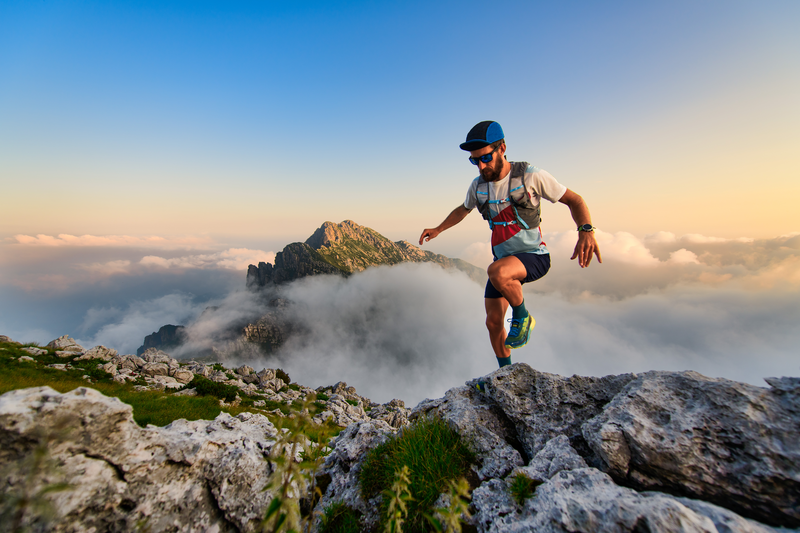
95% of researchers rate our articles as excellent or good
Learn more about the work of our research integrity team to safeguard the quality of each article we publish.
Find out more
ORIGINAL RESEARCH article
Front. Oncol. , 08 December 2021
Sec. Cancer Genetics
Volume 11 - 2021 | https://doi.org/10.3389/fonc.2021.741526
This article is part of the Research Topic Women in Cancer Genetics: 2021 View all 9 articles
Hepatoblastoma (HB) is a rare embryonal tumor, although it is the most common pediatric liver cancer. The aim of this study was to provide an accurate cytogenomic profile of this type of cancer, for which information in cancer databases is lacking. We performed an extensive literature review of cytogenetic studies on HBs disclosing that the most frequent copy number alterations (CNAs) are gains of 1q, 2/2q, 8/8q, and 20; and losses at 1p and 4q. Furthermore, the CNA profile of a Brazilian cohort of 26 HBs was obtained by array-CGH; the most recurrent CNAs were the same as shown in the literature review. Importantly, HBs from female patients, high-risk stratification tumors, tumors who developed in older patients (> 3 years at diagnosis) or from patients with metastasis and/or deceased carried a higher diversity of chromosomal alterations, specifically chromosomal losses at 1p, 4, 11q and 18q. In addition, we distinguished three major CNA profiles: no detectable CNA, few CNAs and tumors with complex genomes. Tumors with simpler genomes exhibited a significant association with the epithelial fetal subtype of HBs; in contrast, the complex genome group included three cases with epithelial embryonal histology, as well as the only HB with HCC features. A significant association of complex HB genomes was observed with older patients who developed high-risk tumors, metastasis, and deceased. Moreover, two patients with HBs exhibiting complex genomes were born with congenital anomalies. Together, these findings suggest that a high load of CNAs, mainly chromosomal losses, particularly losses at 1p and 18, increases the tendency to HB aggressiveness. Additionally, we identified six hot-spot chromosome regions most frequently affected in the entire group: 1q31.3q42.3, 2q23.3q37.3, and 20p13p11.1 gains, besides a 5,3 Mb amplification at 2q24.2q24.3, and losses at 1p36.33p35.1, 4p14 and 4q21.22q25. An in-silico analysis using the genes mapped to these six regions revealed several enriched biological pathways such as ERK Signaling, MicroRNAs in Cancer, and the PI3K-Akt Signaling, in addition to the WNT Signaling pathway; further investigation is required to evaluate if disturbances of these pathways can contribute to HB tumorigenesis. The analyzed gene set was found to be associated with neoplasms, abnormalities of metabolism/homeostasis and liver morphology, as well as abnormal embryonic development and cytokine secretion. In conclusion, we have provided a comprehensive characterization of the spectrum of chromosomal alterations reported in HBs and identified specific genomic regions recurrently altered in a Brazilian HB group, pointing to new biological pathways, and relevant clinical associations.
Hepatoblastoma (HB) is a very rare childhood cancer, accounting for approximately 1% of all pediatric tumors, with an annual incidence of 2.16 per million cases (1); however, it is the most common primary liver tumor in children, generally diagnosed in the first 3 years of life (2). Most HBs are sporadic, although it can occur in association with genetic syndromes, such as Familial Adenomatous Polyposis, Beckwith–Wiedemann syndrome (BWS), Trisomy 18 (Edwards Syndrome), and other inherited syndromes (3), in addition to a well-documented association with congenital abnormalities (4). This embryonal tumor is probably originated from undifferentiated hepatocyte precursor cells, since its histology recapitulates liver embryonic development, showing a combination of histological patterns from different stages of cell differentiation (5).
Alterations involving gene activators of the WNT signaling pathway are frequent, mainly CTNNB1 mutations, and rare AXIN1, AXIN2, and APC alterations; TERT promoter (2) and NFE2L2 mutations (6) were also reported. Another molecular mechanism known to be involved in HB development are imprinting defects at the BWS critical region (11p15), resulting in IGF2 overexpression and H19 downregulation (7); loss of heterozygosity (LOH) at 11p15 was also observed in some sporadic HBs, as well as in other embryonal tumors (8).
Cytogenetically, HB is characterized by copy number alterations involving gains of 1q, 2/2q, 8/8q and 20, as well as 4q and chromosome 18 losses (9, 10). A recurring translocation of chromosomes 1 and 4 was reported by Schneider et al. (11), resulting in a derivative chromosome [der(4)t(1,4) (q12; q34)]. In spite of the acknowledgment about these genomic alterations, their contribution to the molecular mechanisms involved in tumorigenesis and progression of HBs are poorly explored.
In this study, an extensive literature review of 45 published articles on HB cytogenetics/cytogenomics was performed, aiming to evaluate the profile of copy number alterations (CNA) in this type of cancer and their frequencies. Additionally, we investigated the CNA profile of a Brazilian cohort of 26 HBs, in an attempt to further define genomic regions of potential relevance in this tumor type.
This study was carried out through the collaboration of three clinical research groups of childhood cancer in São Paulo, Brazil: A.C Camargo Cancer Center (ACCCC), the Institute of Pediatric Oncology (Support Group for Children and Adolescents with Cancer (IOP-GRAACC), and the Institute of Childhood Cancer Treatment (ITACI). The study was approved by the Research Ethics Committees of the involved institutions, and informed consents were obtained from the patients’ parents or legal guardians. The cohort consisted of 26 HB tumor samples, including the reanalysis at higher resolution of samples previously reported (12). Table 1 presents the clinical features of each patient, and their respective tumors.
DNA was extracted from fresh frozen tumor tissue (specimen of surgery) samples, following standard technical procedures, using QIASymphony DNA Mini kit (QIAGEN).
CMA was performed using a 180K array-CGH platform (Agilent Technologies), following established protocols. Generated data were analyzed for CNAs using the Nexus Copy Number 9.0 software (BioDiscovery), with the FASST2 Segmentation algorithm, threshold log2 Cy3/Cy5 ratio of |0.1| for gains and losses (allowing the detection of mosaicism), and |1.2| for amplifications and homozygous losses. Common germline CNVs were disregarded based on the comparison with the Database of Genomic Variants (http://dgv.tcag.ca/dgv/app/home). The human genome annotation was based on the GRCh37/hg19 from the Genome Browser at the University of California Santa Cruz - UCSC (https://genome.ucsc.edu/).
Gene lists were evaluated using the VarElect tool (https://ve.genecards.org/), with the keywords “cancer” or “hepatoblastoma”. A gene set with the highest scores (top 10% cut-off; score > 6) was selected for an in-silico analysis using the online tool GeneAnalytics (http://geneanalytics.genecards.org/).
CMA revealed alterations in 16 of the 26 HB samples, identifying a total of 121 CNAs in the cohort (Supplementary Table 1), corresponding to ~4.6 CNAs per tumor, with a median size of 44.2 Mb (mean 56.7 Mb). Fifty-nine copy number gains were identified, ranging from 467 kb to 243 Mb (entire chromosome), with a median size of 77.1 Mb (mean 81.2 Mb); sixty-two losses were identified, ranging from 123.7 kb to 191 Mb (entire chromosome), with a median size of 2 Mb (mean 33.4 Mb). A schematic view of the CNA profile of the Brazilian HB cohort is shown in Figure 1. Among the 16 tumors carrying CNAs, the most frequent alterations were gains affecting 1q (61%), 2/2q (44%) and 20/20p (28%), while losses were predominant at 1p/1pter (44%) and 4/4q (28%).
Figure 1 Copy number alterations profile of the Brazilian hepatoblastoma cohort investigated by chromosomal microarray analysis using aCGH. Ideograms of chromosomes are depicted with copy number gains represented in blue bars at right, and losses in red at left; the width of the bars indicates the frequency of the alteration in the entire group. Chromosome Y was not evaluated. Image generated using the software Nexus Copy Number 9.0 (BioDiscovery).
Table 2 presents CNA types and absolute number detected in each tumor, as well as summarized clinical details; the immunohistochemistry information regarding CTNNB1 activation was retrieved from Aguiar et al. (15). We found significant associations with patient’s age at diagnosis < 3 and the presence of CTNNB1 activation (p value < 0.007), and patient’s age at diagnosis > 3 years and death from the disease (p value <0.028). Three categories of cytogenomic profile were defined based on the empirical observation of our own CNA data (Figure 2 and Supplementary Table 2). Considering only the 16 tumors with detectable CNAs, the mean CNA number was 7.6. Tumors with < 7.6 CNAs were classified as samples with few alterations (10 tumors: HB16T; HB17T, HB18T, HB36T, HB39T, HB40T, HB41T, HB43T, HB44T, HB79T), and tumors carrying > 7.6 CNAs were classified as complex genomes (six tumors: HB15T, HB30T, HB33T, HB46T, HB66T, HB70T). The third category was composed of HBs in which no CNAs were detected (10 samples: HB28T, HB31T, HB32T, HB34T, HB35T, HB38T, HB42T, HB45T, HB72T, HB81T).
Table 2 Description of number and types of CNAs in each tumor sample as well as summarized clinical features.
Figure 2 Illustrative CNA profiles representing the three categories of tumors identified by aCGH in the Brazilian HB cohort. No detectable CNA (HB42T), few alterations (HB43T) and complex genomes (HB70T). Microarray probes are plotted according to their genomic coordinates, each color representing a chromosome, from 1 to 22, X, from the short arm to the long arm. The Y axis represents the log2 scale of the copy number ratio tumor/control (values close to 0 indicate regions with similar copy number between tumor and control samples; positive values represent gains; negative values represent losses).
We evaluated the tumor's cytogenomic data looking for relevant differences regarding CNA profiles and clinical parameters such as sex, metastasis, patient’s status (deceased or not), risk stratification, and age at diagnosis. Figure 3 presents the CNA profiles associated with each of the above-mentioned parameters. We compared the complex genome HB group with the tumors carrying few CNAs, and mainly regions classically reported in HBs were detected in the latter category: gain of chromosomes 1q, 2q, 8 and 20, and 4q loss (Figure 3A). Although none specific CNA were enriched in HBs from males or females, the group of HBs from females presented with chromosomal alterations which are distributed along all the genome, including but not restricted to classical HB regions (Figure 3B). Three CNAs were enriched in the group of deceased patients (frequency > 35%; p value <0.04): loss of 1p, and gain of 2q proximal and chromosome 20 (Figure 3C). Moreover, it can be visualized that patients who deceased and/or had metastasis developed tumors with a higher diversity of CNAs, which were distributed in several different chromosomes. Similarly, high-risk HBs carried a higher frequency and diversity of CNAs, mainly chromosomal losses at 1p (frequency > 35%; p value <0.02), 4, 11q and 18q (Figure 3D). HBs from older patients (> 3 years) also exhibited more losses than tumors from younger patients (Figure 3E), with 1p deletion presenting a significant difference among these groups (frequency > 35%; p value <0.03). Tumors with CTNNB1 activation did not present remarkable differences when compared to those with negative CTNNB1 labelling (Supplementary Figure 1).
Figure 3 Comparison of CNA profiles of specific clinical features. The X axis displays chromosome profiles from 1 to 22, and X; chromosome Y was not evaluated. Copy number gains are represented in blue bars and losses in red; the width of the bars indicates the frequency of the alteration in the group. (A) Tumors with complex genomes versus samples with few CNAs. (B) Tumors from females versus tumors from males. (C) Tumors from patients who deceased versus tumors from alive patients. (D) High-risk versus low-risk tumors. (E) Tumors from patients diagnosed > 3 years-old versus samples from patients diagnosed < 3 years-old.
Additionally, the three CNA profile categories were associated with specific clinical features. The group of 10 tumors with no detectable CNA exhibited enrichment for the epithelial fetal histology (7/10 samples; p value <0.05), and were mostly low/intermediate risk classes (6/10 samples), without relapse, metastasis or fatal outcome. The group of 10 HBs carrying few alterations showed an excess of males (9/10 samples), intermediate/low risk tumors (7/10 samples), and was almost without relapse, metastasis, and death by disease. Opposite to this pattern, the group of six HB samples with complex genomes included three cases of the epithelial embryonal histology, in addition to the only HB with HCC features, and the only congenital HB of the casuistry. Moreover, we observed in the complex genome group a predominance of high-risk samples with significant enrichment for older patients (age at diagnosis > 3 years; p value <0.028), deceased status (p value <0.027) and presence of metastasis (p <0.027).
We also compared the cytogenomic data with the mutational status reported in our previous work based on exome sequencing (15), which presented a partial overlap of HB samples. Tumors with complex genomes also presented a higher number of somatic coding SNV/indel mutations when compared to the average of ~6 somatic mutations/sample observed in the whole group of HBs (HB15T = 17 mutations, HB30T = 15, HB33T = 38, HB46T = 7, HB70 = 11; exome data was not available for HB66).
We delimited genomic regions of the CNAs most commonly shared by the 16 HBs with detectable alterations. Six genomic regions (25% frequency cut-off; p value < 0.05) were delimited (1p36.33p35.1, 4p14, and 4q21.22q25 losses, and 1q31.3q42.3, 2q23.3q37.3, and 20p13p11.1 gains). Table 3 presents details of these regions, including genomic coordinates, size, number of encompassed genes, and genes previously reported in COSMIC. In particular, 1p loss was the most frequent deletion, specifically a 34 Mb 1p36.33p35.1 segment shared by ~27% of tumors. Regarding the COSMIC genes encompassed by these regions, four of them were already reported to be somatically mutated in HBs (PAX7, ARID1A, H3F3A, and NFE2L2), and 12 presented expression or epigenetic changes in these tumors (RPL22, CAMTA1, MDM4, ELK4, SLC45A3, CHN1, HOXD13, HOXD11, CREB1, IDH1, ACSL3, and TET2). We also identified a minimum common region of an amplification detected in two tumors (Figure 4), comprising a 5.3 Mb segment at 2q24.2q24.3 (chr2:161698894-167064256; GRCh37/hg19), presenting at least six copies.
Table 3 The six minimum regions delimited within the most frequent CNAs detected in the HB cohort (genomic coordinates given according to GRCh37/hg19).
Figure 4 2q24.2q24.3 amplification detected in two HBs. Microarray probes are plotted according to their genomic coordinates of the affected segment on chromosome 2, from the short arm to the long arm. The Y axis represents the log2 scale of the copy number ratio tumor/control (values close to 0 indicate regions with similar copy number between tumor and control samples, and positive values represent gains). Note the amplification regions as the highest peaks in the graphs.
The six delimited regions encompassed a total of 2,252 genes which were evaluated for their potential contribution to liver tumor biology (see Materials and Methods). The 229 genes with the highest scores (top 10% cut-off; score > 6; Supplementary Table 3) were submitted to an in-silico analysis using GeneAnalytics, with enrichment information regarding Diseases, Pathways, GO Terms, HPO Phenotypes, MGI Phenotypes and others (Supplementary Table 4). The top 10 diseases associated with the list of 229 genes were all cancers: hepatocellular, breast, colorectal, lung (and lung susceptibility), prostate, ovarian, gastric, pancreatic, and medulloblastoma (Supplementary Table 4A). The most relevant disclosed pathways, presenting with the highest scores (Supplementary Table 4B shows the top 50 highest scores), were ERK Signaling, MicroRNAs in Cancer, PI3K-Akt and Akt Signaling, Pathways in Cancer, Mesenchymal Stem Cells and Lineage-specific Markers, PEDF Induced Signaling, Human Embryonic Stem Cell Pluripotency, IL-2, and Proteoglycans in Cancer; these 10 highest scores identified for pathways and respective associated coding and non-coding genes can be found in Table 4. The Supplementary Table 4C detailed the observed top 50 enriched GO biological processes in HBs with their respective associated genes, while Supplementary Table 4D presents data relative to Positive/Negative regulation of GO Biological process. Table 5 presents the top 15 highest scores and the respective genes associated with the top 15 GO biological process in HBs; the 132 unique genes which are related to these enriched biological processes are separately listed in Table 6.
Table 4 List of the top 10 enriched pathways of the set of 229 genes used in the in-silico analysis.
Table 5 List of the top 15 enriched GO Biological process of the set of 229 genes used in the in-silico analysis.
Table 6 List of the 132 unique genes which are related to the top 15 enriched biological processes detected in hepatoblastomas.
Moreover, the analyzed set of 229 genes was found to be relevant for several neoplasms (liver, gastrointestinal and genitourinary tracts, hematological, skin and vascular) as well as abnormality of the liver function and morphology (Supplementary Table 4E). The analysis considering the association of these genes with reported mouse phenotypes highlighted abnormal embryonic development, embryonic lethality during organogenesis and premature death, decreased cell proliferation, decreased body weight and embryo size, and abnormal cytokine secretion (Supplementary Table 4F).
We compiled data from 45 studies, which investigated the entire genome of 480 primary HBs; samples with no detectable alterations (n = 141) were excluded. This literature review was based on CNA data obtained with techniques with different resolution (karyotype, FISH, CGH, and genomic microarrays; Supplementary Table 5), and CNAs were grouped only according to chromosome arm level; the results from Rodrigues et al. (12) were not included, since the analysis of the respective tumors was expanded at higher resolution and presented together with the entire Brazilian cohort, reported here.
The chromosomes most frequently reported to be altered in the 349 HBs with cytogenetic findings according to our review were chromosomes 1 (50.4%), 2 (54.7%), 8 (26.4%) and 20 (37.2%) for gains, while losses were predominant for chromosomes 1 (12.3%) and 4 (14.6%) (Figures 5A, B). The most recurrent alterations were typically restricted to specific chromosome arms, such as 1q (46.1%), 2q (22.4%) and 8q (8.3%) for gains, while the most frequent losses were located at 1p (9.4%) and 4q (11.7%). Moreover, gains affecting the chromosomes 5, 6, 7, 12 and 17 were present in this analysis in a frequency ranging from 10-13%.
Figure 5 Literature review of the cytogenomic data on hepatoblastomas. (A) Summary of the reported hepatoblastoma copy number chromosomal alterations in the literature from 1985 to 2017: chromosomes 1 to 12. (B) Summary of the reported hepatoblastoma copy number chromosomal alterations in the literature from 1985 to 2017: chromosomes 13 to 22, X, Y, and dmin. All references were these data were based can be found in the Supplementary Table 5.
The CNA data on 349 HBs from the literature and 16 samples from the present study are summarized in Figure 6. Taken together, the most recurrent CNAs in these 365 HBs were gains at 1 (51.5%), 2 (54.5%), 8 (27.2%) and 20 (36.8%), and chromosomes 1 (13.9%) and 4 (16%) losses.
Figure 6 Copy number gains and losses distributed per chromosome in 365 hepatoblastomas, based on our review of literature and the present study. Columns above the X axis represent the total number of gains per chromosome; below the X axis, the total number of losses. The frequencies of the most commonly chromosomal alterations detected in HBs in the literature along with our data are presented as percentages.
The analysis of chromosome alterations provides clues regarding biological pathways related to specific cancer types, and can have implications in tumor diagnosis and risk stratification, besides being used as prognostic markers in some cases (16). Therefore, the study of cancer genomes can help not only to understand the general biology, but also to differentiate subgroups within the same type of cancer. The major aim of this study was to identify CNA hot-spot regions in HB, possibly linked to clinical data, and at the same time compile the cytogenetic available literature data on this tumor, due to a lack of information on this tumor type in all cancer databases.
At the best of our knowledge, about 50 studies have been published reporting numerical and structural chromosomal alterations in HB tumors, comprising ~500 tumors that were characterized over a period of approximately 30 years. Among these studies, 45 investigated the entire genome of 480 primary HB tumors. In the present literature review, studies involving cell lines were excluded, but it is worth mentioning the papers from Doi (17) and Miyamoto et al. (18), who established and cytogenetically characterized, respectively, the hepatoblastoma cell line HUH-6-clone 5, with modal karyotype: 49, XY, +i(8q), +12, +20, producing the first cytogenetic information described in the literature on this type of tumor. It is important to point out that this is a compilation of data obtained with different techniques; therefore, this analysis precludes the precise mapping of CNAs based on cytobands. For this reason, CNAs were grouped only according to the chromosome arm where the alteration was located. Besides, HB genomes mostly carry aneuploidies and chromosome arm alterations, with a limited number of small CNAs. Also, tumors with no detectable alterations (n = 141, including ours) were removed from this review.
Literature data have been reporting that the most common CNAs in HBs were gains of 1q, 2/2q, 8/8q and 20; and 4q and 18 losses. Our review confirmed the literature reports for the most prevalent gains; however, the chromosome 18 loss did not stand out as a frequent event. It was noteworthy that 1p was found to be the second most common deletion in HBs in our review; likewise, in the Brazilian cohort, the 1p loss was the most frequent deletion, specifically a 34 Mb 1p36.33p35.1 segment shared by ~27% of the tumors. Therefore, there is a discrepancy regarding the frequency of chromosome 18 loss in HBs between our data and literature review when compared with previous reports. This discrepancy can be attributed to the fact that recent studies on the CNA profile of HBs did not take into account a large number of tumors such as we did (total of 365 HBs with cytogenetic findings). In fact, the last review performed on cytogenetic data was published in 2012 (19), with a smaller number of HBs. Furthermore, the chromosome 18 loss can be linked to a specific cohort, a group of 111 HBs analyzed by (20), in which they found chromosome 18 losses in several samples; probably, the notion that 18 loss is a common alteration in HB was derived from this study and the subsequent revision published in 2012 (19). However, interestingly, we observed in the Brazilian group an enrichment of chromosome 18 loss in high-risk HBs. Sumazin et al. (21) also reported 1p deletion as a frequent alteration in HBs. Deletions in the short arm of chromosome 1, particularly the terminal region, are frequently observed in many cancers, including hepatocellular carcinoma (22), neuroblastoma (23) and rhabdomyosarcoma (24). The frequency of this chromosomal alteration suggests that it is a nonrandom event, probably harboring tumor suppressors relevant for tumorigenesis in different tissues. At least five genes located at 1p36 (CHD5, CAMTA1, KIF1B, CASZ1, and MIR34A) were already suggested to be tumor suppressors (25).
It is also worth mentioning a recent published study proposing a DNA methylation-based classification for HBs (26), in a cohort of 59 samples; CNAs not commonly found in previous studies were reported, such as gains mapped to chromosomes 5, 6, 7, 12 and 17. Another recent study (6) also reported gains on chromosomes 5, 6, 7, 12 and 17 as frequent alterations in their 34 HBs cohort. These gains are represented along our literature review, in a frequency ranging from 10-13%. Another relevant aspect is that CNA gains, particularly of whole-chromosomes and whole-chromosome arms, occur more often than losses in the published studies. This prevalence of gains was not observed in our HB cohort; however, we did detect a significantly larger median length of the gain events (74.7 Mb) in comparison to losses (2 Mb), pointing to a pattern of prevalence of whole-chromosome/chromosome arms for gains, while the majority of losses were focal alterations.
Gene amplifications are frequently observed in cancer, and are one of the major causes of tumorigenesis driven by gene expression alterations as the result of copy number changes. Amplified regions are known to contain oncogenes and have the potential to serve as prognostic factors and therapeutic targets (27). Two tumors in our cohort, one of them previously reported (12), harbored 2q24 amplifications, a cytoband already described as amplified in other studies (28–32). This amplification has been associated with tumor progression (9, 30), and as indicative of poor prognosis (31). Clinically, the two Brazilian patients whose HBs harbor 2q24 amplifications were males diagnosed under one year of age, with high levels of AFP (> 200,000 ng/mL), and one of them classified as high risk, according to CHIC parameters (13, 14). We could restrict the minimum amplified region to a 5.3 Mb segment at 2q24.2q24.3, harboring 33 genes; however, the smallest 2q24 amplified region identified in HBs was documented by Kato et al. (30) a ~2 Mb region (chr2: 162954232-164891865; hg19), fully contained in our delimited region, and encompassing only eight genes (LOC101929532, GCG, FAP, IFIH1, GCA, KCNH7, KCNH7-AS1, FIGN). Two genes mapped on the larger 5.3 Mb amplification region could be highlighted: FAP, expressed mainly during embryogenesis, and in cancer-associated fibroblasts (33), and DPP4, which is overexpressed in several tumors and described as having a pro-oncogenic role in hepatocellular carcinoma (34). We have previously profiled the mRNA expression of 48 genes mapped to a 10 Mb 2q24 amplification (12), a larger segment encompassing ours and the smallest 2q24 amplified region documented by Kato et al. (30); only five genes were found to be significantly upregulated in the investigated tumors (DAPL1, ERMN, GALNT5, SCN1A and SCN3A).
In general, in the Brazilian group, HBs from categories such as females, high risk tumors, tumors who developed in older patients or from patients with metastasis and/or deceased, carried a higher diversity of chromosomal alterations scattered along the genome and not restricted to the reported recurrent regions. In particular, these tumors exhibited mainly chromosomal losses at 1p, 4, 11q and 18q, indicating that losses at these regions can be of worst prognosis.
According to the identified CNA profiles, we distinguished three major groups in our casuistry: no detectable CNA, few CNAs and tumors with complex genomes. Due to the small number of samples, only few significant clinical associations were disclosed in relation to these three CNA profiles. Still, some clinical differences can be pointed out. Tumors with simpler genomes (mainly no detected CNAs) exhibited a significant association with the epithelial fetal subtype of HB. Moreover, HBs with low CNA complexity were mainly stratified as low/intermediate risk (65% of the samples), and mostly observed in patients without relapse and metastasis.
In contrast, the complex genome group included three cases with epithelial embryonal histology, as well as the only HB with HCC features. Of note, we detected a significant association of complex genomes with older patients who developed high-risk tumors, with metastasis and poor outcome. The highest number of CNAs were detected in the HB46 and HB70 samples (27 and 24 CNAs, respectively); however, the reason for this discrepancy even compared to the group of complex genomes is not clear. Similarly, two patients with HB exhibiting complex genomes were clinically unusual; one was the only case of a congenital HB, who also was born with unilateral renal agenesis, while other presented global developmental delay and complex craniosynostosis. The association between congenital anomalies and pediatric cancer is well-documented in the literature (35), and another two HB patients of this cohort were born with congenital kidney abnormalities. Among these four HB patients with congenital anomalies, two of them exhibited complex genomes.
Together, these findings suggest that chromosomal losses, particularly 1p and 18 losses, and a high load of CNAs, increase the tendency to HB aggressiveness, and the presence of the sample with HB/HCC features in this group is in agreement with this hypothesis (36). To further explore this possibility, we integrated somatic CNA and SNV/indel data of overlapping tumors (15). We could observe that HBs which exhibited complex genomes also had a higher number of somatic coding mutations in comparison to the whole group; indeed, HBs with no chromosomal alterations presented with the lowest number of somatic mutations in our exome study, suggesting that other mechanisms must be involved in their tumorigenesis, such as epigenetic changes, as we previously hypothesized (37–40), and was corroborated by large recent studies (6, 26, 41). In addition, different metabolic profiles were shown to be HB biomarkers associated with CTNNB1 mutations and histological subtypes, disclosing an underlying pathway with relevant clinical implications (42).
Six chromosome regions were delimited in our cohort (1p36.33p35.1, 1q31.3q42.3, 2q23.3q37.3, 4p14, 4q21.22q25 and 20p13p11.1), and the encompassed genes were evaluated for their potential contribution to tumor biology, using in-silico analytic tools. Interestingly, the enrichment analysis showed that the most enriched biological pathways were the ERK Signaling, MicroRNAs in Cancer, and the PI3K/AKT Signaling, in addition to the well-known WNT Signaling pathway, listed several times in the analysis, but surprisingly not presenting the highest scores.
Disruptions in the ERK pathway have been associated with many developmental abnormalities as well as with cancer predisposition (43). Mutations resulting in the hyperactivation of ERK are frequently observed in many types of cancer, and the translocation of ERK into the cell nucleus seems to play an important role in the oncogenic process and cell proliferation (44). The PI3K/AKT pathway is also frequently mutated in many cancer types, and it is responsible for several cellular functions related to oncogenesis (45). In a previous work, we also found enrichment of the MAPK and WNT signaling pathways in HBs, suggesting modulation by methylation changes (37). Although studies (46–49) have reported dysregulations related to ERK and PI3K/AKT pathways in HB, further investigation is needed to detail how these pathways are contributing to HB tumorigenesis, possibly not directly linked to WNT. A previous work from our group (15) performed whole-exome sequencing in some samples herein reported, and a ERBB4 mutation, a gene related to ERK and AKT pathways, was detected. Additionally, the enriched biological pathways observed in this work also reinforce the occurrence of deregulation of the chemokine family in HB, as we previously reported (15). The association of chemokines with liver cancer has been increasingly studied, because most of them are involved in activation of the immunological cascades in tumors, frequently linked to worse prognosis and metastasis (50–53).
Based on the identified pathways in this study, we checked for genes or pathways that are drug targets in children and adolescents participating in the ongoing MATCH clinical trial (NCI-COG Pediatric MATCH - National Cancer Institute (https://www.cancer.gov/about-cancer/treatment/clinical-trials/nci-supported/pediatric-match, accessed on 2021-09-24) (54). Indeed, we found three drug targets currently under trial in MATCH: IDH1 (inhibitor ivosidenib), P13K/mTOR (LY3023414), and PARP (olaparib). Moreover, a talk between the PI3K/AKT and MAPK/ERK pathways has been reported and is probably related to cell survival regulation response; co-targeting and inhibition of these pathways has been shown effective in specific cancer treatments (55), and could be a possible new strategy for HB. Nevertheless, the relevance of these drugs for HB treatment is yet unknown.
Our data also highlighted an enrichment in the biological pathway MicroRNAs in Cancer, since several miRNA genes were mapped in the most frequent alterations in this HB cohort, in particular a cluster at 1q32. miRNAs can act as oncogenes or tumor suppressors and may have opposite functions depending on the cancer type (56). Studies exploring miRNAs in HB are scarce, but a recent review (57) pointed to some miRNAs which participate in key signaling pathways, such as WNT, Myc and Hippo.
Carrying out studies with HBs is challenging, mainly due to its rarity, thus resulting in lack of information about this tumor in cancer databases. In this work, we reviewed the data on HB cytogenetics and cytogenomics available in the literature, and also described the genomic CNA profile of a Brazilian cohort of 26 HBs. Even with the resolution limitations inherent to the techniques used in these studies, the most prevalent CNAs in HB were described, and new chromosome segments of interest were highlighted. Our analysis revealed diverse biological pathways that could be related to HB tumorigenesis, although certainly waiting for validations.
The original contributions presented in the study are included in the article/Supplementary Material. Further inquiries can be directed to the corresponding author.
The studies involving human participants were reviewed and approved by Hospital das Clínicas/FMUSP - CAAE 47277115.0.0000.0068, and A. C. Camargo Cancer Center Research Ethics Committee - number 1987/14. Written informed consent to participate in this study was provided by the participants’ legal guardian/next of kin. Written informed consent was obtained from the minor(s)’ legal guardian/next of kin for the publication of any potentially identifiable images or data included in this article.
Conceptualization: AK. Methodology JB, SC, TA,MR, MC, SRCT, EMN, VO, LMC, DMC, IWC, CMLC, and AK. Validation, JB, TA, AK. Formal analysis, JB,TA. Investigation, JB, TA, AK. Resources, JB, MC, ST, EN, VO, LC, DC, IC, CC, CR, and AK. Data curation, JB, SC, TA,MR, MC, SRCT, EMN, VO, LMC, DMC, IWC, CMLC, and AK. Writing—original draft preparation, JB. Writing—review and editing, JB, TA, CR, AMVM, AK Supervision, AK. Funding acquisition, CR, AMVM, AK. All authors contributed to the article and approved the submitted version.
This research was carried out with financial support from CAPES (Brazilian Federal Agency for Support and Evaluation of Graduate Education) project number 2017/1671499; and FAPESP (São Paulo Research Foundation) project numbers: 2019/17423-8, 2018/22893-0, 2018/21047-9 and CEPID - Human Genome and Stem-Cell Research Center - 2013/08028-1.
The authors declare that the research was conducted in the absence of any commercial or financial relationships that could be construed as a potential conflict of interest.
All claims expressed in this article are solely those of the authors and do not necessarily represent those of their affiliated organizations, or those of the publisher, the editors and the reviewers. Any product that may be evaluated in this article, or claim that may be made by its manufacturer, is not guaranteed or endorsed by the publisher.
We would like to thank the patients and their families for participating in this study. We also are indebted to Ligia Vieira whose expertise in molecular biology was essential for this research.
The Supplementary Material for this article can be found online at: https://www.frontiersin.org/articles/10.3389/fonc.2021.741526/full#supplementary-material
Supplementary Figure 1 | CNAs profile comparison between the tumors with CTNNB1 activation and negative tumors. No remarkable differences were seen. The X axis displays chromosome mapping from 1 to 22 and X, chromosome Y was not evaluated. Copy number gains are represented in blue bars and losses in red; the width of the bars indicates the frequency of the alteration in the group.
1. Feng J, Polychronidis G, Heger U, Frongia G, Mehrabi A, Hoffmann K. Incidence Trends and Survival Prediction of Hepatoblastoma in Children: A Population-Based Study. Cancer Commun (2019) 39(1):1–9. doi: 10.1186/s40880-019-0411-7
2. Czauderna P, Lopez-Terrada D, Hiyama E, Häberle B, Malogolowkin MH, Meyers RL. Hepatoblastoma State of the Art: Pathology, Genetics, Risk Stratification, and Chemotherapy. Curr Opin Pediatr (2014) 26(1):19–28. doi: 10.1097/MOP.0000000000000046
3. Aguiar TFM, Carneiro TN, da Costa CML, Rosenberg C, da Cunha IW, Krepischi ACV. The Genetic and Epigenetic Landscapes of Hepatoblastomas. Appl Cancer Res (2017) 37(1):1–7. doi: 10.1186/s41241-017-0021-0
4. Venkatramani R, Spector LG, Georgieff M, Tomlinson G, Krailo M, Malogolowkin M, et al. Congenital Abnormalities and Hepatoblastoma: A Report from the Children’s Oncology Group (COG) and the Utah Population Database (UPDB). Am J Med Genet Part A (2014) 23(1):1–7. doi: 10.1002/ajmg.a.36638
5. Sharma D, Subbarao G, Saxena R. Hepatoblastoma. Semin Diagn Pathol (2017) 34(2):192–200. doi: 10.1053/j.semdp.2016.12.015
6. Carrillo-Reixach J, Torrens L, Simon-Coma M, Royo L, Domingo-Sàbat M, Abril-Fornaguera J, et al. Epigenetic Footprint Enables Molecular Risk Stratification of Hepatoblastoma With Clinical Implications. J Hepatol (2020) 73(2):328–41. doi: 10.1016/j.jhep.2020.03.025
7. Honda S, Arai Y, Haruta M, Sasaki F, Ohira M, Yamaoka H, et al. Loss of Imprinting of IGF2 Correlates With Hypermethylation of the H19 Differentially Methylated Region in Hepatoblastoma. Br J Cancer (2008) 99(11):1891–9. doi: 10.1038/sj.bjc.6604754
8. Suzuki M, Kato M, Yuyan C, Takita J, Sanada M, Nannya Y, et al. Whole-Genome Profiling of Chromosomal Aberrations in Hepatoblastoma Using High-Density Single-Nucleotide Polymorphism Genotyping Microarrays. Cancer Sci (2008) 99(3):564–70. doi: 10.1111/j.1349-7006.2007.00710.x
9. Arai Y, Honda S, Haruta M, Kasai F, Fujiwara Y, Ohshima J, et al. Genome-Wide Analysis of Allelic Imbalances Reveals 4q Deletions as a Poor Prognostic Factor and MDM4 Amplification at 1q32.1 in Hepatoblastoma. Genes Chromosom Cancer (2010) 49(7):596–609. doi: 10.1002/gcc.20770
10. Tomlinson GE, Kappler R. Genetics and Epigenetics of Hepatoblastoma. Pediatr Blood Cancer (2012) 59(5):785–92. doi: 10.1002/pbc.24213
11. Schneider NR, Cooley LD, Finegold MJ, Douglass EC, Tomlinson GE. The First Recurring Chromosome Translocation in Hepatoblastoma: Der(4)T(1;4)(Q12;Q34). Genes Chromosom Cancer (1997) 19(4):291–4. doi: 10.1002/(SICI)1098-2264(199708)19:4<291::AID-GCC13>3.0.CO;2-J
12. Rodrigues TC, Fidalgo F, Da Costa CML, Ferreira EN, Da Cunha IW, Carraro DM, et al. Upregulated Genes at 2q24 Gains as Candidate Oncogenes in Hepatoblastomas. Futur Oncol (2014) 10(15):2449–57. doi: 10.2217/fon.14.149
13. Czauderna P, Haeberle B, Hiyama E, Rangaswami A, Krailo M, Maibach R, et al. The Children’s Hepatic Tumors International Collaboration (CHIC): Novel Global Rare Tumor Database Yields New Prognostic Factors in Hepatoblastoma and Becomes a Research Model. Eur J Cancer (2016) 52(March 2015):92–101. doi: 10.1016/j.ejca.2015.09.023
14. Meyers RL, Maibach R, Hiyama E, Häberle B, Krailo M, Rangaswami A, et al. Risk-Stratified Staging in Paediatric Hepatoblastoma: A Unified Analysis From the Children’s Hepatic Tumors International Collaboration. Lancet Oncol (2017) 18(1):122–31. doi: 10.1016/S1470-2045(16)30598-8
15. Aguiar TFM, Rivas MP, Costa S, Maschietto M, Rodrigues T, Sobral de Barros J, et al. Insights Into the Somatic Mutation Burden of Hepatoblastomas From Brazilian Patients. Front Oncol (2020) 10:1–16. doi: 10.3389/fonc.2020.00556
16. Vargas-Rondón N, Villegas VE, Rondón-Lagos M. The Role of Chromosomal Instability in Cancer and Therapeutic Responses. Cancers (Basel) (2018) 10:1–21. doi: 10.3390/cancers10010004
17. Doi I. Establishment of a Cell Line and Its Clonal Sublines From a Patient With Hepatoblastoma. Gann Japan J Cancer Res (1976) 67(1):1–10.
18. Miyamoto K, Taketa K, Yabe T, Miyano K, Sato J. Chromosome Analysis in a Human Hepatoblastoma Cell Line Producing α-Fetoprotein. Acta Med Okayama (1980) 34(2):127–30. doi: 10.18926/AMO/30534
19. Tomlinson GE. Cytogenetics of Hepatoblastoma. Front Biosci (2012) 4(4):1287–92. doi: 10.2741/e459
20. Tomlinson GE, Douglass EC, Pollock BH, Finegold MJ, Schneider NR. Cytogenetic Evaluation of a Large Series of Hepatoblastomas: Numerical Abnormalities With Recurring Aberrations Involving 1q12-Q21. Genes Chromosom Cancer (2005) 44:177–84. doi: 10.1002/gcc.20227
21. Sumazin P, Chen Y, Treviño LR, Sarabia SF, Hampton OA, Patel K, et al. Genomic Analysis of Hepatoblastoma Identifies Distinct Molecular and Prognostic Subgroups. Hepatology (2017) 65(1):104–21. doi: 10.1002/hep.28888
22. Liu M, Jiang L, Guan X. The Genetic and Epigenetic Alterations in Human Hepatocellular Carcinoma: A Recent Update. Protein Cell (2014) 5(9):673–91. doi: 10.1007/s13238-014-0065-9
23. Henrich K, Bauer T, Schulte J, Ehemann V, Deubzer H, Gogolin S, et al. CAMTA1, a 1p36 Tumor Suppressor Candidate, Inhibits Growth and Activates Differentiation Programs in Neuroblastoma Cells. Cancer Res (2011) 71(8):3142–52. doi: 10.1158/0008-5472.CAN-10-3014
24. Shern JF, Yohe ME, Khan J. Pediatric Rhabdomyosarcoma. Crit Rev Oncog (2018) 20:227–43. doi: 10.1615/CritRevOncog.2015013800
25. Henrich KO, Schwab M, Westermann F. 1p36 Tumor Suppression - A Matter of Dosage? Cancer Res (2012) 72(23):6079–88. doi: 10.1158/0008-5472.CAN-12-2230
26. Sekiguchi M, Seki M, Kawai T, Yoshida K, Yoshida M, Isobe T, et al. Integrated Multiomics Analysis of Hepatoblastoma Unravels Its Heterogeneity and Provides Novel Druggable Targets. NPJ Precis Oncol (2020) 4(1):1–12. doi: 10.1038/s41698-020-0125-y
27. Matsui A, Ihara T, Suda H, Mikami H, Semba K. Gene Amplification: Mechanisms and Involvement in Cancer. Biomol Concepts (2013) 4(6):567–82. doi: 10.1515/bmc-2013-0026
28. Gray SG, Kytölä S, Matsunaga T, Larsson C, Ekström TJ. Comparative Genomic Hybridization Reveals Population-Based Genetic Alterations in Hepatoblastomas. Br J Cancer (2000) 83(8):1020–5. doi: 10.1054/bjoc.2000.1390
29. Hu J, Wills M, Baker BA, Perlman EJ. Comparative Genomic Hybridization Analysis of Hepatoblastomas. Genes Chromosom Cancer (2000) 27(2):196–201. doi: 10.1002/(SICI)1098-2264(200002)27:2<196::AID-GCC12>3.0.CO;2-D
30. Kato M, Takita J, Takahashi K, Mimaki M, Chen Y, Koh K, et al. Hepatoblastoma in a Patient With Sotos Syndrome. J Pediatr (2009) 155(6):937–9. doi: 10.1016/j.jpeds.2009.06.039
31. Kumon K, Kobayashi H, Namiki T, Tsunematsu Y, Miyauchi J, Kikuta A, et al. Frequent Increase of DNA Copy Number in the 2q24 Chromosomal Region and Its Association With a Poor Clinical Outcome in Hepatoblastoma: Cytogenetic and Comparative Genomic Hybridization Analysis. Japan J Cancer Res (2001) 92(8):854–62. doi: 10.1111/j.1349-7006.2001.tb01172.x
32. Weber RG, Pietsch T, Von Schweinitz D, Lichter P. Characterization of Genomic Alterations in Hepatoblastomas: A Role for Gains on Chromosomes 8q and 20 as Predictors of Poor Outcome. Am J Pathol (2000) 157(2):571–8. doi: 10.1016/S0002-9440(10)64567-1
33. Tyulkina DV, Pleshkan VV, Alekseenko IV, Kopantseva MR, Sverdlov ED. Expression of the FAP Gene in Non-Fibroblast Human Cell Lines. Development of Cancer-Associated Fibroblast Models. Dokl Biochem Biophys (2016) 470(1):319–21. doi: 10.1134/S1607672916050033
34. Enz N, Vliegen G, De Meester I, Jungraithmayr W. CD26/DPP4 - a Potential Biomarker and Target for Cancer Therapy. Pharmacol Ther (2019) 198:135–59. doi: 10.1016/j.pharmthera.2019.02.015
35. Johnson KJ, Lee JM, Ahsan K, Padda H, Feng Q, Partap S, et al. Pediatric Cancer Risk in Association With Birth Defects: A Systematic Review. PloS One (2017) 12:1–56. doi: 10.1371/journal.pone.0181246
36. Eichenmüller M, Trippel F, Kreuder M, Beck A, Schwarzmayr T, Häberle B, et al. The Genomic Landscape of Hepatoblastoma and Their Progenies With HCC-Like Features. J Hepatol (2014) 61(6):1312–20. doi: 10.1016/j.jhep.2014.08.009
37. Maschietto M, Rodrigues TC, Kashiwabara AY, de Araujo ÉSS, Aguiar TFM, da Costa CML, et al. DNA Methylation Landscape of Hepatoblastomas Reveals Arrest at Early Stages of Liver Differentiation and Cancer-Related Alterations. Oncotarget (2017) 8(58):97871–89. doi: 10.18632/oncotarget.14208
38. Rivas MP, Aguiar TFM, Fernandes GR, Caires LC, Goulart E, Telles-Silva KA, et al. TET Upregulation Leads to 5-Hydroxymethylation Enrichment in Hepatoblastoma. Front Genet (2019) 10:1–7. doi: 10.3389/fgene.2019.00553
39. Rivas MP, Aguiar TFM, Maschietto M, Lemes RB, Caires-Júnior LC, Goulart E, et al. Hepatoblastomas Exhibit Marked NNMT Downregulation Driven by Promoter DNA Hypermethylation. Tumor Biol (2020) 42(12):1–14. doi: 10.1177/1010428320977124
40. Rivas M, Aguiar T, Fernandes G, Lemes R, Caires-Júnior L, Goulart E, et al. DNA Methylation as a Key Epigenetic Player for Hepatoblastoma Characterization. Clin Res Hepatol Gastroenterol (2021) 45(3). doi: 10.1016/j.clinre.2021.101684
41. Hooks KB, Audoux J, Fazli H, Lesjean S, Ernault T, Dugot-Senant N, et al. New Insights Into Diagnosis and Therapeutic Options for Proliferative Hepatoblastoma. Hepatology (2018) 68(1):89–102. doi: 10.1002/hep.29672
42. Crippa S, Ancey P, Vazquez J, Angelino P, Rougemont A, Guettier C, et al. Mutant CTNNB 1 and Histological Heterogeneity Define Metabolic Subtypes of Hepatoblastoma. EMBO Mol Med (2017) 9(11):1589–604. doi: 10.15252/emmm.201707814
43. Patel AL, Shvartsman SY. Outstanding Questions in Developmental ERK Signaling. Dev (2018) 145(14):1–12. doi: 10.1242/dev.143818
44. Maik-Rachline G, Hacohen-Lev-Ran A, Seger R. Nuclear Erk: Mechanism of Translocation, Substrates, and Role in Cancer. Int J Mol Sci (2019) 20:1–18. doi: 10.3390/ijms20051194
45. Mayer IA, Arteaga CL. The PI3K/AKT Pathway as a Target for Cancer Treatment. Annu Rev Med (2016) 67(September 2015):11–28. doi: 10.1146/annurev-med-062913-051343
46. Cui Y, Lu P, Song G, Liu Q, Zhu D, Liu X. Involvement of PI3K/Akt, ERK and P38 Signaling Pathways in Emodin-Mediated Extrinsic and Intrinsic Human Hepatoblastoma Cell Apoptosis. Food Chem Toxicol (2016) 92:26–37. doi: 10.1016/j.fct.2016.03.013
47. Haines K, Sarabia SF, Alvarez KR, Tomlinson G, Vasudevan SA, Heczey AA, et al. Characterization of Pediatric Hepatocellular Carcinoma Reveals Genomic Heterogeneity and Diverse Signaling Pathway Activation. Pediatr Blood Cancer (2019) 66(7):1–10. doi: 10.1002/pbc.27745
48. Chen L, Tian X, Gong W, Sun B, Li G, Liu D, et al. Periostin Mediates Epithelial-Mesenchymal Transition Through the MAPK/ERK Pathway in Hepatoblastoma. Cancer Biol Med (2019) 16(1):89–99. doi: 10.20892/j.issn.2095-3941.2018.0077
49. Cui X, Liu X, Han Q, Zhu J, Li J, Ren Z, et al. DPEP1 Is a Direct Target of miR-193a-5p and Promotes Hepatoblastoma Progression by PI3K/Akt/mTOR Pathway. Cell Death Dis (2019) 10(10):1–16. doi: 10.1038/s41419-019-1943-0
50. Xu X, Huang P, Yang B, Wang X, Xia J. Roles of CXCL5 on Migration and Invasion of Liver Cancer Cells. J Transl Med (2014) 12(1):1–11. doi: 10.1186/1479-5876-12-193
51. O’Hayre M, Salanga CL, Handel TM, Allen SJ. Chemokines and Cancer: Migration, Intracellular Signalling and Intercellular Communication in the Microenvironment. Biochem J (2008) 409(3):635–49. doi: 10.1042/BJ20071493
52. Liang CM, Chen L, Hu H, Ma HY, Gao LL, Qin J, et al. Chemokines and Their Receptors Play Important Roles in the Development of Hepatocellular Carcinoma. World J Hepatol (2015) 7(10):1390–402. doi: 10.4254/wjh.v7.i10.1390
53. Jeng KS, Jeng CJ, Jeng WJ, Chang CF, Sheen IS. Role of C-X-C Chemokine Ligand 12/C-X-C Chemokine Receptor 4 in the Progression of Hepatocellular Carcinoma (Review). Oncol Lett (2017) 14(2):1905–10. doi: 10.3892/ol.2017.6396
54. Parsons DW, Janeway KA, Patton D, Coffey B, Williams PM, Hamilton SR, et al. Identification of Targetable Molecular Alterations in the NCI-COG Pediatric MATCH Trial. J Clin Oncol (2019) 37(15_suppl):10011–1. doi: 10.1200/JCO.2019.37.15_suppl.10011
55. Peng X, Liu Y, Zhu S, Peng X, Li H, Jiao W, et al. Co-Targeting PI3K/Akt and MAPK/ERK Pathways Leads to an Enhanced Antitumor Effect on Human Hypopharyngeal Squamous Cell Carcinoma. J Cancer Res Clin Oncol (2019) 145(12):2921–36. doi: 10.1007/s00432-019-03047-2
56. Svoronos A, Engelman D, Slack F. OncomiR or Tumor Suppressor? The Duplicity of MicroRNAs in Cancer. Cancer Res (2016) 76(13):3666–70. doi: 10.1158/0008-5472.CAN-16-0359
Keywords: copy number alteration, hepatoblastoma, pediatric cancer, cytogenomics, array-CGH
Citation: Barros JS, Aguiar TFM, Costa SS, Rivas MP, Cypriano M, Toledo SRC, Novak EM, Odone V, Cristofani LM, Carraro DM, Werneck da Cunha I, Costa CML, Vianna-Morgante AM, Rosenberg C and Krepischi ACV (2021) Copy Number Alterations in Hepatoblastoma: Literature Review and a Brazilian Cohort Analysis Highlight New Biological Pathways. Front. Oncol. 11:741526. doi: 10.3389/fonc.2021.741526
Received: 14 July 2021; Accepted: 10 November 2021;
Published: 08 December 2021.
Edited by:
Nada A. Al Tassan, King Faisal Specialist Hospital & Research Centre, Saudi ArabiaReviewed by:
Rajeev K. Singla, Sichuan University, ChinaCopyright © 2021 Barros, Aguiar, Costa, Rivas, Cypriano, Toledo, Novak, Odone, Cristofani, Carraro, werneck da Cunha, Costa, Vianna-Morgante, Rosenberg and Krepischi. This is an open-access article distributed under the terms of the Creative Commons Attribution License (CC BY). The use, distribution or reproduction in other forums is permitted, provided the original author(s) and the copyright owner(s) are credited and that the original publication in this journal is cited, in accordance with accepted academic practice. No use, distribution or reproduction is permitted which does not comply with these terms.
*Correspondence: Ana Cristina Victorino Krepischi, YW5hLmtyZXBpc2NoaUBpYi51c3AuYnI=
Disclaimer: All claims expressed in this article are solely those of the authors and do not necessarily represent those of their affiliated organizations, or those of the publisher, the editors and the reviewers. Any product that may be evaluated in this article or claim that may be made by its manufacturer is not guaranteed or endorsed by the publisher.
Research integrity at Frontiers
Learn more about the work of our research integrity team to safeguard the quality of each article we publish.