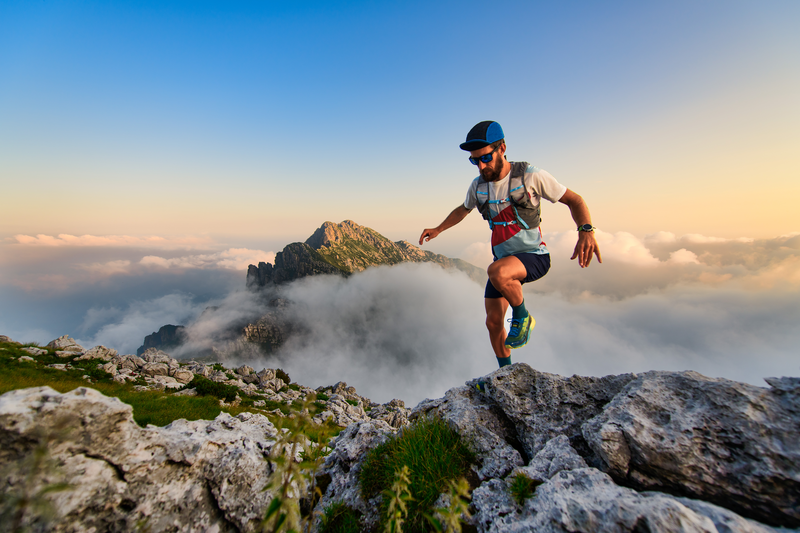
95% of researchers rate our articles as excellent or good
Learn more about the work of our research integrity team to safeguard the quality of each article we publish.
Find out more
ORIGINAL RESEARCH article
Front. Oncol. , 13 January 2022
Sec. Breast Cancer
Volume 11 - 2021 | https://doi.org/10.3389/fonc.2021.755267
This article is part of the Research Topic Insights in Breast Cancer: 2021 View all 13 articles
NAA25 gene variants were reported as risk factors for type 1 diabetes, rheumatoid arthritis and acute arterial stroke. But it’s unknown whether it could contribute to breast cancer. We identified rs11066150 in lncHSAT164, which contributes to breast cancer, in our earlier genome-wide long non-coding RNA association study on Han Chinese women. However, rs11066150 A/G variant is also located in NAA25 intron. Based on the public database, such as TCGA and Curtis dataset, NAA25 gene is highly expressed in breast cancer tissues and this result has also been proved in our samples and cell lines through RT-qPCR and western blot analysis. To better understand the function of NAA25 in breast cancer, we knocked down the expression of NAA25 in breast cancer cell lines, FACS was used to detect cell apoptosis and cell cycle and colony formation assay was used to detect cell proliferation. We found that NAA25-deficient cells could increase cell apoptosis, delay G2/M phase cell and decrease cell clone formation. RNA sequencing was then applied to analyze the molecular profiles of NAA25−deficient cells, and compared to the control group, NAA25 knockdown could activate apoptosis-related pathways, reduce the activation of tumor-associated signaling pathways and decrease immune response-associated pathways. Additionally, RT-qPCR was employed to validate these results. Taken together, our results revealed that NAA25 was highly expressed in breast cancer, and NAA25 knockdown might serve as a therapeutic target in breast cancer.
Breast cancer is the most common and a leading cause of cancer-related deaths of women worldwide (1). And China is undergoing the cancer transition stage, with the occurrence of female breast cancer increasing rapidly (2, 3). With the development of sequencing technologies, a lot of breast cancer associated genes have been validated (4, 5). Our previous case-control genome wide lncRNA association study on Han Chinese women identified that SNP rs11066150 was associated with breast cancer and lncHSAT164 gene could contribute to breast cancer (6). And rs11066150 A/G was an intron variant in N-alpha-acetyltransferase 25 (NAA25) gene (also known as MDM20, C12orf30 and NAP1). NAA25 gene variants were reported to be associated with type 1 diabetes (T1D), rheumatoid arthritis, acute arterial stroke and dyslipidemia (7–10). However, the relationship between NAA25 and breast cancer is still unknown.
NAA25 encodes the auxiliary subunit, which could then affect posttranslational modifications by forming N-terminal acetyltransferase B complex with catalytic subunit NAA20 (11). In yeast, it can regulate actin remodeling, and stabilize actin cytoskeleton and mitochondrial targeting (12, 13). And NAA25 knockdown can disrupt cell cycle and reduce cell growth (14). However, the physiological function and mechanism of NAA25 in breast cancer remain unknown.
To explore the relationship between NAA25 gene and breast cancer, we compared NAA25 gene expression between normal tissues and breast cancer tissues in public databases, such as TCGA and Curtis dataset, and analyzed the relationship between NAA25 gene expression and overall survival (OS) of patients. In addition, we tested NAA25 gene expression in breast cancer tissues, para-carcinoma tissues, breast cancer cell lines and normal breast epithelial cell lines. Furthermore, we specifically knocked down NAA25 gene expression in breast cancer cells and explored its influence on tumor cell proliferation, apoptosis and cell cycle. Finally, RNA-seq analysis was used to clarify the molecular profiles of NAA25-deficient cells.
In this study, four-pairs of breast cancer tissues and para-carcinoma tissues (all from Han Chinese women) were collected at the No.2 Hospital, Anhui Medical University. All cases were diagnosed with breast cancer by at least two pathologists. Para-carcinoma specimens were adipose/skin tissues, which were collected from breast cancer patients who underwent radical mastectomy. All tissue samples were stored in liquid nitrogen immediately after surgical resection. The information of breast cancer patients was provided in Supplementary Table 1.
MCF10A, MCF7, T47D, and HEK293T cell lines were purchased from the Institute of Basic Medical Sciences of the Chinese Academy of Medical Sciences. MCF10A, a kind of normal human breast epithelial cell, was grown in DMEM/F12 (Gibco, Life, China) medium supplemented with 10% fetal bovine serum (FBS) (Gibco, Australia), 10 µg/ml insulin (Macklin, China), 20 ng/ml EGF (Peprotech, China), and 0.5 µg/ml hydrocortisone (Macklin, China). MCF7 and HEK293T cells were maintained in DMEM (Gibco, Life, USA) supplemented with 10% FBS (Gibco, Australia). T47D cells were maintained in RPMI-1640 medium (Gibco, Life, USA) supplemented with 10% FBS (Gibco, Australia). All medium were supplemented with 100 U/ml penicillin–streptomycin (Gibco, Life, China), and all cells were maintained at 37°C in a humidified atmosphere containing 5% CO2 and confirmed to be mycoplasma free.
The total RNA from the cell lines, human breast cancer tissues and para-cancerous tissues used in this study was extracted with TRIzol reagent, and DNase I (Thermo Fisher, USA) was used to remove genomic DNA. First-strand cDNA was synthesized by using the SuperScript III Reverse Transcriptase Kit (Thermo Fisher, USA). Relative RNA levels determined by RT-qPCR were measured on a Rotor-Gene Q real-time PCR machine (Qiagen, Germany). GAPDH was employed as an internal control. The relative expression of RNAs was calculated using the 2−ΔΔCt method. All primer sequences for RT-qPCR are listed in Supplementary Table 2.
Short hairpin RNAs (shRNAs) against NAA25 sh1 and sh2 were designed and synthesized by Taihe Biotechnology (Beijing, China) and cloned into the EGFP-Puro-pll3.7 plasmid. Based on the PSPAX2-PMD2G lentiviral system, a lentivirus was constructed according to the manufacturer’s instructions. After lentivirus infection, 1 µg/ml puromycin (In vivoGen, USA) was added for selection, and 48-72 hours later, the cells were harvested for further experiments. shRNA sequences are listed in Supplementary Table 2.
Tissues and cells were lysed in RIPA buffer (Beyotime, China). 40 μg of protein was used for SDS-PAGE gel electrophoresis (Bio-Rad) and transferred onto PVDF membranes (Millipore, China). Blocking was performed with 5% milk, and then the membranes were incubated with primary antibodies. Anti-NAA25 (1:1, 000 HPA039322, Sigma-Aldrich) or anti-actin (1:5000, A1978, Sigma-Aldrich) was added and incubated overnight at 4°C. After being washed, the membranes were incubated with secondary antibodies (peroxidase conjugated, suitable for each primary antibody) for 2 hours at room temperature. The signal was detected with a Bio-Rad ChemiDoc XRS + System after adding Super Signal West Pico chemiluminescence.
To analyze cell growth, colony formation assays were performed. 1×103 cells of T47D- and MCF7- Ctr, -sh1, -sh2 were seeded in a 6-well plate and incubated for 10 to 15 days at 37°C. Then, the cells were washed twice in PBS, fixed with 90% ethanol for 15 minutes and stained with 0.1% crystal violet for 20 minutes. Images of colonies were taken with a digital camera, and the number of colonies was analyzed by ImageJ v1.8.0 software.
For apoptosis analysis, target cells were transferred to a 15 ml centrifuge tube, and annexin V binding buffer was added. After being centrifugated at 1,000 rpm for 5 min at 4°C, the cells were washed 3 times in PBS. Then, the cells were treated with 100 μl of binding buffer, 5 μl of Annexin V-APC and 1 μl of 100 μg/ml propidium iodide (PI) stain (Thermo Fisher, USA), and incubated in the dark for 25 min. Cell apoptosis was analyzed by flow cytometry (BD Biosciences).
For cell cycle analysis, target cells were fixed with 75% ice-cold ethanol at 4°C overnight. Then, the cells were suspended in PBS supplemented with 100 mg/ml RNase A for 30 min at 37°C and then stained with 50 µg/ml PI (Thermo Fisher, USA) in the dark at room temperature for 15 min. Finally, a total of 20,000 cells were analyzed on a FACS Calibur flow cytometer equipped with Cell Quest software (BD Biosciences).
After NAA25 knockdown in T47D cells, cells from the Ctr, sh1 and sh2 groups were harvested for RNA-seq analysis at Shanghai Majorbio Biopharm Technology Co. mRNAs were isolated from total RNA with the oligo (dT) method. The mRNAs were fragmented, and then first-strand cDNA and second-strand cDNA were synthesized. After being purified, cDNA fragments were linked to adapters. Then, cDNA fragments of suitable size were selected for PCR amplification. The sequencing platform used in this study was Illumina HiSeq, and the paired-end reads were 2×150 bp. TPM (Transcripts Per Million reads) was used to evaluate genes expression, transcript abundance was assessed with the DESeq2, and the significantly affected genes were determined by setting a fold change of ≥ 2. The differentially expressed gene (DEG), Kyoto Encyclopedia of Genes and Genomes (KEGG) pathway, gene ontology (GO), GO term and gene set enrichment analysis (GSEA) described in this paper were performed on the free online platform Majorbio Cloud Platform (www.majorbio.com).
All statistical analyses were performed using Graphpad Prism 8.0 statistical software (California, US). Experiment data are shown as the means ± SEM, and all experiments were conducted for at least three times. Significance was determined using the Student’s t-test: N.S. p > 0.05; ∗p < 0.05; ∗∗p < 0.01; ∗∗∗p < 0.001 and ∗∗∗∗p < 0.0001.
rs11066150 was reported in lncHSAT164 (6), and it is also located within the fifth intron of NAA25 gene (Figure 1A). Based on the eQTLGen database (https://www.eqtlgen.org/), we identified 4 cis-eQTL effects genes, TMEM116, HECTD4, MAPKAPK5 and NAA25, to be associated with rs11066150 (Supplementary Table 3). And TMEM116, HECTD4, MAPKAPK5 was reported to be associated with renal cell carcinoma, prostate cancer and colorectal cancer (15–17). However, NAA25 gene has never been reported to be associated with cancers.
Figure 1 Characterization of NAA25 gene in breast cancer. (A) rs11066150 variant schematic diagram in lncHSAT164 and NAA25. (B, C) NAA25 gene was highly expressed in breast cancer tissues compared to the controls in TCGA dataset and Curtis dataset. (D) OS analysis of patients with high and low NAA25 expression. The p value was calculated using Mann-Whitney U tests. (E) Western-blot and RT-qPCR to analyze NAA25 expression in breast cancer tissues and para-carcinoma tissues, NAA25 was highly expressed in breast cancer tissues. The two bands are all NAA25. *p < 0.05.
To explore the role of NAA25 gene in breast cancer, we analyzed its expression in different public databases. According to TCGA and the Curtis, Finak breast and Richardson breast datasets (18–21), we found that NAA25 was greatly up regulated in breast cancer tissues in comparison with normal breast tissues (Figures 1B, C and Supplementary Figures 1A, B). Furthermore, high mRNA levels of NAA25 showed marginal associations with poor OS in the Curtis database (p = 0.013) (Figure 1D). Additionally, we explored the expression of NAA25 gene in breast cancer tissues and para-cancerous tissues. RT-qPCR and western blot analyses were performed in four-pairs of tissues, and results revealed that NAA25 was highly expressed in cancer tissues (Figure 1E). We also monitored NAA25 expression in normal breast epithelial cell line MCF10A, and breast cancer cell lines MCF7 and T47D. Compared to MCF10A, NAA25 was highly expressed in T47D cells (Figure 2A). Together, our analyses reveal a previously unknown role of NAA25 in breast cancer, and highly expressed NAA25 might influence the progress of breast cancer.
Figure 2 NAA25 gene influences cell apoptosis and the cell cycle in breast cancer. (A) RT-qPCR and western blot analysis of NAA25 gene expression in breast cancer cell lines (The two bands are all NAA25.). (B) RT-qPCR and western blot analysis in the NAA25-deficient T47D cell line. (C) Cell apoptosis in the NAA25-deficient T47D cells. Compared to the Ctr group, NAA25 knockdown could increase cell apoptosis. (D) Cell cycle analysis of the NAA25-deficient T47D cells. Compared to the Ctr group, NAA25 knockdown induced G2/M cell cycle arrest. (E, F) Downregulated NAA25 reduced the clonogenic potential of breast cancer cells. N.S. p > 0.05; *p < 0.05; **p < 0.01; ***p < 0.001 and ****p < 0.0001.
To investigate the physiological roles of NAA25 gene in breast cancer, two shRNA targets were designed to knockdown NAA25 gene in breast cancer cell lines, and the mRNA expression and protein expression of NAA25 were both significantly diminished (Figure 2B and Supplementary Figure 1C). Apoptosis is a key cellular process in breast cancer. We measured the effect of NAA25 on apoptosis and cell cycle by FACE analysis. Compared to the Ctr group, the number of apoptotic cells was relatively larger in the shRNA groups (p < 0.01), as shown in (Figure 2C), and more cells were arrested in the G2/M phase (p < 0.05), as shown in (Figure 2D).
To further investigate whether NAA25 knockdown could influence tumor growth, colony formation assays were applied in this study, which illustrated that clonogenic survival significantly decreased following NAA25 knockdown in T47D cell line (Figures 2E, F). And similar results were investigated in NAA25-deficient MCF7 cells. Hence, based on these results, we conclude that NAA25 is highly expressed in breast cancer and may lead to poor OS in patients by regulating tumor cell apoptosis and cell cycle.
To investigate the importance of NAA25 gene in breast cancer, RNA-seq analysis was applied after NAA25 knockdown in the T47D cell line. Pearson’s correlation analysis (PCA) was performed to cluster all samples (Supplementary Figure 2A). Based on the gene expression matrix, the Venn diagram was used to analyze the co-expressed and specifically expressed genes or transcripts among the Ctr group and shRNA groups (Figure 3A). Furthermore, differentially expressed gene (DEG) analysis was conducted to compare the Ctr group and the sh1 and sh2 groups respectively, and 119 DEGs were identified (Figures 3B, C, Supplementary Figure 2B). All DEGs were presented in Supplementary Table 4.
Figure 3 RNA-seq analysis in the NAA25-deficient T47D cells. (A) Venn diagram analysis of gene or transcript expression among the Ctr group and shRNA groups. (B) Differentially expressed gene (DEG) heatmap analysis. Blue indicates downregulated genes. Red indicates upregulated genes. (C) Volcano plot showing the DEG in the Ctr group and the sh1 group. (D) GO term analysis between the Ctr group and the sh1 group. (E) Gene set enrichment analysis (GSEA) to analyze DEG between the Ctr group and the sh1 group. (F) RT-qPCR analysis to validate DEGs after NAA25 knockdown in the T47D cells. The data shown here are representative of at least 3 independent experiments. N.S. p > 0.05; *p < 0.05; **p < 0.01 and ****p < 0.0001.
Furthermore, KEGG enrichment analyses were performed among the 119 DEGs, and most of them were related to infections, immune responses, cancers and immune diseases (Supplementary Figure 2C). GO term analysis was performed to NAA25-deficient cells, and the results showed that many genes were associated with infection and immunity (Figure 3D and Supplementary Figure 2D).
To assess the molecular pathways involved in NAA25-deficient T47D cells, we performed gene set enrichment analysis (GSEA). And NAA25 knockdown could increase apoptosis associated pathways, and reduce tumor associated pathways, like MYC, HIF1A, ERB2, MEK and TNF (Figure 3E and Supplementary Figure 2E). In addition, immune response associated pathways like IL4, TNF and LTE2 were reduced. Finally, RT-qPCR analysis was used to verify RNA-seq data (Figure 3F). IFIT2, IFIT3, IFIT27, IFITM1, NDRG1, PFKFB4, ZNF395, IFI6, FUT11 and OAS2 mRNA expression was upregulated after NAA25 knockdown, and HSPH1 gene expression was down regulated, consistent with the RNA-seq results.
A large number of breast cancer associated susceptibility SNPs and genes were identified and reported as a molecular marker in tumor incidence, metastasis, prognosis and treatment. Previously, we performed a genome-wide lncRNA association study in Han Chinese women and identified two new susceptibility SNPs, rs11066150 and rs12537 (6) (22). rs11066150 variant had no relationship with the clinical characteristics of breast cancer like family history, menopausal status, and molecular subtypes (22). However, rs11066150 associated lncRNA, lncHSAT164, was highly expressed in breast cancer, and overexpressed lncHSAT164 could promote colony formation and down-expressed lncHSAT164 could promote cell apoptosis and regulate cell cycle (6). In this study, we reported rs11066150 as an intron variant SNP in NAA25 gene. And NAA25 gene is highly expressed in breast cancer tissues relative to normal tissues, while high NAA25 expression is correlated with poor OS. And NAA25 knockdown could induce cell apoptosis, delay G2/M phase cell and decrease cell clone formation. NAA25 was reported to be associated with T1D (7, 23), arthritis (8, 24) and virus infection (25). However, NAA25 gene was reported as a proto-oncogene in breast cancer for the first time, and more research is needed in the future to characterize the impact of rs11066150 A/G variant on breast cancer, and the relationship between lncHSAT164 and NAA25 gene also needs further study.
RNA-seq is a ubiquitous tool in molecular biology that is shaping nearly every aspect of our understanding of genomic function (26). The molecular features of NAA25-deficient T47D cell lines were analyzed by RNA-seq in this work, and analysis results indicated that many infection and immune associated genes were highly expressed, which suggests that immune therapy may be an effective approach in treating NAA25-overexpressed breast cancer.
Highly expressed IFIT2 and NDRG1 could reduce tumor migration and metastasis (27–30). And HSPH1 was highly expressed in different tumors, such as colorectal cancer, B-cell lymphoma, melanoma and esophageal squamous cell carcinoma (31–34), while NAA25 knockdown could upregulate IFIT2 and NDRG1 expression and downregulate HSPH1 expression (Figure 3F). These findings suggest that NAA25 knockdown may also play a positive role in treating other cancers. As an important accessory subunit of the NatB enzymatic complex, NAA25 could work with the NAA20 catalytic subunit to promote enzymatic activity (26, 35), and NAA25 knockdown did not reduce NAA20 expression (12). It’s also verified in the current study.
In conclusion, in this study, we reported NAA25 as a candidate gene of rs11066150, which was highly expressed in breast cancer, and highly expressed NAA25 could reduce patient’s OS. In addition, NAA25 knockdown could induce cell apoptosis, delay G2/M phase cell and decrease cell clone formation. RNA-seq analysis was also applied to clarify the molecular profiling of NAA25-deficient cells, and NAA25 knockdown repressed tumor- and immune response-associated pathways. This study is among the first attempts to clarify the function of NAA25 in breast cancer, and these results have elucidated the mechanism of NAA25 in breast cancer and suggests that NAA25 may serve as a potential therapeutic target of breast cancer.
The datasets presented in this study can be found in online repositories. RNA-seq data presented in the study are deposited in the SRA repository, accession number PRJNA752396. Further inquiries can be directed to the corresponding authors.
This study was approved by the Ethics Committee of Anhui Medical University. The patients/participants provided their written informed consent to participate in this study.
JX, YC, and BZ conceived of the idea. JX, ZL, and GL performed the experiments. JX, XBZ, and XJZ analyzed the data. JX drafted the manuscript. All authors contributed to the article and approved the submitted version.
This work was supported by the scientific and technological innovation leading talents of “Ten Thousand Talents Program” (2018-WRJH-1), the National Natural Science Foundation of China (81872516), the discipline construction project of Peking Union Medical College (xhxk201903) and 2020 medical service and support capability upgrade project (2020-QTL-008).
The authors declare that the research was conducted in the absence of any commercial or financial relationships that could be construed as a potential conflict of interest.
The reviewer, H-FZ, declared a past co-authorship with one of the authors, XJZ, to the handling editor.
All claims expressed in this article are solely those of the authors and do not necessarily represent those of their affiliated organizations, or those of the publisher, the editors and the reviewers. Any product that may be evaluated in this article, or claim that may be made by its manufacturer, is not guaranteed or endorsed by the publisher.
The authors would like to thank all the participating patients and healthy controls, as well as all the doctors and nurses who have contributed to this work.
The Supplementary Material for this article can be found online at: https://www.frontiersin.org/articles/10.3389/fonc.2021.755267/full#supplementary-material
Supplementary Figure 1 | NAA25 gene expression in public databases. (A, B) Compared to normal tissues NAA25 gene was highly expressed in breast cancer tissues in Finak breast and Richardson breast databases. (C) RT-qPCR and western blot analysis in the NAA25-deficient MCF7 cell line. The two bands are all NAA25. p < 0.05; **p < 0.001.
Supplementary Figure 2 | RNA-seq analysis in the NAA25-deficient T47D cells. (A) Pearson’s correlation analysis (PCA) clarified the similarity between RNA-seq samples. (B) Volcano plot showing the DEG in the Ctr group and the sh2 group. (C) GO term analysis between the Ctr group and the sh1 group. (D) GO analysis between the Ctr group and the sh1 group. (E) Gene set enrichment analysis (GSEA) to analyze the DEG between the Ctr group and the sh2 group.
1. Sung H, Ferlay J, Siegel RL, Laversanne M, Soerjomataram I, Jemal A, et al. Global Cancer Statistics 2020: GLOBOCAN Estimates of Incidence and Mortality Worldwide for 36 Cancers in 185 Countries. CA Cancer J Clin (2021) 71(3):209–49. doi: 10.3322/caac.21660
2. Feng RM, Zong YN, Cao SM, Xu RH. Current Cancer Situation in China: Good or Bad News From the 2018 Global Cancer Statistics? Cancer Commun (Lond Engl) (2019) 39(1):22. doi: 10.1186/s40880-019-0368-6
3. Chen W, Zheng R, Baade PD, Zhang S, Zeng H, Bray F, et al. Cancer Statistics in China, 2015. CA Cancer J Clin (2016) 66(2):115–32. doi: 10.3322/caac.21338
4. Zhang B, Chen MY, Shen YJ, Zhuo XB, Gao P, Zhou FS, et al. A Large-Scale, Exome-Wide Association Study of Han Chinese Women Identifies Three Novel Loci Predisposing to Breast Cancer. Cancer Res (2018) 78(11):3087–97. doi: 10.1158/0008-5472.CAN-17-1721
5. Milne RL, Kuchenbaecker KB, Michailidou K, Beesley J, Kar S, Lindstrom S, et al. Identification of Ten Variants Associated With Risk of Estrogen-Receptor-Negative Breast Cancer. Nat Genet (2017) 49(12):1767–78. doi: 10.1038/ng.3785
6. Xu JK, Li GZ, Li Z, Li WJ, Chen RS, Zhang B, et al. Genome-Wide Long non-Coding RNA Association Study on Han Chinese Women Identifies Lnchsat164 as a Novel Susceptibility Gene for Breast Cancer. Chin Med J (Engl) (2021) 134(10):1138–45. doi: 10.1097/CM9.0000000000001429
7. Todd JA, Walker NM, Cooper JD, Smyth DJ, Downes K, Plagnol V, et al. Robust Associations of Four New Chromosome Regions From Genome-Wide Analyses of Type 1 Diabetes. Nat Genet (2007) 39(7):857–64. doi: 10.1038/ng2068
8. Prahalad S, Hansen S, Whiting A, Guthery SL, Clifford B, McNally B, et al. Variants in TNFAIP3, STAT4, and C12orf30 Loci Associated With Multiple Autoimmune Diseases Are Also Associated With Juvenile Idiopathic Arthritis. Arthritis Rheum (2009) 60(7):2124–30. doi: 10.1002/art.24618
9. Bozpolat A, Unal E, Topaloglu T, Taheri S, Bayram AK, Ozcan A, et al. The Relationship Between the Prognosis of Children With Acute Arterial Stroke and Polymorphisms of CDKN2B, HDAC9, NINJ2, NAA25 Genes. J Thromb Thrombolysis (2019) 47(4):578–84. doi: 10.1007/s11239-018-01802-9
10. Yasukochi Y, Sakuma J, Takeuchi I, Kato K, Oguri M, Fujimaki T, et al. Identification of Six Novel Susceptibility Loci for Dyslipidemia Using Longitudinal Exome-Wide Association Studies in a Japanese Population. Genomics (2019) 111(4):520–33. doi: 10.1016/j.ygeno.2018.05.015
11. Van Damme P, Lasa M, Polevoda B, Gazquez C, Elosegui-Artola A, Kim DS, et al. N-Terminal Acetylome Analyses and Functional Insights of the N-Terminal Acetyltransferase NatB. Proc Natl Acad Sci USA (2012) 109(31):12449–54. doi: 10.1073/pnas.1210303109
12. Yasuda K, Takahashi M, Mori N. Mdm20 Modulates Actin Remodeling Through the Mtorc2 Pathway via Its Effect on Rictor Expression. PloS One (2015) 10(11):e0142943. doi: 10.1371/journal.pone.0142943
13. Alves S, Neiri L, Chaves SR, Vieira S, Trindade D, Manon S, et al. N-Terminal Acetylation Modulates Bax Targeting to Mitochondria. Int J Biochem Cell Biol (2018) 95:35–42. doi: 10.1016/j.biocel.2017.12.004
14. Starheim KK, Arnesen T, Gromyko D, Ryningen A, Varhaug JE, Lillehaug JR. Identification of the Human N(alpha)-Acetyltransferase Complex B (Hnatb): A Complex Important for Cell-Cycle Progression. Biochem J (2008) 415(2):325–31. doi: 10.1042/BJ20080658
15. Wrzesinski T, Szelag M, Cieslikowski WA, Ida A, Giles R, Zodro E, et al. Expression of Pre-Selected TMEMs With Predicted ER Localization as Potential Classifiers of ccRCC Tumors. BMC Cancer (2015) 15:518. doi: 10.1186/s12885-015-1530-4
16. Vatapalli R, Sagar V, Rodriguez Y, Zhao JC, Unno K, Pamarthy S, et al. Histone Methyltransferase DOT1L Coordinates AR and MYC Stability in Prostate Cancer. Nat Commun (2020) 11(1):4153. doi: 10.1038/s41467-020-18013-7
17. Ji H, Hui B, Wang J, Zhu Y, Tang L, Peng P, et al. Long Noncoding RNA MAPKAPK5-AS1 Promotes Colorectal Cancer Proliferation by Partly Silencing P21 Expression. Cancer Sci (2019) 110(1):72–85. doi: 10.1111/cas.13838
18. Cancer Genome Atlas Network. Comprehensive Molecular Portraits of Human Breast Tumours. Nature (2012) 490(7418):61–70. doi: 10.1038/nature11412
19. Curtis C, Shah SP, Chin SF, Turashvili G, Rueda OM, Dunning MJ, et al. The Genomic and Transcriptomic Architecture of 2,000 Breast Tumours Reveals Novel Subgroups. Nature (2012) 486(7403):346–52. doi: 10.1038/nature10983
20. Finak G, Bertos N, Pepin F, Sadekova S, Souleimanova M, Zhao H, et al. Stromal Gene Expression Predicts Clinical Outcome in Breast Cancer. Nat Med (2008) 14(5):518–27. doi: 10.1038/nm1764
21. Ma XJ, Dahiya S, Richardson E, Erlander M, Sgroi DC. Gene Expression Profiling of the Tumor Microenvironment During Breast Cancer Progression. Breast Cancer Res (2009) 11(1):R7. doi: 10.1186/bcr2222
22. Xu J, Li G, Chen M, Li W, Wu Y, Zhang X, et al. Rs12537 Is a Novel Susceptibility SNP Associated With Estrogen Receptor Positive Breast Cancer in Chinese Han Population. Front Med (2021) 8:708644. doi: 10.3389/fmed.2021.708644
23. Douroudis K, Kisand K, Nemvalts V, Rajasalu T, Uibo R. Allelic Variants in the PHTF1-PTPN22, C12orf30 and CD226 Regions as Candidate Susceptibility Factors for the Type 1 Diabetes in the Estonian Population. BMC Med Genet (2010) 11:11. doi: 10.1186/1471-2350-11-11
24. Lopez Herraez D, Martinez-Bueno M, Riba L, Garcia de la Torre I, Sacnun M, Goni M, et al. Rheumatoid Arthritis in Latin Americans Enriched for Amerindian Ancestry Is Associated With Loci in Chromosomes 1, 12, and 13, and the HLA Class II Region. Arthritis Rheum (2013) 65(6):1457–67. doi: 10.1002/art.37923
25. Oishi K, Yamayoshi S, Kozuka-Hata H, Oyama M, Kawaoka Y. N-Terminal Acetylation by NatB Is Required for the Shutoff Activity of Influenza A Virus PA-X. Cell Rep (2018) 24(4):851–60. doi: 10.1016/j.celrep.2018.06.078
26. Stark R, Grzelak M, Hadfield J. RNA Sequencing: The Teenage Years. Nat Rev Genet (2019) 20(11):631–56. doi: 10.1038/s41576-019-0150-2
27. Lai KC, Liu CJ, Chang KW, Lee TC. Depleting IFIT2 Mediates Atypical PKC Signaling to Enhance the Migration and Metastatic Activity of Oral Squamous Cell Carcinoma Cells. Oncogene (2013) 32(32):3686–97. doi: 10.1038/onc.2012.384
28. Koh SY, Moon JY, Unno T, Cho SK. Baicalein Suppresses Stem Cell-Like Characteristics in Radio- and Chemoresistant MDA-MB-231 Human Breast Cancer Cells Through Up-Regulation of IFIT2. Nutrients (2019) 11(3):624. doi: 10.3390/nu11030624
29. Godbole M, Togar T, Patel K, Dharavath B, Yadav N, Janjuha S, et al. Up-Regulation of the Kinase Gene SGK1 by Progesterone Activates the AP-1-NDRG1 Axis in Both PR-Positive and -Negative Breast Cancer Cells. J Biol Chem (2018) 293(50):19263–76. doi: 10.1074/jbc.RA118.002894
30. Yang Y, Liu Y, Guo R, Fu Y, Zhang Z, Zhang P, et al. The Novel Dithiocarbamate, DpdtC Suppresses HER2-Overexpressed Cancer Cells by Up-Regulating NDRG1 via Inactivation of HER2-ERK 1/2 Signaling. Sci Rep (2018) 8(1):3398. doi: 10.1038/s41598-018-21768-1
31. Buhard O, Lagrange A, Guilloux A, Colas C, Chouchene M, Wanherdrick K, et al. HSP110 T17 Simplifies and Improves the Microsatellite Instability Testing in Patients With Colorectal Cancer. J Med Genet (2016) 53(6):377–84. doi: 10.1136/jmedgenet-2015-103518
32. Boudesco C, Verhoeyen E, Martin L, Chassagne-Clement C, Salmi L, Mhaidly R, et al. HSP110 Sustains Chronic NF-kappaB Signaling in Activated B-Cell Diffuse Large B-Cell Lymphoma Through MyD88 Stabilization. Blood (2018) 132(5):510–20. doi: 10.1182/blood-2017-12-819706
33. Park HS, Park CH, Choi BR, Lim MS, Heo SH, Kim CH, et al. Expression of Heat Shock Protein 105 and 70 in Malignant Melanoma and Benign Melanocytic Nevi. J Cutan Pathol (2009) 36(5):511–6. doi: 10.1111/j.1600-0560.2008.01085.x
34. Gao H, Zheng Z, Mao Y, Wang W, Qiao Y, Zhou L, et al. Identification of Tumor Antigens That Elicit a Humoral Immune Response in the Sera of Chinese Esophageal Squamous Cell Carcinoma Patients by Modified Serological Proteome Analysis. Cancer Lett (2014) 344(1):54–61. doi: 10.1016/j.canlet.2013.10.007
Keywords: breast cancer, NAA25, cell cycle, apoptosis, RNA sequencing
Citation: Xu J, Li Z, Zuo X, Li G, Zhang X, Zhang B and Cui Y (2022) Knockdown of NAA25 Suppresses Breast Cancer Progression by Regulating Apoptosis and Cell Cycle. Front. Oncol. 11:755267. doi: 10.3389/fonc.2021.755267
Received: 07 September 2021; Accepted: 21 December 2021;
Published: 13 January 2022.
Edited by:
Dirk Geerts, University of Amsterdam, NetherlandsReviewed by:
Jianjun Chen, Tongji University, ChinaCopyright © 2022 Xu, Li, Zuo, Li, Zhang, Zhang and Cui. This is an open-access article distributed under the terms of the Creative Commons Attribution License (CC BY). The use, distribution or reproduction in other forums is permitted, provided the original author(s) and the copyright owner(s) are credited and that the original publication in this journal is cited, in accordance with accepted academic practice. No use, distribution or reproduction is permitted which does not comply with these terms.
*Correspondence: Yong Cui, d3VodWN1aXlvbmdAdmlwLjE2My5jb20=; Bo Zhang, YWx2aW5ib0AxNjMuY29t
Disclaimer: All claims expressed in this article are solely those of the authors and do not necessarily represent those of their affiliated organizations, or those of the publisher, the editors and the reviewers. Any product that may be evaluated in this article or claim that may be made by its manufacturer is not guaranteed or endorsed by the publisher.
Research integrity at Frontiers
Learn more about the work of our research integrity team to safeguard the quality of each article we publish.