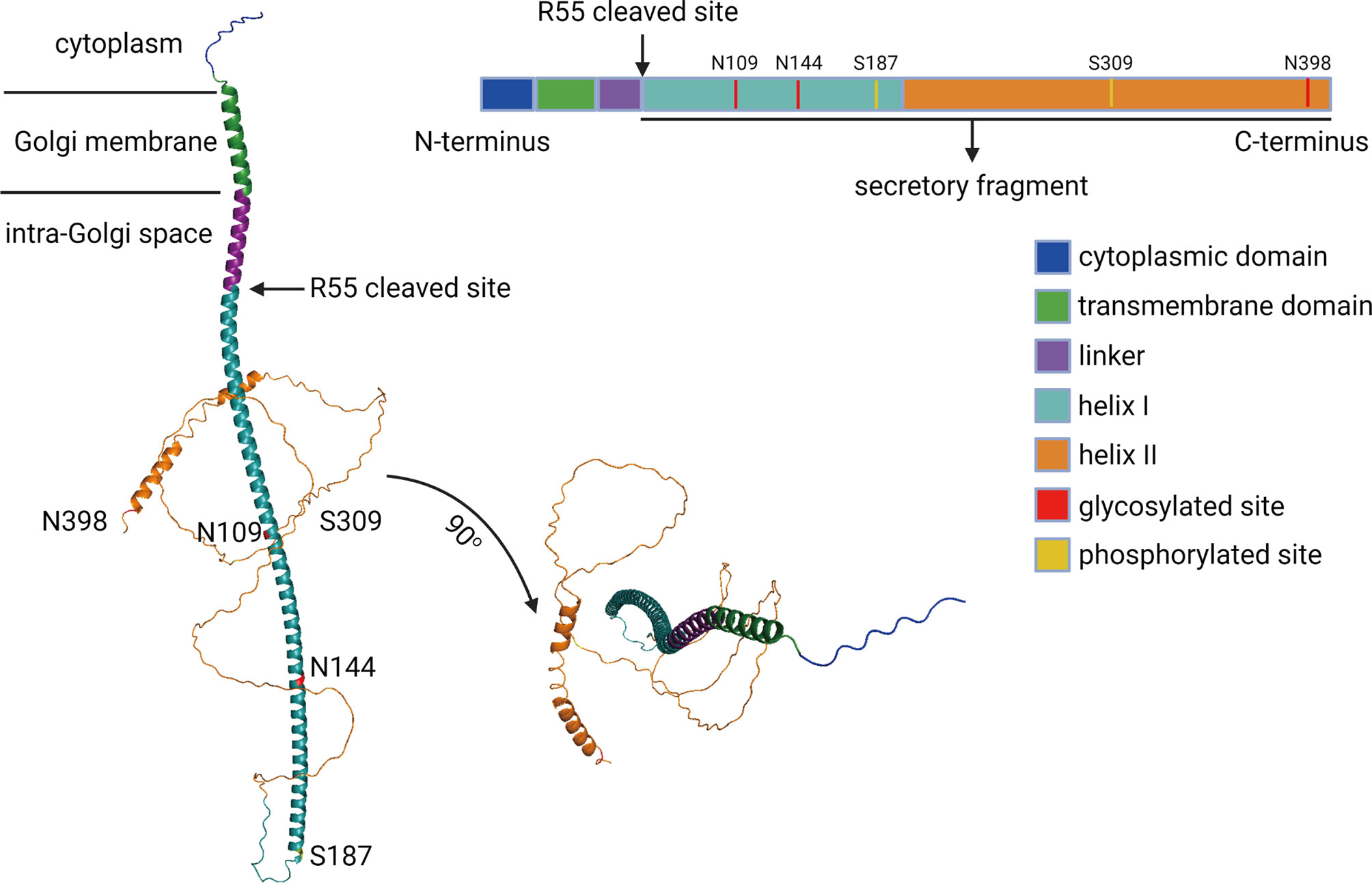
Figure 1 The schematic diagram of GP73. The functional domains, phosphorylated sites, and glycosylated sites of full-length GP73 has been showed. The predicted molecular structure of GP73 was obtained from AlphaFold Protein Structure Database (https://alphafold.ebi.ac.uk).
GP73 Serves as a Biomarker in Cancer Diagnostics
In 2005, a study based on glycoproteomics screened serum glycoproteins and identified serum GP73 as a factor positively correlated with human hepatocellular carcinoma (HCC), which suggested that GP73 serves as a potential serum biomarker for HCC diagnosis (26). Following studies indicate that intracellular GP73 correlates positively with extracellular GP73, and both of them could be potentially used as biomarkers for diagnosis of HCC (23, 24, 27, 28). Notably, GP73 has been indicated to be highly expressed in pathological tissues and serum derived from early cancer patients, which manifests higher diagnostic sensitivity and specificity than classic HCC biomarker alpha-fetoprotein (AFP) (29–31). Thus, GP73 has been used as a novel serum biomarker for clinical diagnostics of HCC. Two follow-up studies have uncovered that GP73 is highly expressed in prostate cancer tissues, which indicate that GP73 may not be an HCC-specific biomarker but potentially applicable for diagnosis of pan-cancers (32, 33). Further studies examined the level of GP73 in different types of cancers, and the results reveal that GP73 is not only an HCC-specific biomarker but also serves as a suitable biomarker for diagnosis of other malignant tumors (Table 1). Similar to HCC, GP73 is also detectable in most other types of early cancers, which suggests that GP73, as a comprehensive and sensitive biomarker, is expected to be applied in clinical diagnostics of different types of cancers.
Transactivation and Expression of GP73 in Cancer Cells
Years after GP73 was identified, the discoverer of GP73 measured the protein level of GP73 in pathological tissues derived from patients with different liver diseases and found that GP73 was highly expressed in patients suffering from acute hepatitis of various etiologies, autoimmune hepatitis, chronic hepatitis C virus (HCV) infection, and alcoholic liver diseases (58). Additional studies have indicated that GP73 is highly expressed in hepatitis B virus (HBV)-infected liver tissues compared with non-HBV-infected liver tissues, implying that viral infection might upregulate expression of GP73 (59–61). Pathogen-associated molecular patterns can be recognized by pattern recognition receptors during the process of viral infection, which leads to the activation and secretion of interferons (IFNs) (62). Therefore, it is supposed that virus might activate GP73 expression via stimulating the expression and secretion of IFNs. Indeed, a recent study reveals that IFN-β activates GP73 expression and represses innate immune response in viral-infected HCC cells through facilitating the degradation of mitochondrial antivirus signaling protein (MAVS)/TNF receptor-associated factor 6 (TRAF6) and attenuating IFN-β promoter (63). However, some other studies indicate that serum GP73 might not be a suitable diagnostic marker for HCC because HBV infection rather than tumorigenesis facilitates GP73 expression (64, 65). Nevertheless, following studies discovered that GP73 is also highly expressed in carcinomas without viral infection, such as nonsmall-cell lung cancer (NSCLC), cutaneous melanoma, cerebroma, prostate cancer, renal cell cancer, and bladder cancer, which suggest that biogenesis of GP73 is regulated by multiple factors and the mechanism is complex (Table 2).
With the rise of researches about micro-RNA (miRNA) in recent years, some studies manifest that the levels of multiple miRNAs targeting the 3′-untranslated region (3′-UTR) of GOLM1 are attenuated in cancer cells, but the regulatory mechanism is still unclear (Table 2). As is well known, miRNAs are not the dominant factors regulating protein expression, it is significant to explain how GP73 is transactivated in viral-infected cells and cancer cells (86–88).
As extracellular stimulations such as epithelial growth factor (EGF), tumor-associated macrophages (TAMs), and immune suppression-related cytokines in tumor microenvironment facilitate cancer progression, it is believed that extracellular factors in tumor microenvironment might play important roles in facilitating GP73 expression (89). It has been discovered that TAM-originated interleukin-1β (IL-1β) can activate the expression of ETS-1, the well-known oncogenic TF, which interacts with the promoter of GOLM1 and promotes its transcription (79). Similarly, a recent study in our group has uncovered that hypoxia upregulates oncogenic protein c-Myc and transactivates GP73 in a mildly hypoxic tumor microenvironment, which suggests that GP73 might be activated to play critical roles against adverse circumstances and promote the survival of cancer cells (Figure 2) (19).
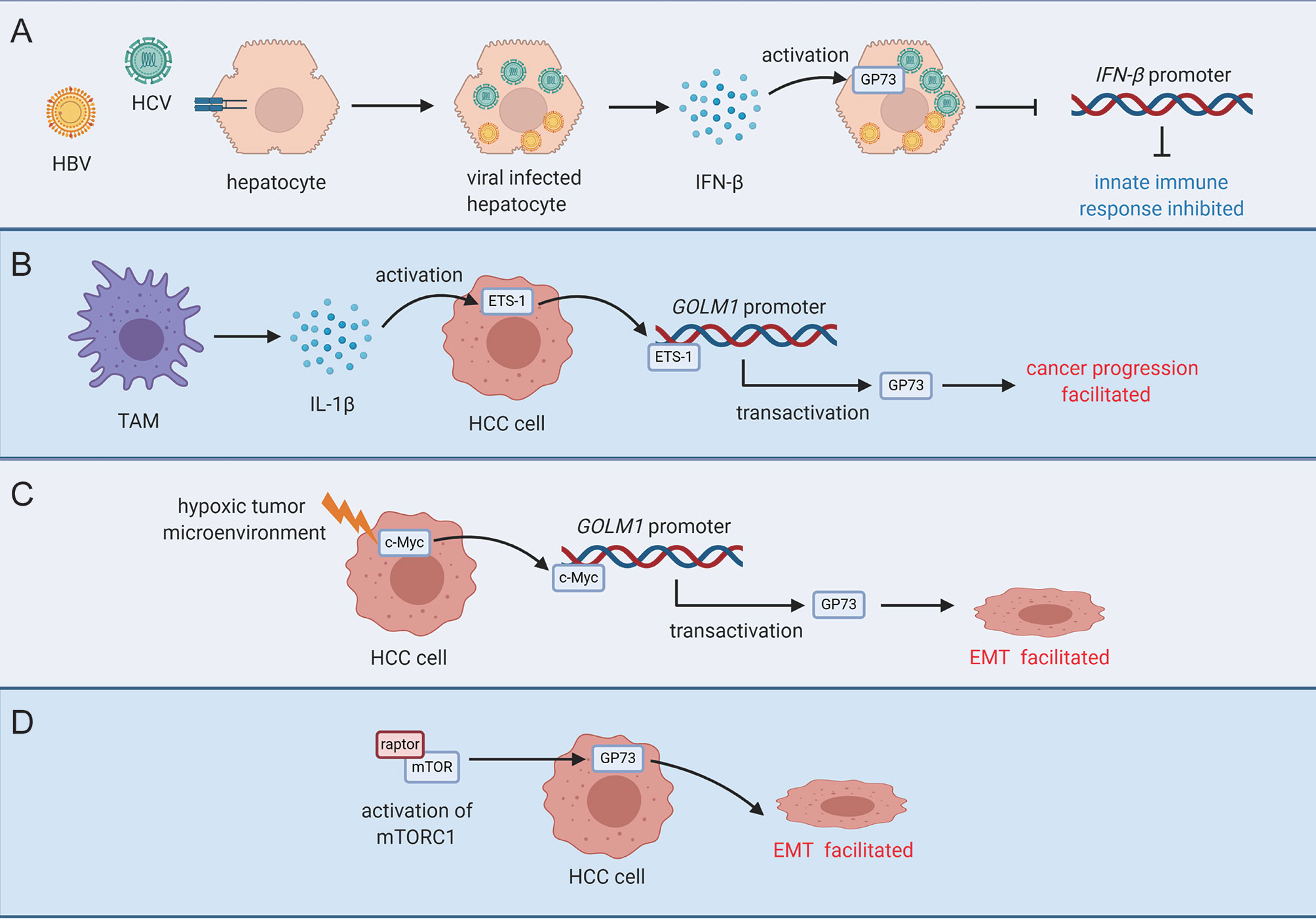
Figure 2 Activation modes of GP73 in viral infected cells or cancer cells. (A) INF-β secreted by viral-infected hepatocytes activates GP73 in target cells and inhibits innate immune response. (B) TAM-secreted IL-1β upregulates ETS-1 in cancer cells and ETS-1 transactivates GP73 to facilitate cancer progression. (C) Upregulation of c-Myc in a mildly hypoxic tumor microenvironment transactivates GP73 and facilitates EMT of cancer cells. (D) GP73 is upregulated by mTORC1, and EMT of cancer cells is facilitated.
It is known that the mammalian target of rapamycin complex 1 (mTORC1) is involved in physiological processes including protein synthesis, cell metabolism, tumor proliferation, and autophagy; however, its functional roles in cancer cells are still poorly understood since the regulatory mechanisms are complex (90–92). An early study has reported that mTORC1 upregulates GP73 in HCC cells and promotes cell proliferation (34). It is well-known that activation of mTORC1 facilitates phosphorylation of p70-S6 kinase (S6K) and eukaryotic translation initiation factor 4E-binding protein 1 (4EBP1), two factors that engage in protein synthesis, and accelerate HCC proliferation (93). This study, however, has indicated that GP73 directly upregulates S6K, then promotes protein synthesis and cell proliferation. Therefore, GP73, as the downstream protein of mTORC1, plays synergistic roles with mTORC1 in facilitating carcinogenesis. Additionally, RNA sequencing in this study has revealed that knockdown of GP73 reduces the levels of matrix metalloproteinase-7 (MMP-7) and CD44, two factors involved in cell invasion, heterotypic adhesion, and HCC metastasis; however, the regulatory mechanisms are unclear (94–96).
The results above have elucidated how GP73 is activated in cancer cells; furthermore, it is proved that GP73 takes important effects on cancer metastasis as well as cancer proliferation and promotes cancer progression.
GP73 Promotes EMT of Cancer Cells
It has been mentioned above that GP73 facilitates cancer metastasis as well as proliferation, and GP73 upregulates MMP-7 and CD44, the factors highly expressed in metastatic cancer cells. Therefore, it is deemed that GP73 might promote EMT of cancer cells through upregulating the levels of EMT-related proteins.
Clinical studies have also demonstrated that GP73 is highly expressed in cancer tissues with infiltration (Table 3). However, since it is difficult to obtain metastatic tissues after cancer recurrence, no study has reported the expression of GP73 in distant metastatic tissues.
Whatever, in recent years, an increasing number of studies have illustrated the functional roles of GP73 in cancer metastasis. In a pioneering study, with the help of laser-capture tissue microdissection and genome-wide cDNA arrays technologies, GOLM1 was identified as a leading gene significantly upregulated in tumor tissues from HCC patients with extrahepatic metastases (EHMH) but not in tissues from metastasis-free HCC (MFH) patients, which suggests that GP73 is a critical factor modulating cancer metastasis (17). Since metastasis is the main cause of cancer-related death and EMT is the essential condition of metastasis, it is clinically significant to investigate the regulatory mechanisms of GP73 on cancer metastasis (100). Following studies have focused on the mechanisms of how GP73 facilitates cancer metastasis, and they have discovered that highly expressed GP73 upregulates the levels of N-cadherin, vimentin, and MMP-13 in HCC cells, which prove that GP73 surely serves as a multifunctional factor modulating the expression of EMT-related proteins (Table 4). Also, GP73 negatively regulates the expression of E-cadherin, the well-known adhesion factor, and promotes EMT through attenuating cell adhesion (103, 108). One of these studies has demonstrated that GP73 upregulates c-AMP element response binding protein (CREB), a common TF highly expressed in cancer cells, and transactivated MMP-13; however, the mechanism is not totally elucidated and no other EMT-associated TFs have been discovered to be regulated by GP73 (104, 109, 110).
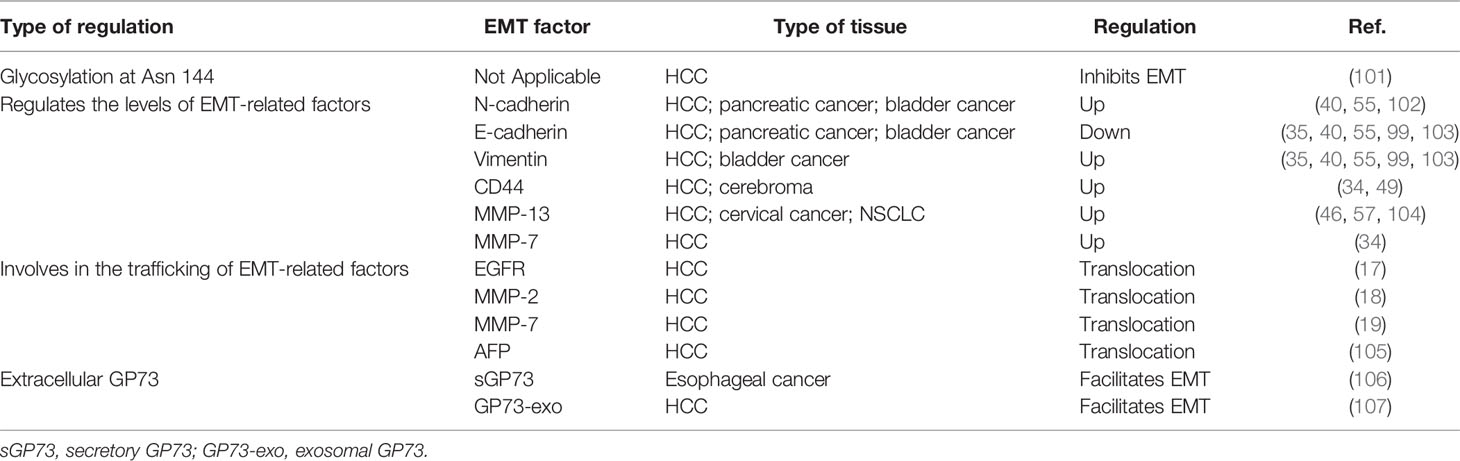
Table 4 GP73 regulates expression and trafficking of EMT-related factors and facilitates cancer metastasis.
On the other hand, since GP73 is a highly glycosylated and phosphorylated protein, it is supposed that specific modified sites of GP73 might impact the process of EMT. LC-MS/MS analysis has discovered that GP73 is N-glycosylated at Asn109, Asn144, and Asn398 (101). Following analyses have demonstrated that removal of N-linked glycosylation of GP73 at Asn144 enhances metastasis of HCC cells, which proves that modified sites of GP73 impact its functions in facilitating EMT. It is believed that other phosphorylated and glycosylated sites might also take effect on EMT, which is worth further exploring.
GP73 Acts as a Transporter of EMT-Related Proteins
As described, GP73 facilitates EMT of cancer cells through regulating the expressions of EMT-related proteins, but the mechanisms are still poorly understood. For solving these puzzles, GP73-interacted proteins were identified using coimmunoprecipitation combined with LC-MS/MS, and epithelial growth factor receptor (EGFR) was identified as a critical GP73-interacted factor in HCC cells, which interacts with GP73 via the cytoplasmic domain of GP73 (17, 111, 112). Since GP73 is a transmembrane protein and the cytoplasmic domain resides on the outside of the membrane of cis-Golgi cisternae and intracellular vesicles, it is suggested that EGFR is translocated onto the cell surface and exerts its biological functions through GP73-dependent vesicular trafficking. Following fluorescent protein-based live-cell imaging and functional experiments have proved the hypothesis. The study above has indicated that GP73 acts as a transporter facilitating the trafficking and translocation of EMT-related proteins, promising EMT of cancer cells promoted.
Similarly, in our early studies, it was observed that knockdown of GP73 induced the accumulation of intracellular matrix metalloproteinase-2 (MMP-2) and MMP-7 but attenuated the levels of extracellular MMP-2 and MMP-7, which suggests that knockdown of GP73 might block the trafficking and secretion of matrix metalloproteinases (MMPs) (18, 19). Further pieces of evidence prove that, as well as GP73/EGFR interaction, MMP-2 and MMP-7 interact with the cytoplasmic domain of GP73, and GP73 is involved in their translocation from cytosol to extracellular spaces through GP73-mediated vesicular trafficking. These findings have manifested that the trafficking of MMPs is GP73 dependent. Therefore, GP73 has been deemed as a transporter for trafficking of EMT-associated factors and facilitating EMT of cancer cells.
Also, it has been revealed that GP73 interacts with Rab11, a lysosome-dependent degradation-related protein residing on the membrane of intracellular vesicles, and mediates lysosome-dependent degradation of EGFR (17, 113). When weak signal is activated, the GP73-Rab11 complex mediates the trafficking of EGFR from cis-Golgi cisternae to lysosome and promotes the degradation of EGFR. Oppositely, GP73 facilitates the polarized delivery of EGFR from cis-Golgi to the plasma membrane when EGFR signaling pathway is activated, which enhances the activation of EGFR signaling pathway. The findings show that GP73 is a switch-modulating metastasis, metabolism, and dormancy of HCC cells through regulating the translocation of growth factor receptors. It has also indicated that GP73 not only modulates the trafficking of EMT-related proteins from cytosol to cell surface or extracellular spaces but also involves in protein recycling and energy saving.
Moreover, an updated study indicated that GP73 directly interacted with AFP and facilitated its secretion, which led to EMT of recipient cells of AFP and promoted immune escape of cancer cells (105).
Taken together, GP73, as a cis-Golgi cisternae-resided protein, exerts its functional roles in the trafficking and recycling of EMT-related factors and promotes EMT of cancer cells.
Extracellular GP73 Facilitates EMT of Cancer Cells
As described, intracellular GP73 can be cleaved at the trans-Golgi network (TGN) due to saturation or mini-stack formation, or cleaved by furin proteinase on the intracellular side of cell surface, then released into extracellular spaces via exosomes (18, 24, 106). The studies have elucidated how GP73 is cleaved and secreted into extracellular spaces; however, the functional roles of extracellular GP73 remain poorly understood. A previous study has indicated that overexpression of GP73 1-55aa-deleted truncated mutant facilitates cell invasion (104). Since exosomal GP73 shares an identical sequence and structure with GP73 1-55aa-deleted truncated mutant, it is supposed that exosomal GP73 might facilitate metastasis of neighboring cancer cells while it is captured by recipient cells. A recent study has discovered that mTOR upregulates GP73 through reducing the level of miR-145, the miRNA targeting 3′UTR of GOLM1, and exosomal GP73 facilitated proliferation and invasion of neighboring cancer cells by upregulating glycogen synthase kinase-3β (GSK-3β) and MMPs (107). Though exosomal GP73 upregulates proliferation and cell invasion-related proteins of recipient cells, it has not elucidated how it activates the expressions of these target factors. Therefore, the molecular mechanisms need further investigations.
The studies above have indicated that cancer cell-originated exosomal GP73 acts as a messenger that functionally activates growth and EMT of recipient cells, which suggests that exosomal GP73 plays vital roles in cell-to-cell interactions in cancer microenvironment.
Conclusion and prospective
GP73 plays functional roles in facilitating EMT of cancer cells through multiple pathways, which proves that GP73 goes beyond a tumor biomarker for cancer diagnosis (Figure 3). As previously reported, GOLM1 has been identified as a leading gene associated with cancer metastasis; it is supposed that GP73 serves as a potential drug target in therapeutics of metastatic cancers (17). Fortunately, as a tumor biomarker, GP73 expresses little in normal tissues, and previous studies have proved that GP73 deletion impacts little on the physiological activities of mice (25, 34). Therefore, it is significant to explore small-molecule inhibitors targeting GP73 for potential therapeutics against cancer metastasis. As reported that tunicamycin, the drug inhibiting N-linked glycosylation of proteins, prevents the glycosylation of GP73 and attenuates its functions in facilitating HCC metastasis, it is potentially utilized in cancer therapy (34, 114). However, since it is a comprehensive inhibitor targeting almost all glycosylated proteins and inducing high cytotoxicity to normal cells, it is not suitable for GP73-targeted clinical therapeutics. Therefore, it is interesting and important to explore novel GP73-specific inhibitors for therapeutics against cancer metastasis.
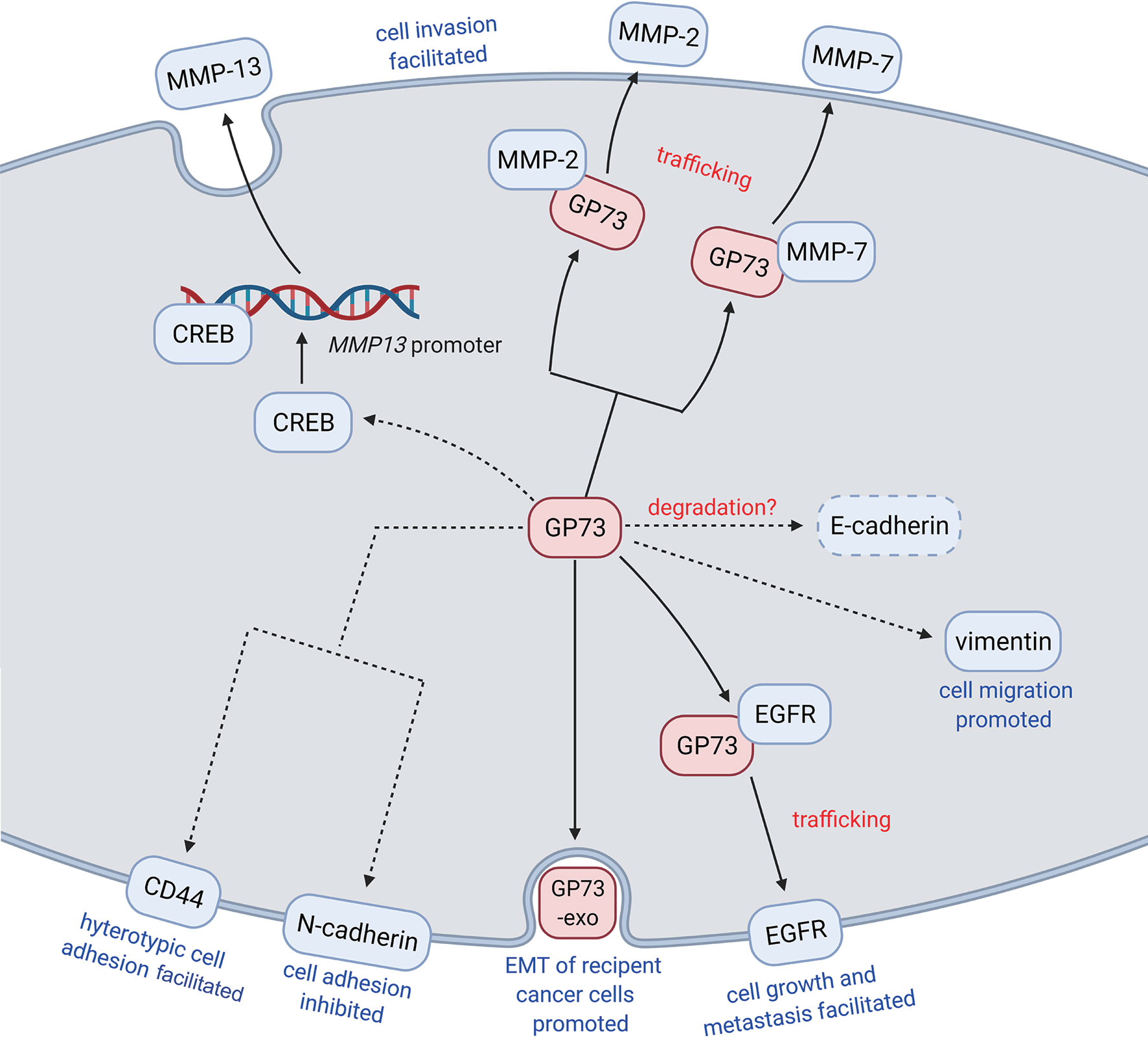
Figure 3 GP73 promotes EMT of cancer cells through different pathways. GP73, as a multifunctional protein, facilitates EMT of cancer cells through regulating the levels, promoting the transactivation, and accelerating the trafficking of EMT-related proteins. Exosomal GP73 also plays functional roles in promoting EMT of recipient cancer cells.
Since GP73 facilitates EMT of cancer cells through regulating expression, trafficking, and secretion of EMT-related proteins, it is supposed that GP73 acts as a vital factor exerting a wide range of physiological functions in cancer cells and the functional roles of GP73 in cancer microenvironment are far more beyond our recognition. Herein, five concerning issues about GP73 are highlighted and further studies might be helpful to explain the regulatory mechanisms and confirm the diagnostic ranges of GP73.
Above all, as described, GP73 is involved in the trafficking of EGFR, MMP-2, and MMP-7. It is considered a transporter assisting the trafficking of EMT-related factors. Therefore, GP73 might facilitate proliferation and metastasis of cancer cells by promoting the trafficking of carcinogenesis-associated cell-surface receptors or secretory proteins. It is believed that more substrates might facilitate EMT of cancer cells through GP73-mediated trafficking.
Secondly, GP73 also plays functional roles in promoting proteasome-dependent degradation of target proteins, such as MAVS and TRAF6 (63). Therefore, GP73 is not only a transporter facilitating the trafficking of cell surface and secretory proteins but also a recycler promoting degradation of intracellular proteins. As shown that overexpression of GP73 reduced the level of E-cadherin, it is worth investigating whether GP73 is involved in proteasome-dependent degradation of E-cadherin (35). In addition, since it has been revealed that GP73 is also involved in the lysosome-dependent degradation of target proteins, it is also interesting and meaningful to discover its substrates in lysosome-dependent degradation (17).
Thirdly, as mentioned above, exosomal GP73 facilitates cell proliferation and metastasis through activating GSK-3β and MMP-related signaling pathways (107). However, it is unclear how GP73 activates these signaling pathways. Therefore, LC-MS/MS and RNA sequencing are essential here to identify the exosomal GP73-interacted proteins and mechanically explain how it facilitates cell growth and EMT.
Fourthly, the pieces of evidence above have indicated that cancer cell-originated exosomal GP73 facilitates growth and EMT of neighboring cells, which implies that it might exert important functions in tumor microenvironment. An early study has described that exosomal GP73 induces endoplasmic reticulum stress of macrophages, which stimulates the secretion of cytokines and chemokines involved in the formation of TAMs (115). Also, two recent studies have reported that GP73 upregulates programmed cell death ligand-1 (PD-L1) and facilitates immune escape of HCC cells through activating EGFR signaling pathway, which prove that, similar to the former study, GP73 also plays key roles in immunomicroenvironment (98, 116). On the contrary, another latest study has indicated that GP73 maintains the intestinal epithelial barrier and suppresses carcinogenesis of colorectal carcinoma (CRC) through restraining protumorigenic inflammation (117). Thus, GP73 not only regulates cell growth and EMT but also involves in immunoregulation and indirectly modulates cancer progression, which deserves further investigation.
Fifthly, since HBV infection upregulates the expression of GP73, its diagnostic values in HCC and other liver diseases are challenged (65). More pathological samples derived from HBV or non-HBV-infected HCC patients are expected to be analyzed to clarify its range of application in diagnostics.
Lastly, since it has been reported that knockdown of GP73 could inhibit cancer proliferation and metastasis in vitro and in vivo, it is deemed that GP73 might serve as a potential drug target (17, 19, 34, 115). Therefore, it is meaningful to explore small molecule inhibitors targeting intracellular and extracellular GP73 and assess their application values in cancer therapeutics.
Herein, the GP73-interacted proteins and functions have been summarized, which might help readers to comprehend its actions in physiological and biochemical processes (Figure 4). Though it has been known that GP73 is a critical factor facilitating EMT of cancer cells, the functions of which are still beyond our recognition. The highlighted points above might illuminate us to gradually uncover the physiological functions of GP73 in cancer cells, which might be helpful in the diagnosis and treatment of cancer metastasis.
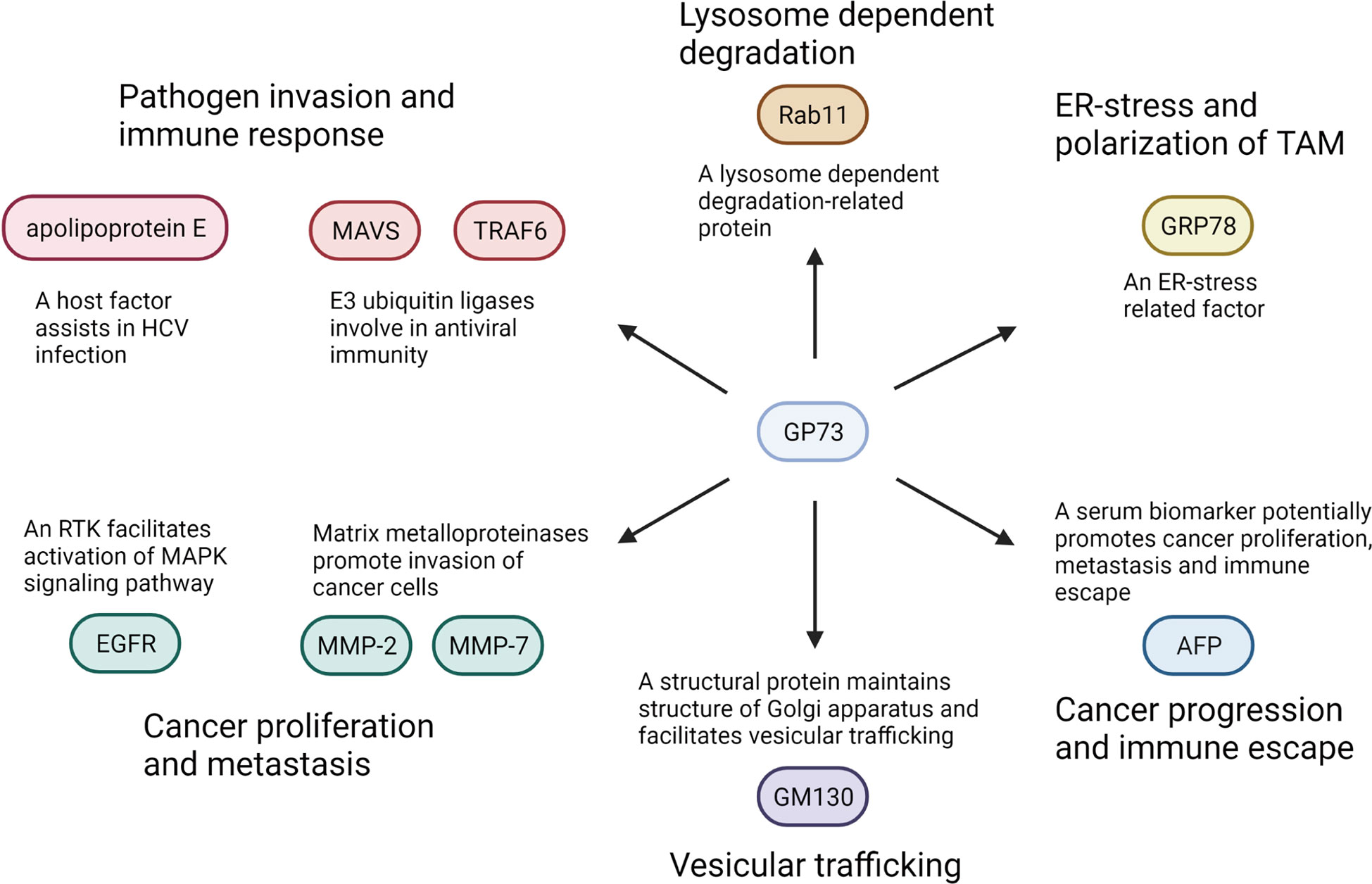
Figure 4 GP73-interacted proteins and their functions in physiological and biomedical processes. GP73 interacts with indicated proteins and facilitates cancer progression or pathogen invasion through various pathways.
Author Contributions
YL assigned the outlines of the manuscript and wrote the manuscript. XH collected relevant references and produced tables. SL analyzed clinical data in Table 3. SZ drew the figures. ZC and HJ revised the manuscripts. All authors contributed to the article and approved the submitted version.
Funding
This work was supported by the Natural Science Foundation of Zhejiang province (grant numbers LR19H160003), the Natural Science Foundation of Zhejiang province (grant numbers LQ21H160029), and the Medical Science and Health Technology Project of Zhejiang province (grant numbers 2020RC067).
Conflict of Interest
The authors declare that the research was conducted in the absence of any commercial or financial relationships that could be construed as a potential conflict of interest.
Publisher’s Note
All claims expressed in this article are solely those of the authors and do not necessarily represent those of their affiliated organizations, or those of the publisher, the editors and the reviewers. Any product that may be evaluated in this article, or claim that may be made by its manufacturer, is not guaranteed or endorsed by the publisher.
Acknowledgments
All figures were created with BioRender.com.
Abbreviations
GP73, Golgi phosphoprotein 73; GOLM1, Golgi membrane protein 1; GOLPH2, Golgi phosphoprotein 2; HCC, hepatocellular carcinoma; EMT, epithelial mesenchymal transition; CTCs, circulating tumor cells; TFs, transcription factors; GCH, giant-cell hepatitis; aa, amino acids; TMD, transmembrane domain; LC-MS/MS, liquid chromatography and high-throughput mass spectrometry; AFP, alpha-fetoprotein; HCV, hepatitis C virus; HBV, hepatitis B virus; IFNs, interferons; NSCLC, nonsmall-cell lung cancer; miRNA, micro-RNA; 3’ UTR, 3′-untranslated region; EGF, epithelial growth factor; TAMs, tumor-associated macrophages; IL-1β, interleukin-1β; mTORC1, mammalian target of rapamycin complex 1; S6K, p70-S6 kinase; 4EBP1, eukaryotic translation initiation factor 4E-binding protein 1; MMP-7, matrix metalloproteinase-7; EHMH, extrahepatic metastases; MFH, metastasis-free HCC; CREB, c-AMP element response binding protein; EGFR, epithelial growth factor receptor; MMP-2, matrix metalloproteinase-2; MMPs, matrix metalloproteinases; TGN, trans-Golgi-network; GSK-3β, glycogen synthase kinase-3β; MAVS, mitochondrial antivirus signaling protein; TRAF6, TNF receptor-associated factor 6; PD-L1, programmed cell death ligand-1; CRC, colorectal carcinoma; ESCC, esophageal squamous cell carcinoma; OSCC, oral squamous cell carcinoma; sGP73, secretory GP73.
References
1. Bray F, Ferlay J, Soerjomataram I, Siegel RL, Torre LA, Jemal A. Global Cancer Statistics 2018: GLOBOCAN Estimates of Incidence and Mortality Worldwide for 36 Cancers in 185 Countries. CA: A Cancer J Clin (2018) 68:394–424. doi: 10.3322/caac.21492
2. Balducci L, Ershler WB. Cancer and Ageing: A Nexus at Several Levels. Nat Rev Cancer (2005) 5:655–62. doi: 10.1038/nrc1675
3. Yang J, Siri JG, Remais JV, Cheng Q, Zhang H, Chan K, et al. The Tsinghua-Lancet Commission on Healthy Cities in China: Unlocking the Power of Cities for a Healthy China. Lancet (2018) 391:2140–84. doi: 10.1016/S0140-6736(18)30486-0
4. Nguyen DX, Bos PD, Massagué J. Metastasis: From Dissemination to Organ-Specific Colonization. Nat Rev Cancer (2009) 9:274–84. doi: 10.1038/nrc2622
5. Rankin EB, Giaccia AJ. Hypoxic Control of Metastasis. Science (2016) 352:175–80. doi: 10.1126/science.aaf4405
6. Christofori G. New Signals From the Invasive Front. Nature (2006) 441:444–50. doi: 10.1038/nature04872
7. Steeg PS. Tumor Metastasis: Mechanistic Insights and Clinical Challenges. Nat Med (2006) 12:895–904. doi: 10.1038/nm1469
8. Dongre A, Weinberg RA. New Insights Into the Mechanisms of Epithelial–Mesenchymal Transition and Implications for Cancer. Nat Rev Mol Cell Bio (2019) 20:69–84. doi: 10.1038/s41580-018-0080-4
9. Beug H. Breast Cancer Stem Cells: Eradication by Differentiation Therapy? Cell (2009) 138:623–5. doi: 10.1016/j.cell.2009.08.007
10. Massague J, Obenauf AC. Metastatic Colonization by Circulating Tumour Cells. Nature (2016) 529:298–306. doi: 10.1038/nature17038
11. Egeblad M, de Visser KE. Sticking Together Helps Cancer to Spread. Nature (2019) 566:459–60. doi: 10.1038/d41586-019-00341-4
12. Jolly MK. Implications of the Hybrid Epithelial/Mesenchymal Phenotype in Metastasis. Front Oncol (2015) 5:155. doi: 10.3389/fonc.2015.00155
13. Korpal M, Lee ES, Hu G, Kang Y. The miR-200 Family Inhibits Epithelial-Mesenchymal Transition and Cancer Cell Migration by Direct Targeting of E-Cadherin Transcriptional Repressors ZEB1 and ZEB2. J Biol Chem (2008) 283:14910–4. doi: 10.1074/jbc.C800074200
14. Brabletz T, Kalluri R, Nieto MA, Weinberg RA. EMT in Cancer. Nat Rev Cancer (2018) 18:128–34. doi: 10.1038/nrc.2017.118
15. Kladney RD, Bulla GA, Guo L, Mason AL, Tollefson AE, Simon DJ, et al. GP73, a Novel Golgi-Localized Protein Upregulated by Viral Infection. Gene (2000) 249:53–65. doi: 10.1016/S0378-1119(00)00136-0
16. Hu L, Li L, Xie H, Gu Y, Peng T. The Golgi Localization of GOLPH2 (GP73/GOLM1) Is Determined by the Transmembrane and Cytoplamic Sequences. PloS One (2011) 6:e28207. doi: 10.1371/journal.pone.0028207
17. Ye Q, Zhu W, Zhang J, Qin Y, Lu M, Lin G, et al. GOLM1 Modulates EGFR/RTK Cell-Surface Recycling to Drive Hepatocellular Carcinoma Metastasis. Cancer Cell (2016) 30:444–58. doi: 10.1016/j.ccell.2016.07.017
18. Liu Y, Zhang X, Zhou S, Shi J, Xu Y, He J, et al. Knockdown of Golgi Phosphoprotein 73 Blocks the Trafficking of Matrix Metalloproteinase-2 in Hepatocellular Carcinoma Cells and Inhibits Cell Invasion. J Cell Mol Med (2019) 23:2399–409. doi: 10.1111/jcmm.14055
19. Liu Y, Zhou S, Shi J, Zhang X, Shentu L, Chen Z, et al. C-Myc Transactivates GP73 and Promotes Metastasis of Hepatocellular Carcinoma Cells Through GP73-Mediated MMP-7 Trafficking in a Mildly Hypoxic Microenvironment. Oncogenesis (2019) 8:58. doi: 10.1038/s41389-019-0166-7
20. Liu T, Qian W, Gritsenko MA, Camp DG, Monroe ME, Moore RJ, et al. Human Plasma N-Glycoproteome Analysis by Immunoaffinity Subtraction, Hydrazide Chemistry, and Mass Spectrometry. J Proteome Res (2005) 4:2070–80. doi: 10.1021/pr0502065
21. Bian Y, Song C, Cheng K, Dong M, Wang F, Huang J, et al. An Enzyme Assisted RP-RPLC Approach for in-Depth Analysis of Human Liver Phosphoproteome. J Proteomics (2014) 96:253–62. doi: 10.1016/j.jprot.2013.11.014
22. Tagliabracci VS, Wiley SE, Guo X, Kinch LN, Durrant E, Wen J, et al. A Single Kinase Generates the Majority of the Secreted Phosphoproteome. Cell (2015) 161:1619–32. doi: 10.1016/j.cell.2015.05.028
23. Norton PA, Comunale MA, Krakover J, Rodemich L, Pirog N, D'Amelio A, et al. N-Linked Glycosylation of the Liver Cancer Biomarker GP73. J Cell Biochem (2008) 104:136–49. doi: 10.1002/jcb.21610
24. Bachert C, Fimmel C, Linstedt AD. Endosomal Trafficking and Proprotein Convertase Cleavage of Cis Golgi Protein GP73 Produces Marker for Hepatocellular Carcinoma. Traffic (2007) 8:1415–23. doi: 10.1111/j.1600-0854.2007.00621.x
25. Mao Y, Yang H, Xu H, Lu X, Sang X, Du S, et al. Golgi Protein 73 (GOLPH2) is a Valuable Serum Marker for Hepatocellular Carcinoma. Gut (2010) 59:1687–93. doi: 10.1136/gut.2010.214916
26. Block TM, Comunale MA, Lowman M, Steel LF, Romano PR, Fimmel C, et al. Use of Targeted Glycoproteomics to Identify Serum Glycoproteins That Correlate With Liver Cancer in Woodchucks and Humans. Proc Natl Acad Sci USA (2005) 102:779–84. doi: 10.1073/pnas.0408928102
27. Marrero JA, Romano PR, Nikolaeva O, Steel L, Mehta A, Fimmel CJ, et al. GP73, a Resident Golgi Glycoprotein, is a Novel Serum Marker for Hepatocellular Carcinoma. J Hepatol (2005) 43:1007–12. doi: 10.1016/j.jhep.2005.05.028
28. Block TM, Marrero J, Gish RG, Sherman M, London WT, Srivastava S, et al. The Degree of Readiness of Selected Biomarkers for the Early Detection of Hepatocellular Carcinoma: Notes From a Recent Workshop. Cancer biomark (2008) 4:19–33. doi: 10.3233/CBM-2008-4103
29. Hu J, Wu D, Liang S, Miao X. GP73, a Resident Golgi Glycoprotein, is Sensibility and Specificity for Hepatocellular Carcinoma of Diagnosis in a Hepatitis B-Endemic Asian Population. Med Oncol (2010) 27:339–45. doi: 10.1007/s12032-009-9215-y
30. Malaguarnera G, Giordano M, Paladina I, Berretta M, Cappellani A, Malaguarnera M. Serum Markers of Hepatocellular Carcinoma. Digest Dis Sci (2010) 55:2744–55. doi: 10.1007/s10620-010-1184-7
31. Li X, Wu K, Fan D. Serum Golgi Phosphoprotein 2 Level: A Better Marker Than Alpha-Fetoprotein for Diagnosing Early Hepatocellular Carcinoma. Hepatology (2009) 50:325. doi: 10.1002/hep.23028
32. Wei S, Dunn TA, Isaacs WB, De Marzo AM, Luo J. GOLPH2 and MYO6: Putative Prostate Cancer Markers Localized to the Golgi Apparatus. Prostate (2008) 68:1387–95. doi: 10.1002/pros.20806
33. Kristiansen G, Fritzsche FR, Wassermann K, Jäger C, Tölle A, Lein M, et al. GOLPH2 Protein Expression as a Novel Tissue Biomarker for Prostate Cancer: Implications for Tissue-Based Diagnostics. Brit J Cancer (2008) 99:939–48. doi: 10.1038/sj.bjc.6604614
34. Chen X, Wang Y, Tao J, Shi Y, Gai X, Huang F, et al. Mtorc1 Up-Regulates GP73 to Promote Proliferation and Migration of Hepatocellular Carcinoma Cells and Growth of Xenograft Tumors in Mice. Gastroenterology (2015) 149:741–52. doi: 10.1053/j.gastro.2015.05.005
35. Yang Y, Liu Q, Li Z, Zhang R, Jia C, Yang Z, et al. GP73 Promotes Epithelial–Mesenchymal Transition and Invasion Partly by Activating TGF-β1/Smad2 Signaling in Hepatocellular Carcinoma. Carcinogenesis (2018) 39:900–10. doi: 10.1093/carcin/bgy010
36. Riener M, Stenner F, Liewen H, Hellerbrand C, Bahra M, Kristiansen G. Alpha-Fetoprotein and Serum Golgi Phosphoprotein 2 are Equally Discriminative in Detecting Early Hepatocellular Carcinomas. Hepatology (2009) 50:326. doi: 10.1002/hep.23053
38. Liu G, Zhang Y, He F, Li J, Wei X, Li Y, et al. Expression of GOLPH2 is Associated With the Progression of and Poor Prognosis in Gastric Cancer. Oncol Rep (2014) 32:2077–85. doi: 10.3892/or.2014.3404
39. Tang QF, Ji Q, Tang Y, Hu SJ, Bao YJ, Peng W, et al. Golgi Phosphoprotein 2 Down-Regulates the Th1 Response in Human Gastric Cancer Cells by Suppressing IL-12a. Asian Pac J Cancer Prev (2013) 14:5747–51. doi: 10.7314/APJCP.2013.14.10.5747
40. Song Y, Xu Z, Li H, Yang P, Du J, Xu J. Overexpression of GP73 Promotes Cell Invasion, Migration and Metastasis by Inducing Epithelial-Mesenchymal Transition in Pancreatic Cancer. Pancreatology (2018) 18:812–21. doi: 10.1016/j.pan.2018.08.009
41. Duan J, Li X, Huang S, Zeng Y, He Y, Liu H, et al. GOLPH2, a Gene Downstream of Ras Signaling, Promotes the Progression of Pancreatic Ductal Adenocarcinoma. Mol Med Rep (2018) 17:4187–94. doi: 10.3892/mmr.2018.8430
42. Zhang H, Lin W, Kannan K, Luo L, Li J, Chao P, et al. Aberrant Chimeric RNA GOLM1-MAK10 Encoding a Secreted Fusion Protein as a Molecular Signature for Human Esophageal Squamous Cell Carcinoma. Oncotarget (2013) 4:2135–43. doi: 10.18632/oncotarget.1465
43. Li H, Yang L, Xiao Y, Deng W, Chen L, Wu L, et al. Overexpression of Golgi Phosphoprotein 2 Is Associated With Poor Prognosis in Oral Squamous Cell Carcinoma. Am J Clin Pathol (2018) 150:74–83. doi: 10.1093/ajcp/aqy029
44. Liu X, Chen L, Zhang T. Increased GOLM1 Expression Independently Predicts Unfavorable Overall Survival and Recurrence-Free Survival in Lung Adenocarcinoma. Cancer Control (2018) 25:544118056. doi: 10.1177/1073274818778001
45. Zhang F, Gu Y, Li X, Wang W, He J, Peng T. Up-Regulated Golgi Phosphoprotein 2 (GOLPH2) Expression in Lung Adenocarcinoma Tissue. Clin Biochem (2010) 43:983–91. doi: 10.1016/j.clinbiochem.2010.05.010
46. Aruna, Li LM. Overexpression of Golgi Membrane Protein 1 Promotes non-Small-Cell Carcinoma Aggressiveness by Regulating the Matrix Metallopeptidase 13. Am J Cancer Res (2018) 8:551–65.
47. Zhang Y, Hu W, Wang L, Han B, Lin R, Wei N. Association of GOLPH2 Expression With Survival in non-Small-Cell Lung Cancer: Clinical Implications and Biological Validation. Biomark Med (2017) 11:967–77. doi: 10.2217/bmm-2017-0199
48. Donizy P, Kaczorowski M, Biecek P, Halon A, Szkudlarek T, Matkowski R. Golgi-Related Proteins GOLPH2 (GP73/GOLM1) and GOLPH3 (GOPP1/MIDAS) in Cutaneous Melanoma: Patterns of Expression and Prognostic Significance. Int J Mol Sci (2016) 17:1619. doi: 10.3390/ijms17101619
49. Yuan Y, Shi L, Wang S. Expression and Correlation of CD44 and GP73 in Cerebroma Tissues. Oncol Lett (2018) 15:4958–62. doi: 10.3892/ol.2018.7904
50. Xu R, Ji J, Zhang X, Han M, Zhang C, Xu Y, et al. PDGFA/Pdgfrα-Regulated GOLM1 Promotes Human Glioma Progression Through Activation of AKT. J Exp Clin Canc Res (2017) 36:193. doi: 10.1186/s13046-017-0665-3
51. Yan G, Ru Y, Wu K, Yan F, Wang Q, Wang J, et al. GOLM1 Promotes Prostate Cancer Progression Through Activating PI3K-AKT-mTOR Signaling. Prostate (2017) 78:166–77. doi: 10.1002/pros.23461
52. Li W, Wang X, Li B, Lu J, Chen G. Diagnostic Significance of Overexpression of Golgi Membrane Protein 1 in Prostate Cancer. Urology (2012) 80:951–2. doi: 10.1016/j.urology.2012.06.017
53. Varambally S, Laxman B, Mehra R, Cao Q, Dhanasekaran SM, Tomlins SA, et al. Golgi Protein GOLM1 Is a Tissue and Urine Biomarker of Prostate Cancer. Neoplasia (2008) 10:1235–85. doi: 10.1593/neo.08922
54. Fritzsche FR, Riener M, Dietel M, Moch H, Jung K, Kristiansen G. GOLPH2 Expression in Renal Cell Cancer. BMC Urol (2008) 8:15. doi: 10.1186/1471-2490-8-15
55. Yang H, Liu G, Liu B, Liu T. GP73 Promotes Invasion and Metastasis of Bladder Cancer by Regulating the Epithelial-Mesenchymal Transition Through the TGF-β1/Smad2 Signalling Pathway. J Cell Mol Med (2018) 22:1650–65. doi: 10.1111/jcmm.13442
56. Fritzsche FR, Kristiansen G, Riener M, Dietel M, Oelrich B. GOLPH2 Expression may Serve as Diagnostic Marker in Seminomas. BMC Urol (2010) 10:4. doi: 10.1186/1471-2490-10-4
57. Li RM, Nai MM, Duan SJ, Li SX, Yin BN, An F, et al. Down-Expression of GOLM1 Enhances the Chemo-Sensitivity of Cervical Cancer to Methotrexate Through Modulation of the MMP13/EMT Axis. Am J Cancer Res (2018) 8:964–80.
58. Iftikhar R, Kladney RD, Havlioglu N, Schmitt-Graff A, Gusmirovic I, Solomon H, et al. Disease- and Cell-Specific Expression of GP73 in Human Liver Disease. Am J Gastroenterol (2004) 99:1087–95. doi: 10.1111/j.1572-0241.2004.30572.x
59. Wei H, Li B, Zhang R, Hao X, Huang Y, Qiao Y, et al. Serum GP73, a Marker for Evaluating Progression in Patients With Chronic HBV Infections. PloS One (2013) 8:e53862. doi: 10.1371/journal.pone.0053862
60. Wei H, Zhang J, Li H, Ren H, Hao X, Huang Y. GP73, a New Marker for Diagnosing HBV-ACLF in Population With Chronic HBV Infections. Diagn Micr Infect Dis (2014) 79:19–24. doi: 10.1016/j.diagmicrobio.2014.01.008
61. Qiao Y, Chen J, Li X, Wei H, Xiao F, Chang L, et al. Serum Gp73 is Also a Biomarker for Diagnosing Cirrhosis in Population With Chronic HBV Infection. Clin Biochem (2014) 47:216–22. doi: 10.1016/j.clinbiochem.2014.08.010
62. Takeuchi O, Akira S. Pattern Recognition Receptors and Inflammation. Cell (2010) 140:805–20. doi: 10.1016/j.cell.2010.01.022
63. Zhang X, Zhu C, Wang T, Jiang H, Ren Y, Zhang Q, et al. GP73 Represses Host Innate Immune Response to Promote Virus Replication by Facilitating MAVS and TRAF6 Degradation. PloS Pathog (2017) 13:e1006321. doi: 10.1371/journal.ppat.1006321
64. Jing JS, Ye W, Jiang YK, Ma J, Zhu MQ, Ma JM, et al. The Value of GPC3 and GP73 in Clinical Diagnosis of Hepatocellular Carcinoma. Clin Lab (2017) 63:1903–9. doi: 10.7754/Clin.Lab.2017.170712
65. Liu T, Yao M, Liu S, Wang L, Wang T, Hou J, et al. Serum Golgi Protein 73 is Not a Suitable Diagnostic Marker for Hepatocellular Carcinoma. Oncotarget (2017) 8(10):16498–506. doi: 10.18632/oncotarget.14954
66. Liang H, Ai-Jun J, Ji-Zong Z, Jian-Bo H, Liang Z, Yong-Xiang Y, et al. Clinicopathological Significance of miR-27b Targeting Golgi Protein 73 in Patients With Hepatocellular Carcinoma. Anti-Cancer Drug (2019) 30:186–94. doi: 10.1097/CAD.0000000000000711
67. Goto Y, Kojima S, Nishikawa R, Enokida H, Chiyomaru T, Kinoshita T, et al. The microRNA-23b/27b/24-1 Cluster is a Disease Progression Marker and Tumor Suppressor in Prostate Cancer. Oncotarget (2014) 5:7748–59. doi: 10.18632/oncotarget.2294
68. Wang B, Sun X, Huang K, Zhou L, Qiu Z. Long non-Coding RNA TP73-AS1 Promotes Pancreatic Cancer Growth and Metastasis Through miRNA-128-3p/GOLM1 Axis. World J Gastroenterol (2021) 27:1993–2014. doi: 10.3748/wjg.v27.i17.1993
69. Zhou M, Chen X, Wu J, He X, Ren R. MicroRNA−143 Regulates Cell Migration and Invasion by Targeting GOLM1 in Cervical Cancer. Oncol Lett (2018) 16:6393–400. doi: 10.3892/ol.2018.9441
70. Kojima S, Enokida H, Yoshino H, Itesako T, Chiyomaru T, Kinoshita T, et al. The Tumor-Suppressive microRNA-143/145 Cluster Inhibits Cell Migration and Invasion by Targeting GOLM1 in Prostate Cancer. J Hum Genet (2014) 59:78–87. doi: 10.1038/jhg.2013.121
71. Zeinali T, Mansoori B, Mohammadi A, Baradaran B. Regulatory Mechanisms of miR-145 Expression and the Importance of its Function in Cancer Metastasis. BioMed Pharmacother (2019) 109:195–207. doi: 10.1016/j.biopha.2018.10.037
72. Yang L, Luo P, Song Q, Fei X. DNMT1/miR-200a/GOLM1 Signaling Pathway Regulates Lung Adenocarcinoma Cells Proliferation. BioMed Pharmacother (2018) 99:839–47. doi: 10.1016/j.biopha.2018.01.161
73. Li Z, Li Y, Wang X, Liang Y, Luo D, Han D, et al. LINC01977 Promotes Breast Cancer Progression and Chemoresistance to Doxorubicin by Targeting miR-212-3p/GOLM1 Axis. Front Oncol (2021) 11:657094. doi: 10.3389/fonc.2021.657094
74. Zhang S, Ge W, Zou G, Yu L, Zhu Y, Li Q, et al. MiR-382 Targets GOLM1 to Inhibit Metastasis of Hepatocellular Carcinoma and its Down-Regulation Predicts a Poor Survival. Am J Cancer Res (2018) 8:120–31.
75. Ma R, Zhang BW, Zhang ZB, Deng QJ. LncRNA MALAT1 Knockdown Inhibits Cell Migration and Invasion by Suppressing Autophagy Through miR-384/GOLM1 Axis in Glioma. Eur Rev Med Pharmacol Sci (2020) 24:2601–15. doi: 10.26355/eurrev_202003_20529
76. Zhao J, Xu T, Wang F, Cai W, Chen L. miR-493-5p Suppresses Hepatocellular Carcinoma Cell Proliferation Through Targeting GP73. BioMed Pharmacother (2017) 90:744–51. doi: 10.1016/j.biopha.2017.04.029
77. Zhang XW, Li Q, Xu Z, Dou J. STAT1-Induced Regulation of lncRNA ZFPM2-AS1 Predicts Poor Prognosis and Contributes to Hepatocellular Carcinoma Progression via the miR-653/GOLM1 Axis. Cell Death Dis (2021) 12:31. doi: 10.1038/s41419-020-03300-4
78. Chen J, Liu X, Ke K, Zou J, Gao Z, Habuchi T, et al. LINC00992 Contributes to the Oncogenic Phenotypes in Prostate Cancer via Targeting miR-3935 and Augmenting GOLM1 Expression. BMC Cancer (2020) 20:749. doi: 10.1186/s12885-020-07141-4
79. Wang F, Long Q, Gong Y, Hu L, Zhang H, Oettgen P, et al. Epithelium-Specific ETS (ESE)-1 Upregulated GP73 Expression in Hepatocellular Carcinoma Cells. Cell Biosci (2014) 4:76. doi: 10.1186/2045-3701-4-76
80. Kladney RD, Cui X, Bulla GA, Brunt EM, Fimmel CJ. Expression of GP73, a Resident Golgi Membrane Protein, in Viral and Nonviral Liver Disease. Hepatology (2002) 35:1431–40. doi: 10.1053/jhep.2002.32525
81. Weiskirchen R, Tacke F. Combining GP73 With Liver Stiffness Measurements: A Proof-of-Concept for non-Invasive Fibrosis Assessment in Antiviral-Naïve HBV Patients. Liver Int (2017) 37:1605–7. doi: 10.1111/liv.13563
82. Cao Z, Li Z, Wang H, Liu Y, Xu Y, Mo R, et al. Algorithm of Golgi Protein 73 and Liver Stiffness Accurately Diagnoses Significant Fibrosis in Chronic HBV Infection. Liver Int (2017) 37:1612–21. doi: 10.1111/liv.13536
83. Hu L, Yao W, Wang F, Rong X, Peng T. GP73 Is Upregulated by Hepatitis C Virus (HCV) Infection and Enhances HCV Secretion. PloS One (2014) 9:e90553. doi: 10.1371/journal.pone.0090553
84. Kladney RD, Tollefson AE, Wold WS, Fimmel CJ. Upregulation of the Golgi Protein GP73 by Adenovirus Infection Requires the E1A CtBP Interaction Domain. Virology (2002) 301:236–46. doi: 10.1006/viro.2002.1523
85. Li Y, Oosting M, Deelen P, Ricaño-Ponce I, Smeekens S, Jaeger M, et al. Inter-Individual Variability and Genetic Influences on Cytokine Responses to Bacteria and Fungi. Nat Med (2016) 22:952–60. doi: 10.1038/nm.4139
86. Mehta A, Baltimore D. MicroRNAs as Regulatory Elements in Immune System Logic. Nat Rev Immunol (2016) 16:279–94. doi: 10.1038/nri.2016.40
87. Lin S, Gregory RI. MicroRNA Biogenesis Pathways in Cancer. Nat Rev Cancer (2015) 15:321–33. doi: 10.1038/nrc3932
88. Ha M, Kim VN. Regulation of microRNA Biogenesis. Nat Rev Mol Cell Bio (2014) 15:509–24. doi: 10.1038/nrm3838
89. Lee SWL, Adriani G, Kamm RD, Gillrie MR. Models for Monocytic Cells in the Tumor Microenvironment. Adv Exp Med Biol (2020) 1224:87–115. doi: 10.1007/978-3-030-35723-8_7
90. Wang M, Xu Y, Liu J, Ye J, Yuan W, Jiang H, et al. Recent Insights Into the Biological Functions of Sestrins in Health and Disease. Cell Physiol Biochem (2018) 43:1731–41. doi: 10.1159/000484060
91. Moscat J, Diaz-Meco MT. Feedback on Fat: P62-Mtorc1-Autophagy Connections. Cell (2011) 147:724–7. doi: 10.1016/j.cell.2011.10.021
92. Zoncu R, Efeyan A, Sabatini DM. mTOR: From Growth Signal Integration to Cancer, Diabetes and Ageing. Nat Rev Mol Cell Bio (2011) 12:21–35. doi: 10.1038/nrm3025
93. Ip CK, Wong AS. Exploiting P70 S6 Kinase as a Target for Ovarian Cancer. Expert Opin Ther Tar (2012) 16:619–30. doi: 10.1517/14728222.2012.684680
94. Fukuda A, Wang SC, Morris JP, Folias AE, Liou A, Kim GE, et al. Stat3 and MMP7 Contribute to Pancreatic Ductal Adenocarcinoma Initiation and Progression. Cancer Cell (2011) 19:441–55. doi: 10.1016/j.ccr.2011.03.002
96. Zöller M. CD44: Can a Cancer-Initiating Cell Profit From an Abundantly Expressed Molecule? Nat Rev Cancer (2011) 11:254–67. doi: 10.1038/nrc3023
97. Dang Y, Yu J, Zhao S, Jin L, Cao X, Wang Q. GOLM1 Drives Colorectal Cancer Metastasis by Regulating Myeloid-Derived Suppressor Cells. J Cancer (2021) 12:7158–66. doi: 10.7150/jca.61567
98. Yan J, Zhou B, Guo L, Chen Z, Zhang B, Liu S, et al. GOLM1 Upregulates Expression of PD-L1 Through EGFR/STAT3 Pathway in Hepatocellular Carcinoma. Am J Cancer Res (2020) 10:3705–20.
99. Bao YX, Cao Q, Yang Y, Mao R, Xiao L, Zhang H, et al. Expression and Prognostic Significance of Golgiglycoprotein73 (GP73) With Epithelial-Mesenchymal Transition (EMT) Related Molecules in Hepatocellular Carcinoma (HCC). Diagn Pathol (2013) 8:197. doi: 10.1186/1746-1596-8-197
100. Brabletz T. EMT and MET in Metastasis: Where Are the Cancer Stem Cells? Cancer Cell (2012) 22:699–701. doi: 10.1016/j.ccr.2012.11.009
101. Jiang K, Li W, Zhang Q, Yan G, Guo K, Zhang S, et al. GP73 N-Glycosylation at Asn144 Reduces Hepatocellular Carcinoma Cell Motility and Invasiveness. Oncotarget (2016) 7:23530–41. doi: 10.18632/oncotarget.8120
102. Liu Y, Zhang X, Sun T, Jiang J, Li Y, Chen M, et al. Knockdown of Golgi Phosphoprotein 2 Inhibits Hepatocellular Carcinoma Cell Proliferation and Motility. Oncotarget (2016) 7:21404–15. doi: 10.18632/oncotarget.7271
103. Yang Y, Liu Q, Zhang H, Zhao H, Mao R, Li Z, et al. Silencing of GP73 Inhibits Invasion and Metastasis via Suppression of Epithelial-Mesenchymal Transition in Hepatocellular Carcinoma. Oncol Rep (2017) 37:1182–8. doi: 10.3892/or.2017.5351
104. Jin D, Tao J, Li D, Wang Y, Li L, Hu Z, et al. Golgi Protein 73 Activation of MMP-13 Promotes Hepatocellular Carcinoma Cell Invasion. Oncotarget (2015) 6:33523–33. doi: 10.18632/oncotarget.5590
105. Liu Y, Wang J, Yang R, Cheng Y, Zhou Y, Li H, et al. GP73-Mediated Secretion of AFP and GP73 Promotes Proliferation and Metastasis of Hepatocellular Carcinoma Cells. Oncogenesis (2021) 10:69. doi: 10.1038/s41389-021-00358-3
106. Byrne A, Bekiaris S, Duggan G, Prichard D, Kirca M, Finn S, et al. Golgi Phosphoprotein 2 (GOLPH2) is a Novel Bile Acid-Responsive Modulator of Oesophageal Cell Migration and Invasion. Brit J Cancer (2015) 113:1332–42. doi: 10.1038/bjc.2015.350
107. Gai X, Tang B, Liu F, Wu Y, Wang F, Jing Y, et al. mTOR/miR-145-Regulated Exosomal GOLM1 Promotes Hepatocellular Carcinoma Through Augmented GSK-3beta/MMPs. J Genet Genomics (2019) 46:235–45. doi: 10.1016/j.jgg.2019.03.013
108. van Roy F. Beyond E-Cadherin: Roles of Other Cadherin Superfamily Members in Cancer. Nat Rev Cancer (2014) 14:121–34. doi: 10.1038/nrc3647
109. Ma H, Groth RD, Cohen SM, Emery JF, Li B, Hoedt E, et al. γcamkii Shuttles Ca2+/CaM to the Nucleus to Trigger CREB Phosphorylation and Gene Expression. Cell (2014) 159:281–94. doi: 10.1016/j.cell.2014.09.019
110. Altarejos JY, Montminy M. CREB and the CRTC Co-Activators: Sensors for Hormonal and Metabolic Signals. Nat Rev Mol Cell Bio (2011) 12:141–51. doi: 10.1038/nrm3072
111. Avraham R, Yarden Y. Feedback Regulation of EGFR Signalling: Decision Making by Early and Delayed Loops. Nat Rev Mol Cell Bio (2011) 12:104–17. doi: 10.1038/nrm3048
112. Pao W, Chmielecki J. Rational, Biologically Based Treatment of EGFR-Mutant non-Small-Cell Lung Cancer. Nat Rev Cancer (2010) 10:760–74. doi: 10.1038/nrc2947
113. Ullrich O, Reinsch S, Urbe S, Zerial M, Parton RG. Rab11 Regulates Recycling Through the Pericentriolar Recycling Endosome. J Cell Biol (1996) 135:913–24. doi: 10.1083/jcb.135.4.913
114. Harding HP, Zhang Y, Ron D. Protein Translation and Folding Are Coupled by an Endoplasmic-Reticulum-Resident Kinase. Nature (1999) 397:271–4. doi: 10.1038/16729
115. Wei C, Yang X, Liu N, Geng J, Tai Y, Sun Z, et al. Tumor Microenvironment Regulation by the Endoplasmic Reticulum Stress Transmission Mediator Golgi Protein 73 in Mice. Hepatology (2019) 70:851–70. doi: 10.1002/hep.30549
116. Ke MY, Xu T, Fang Y, Ye YP, Li ZJ, Ren FG, et al. Liver Fibrosis Promotes Immune Escape in Hepatocellular Carcinoma via GOLM1-Mediated PD-L1 Upregulation. Cancer Lett (2021) 513:14–25. doi: 10.21203/rs.3.rs-117712/v1
Keywords: GP73, cancer biomarker, epithelial mesenchymal transition, cancer metastasis, protein trafficking
Citation: Liu Y, Hu X, Liu S, Zhou S, Chen Z and Jin H (2021) Golgi Phosphoprotein 73: The Driver of Epithelial-Mesenchymal Transition in Cancer. Front. Oncol. 11:783860. doi: 10.3389/fonc.2021.783860
Received: 28 September 2021; Accepted: 15 November 2021;
Published: 07 December 2021.
Edited by:
Gelina Kopeina, Lomonosov Moscow State University, RussiaReviewed by:
Chi-Wai Cheng, The University of Hong Kong, Hong Kong SAR, ChinaPranshu Sahgal, Dana-Farber Cancer Institute, United States
Copyright © 2021 Liu, Hu, Liu, Zhou, Chen and Jin. This is an open-access article distributed under the terms of the Creative Commons Attribution License (CC BY). The use, distribution or reproduction in other forums is permitted, provided the original author(s) and the copyright owner(s) are credited and that the original publication in this journal is cited, in accordance with accepted academic practice. No use, distribution or reproduction is permitted which does not comply with these terms.
*Correspondence: Hongchuan Jin, amluaGNAemp1LmVkdS5jbg==; Zhi Chen, Y2hlbnpoaUB6anUuZWR1LmNu
†These authors have contributed equally to this work