- 1Institute of Modern Physics, Chinese Academy of Sciences, Lanzhou, China
- 2The First School of Clinical Medicine, Lanzhou University, Lanzhou, China
- 3Department of Postgraduate, University of Chinese Academy of Sciences, Beijing, China
- 4Heavy Ion Therapy Center, Lanzhou Heavy Ions Hospital, Lanzhou, China
- 5Evidence-Based Medicine Center, School of Basic Medical Sciences, Lanzhou University, Lanzhou, China
Purpose: Given the higher precision accompanied by optimized sparing of normal tissue, charged particle therapy was thought of as a promising treatment for pancreatic cancer. However, systematic preclinical studies were scarce. We aimed to investigate the radiobiological effects of charged particle irradiation on pancreatic cancer cell lines.
Methods: A systematic literature search was performed in EMBASE (OVID), Medline (OVID), and Web of Science databases. Included studies were in vitro English publications that reported the radiobiological effects of charged particle irradiation on pancreatic cancer cells.
Results: Thirteen carbon ion irradiation and seven proton irradiation in vitro studies were included finally. Relative biological effectiveness (RBE) values of carbon ion irradiation and proton irradiation in different human pancreatic cancer cell lines ranged from 1.29 to 4.5, and 0.6 to 2.1, respectively. The mean of the surviving fraction of 2 Gy (SF2) of carbon ion, proton, and photon irradiation was 0.18 ± 0.11, 0.48 ± 0.11, and 0.57 ± 0.13, respectively. Carbon ion irradiation induced more G2/M arrest and a longer-lasting expression of γH2AX than photon irradiation. Combination therapies enhanced the therapeutic effects of pancreatic cell lines with a mean standard enhancement ratio (SER) of 1.66 ± 0.63 for carbon ion irradiation, 1.55 ± 0.27 for proton irradiation, and 1.52 ± 0.30 for photon irradiation. Carbon ion irradiation was more effective in suppressing the migration and invasion than photon irradiation, except for the PANC-1 cells.
Conclusions: Current in vitro evidence demonstrates that, compared with photon irradiation, carbon ion irradiation offers superior radiobiological effects in the treatment of pancreatic cancer. Mechanistically, high-LET irradiation may induce complex DNA damage and ultimately promote genomic instability and cell death. Both carbon ion irradiation and proton irradiation confer similar sensitization effects in comparison with photon irradiation when combined with chemotherapy or targeted therapy.
Introduction
Pancreatic cancer is one of the most aggressive cancers—of which pancreatic ductal adenocarcinoma (PDAC) is the most frequent type, accounting for 85% of all cases—and is associated with the highest mortality rate (1). The efficacy of available treatments is limited; only approximately 15–20% of all patients can be treated with an R0 resection at the time of diagnosis (2). However, even after resection, a high proportion of patients die from local recurrence and/or distant metastasis (3). New therapies or therapeutic combinations are therefore required to improve outcomes from this cancer type, which are currently very poor. Radiation therapy combined with chemotherapy is an important treatment modality; however, pancreatic cancer is extremely hypoxic, resulting in epithelial to mesenchymal transition and resistance to low linear energy transfer (LET) radiation (4). It has been reported that the 1-year local control rates associated with conventional chemoradiotherapy (CRT) in people with PDAC is just 40–60% (5). Moreover, in conventional photon irradiation, the dose cannot be escalated owing to the risk of toxicity in the surrounding radiosensitive organs, such as the small bowel, liver, and kidneys.
The prevailing use of highly sophisticated, photon-based external beam radiation techniques including stereotactic body radiation therapy (SBRT), intensity-modulated radiotherapy (IMRT) offers more conformal dose distributions and have shed light to people with pancreatic cancer. A meta-analysis estimated that in locally advanced pancreatic cancer (LAPC), the 2-year overall survival (OS) is 26.9% with SBRT and just 14.7% for CRT (6).Charged particle (carbon ion and proton) represents an emerging technological advance in oncology and yielded quite encouraging outcomes. There are articles demonstrated the 2-year OS of LAPC for carbon ion radiotherapy (CIRT) with concurrent gemcitabine (GEM) was approximately 50%, and the 1-year OS of proton radiotherapy (PRT) concurrent chemotherapy was approximately 76%. In addition, CIRT and PRT can significantly reduce toxicity in those who receive these therapies (7–10).
Compared with conventional photon radiation, proton offers the potential physical advantage of improved dose localization offered by a spread-out Bragg peak (SOBP). It can deliver equivalent doses to targets as photons would while sparing integral dose to organs at risk (OARs), which could potentially reduce toxicity (11). Compared with proton, carbon ion offers comparable physical characteristics (12), but has substantially different biological properties. Carbon ion is associated with an enhanced relative biological effectiveness (RBE) due to higher LET, which theoretically induce more direct DNA damage, and double-strand breaks (DSBs). Carbon ion irradiation is also more effective against hypoxic radioresistant tumors, due to its low oxygen enhancement ratio. These characteristics make charged particle therapy a very promising cancer treatment option, however the exact radiobiological responses to charged particle irradiation have not been fully elucidated. It is unclear what modifications charged particle irradiation induces to the DNA and to signal transduction events. Moreover, the effects of combining carbon ion/proton irradiation and drugs with various mechanisms of action may differ from those of photon irradiation.
Several in vitro publications have explored the radiobiological effects of charged particle irradiation for pancreatic cancer. However, these studies differed in the cellular origins, charged particle type, radiation dose, dose rate, and therapeutic combinations (13–15). Therefore, further investigation is needed to combine and assess the current knowledge of this topic offered by in vitro studies. This study is a systematic review (SR) of published in vitro studies on the topic of carbon ion/proton irradiation for pancreatic cancer. It includes a comprehensive analysis of the cellular and molecular effects of charged particle irradiation on pancreatic cancer cell lines to gain insights into the mechanisms of particle therapy alone or as part of combination therapies used to treat pancreatic cancer.
Materials and Methods
This SR was developed according to the Preferred Reporting Items for Systematic Reviews and Meta-Analyses (PRISMA) guidelines (16).
Search Strategy
We used database-specific subject headings and free-text terms describing the populations or interventions to search Embase (OVID), Medline (OVID), and Web of Science databases (dates of inception to August 27, 2021). In addition, these terms were also used to access any unpublished material using Google search. Finally, the references of included studies were checked manually to identify any initially omitted publications. The full search strategy is described in the Supplementary Materials.
Study Selection
After independently screening the titles and abstracts, two trained reviewers intensively read the full text to determine the final inclusion. Disagreements were discussed with the participation of a third reviewer.
The inclusion criteria for this research were:
1. In vitro studies of pancreatic cancer cell lines irradiated by carbon ion/proton.
2. Reported at least one of the following outcomes:
2.1. Cell clonogenic survival.
2.2. DNA damage response (DDR): cell cycle checkpoints, DNA repair, and apoptosis.
2.3. Migration or invasion.
2.4. The standard enhancement ratio (SER) evaluating the therapeutic effects of combination therapy. For studies that failed to report SER, we extracted data from published plots using Web Plot Digitizer and then determined the SER by calculating the ratio of doses in treated and control groups for a given isoeffect (surviving fraction (SF)=0.1) (17).
3. Articles published in English.
The exclusion criteria were:
1. Artificially modified cells lines.
2. No corresponding outcome was reported.
3. Clinical and animal studies, case reports, reviews, commentary, expert opinion, conference abstracts, correspondence.
4. Pilot studies and research projects.
5. Full-text articles were not available.
Data Extraction
Two trained reviewers independently extracted data using a pre-established data extraction form. The following details were collected: name of the first author and the publication year, country, or region, type of particle irradiation, cell type (origin), the carbon ion/proton irradiation schedule (initial energy, average LET, SOBP, single doses, dose rate, and dose group), combination therapy, type of photon radiation. The extracted data were confirmed by a third reviewer.
Assessment of Risk of Bias
For in vitro studies, no standard risk of bias assessment instrument exists now, so we produced these criteria ourselves, detailed in the supplementary materials (Table S1). These criteria evaluated the risk of bias induced by selection, performance, detection, attrition, cell-related, and other bias. The risk of bias was categorized into “Low”, “Moderate”, or “High”. When a study lacked sufficient details to evaluate, the risk of bias was categorized as “Risk Unknown”. In duplicate, two reviewers assessed the risk of bias. Disagreements were solved by the participation of a third reviewer.
Statistical Analysis
In general, we used descriptive statistics to summarize baseline variables. The continuous data were presented as mean with standard deviation (SD) or median with interquartile ranges (IQR). All analyses were performed using R 4.0.3. A value of P < 0.05 was thought statistically significant.
Results
Search Results
The electronic search resulted in 1029 unique citations. After reference and full-text screening, a total of 20, including 13 on carbon ion irradiation and seven on proton irradiation, met the eligibility criteria. The screening and selection processes are presented in Figure 1.
Study Characteristics
The characteristics of included (13–15, 18–34) studies are summarized in Table 1. Briefly, 13 carbon ion irradiation and seven proton irradiation studies were included in our final SR; these studies were published between 2004 and 2021.They were conducted in 7 different countries: Japan, Germany, Belgium, Italy, Korea, the United States of America, and Lithuania. Of these studies, almost all used human pancreatic cancer cell lines, such as AsPC-1, BxPc-3, MIA PaCa-2, PANC-1, and PK45, only one (18) used transgenic mice cell lines (PDA30364/OVA). The studies varied in the initial energy, averaged LET, SOBP, radiation doses, and dose rates used. Two studies (14, 26) explored the effects of carbon ion irradiation on human pancreatic cancer stem-like cells (CSCs), using CD44+CD24+ESA+ and CD133+ as markers for their identification (35, 36). Carbon ion/proton irradiation combined with GEM was reported in three studies (20, 26, 29), poly (ADP-ribose) polymerase (PARP) inhibitor (PARPi) in three studies (24, 31, 33), B02 (a RAD51 inhibitor) in one study (33) and camptothecin (CPT) in another study (27). In addition, one study (19) combined carbon ion irradiation, magnetic nanoparticles (MNPs), and hyperthermia (Hyp) as a new treatment combination for pancreatic cancer. In total, 13 control groups were treated using X-rays, one with γ-rays, and the remaining six control groups were not irradiated. Eleven studies reported RBE values. The study by Hirai and colleagues (24) did not report the RBE values, but they calculated the isoeffective doses of γ-ray, LET 13 carbon ion, and LET 70 carbon ion irradiations that resulted 10% cell survival for their follow-up analyses.
Risk of Bias
The outcomes of the risk of bias evaluation are shown in Figure 2. Among the 20 in vitro studies included in our SR, eight studies (13, 15, 19, 20, 22, 23, 27, 30) provided adequate information on irradiation. Eleven of the studies failed to describe cell counting methods. Seven studies (14, 15, 19, 23, 28, 29, 34) did not report whether the experiments were repeated, resulting in attrition bias. None of the publications stated whether the selection of treatment was blinded, and only one paper (21) reported blinding the outcomes assessors to minimize detection bias. All papers except one (28) described the implementation process. Four publications (19, 25, 28, 33) only partly described how results were measured; the remaining 16 described these methods in detail. One study (23) did not mention the conditions in which cells were cultured, and four studies (18, 31, 33, 34) partly reported the cell origin and cell type. Only one study (25) did not clear whether there was industry sponsoring; the rest were reported to have no connections with or employment at a company.
RBE Values
RBE is defined as the ratio of the amount of dose from test radiation required to generate the same biological endpoint (usually cell-killing) relative to reference radiation (usually 250 kVp X-rays or Co-60 γ-rays) (37).
Four studies (15, 18) (20, 34) used a linear-quadratic model to determine RBE values; the remaining 16 did not declare the model used. Nine studies using six different human pancreatic cancer cell lines in total reported RBE values for carbon ion irradiation, ranging from 1.29 to 4.5 (Figure 3). Two of these studies (14, 26) reported RBE values were higher for CSCs than non-CSCs for the same cell line. Matsui and colleagues (25) used three pancreatic cancer cell lines each irradiated with three different LETs and demonstrated that RBE values increased with increasing LET. Schlaich and colleagues (27) calculated RBE values for carbon ion irradiation alone (2.4 ± 0.4) and in combination with CPT (2.2 ± 0.2). Two studies (15, 28) reported the RBE values for proton irradiation, which varied from 0.6 to 2.1 depending on the pancreatic cell line used. Details are shown in Table 2.
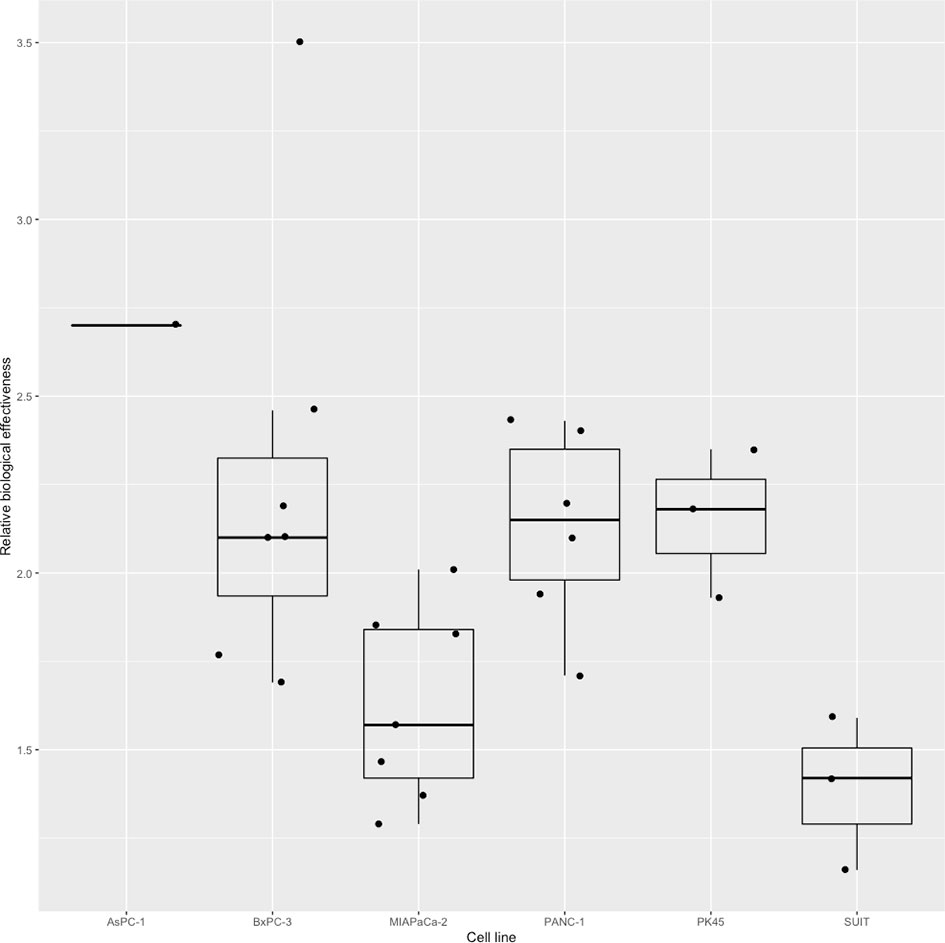
Figure 3 Boxplot representing the RBE value of carbon ion irradiation for the six human pancreatic cell lines.
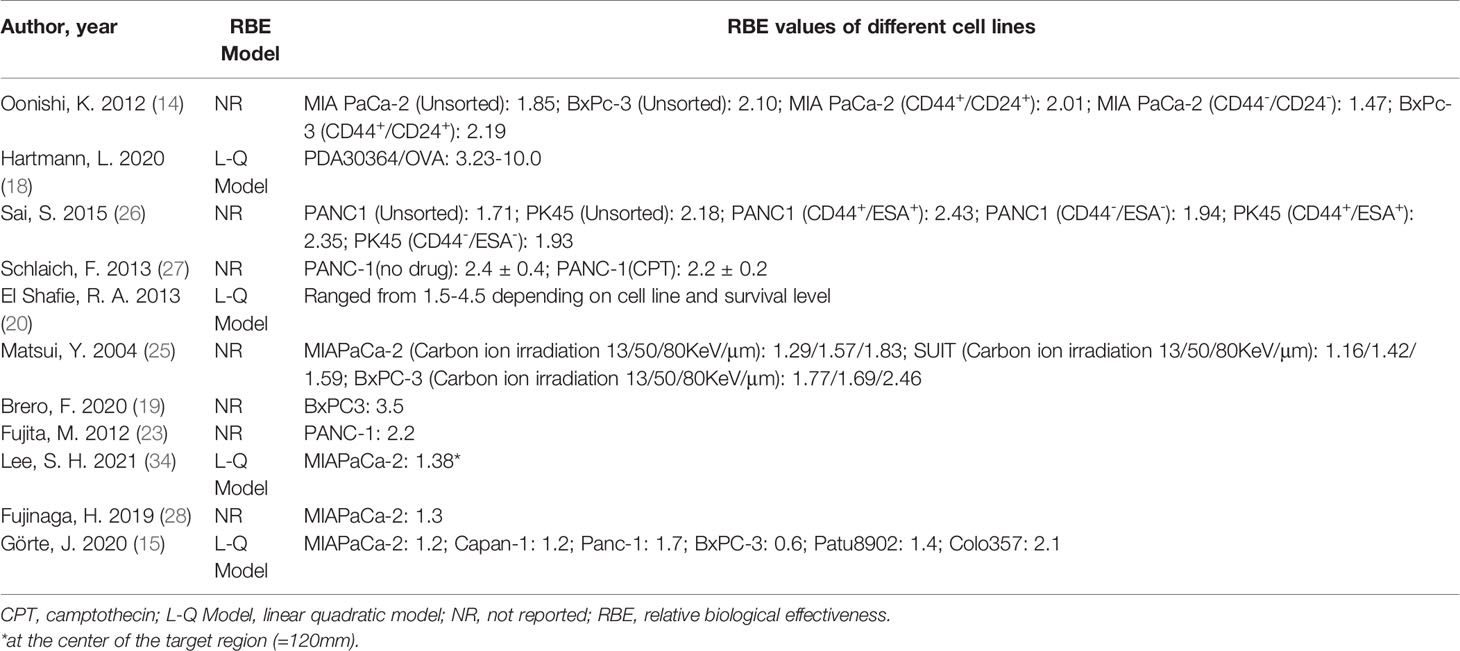
Table 2 RBE values comparing carbon ion/proton to photon irradiation effectiveness in pancreatic cancer cell lines.
Clonogenic Survival
We calculated the SF for carbon ion, proton, and photon irradiation from radiation survival curves. The mean SF of 2 Gy (SF2) of carbon ion, proton, and photon irradiation was 0.18 ± 0.11, 0.48 ± 0.11, and 0.57 ± 0.13, respectively. The SF values associated with different radiation doses are shown in Table 3. Three studies (18, 20, 27) demonstrated that carbon ion irradiation had an enhanced efficacy in the suppression of clonogenic survival compared with standard photon irradiation in human pancreatic cell lines and pancreatic adenocarcinoma cell lines from transgenic mice. The presence of Olaparib (Ola, a PARP inhibitor), MNPs, or Hyp can enhance this cytotoxic effect. The SF for cancer stem cell-like CD44+/CD24+ or CD44+/ESA+ cells was found to be significantly higher than non-cancer stem-like CD44–/CD24– or CD44–/ESA– cells after treatment with either carbon ion or photon irradiation. The number of tumor spheroids was significantly lower in carbon ion-irradiated CSCs than in those that were irradiated with X-rays (14, 26). Matsui and colleagues (25) concluded that the survival rates of pancreatic cell lines after carbon ion irradiation were correlated with LET level and RBE values. Görte and colleagues (15) revealed that proton irradiation is associated with a greater reduction in PDAC tumoroid growth than X-ray irradiation.
The Effects of DDR
Overall, ten studies investigated the DDR effects of carbon ion/proton irradiation on pancreatic cancer cells. Two of these (18, 25) reported that carbon ion irradiation induced more G2/M arrest compared with photon irradiation and that the intensity of G2/M arrest was stronger with an increased LET level. Combined GEM and carbon ion irradiation increased the expression of senescence-related genes such as P21, P16, and P27 compared with carbon ion irradiation alone. The presence of Ola enhanced G2/M phase arrest and reduced the level of histone H3 phosphorylation induced by photon or carbon ion/proton irradiation. One study (29) confirmed proton irradiation can induce significant cell cycle arrest in a dose-dependent manner in both radiosensitive and radioresistant pancreatic cell lines.
Compared with unirradiated controls, Brero and colleagues (19) found that carbon ion irradiation alone increased the formation of γH2AX and 53BP1 foci, which were validated markers of DNA DSBs. Oonishi and colleagues (14) reported on the expression of γH2AX in human pancreatic CSCs after irradiation. The number of γH2AX foci in CD44–/CD24– cells were higher than that of CD44+/CD24+ cells after irradiation with either carbon ion or X-rays, and the number of γH2AX foci in CD44+/CD24+ cells irradiated with carbon ion persisted significantly longer compared with those irradiated with X-rays. Meanwhile, the authors discovered that carbon ion irradiation alone can also strongly increase in the size of γH2AX foci (clustered DNA damage). Sai and colleagues (26) confirmed that combined therapy with carbon ion irradiation and GEM significantly increased the expression of DNA damage and repair-related genes such as ARTEMIS, RAD51, TP53BP1, and BRAC1. Compared with unirradiated cells, the expression of γH2AX was significantly increased in Capan-1, Panc-1, and MIA PaCa-2 cells after proton irradiation. Lee and colleagues (32) demonstrated that the level of the DNA repair protein RAD51 was dose-dependently decreased in Capan-1cells, but not in Panc-1 cells. Two studies (24, 31) reported the expression of γH2AX was increased and prolonged following carbon ion/proton irradiation in the presence of Ola.
Sai and colleagues (26) reported that carbon ion irradiation combined with GEM increased the level of apoptosis in PK45 cells, and the apoptosis-related gene expressions such as Bax, cytochrome c, and Bcl2 were significantly elevated with combined carbon ion irradiation/GEM and with GEM alone. One study (25) demonstrated that carbon ion irradiation induced mitotic death, rather than apoptotic death, in BxPC-3, MIAPaCa-2, and SUIT2 cells. The effects of proton irradiation on apoptosis varied according to cell type: in Capan-1 cells, proton irradiation increased the expression of cleaved PARP, which is known as a marker for apoptotic cell death (32). Proton irradiation alone increased the levels of survivin in MIA PaCa-2 cells with little apoptosis. However, combined proton and GEM therapy induced robust apoptosis with a concomitant reduction in survivin and XIAP (29). In radiation-resistant PANC-1cells, two studies (29, 32) showed that proton irradiation increased survivin levels with little apoptosis, even in combination with GEM. Details are presented in Table 4.
Migration and Invasion Ability
There were five publications (13, 21–23, 26) involved migration, invasion, or the expression of related genes (Table 5). Fujita and colleagues (13, 22, 23) conducted a series of studies and demonstrated carbon ion irradiation (2Gy) could repress the migration of AsPC, BxPC-3, and MIAPaCa-2 cells, diminish MIAPaCa-2 cell invasiveness via prevented the activity of Rac 1 and RhoA, and reduce the invasiveness of AsPC, BxPC-3 via inhibited the activity of Rac 1 through Ub-mediated proteasomal degradation. In contrast, 2Gy carbon ion irradiation promoted the invasion of PANC-1 cells by activating of plasmin and urokinase-type plasminogen activator, and nitric oxide also played an important role in this process via activation of the PI3K-AKT and RhoA pathways. Facoetti and colleagues (21) reported the migration of AsPC-1 cells is regulated by components released by normal fibroblasts and tumor cells through morphological analysis. Sai and colleagues (26) demonstrated carbon ion or photon irradiation alone and/or in combination with GEM in PK 45 cells could enhance the level of tumor invasion-related genes like MMP-2, MMP-9, E-cadherin, and β-catenin.
SER Evaluating the Therapeutic Effect of Combination Therapy
Six studies reported on the therapeutic effects of combination therapy in five different cell lines exposed to carbon ion/proton irradiation (Table 6). SER values depended on the type of irradiation, ranging from 1.02–2.81 for carbon ion, 1.30–1.98 for proton, and 1.07–2.10 for photon irradiation, respectively. Two publications (24, 31) reported the SER associated with low versus high LET radiation levels, confirming that the high LET levels had an enhanced effect. Brero and colleagues (19) demonstrated that combination of MNPs and Hyp substantially enhanced the effects of irradiation, irrespective of the type of irradiation used. CPT had a slight enhancing effect on both carbon ion and photon irradiation (27). Wera and colleagues (33) revealed that the combination of Ola and B02 further sensitized KP4 cells, but not PANC-1 cells, to proton irradiation. Taken together, the pooled analysis indicated that combination therapies enhanced the therapeutic effects of pancreatic cell lines with a mean SER of 1.66 ± 0.63 for carbon ion, 1.55 ± 0.27 for proton, and 1.52 ± 0.30 for photon irradiation (Figure 4). However, no significant differences compared with photon irradiation were observed for carbon ion or proton irradiation (P > 0.05).
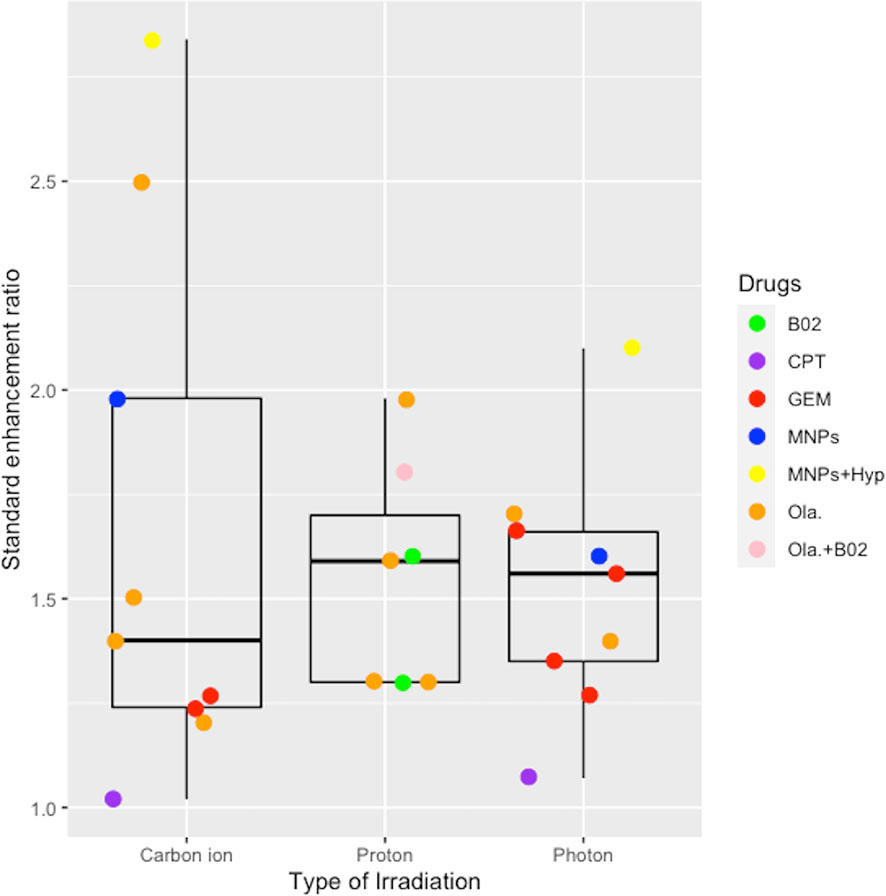
Figure 4 Boxplot representing the SER value of combined effects of chemotherapy or target therapy with the carbon ion, proton, and photon irradiation. B02, a RAD51 inhibitor; CPT, camptothecin; GEM, gemcitabine; MNPs, magnetic nanoparticles; Hyp, hyperthermia; Ola, Olaparib.
Discussion
This SR aimed to evaluate the radiobiological effects of charged particle irradiation on pancreatic cancer cell lines, including cell survival, DDR, migration, and invasion ability. In total, 20 in vitro studies, including 13 on carbon ion irradiation and seven on proton irradiation, were included. Our results revealed that carbon ion irradiation had superior radiobiological effects on pancreatic cancer cells when compared with photon irradiation. The lack of a direct comparison between proton and photon irradiation across the studies included here prevents any similar conclusions for proton irradiation. Both carbon ion irradiation and proton irradiation confer similar sensitization effects in comparison with photon irradiation when combined with chemotherapy or targeted therapy.
RBE is a complex quantity that depends on physical parameters, such as particle type and energy, LET and dose, as well as biological parameters, including tissue/cell type, cell cycle phase, oxygen level, and endpoint (38). Acceptable RBE values are generally considered to be approximately 1.1 for protons and 2.5– 3 for carbon ions (39), which is generally in line with the reported RBE values included in this SR. However, Gorte and colleagues (15) showed that the RBE value associated with proton irradiation in pancreatic cancer cell lines varied from 0.6 in BxPC-3 cells to 2.1 in Colo357 cells. The considerable uncertainty and great variability in the determination of absolute RBE values have long been recognized (40, 41). Furthermore, some hold the perspective that the RBE is a clinically relevant parameter, the clinical RBE involves a medical decision based on empirical data and clinical information. So it should be determined under in vivo growth conditions such as animal models, which enable simulation of clinical treatments, especially in late tissue vascular and fibrotic complications (42). Further research is needed to obtain accurate RBE values for carbon ion/proton irradiation, and thus to understand the mechanisms underpinning the enhanced effectiveness of high LET irradiations and apply them in a clinical setting.
Our SR revealed greater growth inhibition with carbon ion/proton irradiation compared with photon irradiation, which is attributed to the capacity to cause damage to cellular macromolecules, particularly DNA. Radiation-induced DNA damage is classified as direct (ionizing radiation interacts directly with the DNA) and indirect [mediated through reactive oxygen species (ROS)]. With increased LET, and thus increasing ionization density, the proportion of direct damage, the complexity of the DNA damage pattern, and the release of DNA fragments are also increased, and the repairability of the managed site is decreased (43, 44). This is consistent with the phenomenon observed among the studies we included in this SR, which found a strong increase of clustered DNA damage in carbon ion irradiated cells, which are difficult to repair (14, 26). Meanwhile, the less reliance on indirect DNA damage mediated by ROS makes charged particles more effective in treating pancreatic cancers, which is very hypoxic. The complexity of the DNA damage and the lower efficiency of DNA repair leads to increased effectiveness and tolerable toxicity levels with CIRT/PRT in the treatment of cancers (7, 45). The energy absorption of photons and carbon ions/protons are fundamentally different: photons lose their energy exponentially, with higher values at the physical entry point and lower values in deeper tissues (46), while carbon ions/protons deposit most of their energy at the end of the physical range of the particles and reaches the peak, forming the so-called Bragg-peak. After the Bragg-peak, the delivered dose drops rapidly with increased depth. This enables charged particles to target tumors more precisely while reducing the dose and irradiated portion of normal tissue. Compared with IMRT, both intensity-modulated carbon ion therapy and intensity-modulated proton therapy can reduce the OARs integral dose substantially in paraspinal sarcomas, locally recurrent nasopharyngeal carcinoma, and other cancer types in clinical settings (47, 48).
Cell cycle arrest can be induced in the G1/0 or S phase, in which DNA repair occurs via the non-homologous end joining (NHEJ) pathway, or the G2 phase, in which repair occurs via the homologous recombination (HR) pathway (49). Previous studies have demonstrated that HR is relatively low after photon irradiation in PDAC cells (50). Our results showed that, compared with photon, carbon ion irradiation induced greater G2/M arrest and longer-lasting expression of γH2AX as well as an increase in γH2AX clusters, indicating a high level of DNA damage. These clustered DNA lesions are difficult to repair and may induce different signaling pathways compared with photon irradiation, such as DNA repair, type I interferon signaling, and cell cycle pathways (51, 52). Unrepaired or misrepaired damage can inhibit cell proliferation via checkpoints; cells may therefore stay in the G2/M phase, undergoing genomic instability or cell death by several mechanisms, including apoptosis, mitotic catastrophe, or senescence. This is consistent with the results in a study by Gerelchuluun and colleagues, who used Chinese hamster cell lines (ovary AA8 and lung fibroblast V79), which demonstrated that the primary DNA repair pathway after both proton and carbon ion radiation is NHEJ, but activity in the HR pathway is greater with carbon ion (53).
In PDAC, mutations in four genes predominate: KRAS, TP53, SMAD4, and CDKN2A, each of which is mutated in >50% of different but mostly overlapping patients (54), but a mutation in the KRAS oncogene is present in >90% of cases (55), which may lead to resistance to photon irradiation. One included study (32) demonstrated that increased P21 expression after proton irradiation is correlated with inhibition of STAT3 phosphorylation, indicating that proton irradiation is likely to induce cell cycle arrest and/or cell death via the regulation of STAT3 signaling, irrespective of radiosensitivity. Correspondingly, another study (25) demonstrated carbon ion irradiation arrested cell cycle progression in an LET-dependent manner, independent of gene mutation status. This finding is supported in other research (56, 57). We therefore hypothesize that charged particle irradiation with high-LET may induce widely and densely distributed, irreparable DNA breaks and subsequent cell death.
The mammalian Rho family of GTPases consists of 22 members. Rho functions as a molecular switch in cellular processes such as cell morphogenesis, adhesion, migration, and cell cycle progression including cytokinesis (58). Rho-associated coiled-coil forming kinase (ROCK) is a serine/threonine kinase that can phosphorylate a variety of substrates and is one of the major effectors for Rho GTPases. During migration, tumor cells display a great variety of morphological changes; the modalities of single-cell migration can be subdivided into amoeboid and mesenchymal (59). Cells in the amoeboid mode are regulated by ROCK, while cells in the mesenchymal mode depend on the proteolytic activity of matrix metalloproteinases (MMPs), which permit penetration of the extracellular matrix (ECM). As shown by the studies included in this SR, carbon ion irradiation can suppress the invasive potential of pancreatic cells via Rho/ROCK signaling, except in PANC-1 cells. However, the combination of serine protease inhibitors (SerPI) and ROCK inhibitors may suppress the invasion of PANC-1 cells. Aspc-1 cells were preponderant in mesenchymal phenotypes following carbon ion irradiation; this effect could be inhibited by MMP inhibitors (23). Studies on the mechanism underlying the effect of carbon ion/proton irradiation on invasion and migration of pancreatic cancer cells remains limited, and mainly focus on the Rho/ROCK signaling pathway, which is known to be associated with metastasis (60, 61). More in-depth research on this topic is required; moreover, carbon ion/proton irradiation combined with MMP or ROCK inhibitors may suppress invasion and migration.
All the included studies demonstrated the enhanced therapeutic effect of combined therapies. Similarly, Waissi and colleagues (51) confirmed that a combination of GEM, Ola, and proton irradiation can significantly improve local control of pancreatic cancer in vivo. A phase I trial (62) of PARPi in combination with GEM and photon radiotherapy in LAPC has shown that this regimen is safe and tolerable, with a median OS of 15 months. No clinical studies on particle therapy with PARPi have been published until now, but DNA damage induced by charged particles is more complicated than that induced by photons, and this damage is mainly repaired by base excision repair—in which PARP plays a significant and predominant role (63, 64). Thus, charged particle therapy in combination with PARPi may benefit pancreatic cancer patients, and further clinical studies should therefore be prioritized. One clinical trial (9) demonstrated that the 2-year OS in people with LAPC who received CIRT and concurrent GEM therapy was 35%; another phase I/II study (7) of PRT with concurrent GEM therapy for LAPC revealed a 1-year OS of 76.8%, this supports the theory that a combination of GEM chemotherapy and irradiation could benefit people with LAPC. The presence of MNPs and Hyp have been shown to have the strongest sensitization effect on both carbon ion and photon irradiation in this SR; similarly, Ma and colleagues (65) suggested that the combination of MNPs and Hyp has a similar effect on human nasopharyngeal and lung cancer cells, which may be related to their inhibition of DNA repair and induction of apoptosis.
As far as we know, this is the first SR of in vitro studies to evaluate the radiobiological responses of charged particle irradiation on pancreatic cancer cell lines. First, we systematically reviewed published studies on the radiobiological effects of carbon ion/proton irradiation on pancreatic cancer cell lines, including RBE, cell survival, DDR, invasion, migration, and the combined effects of chemotherapy and targeted therapy; this provided a comprehensive overview and avenues for further study. Second, we conducted a radiation-related risk of bias assessment tool for in vitro studies after reference to the Cochrane risk-of-bias tool (66), and the SYstematic Review Center for Laboratory animal Experimentation (SYRCEL) risk-of-bias tool for animal studies (67). This provides a reference for future radiation-related in vitro SRs. However, there are several limitations to this SR. First, searching only English databases may result in certain language bias. Second, the limited number of publications evaluating radiobiological responses in pancreatic cancer, and the heterogeneity of outcome assessment tools, mean that the strength of our findings may also be limited. However, we retrieved and obtained all available data, together representing the latest evidence for carbon ion/proton irradiation on pancreatic cancer in vitro; with the increasing application of carbon ion/proton irradiation worldwide, this review may provide at least a starting point to improve guidance for clinical practice. Third, the endpoints we selected are common and important indicators of the radiobiological effects of charged particle irradiation; however, other endpoints are equally important, for example the production of ROSs, which could provide insights into indirect DNA damage, or the expression of immunomodulatory molecules, which may help improve understanding of the effects of radioimmunotherapy. However, these other endpoints have been only rarely reported now; updates to this review may therefore be warranted in future.
Conclusions
Current in vitro evidence demonstrates that, compared with photon irradiation, carbon ion irradiation offers superior radiobiological effects in the treatment of pancreatic cancer. Mechanistically, high-LET irradiation may induce complex DNA damage and ultimately promote genomic instability and cell death. Carbon ion irradiation can also effectively suppress the invasion and migration of most pancreatic cancer cell lines, but the mechanism is not well understood. The lack of direct comparisons between proton and photon irradiation prevents similar conclusions from being reached for this therapeutic option. Both carbon ion irradiation and proton irradiation confer similar sensitization effects in comparison with photon irradiation when combined with chemotherapy or targeted therapy.
Data Availability Statement
The original contributions presented in the study are included in the article/Supplementary Material. Further inquiries can be directed to the corresponding author.
Author Contributions
Guarantor of the article is XW. XW conceived and designed this article. JC, ZLi, YO, and QZ conducted literature searches, selected studies, and assessed the risk of bias. HL, YW, and RL extracted data. DW, MD, and ZLiu carried out analyses, interpreted results. DW, SS, KY, and JT drafted the manuscript. The lead and corresponding author (XW) affirm that the manuscript is an honest, accurate, and transparent account of the study being reported; that no important aspects of the study have been omitted. All authors contributed to the article and approved the submitted version.
Funding
This study was supported by Science and Technology Plan Project of Chengguan District of Lanzhou (No.2020-2-2-5); Talent innovation and venture project of Lanzhou city (No. 2017-RC-23); Talent innovation and venture project of Lanzhou city (No. 2020-RC-113); Key R&D Program of Science and Technology Department of Gansu Province (No. 20YF8FA116); The authorized project of Lanzhou KejinTaiji Corporation, Ltd (No. BMP-B-02-002). The funder was not involved in the study design, collection, analysis, interpretation of data, the writing of this article or the decision to submit it for publication.
Conflict of Interest
The authors declare that the research was conducted in the absence of any commercial or financial relationships that could be construed as a potential conflict of interest.
Publisher’s Note
All claims expressed in this article are solely those of the authors and do not necessarily represent those of their affiliated organizations, or those of the publisher, the editors and the reviewers. Any product that may be evaluated in this article, or claim that may be made by its manufacturer, is not guaranteed or endorsed by the publisher.
Supplementary Material
The Supplementary Material for this article can be found online at: https://www.frontiersin.org/articles/10.3389/fonc.2021.775597/full#supplementary-material
References
1. Ryan DP, Hong TS, Bardeesy N. Pancreatic Adenocarcinoma. N Engl J Med (2014) 371(11):1039–49. doi: 10.1056/NEJMra1404198
2. Rawla P, Sunkara T, Gaduputi V. Epidemiology of Pancreatic Cancer: Global Trends, Etiology and Risk Factors. World J Oncol (2019) 10(1):10–27. doi: 10.14740/wjon1166
3. Groot VP, Rezaee N, Wu W, Cameron JL, Fishman EK, Hruban RH, et al. Patterns, Timing, and Predictors of Recurrence Following Pancreatectomy for Pancreatic Ductal Adenocarcinoma. Ann Surg (2018) 267(5):936–45. doi: 10.1097/sla.0000000000002234
4. Koong AC, Mehta VK, Le QT, Fisher GA, Terris DJ, Brown JM, et al. Pancreatic Tumors Show High Levels of Hypoxia. Int J Radiat Oncol Biol Phys (2000) 48(4):919–22. doi: 10.1016/s0360-3016(00)00803-8
5. Balaban EP, Mangu PB, Khorana AA, Shah MA, Mukherjee S, Crane CH, et al. Locally Advanced, Unresectable Pancreatic Cancer: American Society of Clinical Oncology Clinical Practice Guideline. J Clin Oncol (2016) 34(22):2654–68. doi: 10.1200/jco.2016.67.5561
6. Tchelebi LT, Lehrer EJ, Trifiletti DM, Sharma NK, Gusani NJ, Crane CH, et al. Conventionally Fractionated Radiation Therapy Versus Stereotactic Body Radiation Therapy for Locally Advanced Pancreatic Cancer (CRiSP): An International Systematic Review and Meta-Analysis. Cancer (2020) 126(10):2120–31. doi: 10.1002/cncr.32756
7. Terashima K, Demizu Y, Hashimoto N, Jin D, Mima M, Fujii O, et al. A Phase I/II Study of Gemcitabine-Concurrent Proton Radiotherapy for Locally Advanced Pancreatic Cancer Without Distant Metastasis. Radiother Oncol (2012) 103(1):25–31. doi: 10.1016/j.radonc.2011.12.029
8. Hiroshima Y, Fukumitsu N, Saito T, Numajiri H, Murofushi KN, Ohnishi K, et al. Concurrent Chemoradiotherapy Using Proton Beams for Unresectable Locally Advanced Pancreatic Cancer. Radiother Oncol (2019) 136:37–43. doi: 10.1016/j.radonc.2019.03.012
9. Shinoto M, Yamada S, Terashima K, Yasuda S, Shioyama Y, Honda H, et al. Carbon Ion Radiation Therapy With Concurrent Gemcitabine for Patients With Locally Advanced Pancreatic Cancer. Int J Radiat Oncol Biol Phys (2016) 95(1):498–504. doi: 10.1016/j.ijrobp.2015.12.362
10. Shinoto M, Terashima K, Suefuji H, Matsunobu A, Toyama S, Fukunishi K, et al. A Single Institutional Experience of Combined Carbon-Ion Radiotherapy and Chemotherapy for Unresectable Locally Advanced Pancreatic Cancer. Radiother Oncol (2018) 129(2):333–9. doi: 10.1016/j.radonc.2018.08.026
11. Schulz-Ertner D, Tsujii H. Particle Radiation Therapy Using Proton and Heavier Ion Beams. J Clin Oncol (2007) 25(8):953–64. doi: 10.1200/jco.2006.09.7816
12. Wang X, Chen X, Li G, Han X, Gao T, Liu W, et al. Application of Carbon Ion and Its Sensitizing Agent in Cancer Therapy: A Systematic Review. Front Oncol (2021) 11:708724. doi: 10.3389/fonc.2021.708724
13. Fujita M, Imadome K, Shoji Y, Isozaki T, Endo S, Yamada S, et al. Carbon-Ion Irradiation Suppresses Migration and Invasiveness of Human Pancreatic Carcinoma Cells MIAPaCa-2 via Rac1 and RhoA Degradation. Int J Radiat Oncol Biol Phys (2015) 93(1):173–80. doi: 10.1016/j.ijrobp.2015.05.009
14. Oonishi K, Cui X, Hirakawa H, Fujimori A, Kamijo T, Yamada S, et al. Different Effects of Carbon Ion Beams and X-Rays on Clonogenic Survival and DNA Repair in Human Pancreatic Cancer Stem-Like Cells. Radiother Oncol (2012) 105(2):258–65. doi: 10.1016/j.radonc.2012.08.009
15. Görte J, Beyreuther E, Danen EHJ, Cordes N. Comparative Proton and Photon Irradiation Combined With Pharmacological Inhibitors in 3D Pancreatic Cancer Cultures. Cancers (Basel) (2020) 12(11):3216. doi: 10.3390/cancers12113216
16. Moher D, Liberati A, Tetzlaff J, Altman DG. Preferred Reporting Items for Systematic Reviews and Meta-Analyses: The PRISMA Statement. PLoS Med (2009) 6(7):e1000097. doi: 10.1371/journal.pmed.1000097
17. Waissi W, Paix A, Nicol A, Noël G, Burckel H. Targeting DNA Repair in Combination With Radiotherapy in Pancreatic Cancer: A Systematic Review of Preclinical Studies. Crit Rev Oncol Hematol (2020) 153:103060. doi: 10.1016/j.critrevonc.2020.103060
18. Hartmann L, Schröter P, Osen W, Baumann D, Offringa R, Moustafa M, et al. Photon Versus Carbon Ion Irradiation: Immunomodulatory Effects Exerted on Murine Tumor Cell Lines. Sci Rep (2020) 10(1):21517. doi: 10.1038/s41598-020-78577-8
19. Brero F, Albino M, Antoccia A, Arosio P, Avolio M, Berardinelli F, et al. Hadron Therapy, Magnetic Nanoparticles and Hyperthermia: A Promising Combined Tool for Pancreatic Cancer Treatment. Nanomater (Basel) (2020) 10(10):1919. doi: 10.3390/nano10101919
20. El Shafie RA, Habermehl D, Rieken S, Mairani A, Orschiedt L, Brons S, et al. In Vitro Evaluation of Photon and Raster-Scanned Carbon Ion Radiotherapy in Combination With Gemcitabine in Pancreatic Cancer Cell Lines. J Radiat Res (2013) 54 Suppl 1(Suppl 1):i113–9. doi: 10.1093/jrr/rrt052
21. Facoetti A, DIG C, Pasi F, Liberto RDI, Corbella F, Nano R, et al. Morphological Analysis of Amoeboid-Mesenchymal Transition Plasticity After Low and High LET Radiation on Migrating and Invading Pancreatic Cancer Cells. Anticancer Res (2018) 38(8):4585–91. doi: 10.21873/anticanres.12763
22. Fujita M, Imadome K, Endo S, Shoji Y, Yamada S, Imai T. Nitric Oxide Increases the Invasion of Pancreatic Cancer Cells via Activation of the PI3K-AKT and RhoA Pathways After Carbon Ion Irradiation. FEBS Lett (2014) 588(17):3240–50. doi: 10.1016/j.febslet.2014.07.006
23. Fujita M, Otsuka Y, Imadome K, Endo S, Yamada S, Imai T. Carbon-Ion Radiation Enhances Migration Ability and Invasiveness of the Pancreatic Cancer Cell, PANC-1, In Vitro. Cancer Sci (2012) 103(4):677–83. doi: 10.1111/j.1349-7006.2011.02190.x
24. Hirai T, Shirai H, Fujimori H, Okayasu R, Sasai K, Masutani M. Radiosensitization Effect of Poly(ADP-Ribose) Polymerase Inhibition in Cells Exposed to Low and High Liner Energy Transfer Radiation. Cancer Sci (2012) 103(6):1045–50. doi: 10.1111/j.1349-7006.2012.02268.x
25. Matsui Y, Asano T, Kenmochi T, Iwakawa M, Imai T, Ochiai T. Effects of Carbon-Ion Beams on Human Pancreatic Cancer Cell Lines That Differ in Genetic Status. Am J Clin Oncol (2004) 27(1):24–8. doi: 10.1097/01.coc.0000046037.75545.ad
26. Sai S, Wakai T, Vares G, Yamada S, Kamijo T, Kamada T, et al. Combination of Carbon Ion Beam and Gemcitabine Causes Irreparable DNA Damage and Death of Radioresistant Pancreatic Cancer Stem-Like Cells In Vitro and In Vivo. Oncotarget (2015) 6(8):5517–35. doi: 10.18632/oncotarget.3584
27. Schlaich F, Brons S, Haberer T, Debus J, Combs SE, Weber KJ. Comparison of the Effects of Photon Versus Carbon Ion Irradiation When Combined With Chemotherapy In Vitro. Radiat Oncol (2013) 8:260. doi: 10.1186/1748-717x-8-260
28. Fujinaga H, Sakai Y, Yamashita T, Arai K, Terashima T, Komura T, et al. Biological Characteristics of Gene Expression Features in Pancreatic Cancer Cells Induced by Proton and X-Ray Irradiation. Int J Radiat Biol (2019) 95(5):571–9. doi: 10.1080/09553002.2019.1558297
29. Galloway NR, Aspe JR, Sellers C, Wall NR. Enhanced Antitumor Effect of Combined Gemcitabine and Proton Radiation in the Treatment of Pancreatic Cancer. Pancreas (2009) 38(7):782–90. doi: 10.1097/MPA.0b013e3181a85999
30. Liubavičiūtė A, Kraśko JA, Mlynska A, Lagzdina J, Sužiedėlis K, Pašukonienė V. Evaluation of Low-Dose Proton Beam Radiation Efficiency in MIA PaCa-2 Pancreatic Cancer Cell Line Vitality and H2AX Formation. Med (Kaunas) (2015) 51(5):302–6. doi: 10.1016/j.medici.2015.10.002
31. Hirai T, Saito S, Fujimori H, Matsushita K, Nishio T, Okayasu R, et al. Radiosensitization by PARP Inhibition to Proton Beam Irradiation in Cancer Cells. Biochem Biophys Res Commun (2016) 478(1):234–40. doi: 10.1016/j.bbrc.2016.07.062
32. Lee M-G, Lee K-S, Nam K-S. The Association of Changes in RAD51 and Survivin Expression Levels With the Proton Beam Sensitivity of Capan-1 and Panc-1 Human Pancreatic Cancer Cells. Int J Oncol (2019) 54(2):744–52. doi: 10.3892/ijo.2018.4642
33. Wera A-C, Lobbens A, Stoyanov M, Lucas S, Michiels C. Radiation-Induced Synthetic Lethality: Combination of Poly(ADP-Ribose) Polymerase and RAD51 Inhibitors to Sensitize Cells to Proton Irradiation. Cell Cycle (2019) 18(15):1770–83. doi: 10.1080/15384101.2019.1632640
34. Lee SH, Mizushima K, Kohno R, Iwata Y, Yonai S, Shirai T, et al. Estimating the Biological Effects of Helium, Carbon, Oxygen, and Neon Ion Beams Using 3D Silicon Microdosimeters. Phys Med Biol (2021) 66(4):045017. doi: 10.1088/1361-6560/abd66f
35. Li C, Heidt DG, Dalerba P, Burant CF, Zhang L, Adsay V, et al. Identification of Pancreatic Cancer Stem Cells. Cancer Res (2007) 67(3):1030–7. doi: 10.1158/0008-5472.Can-06-2030
36. Hermann PC, Huber SL, Herrler T, Aicher A, Ellwart JW, Guba M, et al. Distinct Populations of Cancer Stem Cells Determine Tumor Growth and Metastatic Activity in Human Pancreatic Cancer. Cell Stem Cell (2007) 1(3):313–23. doi: 10.1016/j.stem.2007.06.002
37. Uzawa A, Ando K, Koike S, Furusawa Y, Matsumoto Y, Takai N, et al. Comparison of Biological Effectiveness of Carbon-Ion Beams in Japan and Germany. Int J Radiat Oncol Biol Phys (2009) 73(5):1545–51. doi: 10.1016/j.ijrobp.2008.12.021
38. Held KD, Kawamura H, Kaminuma T, Paz AS, Yoshida Y, Liu Q, et al. Effects of Charged Particles on Human Tumor Cells. Front Oncol (2016) 6:23. doi: 10.3389/fonc.2016.00023
39. Mohamad O, Sishc BJ, Saha J, Pompos A, Rahimi A, Story MD, et al. Carbon Ion Radiotherapy: A Review of Clinical Experiences and Preclinical Research, With an Emphasis on DNA Damage/Repair. Cancers (2017) 9(6):66. doi: 10.3390/cancers9060066
40. Lühr A, von Neubeck C, Krause M, Troost EGC. Relative Biological Effectiveness in Proton Beam Therapy - Current Knowledge and Future Challenges. Clin Transl Radiat Oncol (2018) 9:35–41. doi: 10.1016/j.ctro.2018.01.006
41. Szymonowicz K, Krysztofiak A, Linden JV, Kern A, Deycmar S, Oeck S, et al. Proton Irradiation Increases the Necessity for Homologous Recombination Repair Along With the Indispensability of Non-Homologous End Joining. Cells (2020) 9(4):889. doi: 10.3390/cells9040889
42. Dosanjh M, Jones B, Pawelke J, Pruschy M, Sørensen BS. Overview of Research and Therapy Facilities for Radiobiological Experimental Work in Particle Therapy. Report From the European Particle Therapy Network Radiobiology Group. Radiother Oncol (2018) 128(1):14–8. doi: 10.1016/j.radonc.2018.03.008
43. Höglund E, Blomquist E, Carlsson J, Stenerlöw B. DNA Damage Induced by Radiation of Different Linear Energy Transfer: Initial Fragmentation. Int J Radiat Biol (2000) 76(4):539–47. doi: 10.1080/095530000138556
44. Pang D, Chasovskikh S, Rodgers JE, Dritschilo A. Short DNA Fragments Are a Hallmark of Heavy Charged-Particle Irradiation and May Underlie Their Greater Therapeutic Efficacy. Front Oncol (2016) 6:130. doi: 10.3389/fonc.2016.00130
45. Liermann J, Shinoto M, Syed M, Debus J, Herfarth K, Naumann P. Carbon Ion Radiotherapy in Pancreatic Cancer: A Review of Clinical Data. Radiother Oncol (2020) 147:145–50. doi: 10.1016/j.radonc.2020.05.012
46. Oeck S, Szymonowicz K, Wiel G, Krysztofiak A, Lambert J, Koska B, et al. Relating Linear Energy Transfer to the Formation and Resolution of DNA Repair Foci After Irradiation With Equal Doses of X-Ray Photons, Plateau, or Bragg-Peak Protons. Int J Mol Sci (2018) 19(12):3779. doi: 10.3390/ijms19123779
47. Wang L, Hu J, Liu X, Wang W, Kong L, Lu JJ. Intensity-Modulated Carbon-Ion Radiation Therapy Versus Intensity-Modulated Photon-Based Radiation Therapy in Locally Recurrent Nasopharyngeal Carcinoma: A Dosimetric Comparison. Cancer Manag Res (2019) 11:7767–77. doi: 10.2147/cmar.S205421
48. Weber DC, Trofimov AV, Delaney TF, Bortfeld T. A Treatment Planning Comparison of Intensity Modulated Photon and Proton Therapy for Paraspinal Sarcomas. Int J Radiat Oncol Biol Phys (2004) 58(5):1596–606. doi: 10.1016/j.ijrobp.2003.11.028
49. Schmid TE, Zlobinskaya O, Multhoff G. Differences in Phosphorylated Histone H2AX Foci Formation and Removal of Cells Exposed to Low and High Linear Energy Transfer Radiation. Curr Genomics (2012) 13(6):418–25. doi: 10.2174/138920212802510501
50. Li YH, Wang X, Pan Y, Lee DH, Chowdhury D, Kimmelman AC. Inhibition of Non-Homologous End Joining Repair Impairs Pancreatic Cancer Growth and Enhances Radiation Response. PLoS One (2012) 7(6):e39588. doi: 10.1371/journal.pone.0039588
51. Waissi W, Nicol A, Jung M, Rousseau M, Jarnet D, Noel G, et al. Radiosensitizing Pancreatic Cancer With Parp Inhibitor and Gemcitabine: An In Vivo and a Whole-Transcriptome Analysis After Proton or Photon Irradiation. Cancers (2021) 13(3):1–14. doi: 10.3390/cancers13030527
52. Kirsch DG, Diehn M, Kesarwala AH, Maity A, Morgan MA, Schwarz JK, et al. The Future of Radiobiology. J Natl Cancer Inst (2018) 110(4):329–40. doi: 10.1093/jnci/djx231
53. Gerelchuluun A, Manabe E, Ishikawa T, Sun L, Itoh K, Sakae T, et al. The Major DNA Repair Pathway After Both Proton and Carbon-Ion Radiation Is NHEJ, But the HR Pathway Is More Relevant in Carbon Ions. Radiat Res (2015) 183(3):345–56. doi: 10.1667/rr13904.1
54. Collisson EA, Bailey P, Chang DK, Biankin AV. Molecular Subtypes of Pancreatic Cancer. Nat Rev Gastroenterol Hepatol (2019) 16(4):207–20. doi: 10.1038/s41575-019-0109-y
55. Smit VT, Boot AJ, Smits AM, Fleuren GJ, Cornelisse CJ, Bos JL. KRAS Codon 12 Mutations Occur Very Frequently in Pancreatic Adenocarcinomas. Nucleic Acids Res (1988) 16(16):7773–82. doi: 10.1093/nar/16.16.7773
56. Iwadate Y, Mizoe J, Osaka Y, Yamaura A, Tsujii H. High Linear Energy Transfer Carbon Radiation Effectively Kills Cultured Glioma Cells With Either Mutant or Wild-Type P53. Int J Radiat Oncol Biol Phys (2001) 50(3):803–8. doi: 10.1016/s0360-3016(01)01514-0
57. Takahashi T, Fukawa T, Hirayama R, Yoshida Y, Musha A, Furusawa Y, et al. In Vitro Interaction of High-LET Heavy-Ion Irradiation and Chemotherapeutic Agents in Two Cell Lines With Different Radiosensitivities and Different P53 Status. Anticancer Res (2010) 30(6):1961–7.
58. Burridge K, Wennerberg K. Rho and Rac Take Center Stage. Cell (2004) 116(2):167–79. doi: 10.1016/s0092-8674(04)00003-0
59. Yamazaki D, Kurisu S, Takenawa T. Regulation of Cancer Cell Motility Through Actin Reorganization. Cancer Sci (2005) 96(7):379–86. doi: 10.1111/j.1349-7006.2005.00062.x
60. Liu S, Goldstein RH, Scepansky EM, Rosenblatt M. Inhibition of Rho-Associated Kinase Signaling Prevents Breast Cancer Metastasis to Human Bone. Cancer Res (2009) 69(22):8742–51. doi: 10.1158/0008-5472.Can-09-1541
61. Narumiya S, Tanji M, Ishizaki T. Rho Signaling, ROCK and Mdia1, in Transformation, Metastasis and Invasion. Cancer Metastasis Rev (2009) 28(1-2):65–76. doi: 10.1007/s10555-008-9170-7
62. Tuli R, Shiao SL, Nissen N, Tighiouart M, Kim S, Osipov A, et al. A Phase 1 Study of Veliparib, a PARP-1/2 Inhibitor, With Gemcitabine and Radiotherapy in Locally Advanced Pancreatic Cancer. EBioMedicine (2019) 40:375–81. doi: 10.1016/j.ebiom.2018.12.060
63. Carter RJ, Nickson CM, Thompson JM, Kacperek A, Hill MA, Parsons JL. Complex DNA Damage Induced by High Linear Energy Transfer Alpha-Particles and Protons Triggers a Specific Cellular DNA Damage Response. Int J Radiat Oncol Biol Phys (2018) 100(3):776–84. doi: 10.1016/j.ijrobp.2017.11.012
64. Chaudhary P, Marshall TI, Currell FJ, Kacperek A, Schettino G, Prise KM. Variations in the Processing of DNA Double-Strand Breaks Along 60-MeV Therapeutic Proton Beams. Int J Radiat Oncol Biol Phys (2016) 95(1):86–94. doi: 10.1016/j.ijrobp.2015.07.2279
65. Ma J, Zhang Z, Zhang Z, Huang J, Qin Y, Li X, et al. Magnetic Nanoparticle Clusters Radiosensitise Human Nasopharyngeal and Lung Cancer Cells After Alternating Magnetic Field Treatment. Int J Hyperthermia (2015) 31(7):800–12. doi: 10.3109/02656736.2015.1063168
66. Higgins JP, Altman DG, Gøtzsche PC, Jüni P, Moher D, Oxman AD, et al. The Cochrane Collaboration’s Tool for Assessing Risk of Bias in Randomised Trials. BMJ (2011) 343:d5928. doi: 10.1136/bmj.d5928
Keywords: pancreatic cancer, particle radiation, clonogenic survival, DDR, migration, invasion, systematic review
Citation: Wang D, Liu R, Zhang Q, Luo H, Chen J, Dong M, Wang Y, Ou Y, Liu Z, Sun S, Yang K, Tian J, Li Z and Wang X (2022) Charged Particle Irradiation for Pancreatic Cancer: A Systematic Review of In Vitro Studies. Front. Oncol. 11:775597. doi: 10.3389/fonc.2021.775597
Received: 14 September 2021; Accepted: 06 December 2021;
Published: 04 January 2022.
Edited by:
Alessio G. Morganti, University of Bologna, ItalyReviewed by:
Wan Nordiana Rahman, Universiti Sains Malaysia Health Campus, MalaysiaBarbara Rombi, Azienda Provinciale per i Servizi Sanitari (APSS), Italy
Copyright © 2022 Wang, Liu, Zhang, Luo, Chen, Dong, Wang, Ou, Liu, Sun, Yang, Tian, Li and Wang. This is an open-access article distributed under the terms of the Creative Commons Attribution License (CC BY). The use, distribution or reproduction in other forums is permitted, provided the original author(s) and the copyright owner(s) are credited and that the original publication in this journal is cited, in accordance with accepted academic practice. No use, distribution or reproduction is permitted which does not comply with these terms.
*Correspondence: Xiaohu Wang, xhwang@impcas.ac.cn; Qiuning Zhang, zhangqn@impcas.ac.cn
†These authors have contributed equally to this work and share first authorship