- 1Department of Medical Genetics, School of Medicine, Shahid Beheshti University of Medical Sciences, Tehran, Iran
- 2Biochemistry Group, School of Medicine, Golestan University of Medical Science, Gorgan, Iran
- 3Men’s Health and Reproductive Health Research Center, Shahid Beheshti University of Medical Sciences, Tehran, Iran
- 4Department of Anatomical Sciences, Faculty of Medicine, Birjand University of Medical Sciences, Birjand, Iran
- 5Department of Surgery, Loghman Hakim Hospital, Shahid Beheshti University of Medical Sciences, Tehran, Iran
- 6Skull Base Research Center, Loghman Hakim Hospital, Shahid Beheshti University of Medical Sciences, Tehran, Iran
Breast cancer is the most frequently diagnosed cancer among females. Gene expression profiling methods have shown the deregulation of several genes in breast cancer samples and have confirmed the heterogeneous nature of breast cancer at the genomic level. microRNAs (miRNAs) are among the recently appreciated contributors in breast carcinogenic processes. These small-sized transcripts have been shown to partake in breast carcinogenesis through modulation of apoptosis, autophagy, and epithelial–mesenchymal transition. Moreover, they can confer resistance to chemotherapy. Based on the contribution of miRNAs in almost all fundamental aspects of breast carcinogenesis, therapeutic intervention with their expression might affect the course of this disorder. Moreover, the presence of miRNAs in the peripheral blood of patients potentiates these transcripts as tools for non-invasive diagnosis of breast cancer.
Introduction
Breast cancer is the most frequently diagnosed cancer among females. With approximately 2.3 million new cases, breast cancer accounts for 11.7% of all diagnosed cancers. In terms of cancer-related mortalities, female breast cancer is responsible for 6.9% of mortalities and ranks fifth. Notably, the mortality rate from female breast cancer is significantly higher in developing countries compared with that in developed countries (1). This cancer has been found to be associated with a number of lifestyle and reproductive risk factors, namely, early menarche age, late menopause age, first birth high age, lower period of breastfeeding, hormone replacement therapy after menopause, taking oral contraceptive pills, alcohol intake, and obesity (2). Approximately 5–10% of breast neoplasms are associated with inherited mutations in a number of genes, particularly the BRCA1 and BRCA2 genes (3). In addition, gene expression profiling methods have shown the deregulation of several genes in breast cancer samples and have confirmed the heterogeneous nature of breast cancer at the genomic level (4). More recently, several investigations have reported the dysregulation of microRNAs (miRNAs) in breast cancer samples or plasma samples from these patients in correlation with the functional aspects of tumorigenesis (5–7). miRNAs are produced through a multistep process mediated by two RNase III proteins, namely, Drosha and Dicer (8, 9). These small-sized non-coding transcripts have been found to regulate the expression of a significant proportion of human genes and play fundamental roles in the development of human disorders (10). miRNAs mainly regulate gene expression at post-transcriptional level. Meanwhile, miRNA metabolism and functions are regulated through sophisticated mechanisms (10). Moreover, the expression of miRNA genes is regulated at the transcriptional level through mechanisms similar to the regulatory mechanisms of protein-coding genes. This type of regulation defines the tissue- or developmental stage-specific expression of miRNAs. Most notably, miRNAs can suppress the expression of mRNAs that code factors participating in miRNA biogenesis; thus, they contribute in autoregulatory feedback paths (10). The expression of miRNAs has been reported to be altered in breast cancer samples. As an illustration, recent studies have detected the aberrant expression of miR-221 and miR-222 in breast malignancy (11, 12). In the current review, we describe the impact of miRNAs in breast carcinogenesis and explain their participation in the regulation of apoptosis, autophagy, epithelial–mesenchymal transition (EMT), and resistance to chemotherapy. These processes have important roles in the pathogenesis of cancer. EMT is regarded as a key participant in the invasion and metastasis of cancers. Thus, identifying the main regulators of this process has important implications in cancer treatment. Autophagy has dual roles in cancer progression. Its activation can provide energy and nutrient supplies during the metastatic process, which promotes cell survival in stressful situations (13). In contrast, autophagy can act as a cancer suppressor in the early phase of cancer progression and hinder metastasis through decreasing the expression of important transcription factors for EMT (13). Resistance to apoptotic signals is a key feature in cancer development (14). Moreover, defects in the apoptotic mechanisms enhance malignant transformation and induce the resistance of transformed cells to chemotherapy (14). Finally, resistance to chemotherapy is an important feature gained by tumor cells during tumor evolution, precluding cancer management.
Regulation of Apoptosis by miRNAs in Breast Cancer
Apoptosis is a coordinated process that happens in physiological and pathological contexts. Cancer is one of the contexts where lack of appropriate cell apoptosis results in the survival of malignant cells. Several pathways are involved in the regulation of apoptosis. Defects can happen at any portion of these pathways, resulting in the malignant transformation of cells, facilitation of tumor metastasis, and induction of resistance to anticancer agents (15). miR-7-5p is an example of miRNAs that regulate the apoptosis of breast cancer cells. This miRNA has been shown to target proteasome activator subunit 3 (REGγ), an important modulator of breast cancer and activator of protein proteolysis. The upregulation of miR-7-5p has led to the suppression of proliferation and induction of cell apoptosis in breast cancer through influencing the expression of REGγ (16). This member of the REG family has an oncogenic function which depends on the proteolysis of p21 and p53 (17, 18). miR-15a and miR-16 are two other miRNAs that regulate the apoptosis of breast cancer cells. Luciferase reporter assay has confirmed the interaction between these miRNAs and 3′ UTR of BMI1 transcript. Both miRNAs could suppress the expression of BMI1 at the transcript and protein levels, resulting in the downregulation of anti-apoptotic protein BCL2 and the upregulation of pro-apoptotic proteins. The forced over-expression of these miRNAs has enhanced the levels of mitochondrial reactive oxygen species (ROS), leading to impairment of mitochondrial membrane potential, release of cytochrome c into the cytosol, and activation of Caspase-3 and Caspase-6/9. These events altogether induce the intrinsic pathway of apoptosis (19). miR-17-5p is another miRNA that has been found to induce apoptosis in breast cancer cells. Notably, the upregulation of miR-17-5p has enhanced the sensitivity of breast cancer cells to paclitaxel-associated cell apoptosis through the modulation of STAT3. Consistent with this finding, the upregulation of STAT3 has reduced the paclitaxel-associated apoptosis of MCF-7 cells. miR-17-5p has been found to enhance apoptosis through upregulating the p53 expression, which was suppressed by STAT3. Therefore, miR-17-5p suppresses STAT3 and upregulates p53 to increase breast cancer cell apoptosis (20).
Another study has demonstrated the impact of miR-23a on the suppression of apoptosis in breast cancer cells. Notably, this impact has been exerted in an independent manner from its inhibitory role on the X-linked inhibitor of apoptosis protein, the most potent anti-apoptotic member of the inhibitor-of-apoptosis proteins (21). Notably, the role of miR-23a on the enhancement of invasiveness of breast cancer cells has been verified in xenograft models (22). Several other upregulated miRNAs in breast cancer, such as miR-27a, miR-32, miR-205-3p, miR-221/222, and miR-1271, as well as downregulated miRNAs in breast cancer, such as miR-17-5p, miR-134, miR-139-5p, miR-200b, miR-214, miR-218, miR-543, miR-1301-3p, and miR-4458, have been found to regulate apoptosis in breast cancer cells. Table 1 shows the regulation of apoptosis by miRNAs in breast cancer. Figure 1 demonstrates that the aberrant expression of various miRNAs could contribute in adversely modulating the mitochondrial pathway of apoptosis which is involved in triggering human breast cancer.
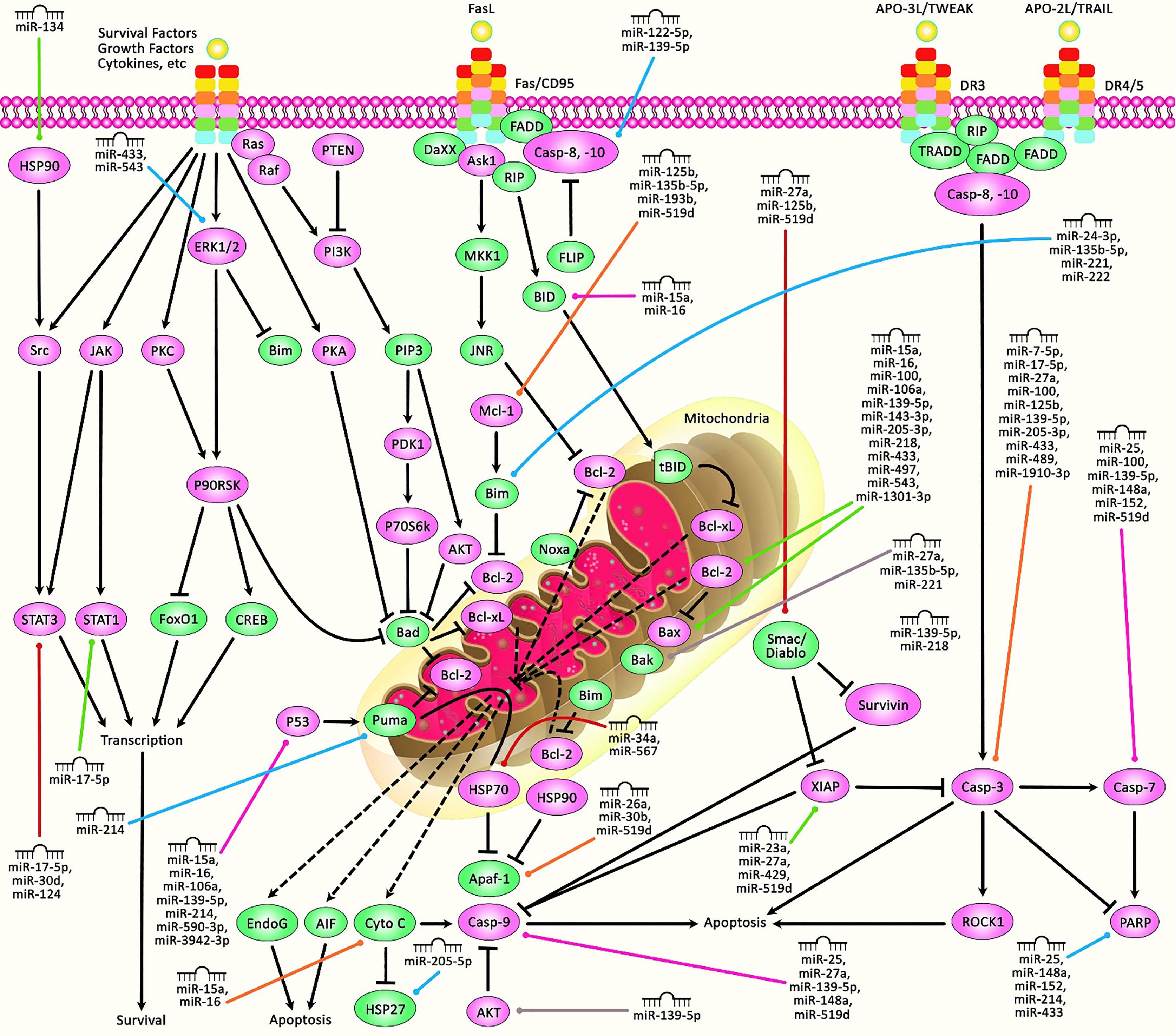
Figure 1 A schematic diagram of the role of miRNAs in triggering the mitochondrial cascade of apoptosis in human breast cancer. Apoptosis pathway could be activated via both extrinsic and intrinsic cascades. The intrinsic pathway is generally occurring through the release of cytochrome c from the mitochondria and modulates mitochondrial outer membrane permeabilization via Bcl-2 family proteins. The activation of extrinsic cascade could be triggered via ligand binding to death receptor, including DR3, DR4, DR5, Fas, and TNFαR. Following that, caspase proteins have a significant part in cleaving target proteins as well as nuclear lamins to elevate DNA degradation, leading to apoptotic cells undergoing phagocytosis. Furthermore, P53, via triggering the upregulation of various proteins containing Bid, Bax, CD95, Puma, and TRAIL-R2, could get effectively involved in activating intrinsic and extrinsic apoptosis cascades. Therefore, any alterations or abnormalities occurring during apoptotic pathways could considerably contribute to the progression of human diseases, including cancer. Previous studies have authenticated that several miRNAs could have a crucial role in regulating the apoptosis pathway in breast cancer. All the information regarding the role of these miRNAs involved in the modulation of breast tumors can be seen in Table 1.
Regulation of Autophagy by miRNAs in Breast Cancer
An autophagy mechanism is initiated by the establishment of autophagosomes that seizure degraded apparatuses and then fuse with lysosomes to induce recycling processes. Autophagy has dual impacts in tumor inhibition and promotion in several types of malignancies. Moreover, autophagy influences cancer stem cell properties through participating in the maintenance of stemness, regulation of tumor recurrence, and induction of resistance to anticancer drugs (56). Autophagy is another subject of regulation by miRNAs in breast cancer cells. miR-20a is among the upregulated miRNAs in breast cancer, particularly in triple-negative breast cancer cells. The expression of miR-20a has been negatively correlated with the activity of the autophagy/lysosome pathway. miR-20a suppresses the basal and nutrient starvation-associated autophagic flux and activity of lysosomal-associated proteolysis. Moreover, this miRNA enhances the intracellular ROS levels and DNA damage response through modulating numerous important regulators of autophagy; among them are BECN1, ATG16L1, and SQSTM1. The expression of miR-20a has been negatively correlated with the expressions of these target genes in breast cancer tissues. Notably, triple-negative cancers have exhibited a particular downregulation of BECN1, ATG16L1, and SQSTM1 genes. The upregulation of miR-20a has also been associated with a higher occurrence of copy number variations and genetic mutations in breast cancer samples. The effects of miR-20a on the enhancement of tumor evolution and growth have also been confirmed in a xenograft model of breast cancer (57). Another study has shown the regulatory effects of miR-20a and miR-20b on the expression of RB1CC1/FIP200. Both miRNAs could decrease the expression of RB1CC1/FIP200 transcripts and proteins. The upregulation of these miRNAs has reduced basal and rapamycin-associated autophagy. Therefore, miR-20a and miR-20b can regulate autophagy through influencing the expression of RB1CC1/FIP200 (58). A high-throughput miRNA sequencing experiment has reported miR-25 as the most important target of isoliquiritigenin (ISL) in inducing autophagy flux. Moreover, mechanistical studies have shown that miR-25 silencing results in cell autophagy through enhancing the expression of ULK1, an early regulator of autophagy initiation. miR-25 upregulation blocks ISL-associated autophagy. ISL has been found to sensitize cancer cells to chemotherapeutic agents as demonstrated by the enhancement in LC3-II levels, decrease in ABCG2 levels, downregulation of miR-25, and activation of ULK1 (59). The inhibitory roles of miR-26b, miR-129-5p, and miR-200c on autophagy are exerted through the modulation of DRAM1 (60), HMGB1 (61), and UBQLN1 (62) expressions, respectively. Notably, miR-129-5p and miR-200c could attenuate irradiation-induced autophagy and decrease the radioresistance of breast cancer cells through this route (61) (62). Table 2 shows the regulation of autophagy by miRNAs in breast cancer. Figure 2 presents the role of several miRNAs in breast cancer cells via regulating the autophagy pathway.
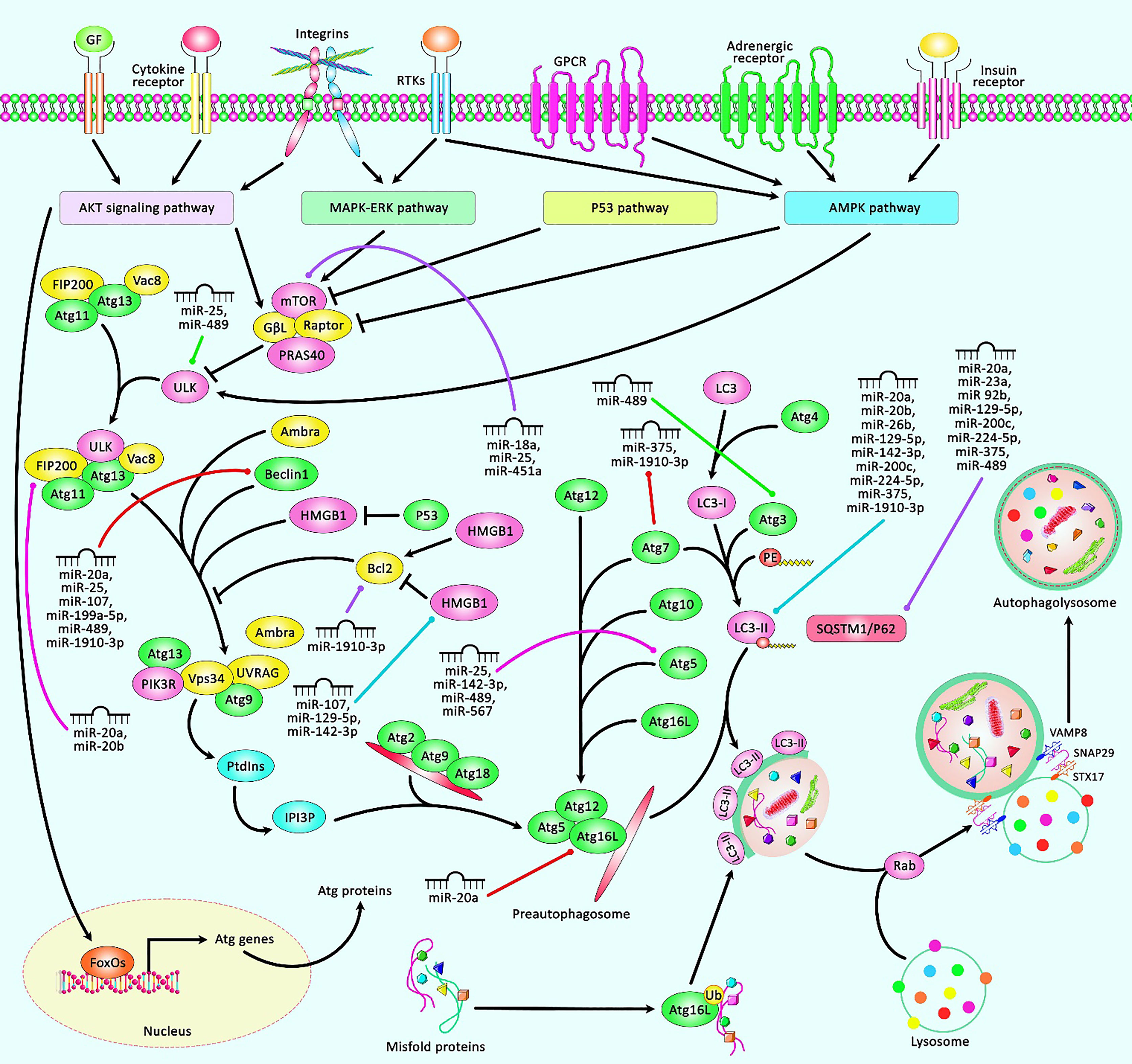
Figure 2 A schematic representation of the role of several miRNAs in regulating the autophagy cascade in human breast cancer. The autophagy pathway is comprised of multiple sequential steps containing sequestration, transport to lysosomes, and degradation. The expression of Atgs could be triggered via AKT, MAKP-ERK, P53, and AMPK pathways. Autophagy is a fundamental substantial biological cascade by removing damaged organelles, but dysregulation of autophagy could contribute to several diseases, including cancers. Accumulating evidence has illustrated that various miRNAs could have a remarkable part in modulating the apoptosis cascade in breast tumors. All the information regarding the role of these miRNAs contributing to the regulation of breast cancer can be seen in Table 2.
Regulation of EMT by miRNAs in Breast Cancer
EMT is a complicated developmental program that permits carcinoma cells to change the epithelial characteristics to mesenchymal features. This alteration permits them to obtain mobility and migration ability. EMT is involved in numerous stages of the metastatic program, from dedifferentiation to aggressiveness (74). TGF-β1-induced EMT has been shown to participate in the metastasis of breast cancer cells. This process is regulated by a number of miRNAs—for instance, miR-23a as an upregulated miRNA in breast cancer cells, particularly in metastatic samples, has been shown to be induced by TGF-β1. The TGF-β1-associated regulation of miR-23a is mediated by direct binding of Smads with the RNA Smad-binding element in miR-23a. The suppression of miR-23a expression has inhibited TGF-β1-associated EMT and attenuated the migration, invasiveness, and metastatic ability of breast cancer cells. miR-23a can directly inhibit the expression of CDH1, a key modulator of EMT. The miR-23a-mediated suppression of CDH1 has been found to activate Wnt/β-catenin signaling. Taken together, miR-23a enhances TGF-β1-associated breast cancer metastasis through influencing the expression of CDH1 and inducing Wnt/β-catenin cascade (75). miR−27a is another upregulated miRNA in breast cancer samples and cell lines. The upregulation of miR−27a has increased the migratory potential of breast cancer cells through induction of EMT. FBXW7 has been identified as a downstream target of miR−27a. The over-expression of FBXW7 in breast cancer cells could inhibit EMT and the migratory aptitude of these cells. Therefore, miR−27a can regulate the metastatic potential of breast cancer through the suppression of FBXW7 (76). miR-29a has also been found to be upregulated in breast cancer samples in correlation with distant metastasis and poor clinical outcome of patients. miR-29a silencing has suppressed the proliferation and migration of breast cancer cells. Ten eleven translocation 1 (TET1) has been identified as a target of miR-29a. The upregulation of TET1 has attenuated the proliferation and migration of breast cancer cells. The miR-29a-mediated downregulation of TET1 enhances EMT (77). Several upregulated miRNAs in breast cancer, such as miR-93, miR-125b, miR-199a-3p, and miR-221, as well as downregulated miRNAs, such as miR-34a, miR-92b, miR-124, miR-138-5p, miR-153, miR-516a-3p, and miR-524-5p, affect the EMT process. Table 3 shows the regulation of EMT by miRNAs in breast cancer. Figure 3 depicts the role of various miRNAs in the modulation of EMT via targeting receptors that convey signals from EMT inducers or multiple EMT components.
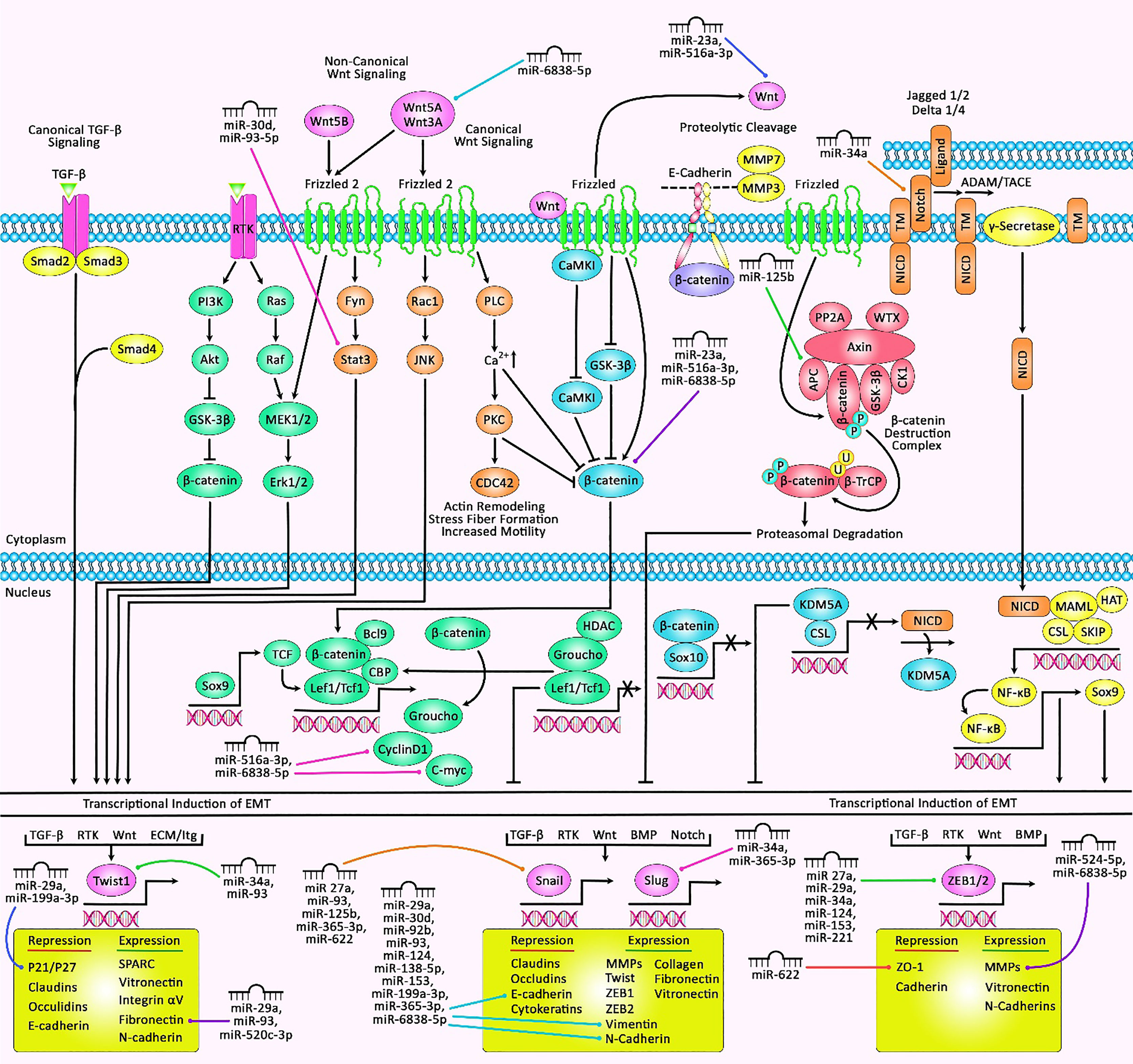
Figure 3 A schematic illustration of the epithelial–mesenchymal transition (EMT)‐associated miRNAs and their roles in human breast cancer. EMT is a process that can be induced via a variety of growth factors and cytokines in cancer cells. These elements may be secreted through the cancer cells themselves or via the stromal cells in the tumor microenvironment. These soluble ligands can interact with their cognate receptors, such as TGF-β receptors and RTKs, resulting in the activation of several oncogenic pathways (TGF-β, Wnt/β-catenin, integrins, Notch, etc.) which have a significant role in inducing the EMT cascade. Thereby, the activation of EMT can be triggered through the overexpression of selected zinc finger, including ZEB1/2, snail, slug, or basic helix–loop–helix containing TWIST1 transcription factors (95, 96). Recent studies have detected he regulatory role of multiple miRNAs in EMT and breast cancer cells. All the information regarding the influence of these miRNAs in EMT and the control that they exert in major signaling cascades in breast cancer can be seen in Table 3.
Regulation of Chemoresistance Breast Cancer Cells by miRNAs
Chemoresistance is a phenotype which is associated with several signaling pathways as well as cellular processes such as apoptosis, autophagy, and EMT. miRNAs have also been found to affect the resistance of breast cancer cells to important chemotherapeutic drugs—for instance, miR-7 has been shown to be downregulated in MCF-7 and adriamycin-resistant cells (MCF-7/ADR cells), particularly in MCF-7/ADR cells. The upregulation of miR-7 has enhanced sensitivity of MCF-7/ADR cells to ADR. The downregulation has led to the upregulation of EGFR and PI3K, while the upregulation of miR-7 has been associated with opposite effects. Moreover, the suppression of miR-7 has been associated with the enhancement of proliferation and inhibition of apoptosis. Therefore, miR-7 has been found to affect the resistance of breast cancer cells to ADR, and its upregulation can enhance the effects of ADR through the suppression of EGFR/PI3K signaling (97). miR-30c is another miRNA that is involved in intrinsic adriamycin resistance in p53-mutated breast cancer (98).
Moreover, another study in breast cancer has shown a correlation between high miR-7 levels and better pathological complete response to paclitaxel/carboplatin. Functionally, miR-7 has been shown to sensitize MCF-7 and MDA-MB-231 cells to the cytotoxic effects of paclitaxel and carboplatin through targeting MRP1 and BCL2. Taken together, miR-7 has been suggested as a predictive marker for the assessment of chemotherapy efficacy and therapeutic target for the enhancement of response of breast cancer patients to chemotherapy (99). The expression assays in an Src inhibitor saracatinib-resistant breast cancer cell line (SK-BR-3/SI) has shown the downregulation of miR-19b-3p in saracatinib-resistant cells compared with saracatinib-sensitive ones. The under-expression of miR-19b-3p not only has been associated with higher IC50 value of saracatinib but also has increased the migratory potential of breast cancer cells. Functionally, miR-19b-3p targets PIK3CA. Thus, the resistance to Src inhibitors might be due to the enhancement of the activity of PI3K/Akt pathway following miR-19b-3p downregulation (100). In addition, miR-34a could affect the sensitivity of breast cancer cells to sunitinib by regulating the Wnt/β-catenin signaling pathway (101).
miR-24-3p is another miRNA which can regulate the sensitivity of breast cancer cells to tamoxifen. The upregulation of miR-24-3p has been shown to increase tamoxifen-induced cytotoxicity in breast cancer cells, while its silencing has decreased these effects. Bim has been identified as a target of miR-24-3p in breast cancer. Further experiments have shown the upregulation of miR-24-3p and the downregulation of BIM expression in tamoxifen-resistant MCF7 cells compared with original cells. Moreover, the suppression of miR-24-3p has enhanced the sensitivity of MCF7/TAM cells to tamoxifen through the enhancement of cell apoptosis (102). Besides this, miR-148a and miR-152, by downregulating ALCAM, could reduce tamoxifen resistance in ER+ breast cancer cells (33). miR-375 is another miRNA that could inhibit cancer stem cell phenotype and tamoxifen resistance in human ER+ breast cancer cells through degrading HOXB3 (103). Meanwhile, tamoxifen has been shown to regulate the expressions of miR-29b-1 and miR-29a (104). Table 4 shows the role of miRNAs in the regulation of response of breast cancer to therapeutic agents.
Discussion
Non-coding RNAs can influence the expression of several groups of mRNAs through different mechanisms, such as modulation of chromatin structure as well as regulation of transcription and translation. miRNAs are mostly exerting their regulatory roles at the post-transcriptional level through binding to different regions of mRNAs to suppress their translation via mRNA degradation or translation inhibition. miRNAs have been found to regulate important aspects of breast carcinogenesis through the regulation of apoptosis, autophagy, and EMT. miRNAs affect the apoptosis of breast cancer cells through several mechanisms; among them are modulation of p53-related pathways, expression of caspases, and regulation of response to ROS. Through modulating the expression of EMT-related genes as well as those influencing cell motility and invasiveness, miRNAs regulate breast cancer metastasis. Notably, miRNAs can also influence the response of breast cancer cells to a wide array of therapeutic agents ranging from conventional chemotherapeutic drugs to tyrosine kinase inhibitors and hormone therapy agents. Based on in vitro experiments, miRNAs can regulate the cytotoxic effects of adriamycin, cisplatin, doxorubicin, docetaxel, paclitaxel, gemcitabine, trastuzumab, saracatinib, sunitinib, tamoxifen, and a number of other anti-cancer drugs. In addition to miRNAs whose direct effects on the modulation of response to therapeutic agents have been verified, other miRNAs that regulate cell apoptosis or autophagy can potentially influence therapeutic responses. The modulation of cellular DNA damage response and the activity of cancer stem cells are other routes of participation of miRNAs in the regulation of response of breast cancer cells to chemotherapy. A possible role of miRNAs in the determination of breast cancer stem cells has been suggested through the demonstration of differential expression of miRNAs in CD44+/CD24-/low breast cancer stem cells versus non-tumorigenic cancer cells (145). This kind of function of miRNAs has a practical significance in the determination of the behavior of breast cancer as well as its response to therapeutic modalities. In addition, a number of anti-cancer agents exert their effects through the modulation of the expression of miRNAs that affect apoptosis or autophagy—for instance, curcumol, via regulating the miR-181b-2-3p/ABCC3 axis, could enhance the sensitivity of triple-negative breast cancer cells to doxorubicin (133). Some miRNAs can affect several aspects of breast carcinogenesis—for instance, miR-34a can affect apoptosis, EMT, and drug resistance. miR-23a has an essential role in the regulation of apoptosis and EMT. Moreover, miR-15a and miR-16a regulate apoptosis and drug resistance. NF-κB, mTOR, and Wnt/β-catenin pathways are among the shared pathways between several miRNAs acting on these processes. Since miRNAs can target multiple transcripts, they can often modulate numerous pathways. Notably, miRNAs exert their inhibitory roles via a complex process which is dependent on cellular constituents, indicating tissue or cell type-specific features (146).
The small molecular size of miRNAs and their capacity in the regulation of the expression of genes participating in the evolution of cancer have endowed miRNAs the potential to influence the treatment of breast cancer (147). As miRNAs can affect both the development of breast cancer and the response of cancerous cells to therapeutic options, intervention with their expression is regarded as an appropriate treatment modality for almost every stage of cancer development and progression. Forced over-expression or suppression of miRNA expression is a possible therapeutic modality for breast cancer. Examples of miRNA-antagonism methods are 2′-O-methyl-modified oligonucleotides, locked nucleic acid anti-miRNAs, and cholesterol-conjugated antagomirs. These methods are being used as miRNA-inhibitory tools (148). In fact, the over-expression of miRNAs that induce cell apoptosis, such as miR-7-5p (16), miR-15a, miR-16 (19), and miR-17-5p (20), or inhibit cell cycle progression can suppress the progression of breast cancer. On the other hand, the suppression of expression of oncogenic miRNAs by oligo antisense mechanisms is a treatment modality. In vitro studies have provided a firm evidence for the specificity and efficacy of miRNA-based modalities in the modulation of the expression of target genes, yet future studies should focus on the improvement of delivery systems, enhancement of stability of the prescribed molecules, decreasing off-target effects, and assessment of long-term safety of these molecules (149). Only after solving these issues can miRNA-based therapeutics enter clinical practice.
The identification of the miRNA-associated network and interplay between miRNAs and other types of regulatory transcripts will open new opportunities for diagnostics and therapeutic modalities in breast cancer. System biology methods can be used to predict the role of miRNAs in the determination of response to anti-cancer therapies and prognostic approaches in clinical settings. Targeting miRNAs with essential roles in a drug-resistant network has been suggested as a putative approach in overcoming chemoresistance in breast cancer (146). Finally, the combinations of conventional anticancer drugs with anti-oncogenic miRNA reagents are expected to enhance treatment responses. In fact, the recognition of miRNA profiles in different stages of breast cancer development and development of miRNA-based targeted therapies are two wings of miRNA studies which can introduce novel promising results in clinical settings.
In brief, the contribution of miRNAs in the regulation of cell death, cell motility and invasion, activity of cancer stem cells, regulation of EMT, and modulation of response to therapeutics potentiate miRNAs as proper targets for the treatment of breast cancer. However, the clinical application of miRNA-based therapies depends on the effective documentation of miRNA profiles in different subtypes of breast cancer and the construction of the interaction network between miRNAs and genes that regulate breast carcinogenesis and chemoresistance phenotype.
Author Contributions
SG-F wrote the draft and revised it. MT designed and supervised the study. AK, AA, HS, and AS collected the data, designed the figures and tables. All the authors read and approved the submitted version.
Conflict of Interest
The authors declare that the research was conducted in the absence of any commercial or financial relationships that could be construed as a potential conflict of interest.
Publisher’s Note
All claims expressed in this article are solely those of the authors and do not necessarily represent those of their affiliated organizations, or those of the publisher, the editors and the reviewers. Any product that may be evaluated in this article, or claim that may be made by its manufacturer, is not guaranteed or endorsed by the publisher.
References
1. Sung H, Ferlay J, Siegel RL, Laversanne M, Soerjomataram I, Jemal A, et al. Global Cancer Statistics 2020: GLOBOCAN Estimates of Incidence and Mortality Worldwide for 36 Cancers in 185 Countries. CA: Cancer J Clin (2021) 71(3):209–49. doi: 10.3322/caac.21660
2. Brinton L, Gaudet M, Gierach G. Cancer Epidemiology and Prevention. New York: Oxford University Press (2018).
3. Feng Y, Spezia M, Huang S, Yuan C, Zeng Z, Zhang L, et al. Breast Cancer Development and Progression: Risk Factors, Cancer Stem Cells, Signaling Pathways, Genomics, and Molecular Pathogenesis. Genes Dis (2018) 5(2):77–106. doi: 10.1016/j.gendis.2018.05.001
4. Bao T, Davidson NE. Gene Expression Profiling of Breast Cancer. Adv Surg (2008) 42:249–60. doi: 10.1016/j.yasu.2008.03.002
5. Elango R, Alsaleh KA, Vishnubalaji R, Manikandan M, Ali AM, El-Aziz A, et al. MicroRNA Expression Profiling on Paired Primary and Lymph Node Metastatic Breast Cancer Revealed Distinct microRNA Profile Associated With LNM. Front Oncol (2020) 10:756. doi: 10.3389/fonc.2020.00756
6. Hamam R, Ali AM, Alsaleh KA, Kassem M, Alfayez M, Aldahmash A, et al. microRNA Expression Profiling on Individual Breast Cancer Patients Identifies Novel Panel of Circulating microRNA for Early Detection. Sci Rep (2016) 6(1):1–8. doi: 10.1038/srep25997
7. Hu Z, Dong J, Wang L-E, Ma H, Liu J, Zhao Y, et al. Serum microRNA Profiling and Breast Cancer Risk: The Use of miR-484/191 as Endogenous Controls. Carcinogenesis (2012) 33(4):828–34. doi: 10.1093/carcin/bgs030
8. Ha M, Kim VN. Regulation of microRNA Biogenesis. Nat Rev Mol Cell Biol (2014) 15(8):509–24. doi: 10.1038/nrm3838
9. Treiber T, Treiber N, Meister G. Regulation of microRNA Biogenesis and its Crosstalk With Other Cellular Pathways. Nat Rev Mol Cell Biol (2019) 20(1):5–20. doi: 10.1038/s41580-018-0059-1
10. Krol J, Loedige I, Filipowicz W. The Widespread Regulation of microRNA Biogenesis, Function and Decay. Nat Rev Genet (2010) 11(9):597–610. doi: 10.1038/nrg2843
11. Abak A, Amini S, Estiar MA, Montazeri V, Sakhinia E, Abhari A. Analysis of miRNA-221 Expression Level in Tumors and Marginal Biopsies From Patients With Breast Cancer (Cross-Sectional Observational Study). Clin Lab (2018) 64(1):169–75. doi: 10.7754/Clin.Lab.2017.170821
12. Amini S, Abak A, Estiar MA, Montazeri V, Abhari A, Sakhinia E. Expression Analysis of MicroRNA-222 in Breast Cancer. Clin Lab (2018) 64(4):491–6. doi: 10.7754/Clin.Lab.2017.171002
13. Chen H-T, Liu H, Mao M-J, Tan Y, Mo X-Q, Meng X-J, et al. Crosstalk Between Autophagy and Epithelial-Mesenchymal Transition and its Application in Cancer Therapy. Mol Cancer (2019) 18(1):1–19. doi: 10.1186/s12943-019-1030-2
14. Plati J, Bucur O, Khosravi-Far R. Dysregulation of Apoptotic Signaling in Cancer: Molecular Mechanisms and Therapeutic Opportunities. J Cell Biochem (2008) 104(4):1124–49. doi: 10.1002/jcb.21707
15. Wong RSY. Apoptosis in Cancer: From Pathogenesis to Treatment. J Exp Clin Cancer Res (2011) 30(1):87–. doi: 10.1186/1756-9966-30-87
16. Shi Y, Luo X, Li P, Tan J, Wang X, Xiang T, et al. miR-7-5p Suppresses Cell Proliferation and Induces Apoptosis of Breast Cancer Cells Mainly by Targeting Regγ. Cancer Lett (2015) 358(1):27–36. doi: 10.1016/j.canlet.2014.12.014
17. Gao G, Wong J, Zhang J, Mao I, Shravah J, Wu Y, et al. Proteasome Activator Regγ Enhances Coxsackieviral Infection by Facilitating P53 Degradation. J Virol (2010) 84(21):11056–66. doi: 10.1128/JVI.00008-10
18. Li X, Amazit L, Long W, Lonard DM, Monaco JJ, O’Malley BW. Ubiquitin-And ATP-Independent Proteolytic Turnover of P21 by the Regγ-Proteasome Pathway. Mol Cell (2007) 26(6):831–42. doi: 10.1016/j.molcel.2007.05.028
19. Patel N, Garikapati KR, Ramaiah MJ, Polavarapu KK, Bhadra U, Bhadra MP. miR-15a/miR-16 Induces Mitochondrial Dependent Apoptosis in Breast Cancer Cells by Suppressing Oncogene BMI1. Life Sci (2016) 164:60–70. doi: 10.1016/j.lfs.2016.08.028
20. Liao X-H, Xiang Y, Yu C-X, Li J-P, Li H, Nie Q, et al. STAT3 is Required for MiR-17-5p-Mediated Sensitization to Chemotherapy-Induced Apoptosis in Breast Cancer Cells. Oncotarget (2017) 8(9):15763. doi: 10.18632/oncotarget.15000
21. Obexer P, Ausserlechner MJ. X-Linked Inhibitor of Apoptosis Protein–a Critical Death Resistance Regulator and Therapeutic Target for Personalized Cancer Therapy. Front Oncol (2014) 4:197. doi: 10.3389/fonc.2014.00197
22. Chen P, He Y-H, Huang X, Tao S-Q, Wang X-N, Yan H, et al. MiR-23a Modulates X-Linked Inhibitor of Apoptosis-Mediated Autophagy in Human Luminal Breast Cancer Cell Lines. Oncotarget (2017) 8(46):80709. doi: 10.18632/oncotarget.21080
23. Zhou S, Huang Q, Zheng S, Lin K, You J, Zhang X. miR-27a Regulates the Sensitivity of Breast Cancer Cells to Cisplatin Treatment via BAK-SMAC/DIABLO-XIAP Axis. Tumor Biol (2016) 37(5):6837–45. doi: 10.1007/s13277-015-4500-1
24. Xia W, Zhou J, Luo H, Liu Y, Peng C, Zheng W, et al. MicroRNA-32 Promotes Cell Proliferation, Migration and Suppresses Apoptosis in Breast Cancer Cells by Targeting FBXW7. Cancer Cell Int (2017) 17(1):1–11. doi: 10.1186/s12935-017-0383-0
25. He R, Liu P, Xie X, Zhou Y, Liao Q, Xiong W, et al. Circgfra1 and GFRA1 Act as ceRNAs in Triple Negative Breast Cancer by Regulating miR-34a. J Exp Clin Cancer Res (2017) 36(1):1–12. doi: 10.1186/s13046-017-0614-1
26. Gong Y, He T, Yang L, Yang G, Chen Y, Zhang X. The Role of miR-100 in Regulating Apoptosis of Breast Cancer Cells. Sci Rep (2015) 5(1):1–13. doi: 10.1038/srep11650
27. You F, Li J, Zhang P, Zhang H, Cao X. Mir106a Promotes the Growth of Transplanted Breast Cancer and Decreases the Sensitivity of Transplanted Tumors to Cisplatin. Cancer Manag Res (2020) 12:233–46. doi: 10.2147/CMAR.S231375
28. Xie X, Hu Y, Xu L, Fu Y, Tu J, Zhao H, et al. The Role of miR-125b-Mitochondria-Caspase-3 Pathway in Doxorubicin Resistance and Therapy in Human Breast Cancer. Tumor Biol (2015) 36(9):7185–94. doi: 10.1007/s13277-015-3438-7
29. O’Brien K, Lowry MC, Corcoran C, Martinez VG, Daly M, Rani S, et al. miR-134 in Extracellular Vesicles Reduces Triple-Negative Breast Cancer Aggression and Increases Drug Sensitivity. Oncotarget (2015) 6(32):32774. doi: 10.18632/oncotarget.5192
30. Gu S, Luo J, Yao W. The Regulation of miR-139-5p on the Biological Characteristics of Breast Cancer Cells by Targeting COL11A1. Math Biosci Eng (2020) 17(2):1428–41. doi: 10.3934/mbe.2020073
31. Sun D-w, Mao L, Zhang J, Jiang L-h, Li J, Wu Y, et al. MiR-139-5p Inhibits the Biological Function of Breast Cancer Cells by Targeting Notch1 and Mediates Chemosensitivity to Docetaxel. Biochem Biophys Res Commun (2015) 465(4):702–13. doi: 10.1016/j.bbrc.2015.08.053
32. Chen J, Chen X. MYBL2 Is Targeted by miR-143-3p and Regulates Breast Cancer Cell Proliferation and Apoptosis. Oncol Res (2017) 26(6):913–22. doi: 10.3727/096504017X15135941182107
33. Chen M-J, Cheng Y-M, Chen C-C, Chen Y-C, Shen C-J. MiR-148a and miR-152 Reduce Tamoxifen Resistance in ER+ Breast Cancer via Downregulating ALCAM. Biochem Biophys Res Commun (2017) 483(2):840–6. doi: 10.1016/j.bbrc.2017.01.012
34. Jin Y, Yang L, Li X, Liu F. Circular RNA KIF4A Promotes Cell Migration, Invasion and Inhibits Apoptosis Through miR-152/ZEB1 Axis in Breast Cancer. Diagn Pathol (2020) 15:1–9. doi: 10.1186/s13000-020-00963-7
35. Long J, Ji Z, Jiang K, Wang Z, Meng G. miR-193b Modulates Resistance to Doxorubicin in Human Breast Cancer Cells by Downregulating MCL-1. BioMed Res Int (2015) 2015. doi: 10.1155/2015/373574
36. Fan X, Zhou S, Zheng M, Deng X, Yi Y, Huang T. MiR-199a-3p Enhances Breast Cancer Cell Sensitivity to Cisplatin by Downregulating TFAM (TFAM). Biomed Pharmacother (2017) 88:507–14. doi: 10.1016/j.biopha.2017.01.058
37. Yao Y, Hu J, Shen Z, Yao R, Liu S, Li Y, et al. MiR-200b Expression in Breast Cancer: A Prognostic Marker and Act on Cell Proliferation and Apoptosis by Targeting Sp1. J Cell Mol Med (2015) 19(4):760–9. doi: 10.1111/jcmm.12432
38. Qiu C, Huang F, Zhang Q, Chen W, Zhang H. miR-205-3p Promotes Proliferation and Reduces Apoptosis of Breast Cancer MCF-7 Cells and is Associated With Poor Prognosis of Breast Cancer Patients. J Clin Lab Anal (2019) 33(8):e22966. doi: 10.1002/jcla.22966
39. Zhang J, Su B, Gong C, Xi Q, Chao T. miR-214 Promotes Apoptosis and Sensitizes Breast Cancer Cells to Doxorubicin by Targeting the RFWD2-P53 Cascade. Biochem Biophys Res Commun (2016) 478(1):337–42. doi: 10.1016/j.bbrc.2016.07.054
40. Liu B, Tian Y, Li F, Zhao Z, Jiang X, Zhai C, et al. Tumor-Suppressing Roles of miR-214 and miR-218 in Breast Cancer. Oncol Rep (2016) 35(6):3178–84. doi: 10.3892/or.2016.4749
41. Hu Y, Xu K, Yagüe E. miR-218 Targets Survivin and Regulates Resistance to Chemotherapeutics in Breast Cancer. Breast Cancer Res Treat (2015) 151(2):269–80. doi: 10.1007/s10549-015-3372-9
42. Ye Z, Hao R, Cai Y, Wang X, Huang G. Knockdown of miR-221 Promotes the Cisplatin-Inducing Apoptosis by Targeting the BIM-Bax/Bak Axis in Breast Cancer. Tumor Biol (2016) 37(4):4509–15. doi: 10.1007/s13277-015-4267-4
43. Zong Y, Zhang Y, Sun X, Xu T, Cheng X, Qin Y. miR-221/222 Promote Tumor Growth and Suppress Apoptosis by Targeting lncRNA GAS5 in Breast Cancer. Biosci Rep (2019) 39(1). doi: 10.1042/BSR20181859
44. Wang C, Ju H, Shen C, Tong Z. miR-429 Mediates δ-Tocotrienol-Induced Apoptosis in Triple-Negative Breast Cancer Cells by Targeting XIAP. Int J Clin Exp Med (2015) 8(9):15648.
45. Zhang T, Jiang K, Zhu X, Zhao G, Wu H, Deng G, et al. miR-433 Inhibits Breast Cancer Cell Growth via the MAPK Signaling Pathway by Targeting Rap1a. Int J Biol Sci (2018) 14(6):622. doi: 10.7150/ijbs.24223
46. Wang W, Zhang L, Wang Y, Ding Y, Chen T, Wang Y, et al. Involvement of miR-451 in Resistance to Paclitaxel by Regulating YWHAZ in Breast Cancer. Cell Death Dis (2017) 8(10):e3071–e. doi: 10.1038/cddis.2017.460
47. Wu Z, Li X, Cai X, Huang C, Zheng M. miR-497 Inhibits Epithelial Mesenchymal Transition in Breast Carcinoma by Targeting Slug. Tumor Biol (2016) 37(6):7939–50. doi: 10.1007/s13277-015-4665-7
48. Xie Q, Wang S, Zhao Y, Zhang Z, Qin C, Yang X. MiR-519d Impedes Cisplatin-Resistance in Breast Cancer Stem Cells by Down-Regulating the Expression of MCL-1. Oncotarget (2017) 8(13):22003. doi: 10.18632/oncotarget.15781
49. Chen P, Xu W, Luo Y, Zhang Y, He Y, Yang S, et al. MicroRNA 543 Suppresses Breast Cancer Cell Proliferation, Blocks Cell Cycle and Induces Cell Apoptosis via Direct Targeting of ERK/MAPK. OncoTargets Ther (2017) 10:1423. doi: 10.2147/OTT.S118366
50. Abdolvahabi Z, Nourbakhsh M, Hosseinkhani S, Hesari Z, Alipour M, Jafarzadeh M, et al. MicroRNA-590-3P Suppresses Cell Survival and Triggers Breast Cancer Cell Apoptosis via Targeting Sirtuin-1 and Deacetylation of P53. J Cell Biochem (2019) 120(6):9356–68. doi: 10.1002/jcb.28211
51. Liang H-F, Zhang X-Z, Liu B-G, Jia G-T, Li W-L. Circular RNA Circ-ABCB10 Promotes Breast Cancer Proliferation and Progression Through Sponging miR-1271. Am J Cancer Res (2017) 7(7):1566.
52. Peng X, Yan B, Shen Y. MiR-1301-3p Inhibits Human Breast Cancer Cell Proliferation by Regulating Cell Cycle Progression and Apoptosis Through Directly Targeting ICT1. Breast Cancer (2018) 25(6):742–52. doi: 10.1007/s12282-018-0881-5
53. Zhao J, Zou H, Han C, Ma J, Zhao J, Tang J. Circlular RNA BARD1 (Hsa_circ_0001098) Overexpression in Breast Cancer Cells With TCDD Treatment Could Promote Cell Apoptosis via miR-3942/BARD1 Axis. Cell Cycle (2018) 17(24):2731–44. doi: 10.1080/15384101.2018.1556058
54. Gholipour N, Ohradanova-Repic A, Ahangari G. A Novel Report of MiR-4301 Induces Cell Apoptosis by Negatively Regulating DRD2 Expression in Human Breast Cancer Cells. J Cell Biochem (2018) 119(8):6408–17. doi: 10.1002/jcb.26577
55. Liu X, Wang J, Zhang G. miR-4458 Regulates Cell Proliferation and Apoptosis Through Targeting SOCS1 in Triple-Negative Breast Cancer. J Cell Biochem (2019) 120(8):12943–8. doi: 10.1002/jcb.28565
56. Yun CW, Lee SH. The Roles of Autophagy in Cancer. Int J Mol Sci (2018) 19(11):3466. doi: 10.3390/ijms19113466
57. Liu L, He J, Wei X, Wan G, Lao Y, Xu W, et al. MicroRNA-20a-Mediated Loss of Autophagy Contributes to Breast Tumorigenesis by Promoting Genomic Damage and Instability. Oncogene (2017) 36(42):5874–84. doi: 10.1038/onc.2017.193
58. Alameen AA, Simioni C, Martelli AM, Zauli G, Ultimo S, McCubrey JA, et al. Healthy CD4+ T Lymphocytes are Not Affected by Targeted Therapies Against the PI3K/Akt/mTOR Pathway in T-Cell Acute Lymphoblastic Leukemia. Oncotarget (2016) 7(34):55690. doi: 10.18632/oncotarget.10984
59. Wang Z, Wang N, Liu P, Chen Q, Situ H, Xie T, et al. MicroRNA-25 Regulates Chemoresistance-Associated Autophagy in Breast Cancer Cells, a Process Modulated by the Natural Autophagy Inducer Isoliquiritigenin. Oncotarget (2014) 5(16):7013. doi: 10.18632/oncotarget.2192
60. Meng C, Liu Y, Shen Y, Liu S, Wang Z, Ye Q, et al. MicroRNA-26b Suppresses Autophagy in Breast Cancer Cells by Targeting DRAM1 mRNA, and is Downregulated by Irradiation. Oncol Lett (2018) 15(2):1435–40. doi: 10.3892/ol.2017.7452
61. Luo J, Chen J, He L. Mir-129-5p Attenuates Irradiation-Induced Autophagy and Decreases Radioresistance of Breast Cancer Cells by Targeting Hmgb1. Med Sci Monit (2015) 21:4122–9. doi: 10.12659/MSM.896661
62. Sun Q, Liu T, Yuan Y, Guo Z, Xie G, Du S, et al. MiR-200c Inhibits Autophagy and Enhances Radiosensitivity in Breast Cancer Cells by Targeting UBQLN1. Int J Cancer (2015) 136(5):1003–12. doi: 10.1002/ijc.29065
63. Liu L, Shen W, Zhu Z, Lin J, Fang Q, Ruan Y, et al. Combined Inhibition of EGFR and C-ABL Suppresses the Growth of Fulvestrant-Resistant Breast Cancer Cells Through miR-375-Autophagy Axis. Biochem Biophys Res Commun (2018) 498(3):559–65. doi: 10.1016/j.bbrc.2018.03.019
64. Cheng Y, Li Z, Xie J, Wang P, Zhu J, Li Y, et al. MiRNA-224-5p Inhibits Autophagy in Breast Cancer Cells via Targeting Smad4. Biochem Biophys Res Commun (2018) 506(4):793–8. doi: 10.1016/j.bbrc.2018.10.150
65. Liu Z-R, Song Y, Wan L-H, Zhang Y-Y, Zhou L-M. Over-Expression of miR-451a can Enhance the Sensitivity of Breast Cancer Cells to Tamoxifen by Regulating 14-3-3ζ, Estrogen Receptor α, and Autophagy. Life Sci (2016) 149:104–13. doi: 10.1016/j.lfs.2016.02.059
66. Liang L, Fu J, Wang S, Cen H, Zhang L, Mandukhail SR, et al. MiR-142-3p Enhances Chemosensitivity of Breast Cancer Cells and Inhibits Autophagy by Targeting HMGB1. Acta Pharm Sin B (2020) 10(6):1036–46. doi: 10.1016/j.apsb.2019.11.009
67. Wang B, Mao J-h, Wang B-y, Wang L-x, Wen H-y, Xu L-j, et al. Exosomal miR-1910-3p Promotes Proliferation, Metastasis, and Autophagy of Breast Cancer Cells by Targeting MTMR3 and Activating the NF-κb Signaling Pathway. Cancer Lett (2020) 489:87–99. doi: 10.1016/j.canlet.2020.05.038
68. Soni M, Patel Y, Markoutsa E, Jie C, Liu S, Xu P, et al. Autophagy, Cell Viability, and Chemoresistance are Regulated by miR-489 in Breast Cancer. Mol Cancer Res (2018) 16(9):1348–60. doi: 10.1158/1541-7786.MCR-17-0634
69. Shi Y, Gong W, Lu L, Wang Y, Ren J. Upregulation of miR-129-5p Increases the Sensitivity to Taxol Through Inhibiting HMGB1-Mediated Cell Autophagy in Breast Cancer MCF-7 Cells. Braz J Med Biol Res (2019) 52(11). doi: 10.1590/1414-431x20198657
70. Fan Y, Dai Y, Wang X, Ren Y, Han J, Zhang H. MiR-18a Upregulation Enhances Autophagy in Triple Negative Cancer Cells via Inhibiting mTOR Signaling Pathway. Eur Rev Med Pharmacol Sci (2016) 20(11):2194–200.
71. Ai H, Zhou W, Wang Z, Qiong G, Chen Z, Deng S. microRNAs-107 Inhibited Autophagy, Proliferation, and Migration of Breast Cancer Cells by Targeting HMGB1. J Cell Biochem (2019) 120(5):8696–705. doi: 10.1002/jcb.28157
72. Liu F, Sang M, Meng L, Gu L, Liu S, Li J, et al. Mir−92b Promotes Autophagy and Suppresses Viability and Invasion in Breast Cancer by Targeting EZH2. Int J Oncol (2018) 53(4):1505–15. doi: 10.3892/ijo.2018.4486
73. Yi H, Liang B, Jia J, Liang N, Xu H, Ju G, et al. Differential Roles of miR-199a-5p in Radiation-Induced Autophagy in Breast Cancer Cells. FEBS Lett (2013) 587(5):436–43. doi: 10.1016/j.febslet.2012.12.027
74. Ramos FS, Wons L, Cavalli IJ, Ribeiro E. Epithelial-Mesenchymal Transition in Cancer: An Overview. Integr Cancer Sci Ther (2017) 4:1–5.
75. Ma F, Li W, Liu C, Li W, Yu H, Lei B, et al. MiR-23a Promotes TGF-β1-Induced EMT and Tumor Metastasis in Breast Cancer Cells by Directly Targeting CDH1 and Activating Wnt/β-Catenin Signaling. Oncotarget (2017) 8(41):69538. doi: 10.18632/oncotarget.18422
76. Jiang G, Shi W, Fang H, Zhang X. Mir−27a Promotes Human Breast Cancer Cell Migration by Inducing EMT in a FBXW7−dependent Manner. Mol Med Rep (2018) 18(6):5417–26. doi: 10.3892/mmr.2018.9587
77. Pei Y-F, Lei Y, Liu X-Q. MiR-29a Promotes Cell Proliferation and EMT in Breast Cancer by Targeting Ten Eleven Translocation 1. Biochim Biophys Acta (BBA) Molecular Basis Dis (2016) 1862(11):2177–85. doi: 10.1016/j.bbadis.2016.08.014
78. Han M, Wang Y, Guo G, Li L, Dou D, Ge X, et al. MicroRNA-30d Mediated Breast Cancer Invasion, Migration, and EMT by Targeting KLF11 and Activating STAT3 Pathway. J Cell Biochem (2018) 119(10):8138–45. doi: 10.1002/jcb.26767
79. Imani S, Wei C, Cheng J, Khan MA, Fu S, Yang L, et al. MicroRNA-34a Targets Epithelial to Mesenchymal Transition-Inducing Transcription Factors (EMT-TFs) and Inhibits Breast Cancer Cell Migration and Invasion. Oncotarget (2017) 8(13):21362. doi: 10.18632/oncotarget.15214
80. Ippen FM, Alvarez-Breckenridge CA, Kuter BM, Fink AL, Bihun IV, Lastrapes M, et al. The Dual PI3K/mTOR Pathway Inhibitor GDC-0084 Achieves Antitumor Activity in PIK3CA-Mutant Breast Cancer Brain Metastases. (2019) 25: (11):3374–83. doi: 10.1158/1078-0432.CCR-18-3049
81. Chu S, Liu G, Xia P, Chen G, Shi F, Yi T, et al. miR-93 and PTEN: Key Regulators of Doxorubicin-Resistance and EMT in Breast Cancer. Oncol Rep (2017) 38(4):2401–7. doi: 10.3892/or.2017.5859
82. Xiang Y, Liao X-H, Yu C-X, Yao A, Qin H, Li J-P, et al. MiR-93-5p Inhibits the EMT of Breast Cancer Cells via Targeting MKL-1 and STAT3. Exp Cell Res (2017) 357(1):135–44. doi: 10.1016/j.yexcr.2017.05.007
83. Zhang L, Chen T, Yan L, Xu H, Wang Y, Li Y, et al. MiR-155-3p Acts as a Tumor Suppressor and Reverses Paclitaxel Resistance via Negative Regulation of MYD88 in Human Breast Cancer. Gene (2019) 700:85–95. doi: 10.1016/j.gene.2019.02.066
84. Nie J, Jiang H-C, Zhou Y-C, Jiang B, He W-J, Wang Y-F, et al. MiR-125b Regulates the Proliferation and Metastasis of Triple Negative Breast Cancer Cells via the Wnt/β-Catenin Pathway and EMT. Biosci Biotechnol Biochem (2019) 83(6):1062–71. doi: 10.1080/09168451.2019.1584521
85. Shi D, Li Y, Fan L, Zhao Q, Tan B, Cui G. Upregulation Of miR-153 Inhibits Triple-Negative Breast Cancer Progression by Targeting ZEB2-Mediated EMT and Contributes to Better Prognosis. OncoTargets Ther (2019) 12:9611. doi: 10.2147/OTT.S223598
86. Makii C, Ikeda Y, Oda K, Uehara Y, Nishijima A, Koso T, et al. Anti-Tumor Activity of Dual Inhibition of Phosphatidylinositol 3-Kinase and MDM2 Against Clear Cell Ovarian Carcinoma. Gynecol Oncol (2019) 155(2):331–9. doi: 10.1016/j.ygyno.2019.08.028
87. Huang R, Li J, Pan F, Zhang B, Yao Y. The Activation of GPER Inhibits Cells Proliferation, Invasion and EMT of Triple-Negative Breast Cancer via CD151/miR-199a-3p Bio-Axis. Am J Trans Res (2020) 12(1):32.
88. Anastasov N, Hirmer E, Klenner M, Ott J, Falkenberg N, Bao X, et al. MEK1 Inhibitor Combined With Irradiation Reduces Migration of Breast Cancer Cells Including miR-221 and ZEB1 EMT Marker Expression. Cancers (2020) 12(12):37600. doi: 10.3390/cancers12123760
89. Gao F, Tian J. FOXK1, Regulated by miR-365-3p, Promotes Cell Growth and EMT Indicates Unfavorable Prognosis in Breast Cancer. OncoTargets Ther (2020) 13:623. doi: 10.2147/OTT.S212702
90. Chi Y, Wang F, Zhang T, Xu H, Zhang Y, Shan Z, et al. miR-516a-3p Inhibits Breast Cancer Cell Growth and EMT by Blocking the Pygo2/Wnt Signalling Pathway. J Cell Mol Med (2019) 23(9):6295–307. doi: 10.1111/jcmm.14515
91. Tang C-P, Zhou H-J, Qin J, Luo Y, Zhang T. MicroRNA-520c-3p Negatively Regulates EMT by Targeting IL-8 to Suppress the Invasion and Migration of Breast Cancer. Oncol Rep (2017) 38(5):3144–52. doi: 10.3892/or.2017.5968
92. Jin T, Zhang Y, Zhang T. MiR-524-5p Suppresses Migration, Invasion, and EMT Progression in Breast Cancer Cells Through Targeting FSTL1. Cancer Biother Radiopharm (2020) 35(10):789–801. doi: 10.1089/cbr.2019.3046
93. Liu C, Min L, Kuang J, Zhu C, Qiu X-Y, Zhu L. Bioinformatic Identification of miR-622 Key Target Genes and Experimental Validation of the miR-622-RNF8 Axis in Breast Cancer. Front Oncol (2019) 9:1114–. doi: 10.3389/fonc.2019.01114
94. Liu G, Wang P, Zhang H. MiR-6838-5p Suppresses Cell Metastasis and the EMT Process in Triple-Negative Breast Cancer by Targeting WNT3A to Inhibit the Wnt Pathway. J Gene Med (2019) 21(12):e3129. doi: 10.1002/jgm.3129
95. Sánchez-Tilló E, Liu Y, de Barrios O, Siles L, Fanlo L, Cuatrecasas M, et al. EMT-Activating Transcription Factors in Cancer: Beyond EMT and Tumor Invasiveness. Cell Mol Life Sci (2012) 69(20):3429–56. doi: 10.1007/s00018-012-1122-2
96. Huber MA, Kraut N, Beug H. Molecular Requirements for Epithelial–Mesenchymal Transition During Tumor Progression. Curr Opin Cell Biol (2005) 17(5):548–58. doi: 10.1016/j.ceb.2005.08.001
97. Huang Q, Wu Y, Xing S, Yu Z. Effect of miR-7 on Resistance of Breast Cancer Cells to Adriamycin via Regulating EGFR/PI3K Signaling Pathway. Eur Rev Med Pharmacol Sci (2019) 23(12):5285–92. doi: 10.26355/eurrev_201906_18195
98. Lin S, Yu L, Song X, Bi J, Jiang L, Wang Y, et al. Intrinsic Adriamycin Resistance in P53-Mutated Breast Cancer is Related to the miR-30c/FANCF/REV1-Mediated DNA Damage Response. Cell Death Dis (2019) 10(9):1–15. doi: 10.1038/s41419-019-1871-z
99. Hong T, Ding J, Li W. Mir-7 Reverses Breast Cancer Resistance to Chemotherapy by Targeting Mrp1 and Bcl2. OncoTargets Ther (2019) 12:11097. doi: 10.2147/OTT.S213780
100. Jin J, Sun Z, Yang F, Tang L, Chen W, Guan X. miR-19b-3p Inhibits Breast Cancer Cell Proliferation and Reverses Saracatinib-Resistance by Regulating PI3K/Akt Pathway. Arch Biochem Biophys (2018) 645:54–60. doi: 10.1016/j.abb.2018.03.015
101. Gong L, Shi J, Shang J, Hao J, Du X. Effect of miR-34a on Resistance to Sunitinib in Breast Cancer by Regulating the Wnt/β-Catenin Signaling Pathway. Eur Rev Med Pharmacol Sci (2019) 23(3):1151–7. doi: 10.26355/eurrev_201902_17006
102. Han X, Li Q, Liu C, Wang C, Li Y. Overexpression miR-24-3p Repressed Bim Expression to Confer Tamoxifen Resistance in Breast Cancer. J Cell Biochem (2019) 120(8):12966–76. doi: 10.1002/jcb.28568
103. Fu H, Fu L, Xie C, Zuo W-S, Liu Y-S, Zheng M-Z, et al. miR-375 Inhibits Cancer Stem Cell Phenotype and Tamoxifen Resistance by Degrading HOXB3 in Human ER-Positive Breast Cancer. Oncol Rep (2017) 37(2):1093–9. doi: 10.3892/or.2017.5360
104. Muluhngwi P, Krishna A, Vittitow SL, Napier JT, Richardson KM, Ellis M, et al. Tamoxifen Differentially Regulates miR-29b-1 and miR-29a Expression Depending on Endocrine-Sensitivity in Breast Cancer Cells. Cancer Lett (2017) 388:230–8. doi: 10.1016/j.canlet.2016.12.007
105. Tormo E, Adam-Artigues A, Ballester S, Pineda B, Zazo S, González-Alonso P, et al. The Role of miR-26a and miR-30b in HER2+ Breast Cancer Trastuzumab Resistance and Regulation of the CCNE2 Gene. Sci Rep (2017) 7(1):1–9. doi: 10.1038/srep41309
106. Wu M-Y, Fu J, Xiao X, Wu J, Wu R-C. MiR-34a Regulates Therapy Resistance by Targeting HDAC1 and HDAC7 in Breast Cancer. Cancer Lett (2014) 354(2):311–9. doi: 10.1016/j.canlet.2014.08.031
107. Zhang W, Jiang H, Chen Y, Ren F. Resveratrol Chemosensitizes Adriamycin-Resistant Breast Cancer Cells by Modulating miR-122-5p. J Cell Biochem (2019) 120(9):16283–92. doi: 10.1002/jcb.28910
108. Hou L, Zhao Y, Song G-q, Ma Y-h, Jin X-h, Jin S-l, et al. Interfering Cellular Lactate Homeostasis Overcomes Taxol Resistance of Breast Cancer Cells Through the microRNA-124-Mediated Lactate Transporter (MCT1) Inhibition. Cancer Cell Int (2019) 19(1):1–12. doi: 10.1186/s12935-019-0904-0
109. Zhang Y, Wang Y, Wei Y, Li M, Yu S, Ye M, et al. MiR-129-3p Promotes Docetaxel Resistance of Breast Cancer Cells via CP110 Inhibition. Sci Rep (2015) 5(1):1–12. doi: 10.1038/srep15424
110. Du F, Yu L, Wu Y, Wang S, Yao J, Zheng X, et al. miR-137 Alleviates Doxorubicin Resistance in Breast Cancer Through Inhibition of Epithelial-Mesenchymal Transition by Targeting DUSP4. Cell Death Dis (2019) 10(12):1–10. doi: 10.1038/s41419-019-2164-2
111. Zang H, Li Y, Zhang X, Huang G. Circ-RNF111 Contributes to Paclitaxel Resistance in Breast Cancer by Elevating E2F3 Expression via miR-140-5p. Thorac Cancer (2020) 11(7):1891–903. doi: 10.1111/1759-7714.13475
112. Gao Y, Zhang W, Liu C, Li G. miR-200 Affects Tamoxifen Resistance in Breast Cancer Cells Through Regulation of MYB. Sci Rep (2019) 9(1):1–6. doi: 10.1038/s41598-019-54289-6
113. Bai WD, Ye XM, Zhang MY, Zhu HY, Xi WJ, Huang X, et al. MiR-200c Suppresses TGF-β Signaling and Counteracts Trastuzumab Resistance and Metastasis by Targeting ZNF217 and ZEB1 in Breast Cancer. Int J Cancer (2014) 135(6):1356–68. doi: 10.1002/ijc.28782
114. Dai H, Xu LY, Qian Q, Zhu QW, Chen W-X. MicroRNA-222 Promotes Drug Resistance to Doxorubicin in Breast Cancer via Regulation of miR-222/Bim Pathway. Biosci Rep (2019) 39(7):BSR20190650. doi: 10.1042/BSR20190650
115. Liang Z, Wu H, Xia J, Li Y, Zhang Y, Huang K, et al. Involvement of miR-326 in Chemotherapy Resistance of Breast Cancer Through Modulating Expression of Multidrug Resistance-Associated Protein 1. Biochem Pharmacol (2010) 79(6):817–24. doi: 10.1016/j.bcp.2009.10.017
116. Yi D, Xu L, Wang R, Lu X, Sang J. miR-381 Overcomes Cisplatin Resistance in Breast Cancer by Targeting MDR1. Cell Biol Int (2019) 43(1):12–21. doi: 10.1002/cbin.11071
117. Dou D, Ge X, Wang X, Xu X, Zhang Z, Seng J, et al. EZH2 Contributes to Cisplatin Resistance in Breast Cancer by Epigenetically Suppressing miR-381 Expression. OncoTargets Ther (2019) 12:9627. doi: 10.2147/OTT.S214104
118. Xia W, Liu Y, Du Y, Cheng T, Hu X, Li X. MicroRNA-423 Drug Resistance and Proliferation of Breast Cancer Cells by Targeting ZFP36. OncoTargets Ther (2020) 13:769. doi: 10.2147/OTT.S217745
119. Jiang L, He D, Yang D, Chen Z, Pan Q, Mao A, et al. MiR-489 Regulates Chemoresistance in Breast Cancer via Epithelial Mesenchymal Transition Pathway. FEBS Lett (2014) 588(11):2009–15. doi: 10.1016/j.febslet.2014.04.024
120. Geng W, Song H, Zhao Q, Dong K, Pu Q, Gao H, et al. Mir-520h Stimulates Drug Resistance to Paclitaxel by Targeting the Otud3-Pten Axis in Breast Cancer. BioMed Res Int (2020) 2020. doi: 10.1155/2020/9512793
121. Han M, Hu J, Lu P, Cao H, Yu C, Li X, et al. Exosome-Transmitted miR-567 Reverses Trastuzumab Resistance by Inhibiting ATG5 in Breast Cancer. Cell Death Dis (2020) 11(1):1–15. doi: 10.1038/s41419-020-2250-5
122. Wang G, Dong Y, Liu H, Ji N, Cao J, Liu A, et al. Loss of Mir−873 Contributes to Gemcitabine Resistance in Triple−Negative Breast Cancer via Targeting ZEB1. Oncol Lett (2019) 18(4):3837–44. doi: 10.3892/ol.2019.10697
123. Li XJ, Ren ZJ, Tang JH, Yu Q. Exosomal MicroRNA MiR-1246 Promotes Cell Proliferation, Invasion and Drug Resistance by Targeting CCNG2 in Breast Cancer. Cell Physiol Biochem (2017) 44(5):1741–8. doi: 10.1159/000485780
124. Patel N, Garikapati KR, Pandita RK, Singh DK, Pandita TK, Bhadra U, et al. miR-15a/miR-16 Down-Regulates BMI1, Impacting Ub-H2A Mediated DNA Repair and Breast Cancer Cell Sensitivity to Doxorubicin. Sci Rep (2017) 7(1):1–17. doi: 10.1038/s41598-017-02800-2
125. Wolfe AR, Bambhroliya A, Reddy JP, Debeb BG, Huo L, Larson R, et al. MiR-33a Decreases High-Density Lipoprotein-Induced Radiation Sensitivity in Breast Cancer. Int J Radiat Oncol Biol Phys (2016) 95(2):791–9. doi: 10.1016/j.ijrobp.2016.01.025
126. Luo Y, Hua T, You X, Lou J, Yang X, Tang N. Effects of MiR-107 on the Chemo-Drug Sensitivity of Breast Cancer Cells. Open Med (2019) 14(1):59–65. doi: 10.1515/med-2019-0009
127. Ma C, Shi X, Guo W, Niu J, Wang G. miR-107 Enhances the Sensitivity of Breast Cancer Cells to Paclitaxel. Open Med (2019) 14(1):456–66. doi: 10.1515/med-2019-0049
128. Xu X, Lv Y-g, Yan C-y, Yi J, Ling R. Enforced Expression of hsa-miR-125a-3p in Breast Cancer Cells Potentiates Docetaxel Sensitivity via Modulation of BRCA1 Signaling. Biochem Biophys Res Commun (2016) 479(4):893–900. doi: 10.1016/j.bbrc.2016.09.087
129. Zhang Y, Xia F, Zhang F, Cui Y, Wang Q, Liu H, et al. miR-135b-5p Enhances Doxorubicin-Sensitivity of Breast Cancer Cells Through Targeting Anterior Gradient 2. J Exp Clin Cancer Res (2019) 38(1):1–13. doi: 10.1186/s13046-019-1024-3
130. Ninio-Many L, Hikri E, Burg Golani T, Stemmer SM, Shalgi R, Ben-Aharon I. miR-125a Induces HER2 Expression and Sensitivity to Trastuzumab in Triple Negative Breast Cancer Lines. Front Oncol (2020) 10:191. doi: 10.3389/fonc.2020.00191
131. Yu L, Yang Y, Hou J, Zhai C, Song Y, Zhang Z, et al. MicroRNA-144 Affects Radiotherapy Sensitivity by Promoting Proliferation, Migration and Invasion of Breast Cancer Cells. Oncol Rep (2015) 34(4):1845–52. doi: 10.3892/or.2015.4173
132. Jiao X, Zhao L, Ma M, Bai X, He M, Yan Y, et al. MiR-181a Enhances Drug Sensitivity in Mitoxantone-Resistant Breast Cancer Cells by Targeting Breast Cancer Resistance Protein (BCRP/Abcg2). Breast Cancer Res Treat (2013) 139(3):717–30. doi: 10.1007/s10549-013-2607-x
133. Zeng C, Fan D, Xu Y, Li X, Yuan J, Yang Q, et al. Curcumol Enhances the Sensitivity of Doxorubicin in Triple-Negative Breast Cancer via Regulating the miR-181b-2-3p-ABCC3 Axis. Biochem Pharmacol (2020) 174:113795. doi: 10.1016/j.bcp.2020.113795
134. Wu Y, Tao L, Liang J, Qiao Y, Liu W, Yu H, et al. Mir−187−3p Increases Gemcitabine Sensitivity in Breast Cancer Cells by Targeting FGF9 Expression. Exp Ther Med (2020) 20(2):952–60. doi: 10.3892/etm.2020.8770
135. Yu Y, Yin W, Yu Z-H, Zhou Y-J, Chi J-R, Ge J, et al. miR-190 Enhances Endocrine Therapy Sensitivity by Regulating SOX9 Expression in Breast Cancer. J Exp Clin Cancer Res (2019) 38(1):1–13. doi: 10.1186/s13046-019-1039-9
136. Yang G, Wu D, Zhu J, Jiang O, Shi Q, Tian J, et al. Upregulation of miR-195 Increases the Sensitivity of Breast Cancer Cells to Adriamycin Treatment Through Inhibition of Raf-1. Oncol Rep (2013) 30(2):877–89. doi: 10.3892/or.2013.2532
137. Ma C, Shi X, Guo W, Feng F, Wang G. Mir−205−5p Downregulation Decreases Gemcitabine Sensitivity of Breast Cancer Cells via ERp29 Upregulation. Exp Ther Med (2019) 18(5):3525–33. doi: 10.1515/med-2019-0049
138. Wang Y, Zhao L, Xiao Q, Jiang L, He M, Bai X, et al. miR-302a/B/C/D Cooperatively Inhibit BCRP Expression to Increase Drug Sensitivity in Breast Cancer Cells. Gynecol Oncol (2016) 141(3):592–601. doi: 10.1016/j.ygyno.2015.11.034
139. Cataldo A, Cheung DG, Balsari A, Tagliabue E, Coppola V, Iorio MV, et al. miR-302b Enhances Breast Cancer Cell Sensitivity to Cisplatin by Regulating E2F1 and the Cellular DNA Damage Response. Oncotarget (2016) 7(1):786. doi: 10.18632/oncotarget.6381
140. Ikeda K, Horie-Inoue K, Ueno T, Suzuki T, Sato W, Shigekawa T, et al. miR-378a-3p Modulates Tamoxifen Sensitivity in Breast Cancer MCF-7 Cells Through Targeting GOLT1A. Sci Rep (2015) 5(1):1–12. doi: 10.1038/srep13170
141. Mi H, Wang X, Wang F, Li L, Zhu M, Wang N, et al. miR-381 Induces Sensitivity of Breast Cancer Cells to Doxorubicin by Inactivation of MAPK Signaling via FYN. Eur J Pharmacol (2018) 839:66–75. doi: 10.1016/j.ejphar.2018.09.024
142. Zhao G, Li Y, Wang T. Potentiation of Docetaxel Sensitivity by miR-638 via Regulation of STARD10 Pathway in Human Breast Cancer Cells. Biochem Biophys Res Commun (2017) 487(2):255–61. doi: 10.1016/j.bbrc.2017.04.045
143. Tan X, Peng J, Fu Y, An S, Rezaei K, Tabbara S, et al. miR-638 Mediated Regulation of BRCA1 Affects DNA Repair and Sensitivity to UV and Cisplatin in Triple-Negative Breast Cancer. Breast Cancer Res (2014) 16(5):1–14. doi: 10.1186/s13058-014-0435-5
144. Hou X, Niu Z, Liu L, Guo Q, Li H, Yang X, et al. Mir−1207−5p Regulates the Sensitivity of Triple−Negative Breast Cancer Cells to Taxol Treatment via the Suppression of LZTS1 Expression. Oncol Lett (2019) 17(1):990–8. doi: 10.3892/ol.2018.9687
145. Shimono Y, Zabala M, Cho RW, Lobo N, Dalerba P, Qian D, et al. Downregulation of miRNA-200c Links Breast Cancer Stem Cells With Normal Stem Cells. cell (2009) 138(3):592–603. doi: 10.1016/j.cell.2009.07.011
146. Singh R, Mo Y-Y. Role of microRNAs in Breast Cancer. Cancer Biol Ther (2013) 14(3):201–12. doi: 10.4161/cbt.23296
147. Simonson B, Das S. MicroRNA Therapeutics: The Next Magic Bullet? Mini Rev Medicinal Chem (2015) 15(6):467–74. doi: 10.2174/1389557515666150324123208
148. Van Rooij E, Kauppinen S. Development of Micro RNA Therapeutics is Coming of Age. EMBO Mol Med (2014) 6(7):851–64. doi: 10.15252/emmm.201100899
Keywords: miRNA, microRNA, breast cancer, apoptosis, biomarker
Citation: Ghafouri-Fard S, Khanbabapour Sasi A, Abak A, Shoorei H, Khoshkar A and Taheri M (2021) Contribution of miRNAs in the Pathogenesis of Breast Cancer. Front. Oncol. 11:768949. doi: 10.3389/fonc.2021.768949
Received: 01 September 2021; Accepted: 18 October 2021;
Published: 05 November 2021.
Edited by:
Pasquale Simeone, University of Studies G. d’Annunzio Chieti and Pescara, ItalyReviewed by:
Javier Gaytan, IMSS, MexicoGisela Ceballos, Instituto Nacional de Medicina Genómica (INMEGEN), Mexico
Copyright © 2021 Ghafouri-Fard, Khanbabapour Sasi, Abak, Shoorei, Khoshkar and Taheri. This is an open-access article distributed under the terms of the Creative Commons Attribution License (CC BY). The use, distribution or reproduction in other forums is permitted, provided the original author(s) and the copyright owner(s) are credited and that the original publication in this journal is cited, in accordance with accepted academic practice. No use, distribution or reproduction is permitted which does not comply with these terms.
*Correspondence: Mohammad Taheri, TW9oYW1tYWRfODIzQHlhaG9vLmNvbQ==