- 1Department of Nephrology, The Fourth Affiliated Hospital, and Institute of Translational Medicine, Zhejiang University School of Medicine, Jinhua, China
- 2Department of Urology, The First Affiliated Hospital, Zhejiang University School of Medicine, Hangzhou, China
Liquid biopsy includes non-invasive analysis of circulating tumor-derived substances. It is a novel, innovative cancer screening tool that overcomes the limitations of current invasive tissue examinations in precision oncology. Circular RNA (circRNA) is a recent, novel, and attractive liquid biomarker showing stability, abundance, and high specificity in various diseases, especially in human cancers. This review focused on the emerging potential of human circRNA in body fluids as the liquid biopsy biomarkers for cancers and the methods used to detect the circRNA expression and summarized the construction of circRNA biomarkers in body fluids for treating human cancers and their limitations before they become part of routine clinical medicine. Furthermore, the future opportunities and challenges of translating circRNAs in liquid biopsy into clinical practices were explored.
Introduction
Cancer is one of the leading causes of death worldwide, with more than 10 million people deaths estimated in 2020, and the morbidity and mortality rates are increasing. The development of “omics” technology has facilitated the progress of precision oncology, which includes tailoring treatment plans based on the molecular characteristics of each patient’s tumor (1). In the current era of precision oncology, liquid biopsy specimen collection is far less invasive than tissue specimen collection and can be repeated several times during the follow-up process to achieve real-time monitoring. Liquid biopsy can eliminate the tumor heterogeneity effect by determining the genomic profile of cancer patients, assist with a systemic and comprehensive representation of disease, help with early cancer detection (even before radiological and imaging tests detect the presence of tumors), aid in evaluating therapeutic reaction, detect minimal residual disease, and test for cancer cells’ drug resistance. Besides blood, several other body fluids such as urine (2), saliva (3), pleural effusions (4), and cerebrospinal fluid (CSF) (5) as well as stool (6) can be used to reveal information about the tumor. Biofluid sampling and analysis provide an accurate account of the molecular traits of primary tumors and distant metastases. Most liquid biopsy analyses have displayed their clinical utility in human cancers (7–10). The first important milestone in this field was reached in 2016 with the Food and Drug Administration (FDA) approval of the first companion diagnostic test for lung cancer based on the circulating tumor DNA (ctDNA) content of a liquid biopsy. Besides ctDNA, several other biomarkers in circulating body fluids have been studied, such as circulating tumor cells (CTCs) (11), extracellular vesicles (EVs) (12), cell-free RNA (cfRNA) (13), circulating proteins (14), circulating metabolites (15), and platelets (16). Among these, RNA-based liquid biomarkers have received increasing attention because of their varied expression levels and strong links with cancer (17).
CircRNA stems from spliceosome-mediated non-sequential backsplicing of precursor mRNA (pre-mRNA); that is, the downstream 5′ splice donor site is connected to the upstream 3′ splice acceptor site (18). They were found in RNA viruses as viroids for the first time in 1976 and have been considered a result of splicing errors for several years (19). CircRNAs can be divided into five categories: exonic circular RNAs (ecircRNAs) (20, 21), intronic circular RNAs (ciRNAs) (20, 22), exon–intron circular RNAs (EIciRNAs) (21), intergenic circRNAs (20), and antisense circular circRNAs (20). EcircRNA, mostly distributed in the cytoplasm, accounts for nearly 85% of all recognized circRNAs (20). CiRNAs and EIciRNAs are primarily concentrated in the nucleus, indicating that they may have different biological functions (23).
Compared to the traditional tumor tissue biopsy biomarkers, circRNAs are novel, convenient, and non-invasive liquid biopsy biomarkers. Their high abundance and stability (24), abundant expression (25), and high specificity (26) make circRNAs a promising biomarker for various diseases, particularly cancers (27). Lots of research have been done to study circRNAs as a liquid biopsy biomarker in cancer (Figure 1) owing to the advantages they offer (27) and the significance of liquid biopsy biomarkers in precision medicine (28). In this review, we have summarized the latest information about circRNAs in liquid biopsy, including their biological significance in the development and progression of cancer, their potency in cancer diagnosis, monitoring, and prognosis and outlined the advantages and limitations of available methods used to detect the circRNA expression and their different roles in various human cancers. Moreover, the various circRNA biomarkers constructed for treating human tumors were summarized. We have further investigated future opportunities and challenges of translating circRNAs into clinical applications and outlined significant technological advances that may overcome these shortcomings.
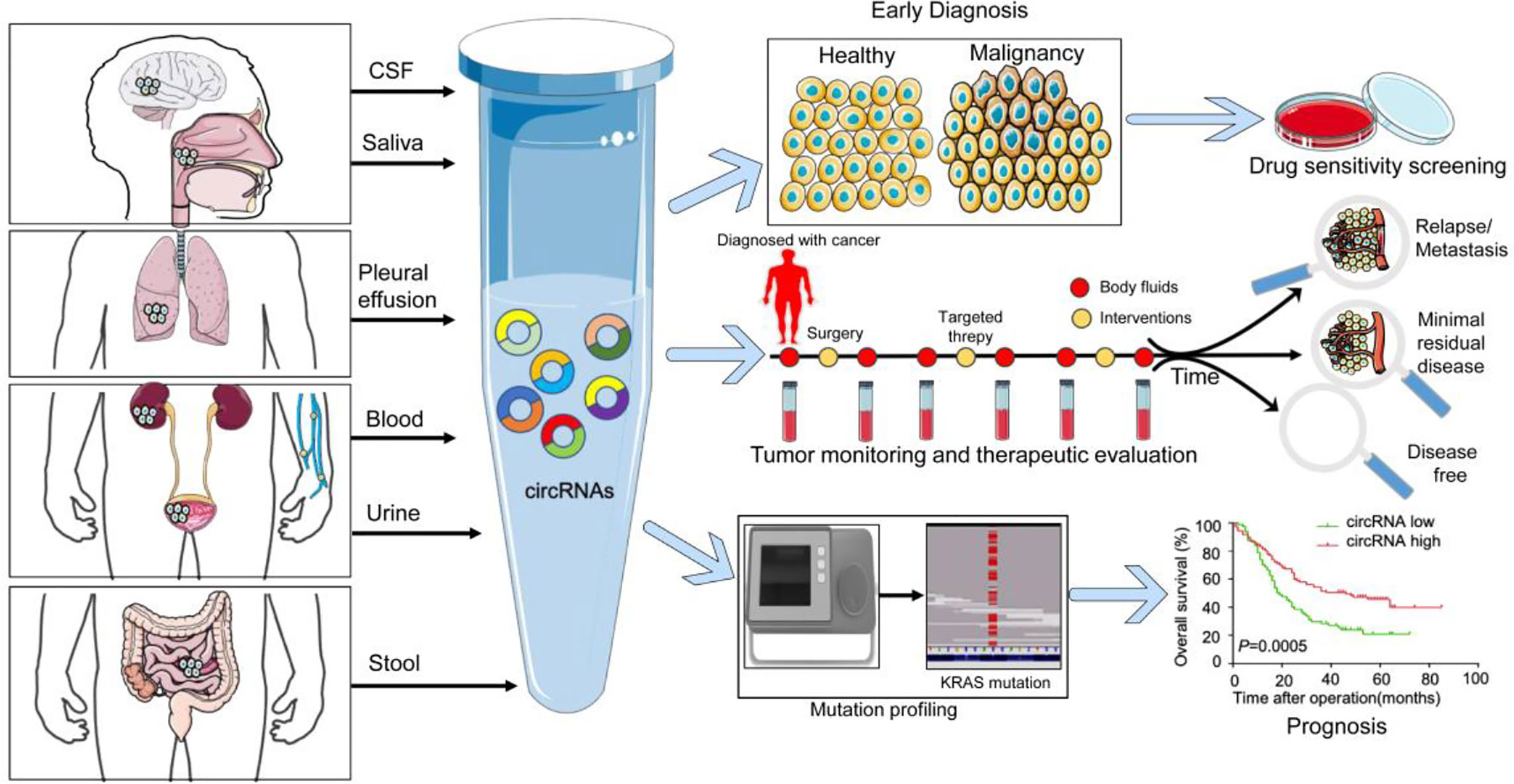
Figure 1 The value of circRNA as a liquid biopsy marker in tumor diagnosis and precision medicine. Schematic representation of different body fluids that contain circRNAs, specifically including cerebrospinal fluid (CSF), saliva, pleural effusions, blood, urine, and stool. The localization of the primary or metastatic lesions influences the presence of circulating circRNAs in individual body fluids. As indicated, cancers can be detected through analysis of the circRNA content derived from the biofluids and further predict the sensitivity of treatment drugs. Longitudinal monitoring of circRNAs could be used to monitor tumor progression and therapeutic effects. Genomic mutation associated with the circRNA expression could be used to assess prognosis.
Biological Function of Body-Fluid CircRNAs in Cancer
The potential functions of circRNAs have been extensively studied. It can regulate fundamental biological processes and display an essential function in various human illnesses, including cancers (29–34). One of the most important functions of circRNAs is their role in cell viability and distant metastasis.
CircRNAs and Proliferation
Proliferation disorder is one of the critical elements of tumor transformation, making the cell-cycle regulation mechanism a much sought-after research topic (35). Xue et al. demonstrated that exosomal circRNA_100284 acts as the microRNA-217 (miR-217) sponge (36), regulating the cell cycle by inducing G2/M phase arrest, inhibiting cell proliferation, and targeting EZH2 (proliferation indicator) in various cancers (37, 38). Exosomal circSATB2 promotes the cell proliferation of non-small lung cancer cells (NSLCCs) via sponging miR-326 (39). These proofs validate the role of exosomal circRNAs in regulating the cell cycle and cell proliferation. Another research reported a significantly higher circRASSF2 level of laryngeal squamous cell carcinoma (LSCC) in serum exosomes than the normal groups (40). Moreover, knockdown of circ-RASSF2 remarkably suppressed cell proliferation through the miR-302b-3p/insulin-like growth factor 1 (IGF-1R) axis, suggesting the importance of serum circRNAs in tumor proliferation.
CircRNAs and Metastasis
The increasing evidence shows that the circRNA expression in certain biofluids is associated with cancer progression and metastasis. For instance, Lu et al. demonstrated a critical role of plasma circ-RanGAP1 in gastric cancer (GC). They showed that the expression level of circ-RanGAP1 in GC and plasma was closely connected with the advanced tumor-node-metastasis (TNM) stage, lymph node metastases, and low survival rate. Circ-RanGAP1 has been shown to accelerate tumor-cell growth by mediating the miR-877-3a/vascular endothelial growth factor A (VEGFA axis) (41). Likewise, a recent study verified that enriched circ-133 in the plasma exosome of colorectal cancer cells (CRC) patients could promote cancer metastasis via circ-133-mediated miR-133a/guanine nucleotide exchange factor-H1 (GEF-H1)/Ras homolog family member A (RhoA) signaling pathway, suggesting that exosomal circ-133 can be a surveillance biomarker for progression of CRC (42). This phenomenon has also been observed in hepatocellular carcinoma cells and patients (43). Chen et al. have also reported significantly increased circPRMT5 expression in serum and urine of patients with urothelial carcinoma of the bladder (UCB) (44). The research also reported that exosomal circPRMT5 facilitated the epithelial–mesenchymal transition (EMT) of UCB cells through miR-30c/SNAIL1/E-cadherin signaling.
CircRNAs in Body Fluids Are Promising Biomarkers for Cancer
A good clinically significant biomarker must fit the analytical and clinical validity and clinical utility standards (45). As a newly identified noncoding RNA, circRNA has obvious advantages over typical linear RNAs as an illness biomarker (27). The circular covalent bond structure of circRNAs makes them highly resistant to exonuclease degradation and prolongs their lifespan (24, 46). Additionally, circRNAs express themselves abundantly in various human tissue samples, such as saliva (47) and plasma (48), and even in serum exosomes (49), indicating their role as suitable biomarkers for liquid biopsy (Figure 1). A number of circRNAs have been considered as biomarkers of human diseases recently due to the high stability of circRNAs in mammalian cells (27), especially in some malignancies (50–53). A study with 1,195 plasma samples used plasma circRNAs to diagnose hepatitis B virus (HBV)-related hepatocellular carcinoma (HCC) (54). The study reported that the plasma expression levels of hsa_circ_0000976, hsa_circ_00077550, and hsa_circ_0139897 in HCC patients are significantly higher than those in the control group. Lin et al. (55) also found that the plasma levels of circ-CCDC66, circ-ABCC1, and circ-STIL are significantly reduced in CRC patients compared to the control group. The sensitivity and specificity of the combined diagnosis of CRC using these three circRNAs were 64.4% and 85.2%, respectively. Gastric cancer is the most widely studied category of cancer in the field of circRNA research, and some publications have depicted the dysregulation of circRNAs in plasma such as hsa_circ_0001017, hsa_circ_0061276 (56), hsa_circ_0000745 (57), hsa_circ_0000520 (58), hsa_circ_0000190 (59), hsa_circ_0001649 (60), hsa_circ_0000181 (61), and circ_002059 (50), all of which have displayed diagnostic effectiveness. A markedly downregulated expression of hsa_circ_002059 was detected in GC tissues compared to the matched para-carcinoma tissues (50). Different expressions of hsa_circ_002059 in plasma were detected in 36 paired plasma samples from pre- and postoperative GC patients. A low expression of hsa_circ_002059 was closely associated with age, gender, TNM stage, and distant metastasis. The assessment of the diagnostic value of hsa_circ_002059 showed that its sensitivity and specificity were 81.0% and 62.0%, respectively, and the area under the curve (AUC) was 0.73, suggesting hsa_circ_002059 to be a potentially stable GC biomarker. Tan et al. reported a fusion circRNA called F-circEA, derived from an EML4-ALK fusion gene, and it is positively expressed in five of six NSCLC patients with EML4-ALK translocation (62). F-circEA also exists in the plasma of EMLA4-ALK-positive NSCLC patients specifically.
CircRNAs have broad application prospects in monitoring the treatment efficacy and assessing cancer prognosis. Li et al. identified more than 1,000 circRNAs in human serum exosomes for the first time and proposed that these circRNAs could distinguish colon cancer patients from the healthy controls (46). The decreased expression of circKIAA1244 in plasma (63) and increased expression of hsa_circ_0000467 (64) were closely correlated with the shorter overall survival time of GC patients. Pan et al. (65) used quantitative reverse transcriptase-polymerase chain reaction (qRT-PCR) to detect the expression of hsa-circ-0004771 in circulating exosomes of 170 patients and 45 healthy controls and pointed out that the hsa-circ-0004771 expression in the serum of CRC patients was significantly upregulated compared to patients with benign enteral illness and hematopoietic cytokines (HCs). The hsa-circ-0004771 expression level in CRC patients was 14 times higher than that of HCs, and it was closely related to stage and distal metastasis. The sensitivity and specificity of hsa-circ-0004771 to differentiate CRC patients from HCs were 80.91% and 82.86%, respectively. These results indicated that the exosomal hsa-circ-0004771 overexpression was the source of tumors and can be used as biomarkers for CRC diagnosis. The expression level of circ_0000285 in sera of 150 patients with nasopharyngeal carcinoma was elevated compared to a healthy control; this level was correlated with the tumor size, degree of differentiation, lymph node metastasis, distal metastasis, and the TNM stage (66). Moreover, the expression level increased by more than three times in radiotherapy-resistant patients. Li et al. (67) detected high expression of circ-PDE8A in liver metastatic pancreatic ductal adenocarcinoma (PDAC) tissues, confirming that this feature is closely related to lymphatic infiltration and TNM stage. Circ-PDE8A is an independent risk factor affecting the survival of PDAC patients; further study verified the presence of circ-PDE8A-rich exosomes secreted by tumor cells in the plasma of PDAC patients. Similarly, circ-PDE8A in plasma exosomes may also be a diagnostic and prognostic marker of PDAC.
In addition to plasma, serum, and exosome, circRNAs were also found in some other body fluids such as saliva (47, 68, 69), gastric juice (70), and urine (44). Saliva is a body fluid used in disease research due to its convenient and non-invasive sampling method. When compared with healthy controls, the saliva of oral squamous cell carcinoma (OSCC) patients showed increased levels of hsa_circ_0001874 and hsa_circ_0001971 (69). Clinical data results associated salivary hsa_circ_0001874 with TNM stage and tumor grade, and hsa_circ_0001971 with TNM stage. Taken together, hsa_circ_0001874 and hsa_circ_0001971 display the AUC value to be 0.922. These results revealed the possibility of salivary hsa_circ_0001874 and hsa_circ_0001971 as the OSCC diagnostic biomarkers. Bahn et al. (47) detected and confirmed the presence of circRNAs in cell-free saliva, providing a new direction for using circRNAs as biomarkers. Gastric juice examination is a highly organ-specific test for the diagnosis of gastric diseases. Shao et al. discussed the feasibility of hsa_circ_0014717 in gastric juice as a biomarker for screening high-risk GC patients (70). The expression of hsa_circ_0014717 in gastric juice of 38 healthy individuals, 30 gastric ulcer patients, 15 chronic atrophic gastritis patients, and 39 GC patients was detected by qRT-PCR. Hsa_circ_0014717 was significantly downregulated in patients with chronic atrophic gastritis than the healthy control, indicating that hsa_circ_0014717 in gastric juice can be used as a biomarker for screening high-risk GC groups. Additionally, Chen et al. detected high expression of circPRMT5 in exosomes isolated from the urine samples of patients with UCB (44). Additional studies are listed in Table 1.
CircRNAs have also been used to evaluate the effectiveness of tumor-treatment methods and supervise tumor stress. For instance, Xie et al. (70) demonstrated an abundant expression of circSHKBP1 (hsa_circ_0000936) in GC serum and linked the abundance with the late TNM stage and low survival rates. The exosomal circSHKBP1 level metastasized early after gastrectomy was significantly reduced. Notably, the expression of circRNA reflects the clinical dynamics of sequential therapy and might offer a promising scheme for mirroring tumor stress and prognosis. The hsa_circ_0001649 expression levels in matched serum samples of patients with GC before and after surgery indicated that serum hsa_circ_0001649 expression levels in GC patients increase significantly after surgery. Also, the hsa_circ_0001649 expression in poorly differentiated and undifferentiated tumors was significantly lower than that in the well-differentiated tumors, indicating a negative correlation with the pathological differentiation of GC (60). The sensitivity and specificity of the receiver operating characteristic (ROC) curve were 0.711 and 0.816, respectively, and the AUC was 0.834. Therefore, hsa_circ_0001649 can be used as a blood-based biomarker to supervise illness progression and cure response. Ping et al. also found that compared to healthy people, circ_100053 expression in serum of patients with chronic myeloma leukemia (CML) was significantly upregulated. Additionally, the circ_100053 level in imatinib-resistant patients was almost the same as that of imatinib-sensitive patients, whereas it was four times in CML patients. Therefore, high circ_100053 expression predicts a poor prognosis and imatinib resistance in CML patients (93). Furthermore, circ-SORE mediated sorafenib resistance in HCC partially through its combination with YBX1 (98). There are also reports that hsa_circ_0005963 derived from extracellular vesicles induces CRC resistance through the miR-122-PKM2 pathway (99). Another study reported that has_circ_0035483 was highly expressed in RCC and promoted gemcitabine resistance by regulating the hsa-miR-355/CCNB1 axis. Reducing the expression of has_circ_0035483 can enhance gemcitabine sensitivity (100). A confirmed relationship between circRNA expression and drug sensitivity would guide the rational clinical use of drugs and improve patients’ prognosis.
Detection of CircRNAs in Body Fluids
The methods suitable for detecting circRNAs must distinguish circRNA from linear RNA species and be sensitive enough to allow reliable quantification of the closed-loop structure of circRNAs. The currently available technologies for circRNA assay are far from perfect (Figure 2) and fail to meet modern research demands. The qRT-PCR methods the most widely used technique for circRNA analysis, but it cannot distinguish between circRNAs and linear RNAs when the linear genome is used as a template to design the primer. Microarray technology is an effective and relatively sensitive technology to test and investigate circRNAs (99), but it can test only known circRNAs. As for traditional RNA sequencing (RNA-seq), another widely used method in circRNA research, the sequence reads spanning backsplicing junction sites of circRNA are filtered out in standard bioinformatics pipelines (34). The head-to-tail junction is the only instant proof of a circRNA. The testing efficiency is relatively low as only 0.1% of RNA-seq can contain this peculiar bond, and only part of circRNAs can be detected in one specimen (21). Besides, the next-generation sequencing (NGS) has other defects in circRNA detection, especially the incomplete capture of full-length circRNAs and the difficulty in accurate circRNA quantification. Although there are some strategies making up for the shortcomings, the short sequence obtained by NGS sequencing cannot be solved fundamentally. The third-generation high-throughput single-molecule sequencing technology has great advantages in the field of circular RNA research. Its long read-length eliminates the over-fragmentation characteristics of second-generation sequencing fundamentally, which brings hope about the detection of the full length of circRNA and accurate quantification. In addition, the biggest advantage of third-generation sequencing is that it can completely capture RNA transcript molecules. Unlike the NGS, which requires algorithms to splice, this allows us to see the complete sequence of the transcript rather than guessing by the algorithm. This ability guarantees the natural advantage of studying variable shear events. However, third-generation sequencing is inherently inadequate in the accuracy of single-base sequencing. Therefore, if single-base resolution studies are to be conducted, such as gene mutations, it is often necessary to perform multiple repetitive detections on the same location or combine with the second-generation sequencing for correction. The latest research strategies of third-generation sequencing technology in the circRNA field are isoCirc (101) and CIRI-long (102). They have optimized the detection technology based on conventional third-generation sequencing technology, which not only ensures the detection of the full-length circRNA detection, reduced low-accuracy defects, high detection rate, low expression levels of circRNA detection, and the advantages of mitochondrial circRNAs detection, thereby guaranteeing the future of circRNA research.
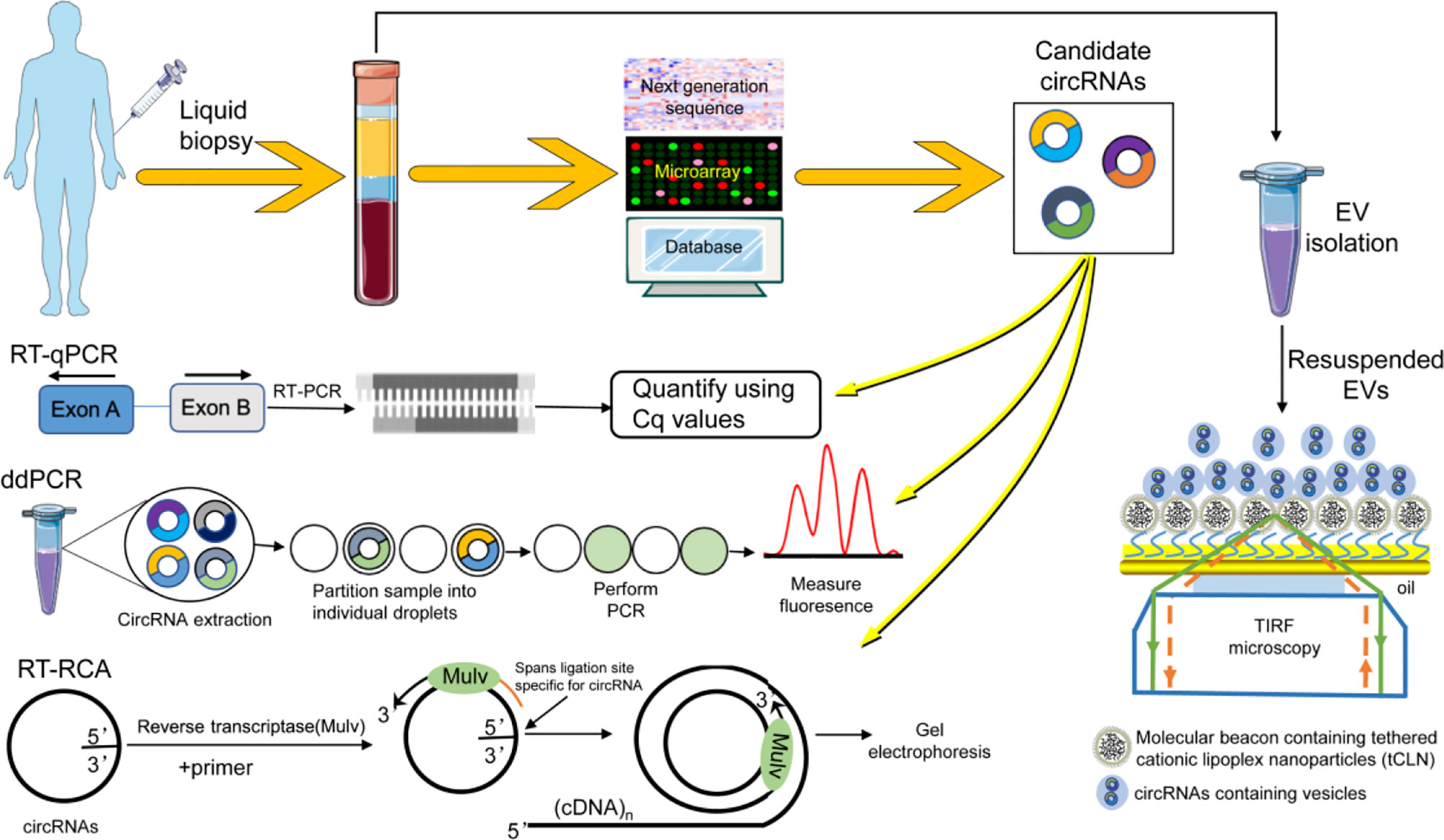
Figure 2 The detection method of circRNA in liquid biopsy. RNA-seq was used to identify and analyze candidate circRNAs with differential expression. Techniques such as RT-qPCR, ddPCR, RT-PCA, and tCLN were used to detect the level of circRNA in biological fluids.
Compared with the traditional circRNA detection methods, reverse transcription rolling circle amplification (RT-RCA) assay has the advantages of simple operation, low cost, and high sensitivity (103). It can even distinguish the circRNA from the linear RNA bearing the same sequence, which is superior to most methods such as qRT-PCR. Droplet digital polymerase chain reaction (ddPCR) is another novel nucleic acid testing method that dilutes template DNA into non-interacting droplets (104). However, these two methods also have shortcomings in large-scale multiplex detection of genes. Additionally, these technologies, such as qRT-PCR, microarray, and NGS, use RNA isolation procedures to mix the RNA released by the tumor cells with the circRNA from all other non-tumor cells. Therefore, these technologies only measure the total amount of circRNA in body fluids and cannot detect the circRNA from specific cells or vesica. Their clinical application is also limited because of their cumbersome and time-consuming procedures and high costs.
Cationic liposome nanoparticle (tCLN) biochips are advanced over the previous technologies. The positively charged chips trap the negatively charged exosomes in solution by attaching tCLN coated with a specific molecular probe to the chip. The molecular probes in nanoparticles can recognize specific substrates in exosomes and generate corresponding fluorescence signals. This method is simple and cost-effective, has good repeatability, and can effectively detect the differences between different exosomes compared to the currently available methods. Although it is mainly used to detect the microRNA (miRNA) or messenger RNA (mRNA) content in vesicles to predict cancer (105, 106), the technology can also be used for circRNA detection. However, this technique can only perform a qualitative RNA expression analysis and not perform a precise quantitative analysis. Moreover, a single circRNA test can predict cancers with a certain probability of missed detection. In the future, it may be necessary to use several circRNAs as a platform to jointly detect their expression and make diagnoses and predictions based on the comprehensive expression. In the light of insufficient detection methods discussed so far, further developing detection methods, development is required before an ideal circRNA detection method is found.
The Potential Application of CircRNAs in Anticancer Therapy
After years of clinical and basic research, RNA-based cancer therapy is booming, and various oligonucleotides have been approved for clinical trials, the most successful of which is the cancer therapy based on interfering RNA (siRNA) or antisense oligonucleotide (ASO). Earlier research data have confirmed that the siRNA can treat various solid tumors, including liver cancer, pancreatic cancer, lung cancer, prostate cancer, breast cancer, and ovarian cancer. Among them, two lipid carrier-based siRNA drugs ALN-VSP02 and TKM-080301 targeting the vascular endothelial growth and Polo-like kinase 1 (PLK1) gene for liver cancer treatment have completed phase I clinical trials (1). The polymer carrier-based siRNA implant siG12D LODER for treating pancreatic cancer has entered phase II clinical trials. Additionally, the nucleic acid drug Trabedersen (OT-101) based on ASO has been approved for the treatment of brain tumors treatment and is currently undergoing phase III clinical trials (107, 108). Although there is no preclinical report on using circRNAs alone as a therapeutic target, the strategy for targeting circRNAs is emerging rapidly from the laboratories.
Recently, several approaches have been developed to study circRNAs and target circRNAs for therapeutic purposes of cancer in vivo. The primary strategy is to intervene in cancer carcinogenesis, metastasis, apoptosis, and drug resistance by knocking down or overexpressing circRNAs, including circRNA knockdown strategies mediated by siRNA, short hairpin RNA (shRNA), ASO, and CRISPR/cas9 (109) (Figures 3A–D), the circRNA overexpression strategies mediated by vector and synthesis of circRNAs (110, 111), and circRNA delivery strategies mediated by exosome or nanoparticle (Figures 3E, F) (112, 113). Numerous studies have revealed that using siRNAs to target degradation or knock down the expression of circRNAs in vivo or in vitro is the most commonly used strategy for cancer therapy with circRNAs as the therapeutic target (114). Zhang et al. reported the successful knockdown of circNRIP1 by cholesterol-conjugated circNRIP1 siRNA in patient-derived xenograft (PDX) mouse models and reduced the PDX tumor growth via downstream metabolism alterations mediated by the AKT/mTOR signaling pathway (73). Wang et al. also demonstrated that silencing circHIPK3 through intratumorally injected si-circHIPK3 exhibits an inhibitory effect on CRC growth and metastasis in xenograft animal models (115). However, the siRNA-mediated circRNA knockdown strategies might have some obstacles, such as rapid degradation, delivery barriers, off-target effects, and immunogenicity. ASOs, DNA, or RNA molecules with a length of 8–50 nt can be an ideal alternative for siRNAs to knock down the circRNA expression by targeting intron sequences or reversing splice junctions and pre-mRNAs (116) (Figure 3C). Moreover, some ASOs that target circRNAs are also complementary to the intron-containing pre-mRNA; it is possible that these ASOs bind directly to pre-mRNA, leading to its degradation and causing reduced mRNA and circRNA expression. Therefore, careful selection of ASOs’ target sequence is necessary to avoid unwanted degradation of the parental RNA.
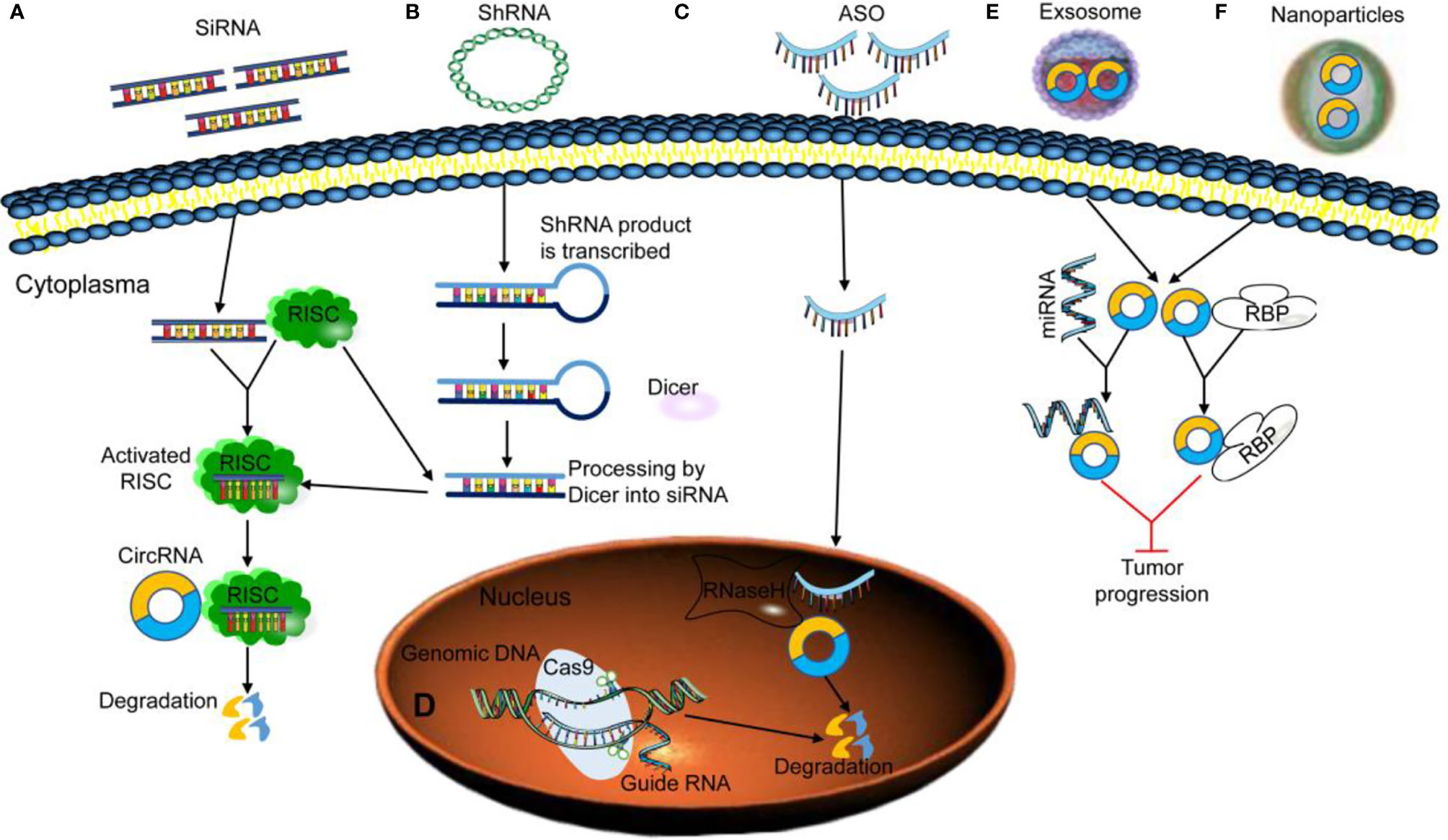
Figure 3 Strategies for targeting circRNAs. (A, B) Exogenous siRNA/shRNA activates RNA-induced silencing complex (RISC) and interacts with its specific target circRNA to induce degradation. (C) Exogenous antisense oligodeoxynucleotide (ASO) activates RNase H to induce circRNA to degradation. (D) Knockout of circRNA-associated genomic DNA using clustered regularly interspaced short palindromic repeats (CRISPR)/Cas9. (E, F) The loss of specific circRNAs can be rescued by reintroducing exosomal or nanoparticles’ circRNA into the cells as miRNA or RNA-binding protein (RBP) sponges to inhibit tumor progression.
CRISPR/Cas9 systems have lower mismatch tolerance and inhibit circRNAs more specifically than RNAi (Figure 3D). Rajewsky et al. (117) confirmed that a circRNA CircHIPK3, usually upregulated in liver cancer, could be targeted and inhibited by specially designed gRNAs of CRISPR/Cas9 in vitro; in subsequent mouse models, another circRNAs Cdr1as could be selectively knocked out by the CRISPR/Cas9 strategy. The success of this experiment confirmed the feasibility of CRISPR/Cas9 technology for circRNAs in vivo. However, the CRISPR-Cas9-mediated knockout may affect the parental mRNA expression. Recent research showed that some Cas9 enzyme variants could mediate RNA-dependent RNA degradation in a programmable and site-specific manner (118). The technology could be helpful to directly target circRNAs at their unique splice junction, thereby circumventing the non-specificity against the parental mRNA while providing better selectivity and safety. These studies have indicated that the CRISPR/Cas9 system may be one of the most promising therapeutic strategies for clinical cancer treatment with circRNAs as the therapeutic target.
Reintroducing circRNAs as a drug into cells and inhibiting the invasion, metastasis, drug resistance, and inducing apoptosis of cancer cells by overexpressing certain important circRNAs is another strategy for cancer treatment. Using a natural circRNA molecule to treat cancer has the advantage of mimicking the endogenous physiological situation. However, targeted delivery of circRNAs with specific functions to human cells is still difficult, especially for safe delivery in vivo (119). Commonly used circRNA overexpression strategies include plasmid vector delivery and viral vector delivery. The plasmid vector construction process is relatively simple; however, its application is restricted due to low transfection efficiency. Compared with plasmid vectors, the viral vectors have obvious advantages. One can achieve efficient and stable transduction of different cells and animals using different viral vector tools. However, the circRNA expression viral vectors could also generate unexpected side effects. Penaud-Budloo et al. observed that despite significant improvements in upstream and downstream processes, adeno-associated virus (AAV) stocks still contain significant impurities that should be minimized during production; otherwise, systemic administration of very high doses may result in immunotoxicity (120). Similarly, the strategy of vector delivery to achieve the circRNA overexpression should also confront the non-specific and potentially harmful effects caused by the by-products of wrong splicing. Highly purified circRNA molecules synthesized in vitro may be used to overcome these shortcomings. However, the artificially synthesized circRNAs are immunogenic, which may be due to the lack of the m6A modification of exogenous circRNAs. Additionally, exosomes can be used as stable natural transport carriers to deliver specific circular RNAs through tumor cell adsorption (Figure 3E). Chen et al. (121) found that exosomal circ-0051443 decreases the tumor growth through competitive binding to miR-331-3p and promotes BAK1 upregulation in vivo and in vitro. Zhang et al. (122) also found that exosomal CircGDI2 suppresses oral squamous cell carcinoma progression through the MiR-424-5p/SCAI axis regulation. Thus, exosomes may transport specific circRNAs to target tissue and play a prominent role in cancer intervention treatment.
Various studies have confirmed nanoparticles as good drug carriers that make significant application progress in the delivery of various clinical drugs (Figure 3F) (123). Zhao et al. found that nanoparticles loaded with circRNA SCAR successfully delivered circRNA SCAR to the mitochondria of hepatocyte cells in vivo, improving the glucose tolerance and insulin resistance of mice on a high-fat diet and alleviated nonalcoholic steatohepatitis (NASH) effectively (124). Additionally, Wu et al. (125) successfully used the circ-Foxo3 plasmid-PEG-gold nanoparticle complex to complete the delivery of circRNA to cancer cells. They also verified the effect of circ-RNAFoxo3 in inhibiting the tumor growth in vivo. The new gold nanoparticle carrier has a good potential for circular RNA delivery, although its biological safety remains to be studied.
Many obstacles still remain in the field of cancer treatment targeting circRNAs. However, in the coming years, with a better understanding of the functional mechanism of circRNAs and further development of methods targeting the specificity and effectiveness of circRNAs in vivo, the clinical potential of circRNA-based cancer treatment will be further explored. The broad selection (cancer or genetically well-defined rare diseases) and good therapeutic effects of RNA-targeted therapy have attracted widespread interest from academic research institutions and pharmaceutical companies (Orna Therapeutics); increased revenues from approved RNA therapies have led to increased investments in this field (126). Further research will only make liquid biopsy and therapeutic strategy with circRNAs an important tumor diagnosis and treatment method.
Challenges and Perspectives
Although circRNAs in body fluids are closely associated with cancer, their application as a great source of biomarkers for cancer diagnosis deserves further prospective verification. Biofluids are latent cancer biomarkers; nevertheless, there is still a wide gap between the discovery and clinical validation of biomarkers and also the clinical enforcement of these trials. Therefore, several hurdles must be overcome before circRNA biomarkers can be translated into clinical applications. One of the major concerns is that the methods for discovering and analyzing circRNAs are far from ideal, although enormous progress has been made in the past decades (127, 128). Future research need to gauge the analytical performance of disparate circRNA analysis in clinical specimens, such as RNA-seq, circRNA microarray, RT-qPCR, ddPCR, and tCLN (103, 128). Attention must be paid to standardizing in circRNA discovery and analysis when estimating the sensitivity and specificity (129). It must be noted that the circRNA biomarkers of biofluids in these studies (Table 1) represent only elementary features of biomarkers in cancers. The methods used in most of these studies are case–control studies on small samples with apparent phenotypes. Additional clinical specimens are needed to verify their sensitivity and specificity in a larger cohort, especially by identifying patients with similar clinical symptoms and translating them into clinical availability. Furthermore, their utility in illness diagnosis or prognostic prediction also requires detection in a prospective study. The greatest challenge in translating circRNA research from bench to bedside lies in the lack of a potentially designed and independently verified biomarker research. Further research is required to detect and verify the biomarkers’ availability in clinical medicine, such as their power to improve outcomes. The rapid and accurate detection of circRNAs from different tissues or fluids is also a significant obstacle to promote circRNAs in clinical liquid biopsy. Finally, linking the circRNA expression to other molecular changes obtained in cancer is also the goal of the follow-up research. Previous research has found that circRNA expression, KRAS, and EGFR mutations are associated with lung and colorectal cancer (130, 131). However, most published research has not studied this potential correlation. CircRNA-based cancer treatment strategies also face challenges about the choice of treatment vectors and treatment effects. In short, the circRNA research remains in its primary stages, and its effect on tumorigenesis has only begun to be illuminated. The discoveries till now make circRNA a prospective cancer biomarker and a potential target in cancer therapy. Although there are multiple challenges and questions to be faced and answered, the prospective possibility of translating the biofluid circRNA biomarkers into clinical applications gives us novel and hopeful options for liquid biopsy.
Data Availability Statement
The original contributions presented in the study are included in the article/supplementary material. Further inquiries can be directed to the corresponding author.
Author Contributions
YZ and YW conducted the literature review and drafted the manuscript. WL, XS, and PW revised the manuscript. All authors have read and agreed to the published version of the manuscript.
Funding
This work was supported by grants from the National Key R&D Program of China (2018YFC2000400) and the National Natural Science Foundation of China (81772270).
Conflict of Interest
The authors declare that the research was conducted in the absence of any commercial or financial relationships that could be construed as a potential conflict of interest.
Publisher’s Note
All claims expressed in this article are solely those of the authors and do not necessarily represent those of their affiliated organizations, or those of the publisher, the editors and the reviewers. Any product that may be evaluated in this article, or claim that may be made by its manufacturer, is not guaranteed or endorsed by the publisher.
Acknowledgments
We thank Jicheng Wu and Xiaoxiong Zhao for critically constructive suggestion.
References
1. Titze-de-Almeida R, David C, Titze-de-Almeida SS. The Race of 10 Synthetic RNAi-Based Drugs to the Pharmaceutical Market. Pharm Res (2017) 34:1339–63. doi: 10.1007/s11095-017-2134-2
2. Reckamp KL, Melnikova VO, Karlovich C, Sequist LV, Gadgeel SA. Highly Sensitive and Quantitative Test Platform for Detection of NSCLC EGFR Mutations in Urine and Plasma. J Thorac Oncol (2016) 11(10):1690–700. doi: 10.1016/j.jtho.2016.05.035
3. Wang Y, Springer S, Mulvey CL, Silliman N, Schaefer J, Sausen M, et al. Detection of Somatic Mutations and HPV in the Saliva and Plasma of Patients With Head and Neck Squamous Cell Carcinomas. Sci Transl Med (2015) 7:293ra104. doi: 10.1126/scitranslmed.aaa8507
4. Kimura H, Fujiwara Y, Sone T, Kunitoh H, Tamura T, Kasahara K, et al. EGFR Mutation Status in Tumour-Derived DNA From Pleural Effusion Fluid is a Practical Basis for Predicting the Response to Gefitinib. Br J Cancer (2006) 95(10):1390–5. doi: 10.1038/sj.bjc.6603428
5. De Mattos-Arruda L, Mayor R, Ng CKY, Weigelt B, Martinez-Ricarte F, Torrejon D, et al. Cerebrospinal Fluid-Derived Circulating Tumour DNA Better Represents the Genomic Alterations of Brain Tumours Than Plasma. Nat Commun (2015) 6:8839. doi: 10.1038/ncomms9839
6. Diehl F, Schmidt K, Durkee KH, Moore KJ, Goodman SN, Shuber AP, et al. Analysis of Mutations in DNA Isolated From Plasma and Stool of Colorectal Cancer Patients. Gastroenterology (2008) 135:489–498.e487. doi: 10.1053/j.gastro.2008.05.039
7. Heitzer E, Haque IS, Roberts CES, Speicher MR. Current and Future Perspectives of Liquid Biopsies in Genomics-Driven Oncology. Nat Rev Genet (2019) 20:71–88. doi: 10.1038/s41576-018-0071-5
8. Siravegna G, Marsoni S, Siena S, Bardelli A. Integrating Liquid Biopsies Into the Management of Cancer. Nat Rev Clin Oncol (2017) 14:531–48. doi: 10.1038/nrclinonc.2017.14
9. Mattox AK, Bettegowda C, Zhou S, Papadopoulos N, Kinzler KW, Vogelstein B. Applications of Liquid Biopsies for Cancer. Sci Transl Med (2019) 11(507):eaay1984. doi: 10.1126/scitranslmed.aay1984
10. De Rubis G, Rajeev Krishnan S, Bebawy M. Liquid Biopsies in Cancer Diagnosis, Monitoring, and Prognosis. Trends Pharmacol Sci (2019) 40:172–86. doi: 10.1016/j.tips.2019.01.006
11. Yu M, Bardia A, Wittner BS, Stott SL, Smas ME, Ting DT, et al. Circulating Breast Tumor Cells Exhibit Dynamic Changes in Epithelial and Mesenchymal Composition. Science (2013) 339:580–4. doi: 10.1126/science.1228522
12. Torrano V, Royo F, Peinado H, Loizaga-Iriarte A, Unda M, Falcon-Perez JM, et al. Vesicle-MaNiA: Extracellular Vesicles in Liquid Biopsy and Cancer. Curr Opin Pharmacol (2016) 29:47–53. doi: 10.1016/j.coph.2016.06.003
13. Zaporozhchenko IA, Ponomaryova AA, Rykova EY, Laktionov PP. The Potential of Circulating Cell-Free RNA as a Cancer Biomarker: Challenges and Opportunities. Expert Rev Mol Diagn (2018) 18:133–45. doi: 10.1080/14737159.2018.1425143
14. Surinova S, Radova L, Choi M, Srovnal J, Brenner H, Vitek O, et al. Non-Invasive Prognostic Protein Biomarker Signatures Associated With Colorectal Cancer. EMBO Mol Med (2015) 7:1153–65. doi: 10.15252/emmm.201404874
15. Crutchfield CA, Thomas SN, Sokoll LJ, Chan DW. Advances in Mass Spectrometry-Based Clinical Biomarker Discovery. Clin Proteomics (2016) 13:1. doi: 10.1186/s12014-015-9102-9
16. Best MG, Vancura A, Wurdinger T. Platelet RNA as a Circulating Biomarker Trove for Cancer Diagnostics. J Thromb Haemost (2017) 15(7):1295–306. doi: 10.1111/jth.13720
17. Sole C, Arnaiz E, Manterola L, Otaegui D, Lawrie CH. The Circulating Transcriptome as a Source of Cancer Liquid Biopsy Biomarkers. Semin Cancer Biol (2019) 58:100–8. doi: 10.1016/j.semcancer.2019.01.003
18. Wang Y, Wang Z. Efficient Backsplicing Produces Translatable Circular mRNAs. Rna-a Publ RNA Soc (2015) 21:172–9. doi: 10.1261/rna.048272.114
19. Sanger HL, Klotz G, Riesner D, Gross HJ, Kleinschmidt AK. Viroids are Single-Stranded Covalently Closed Circular RNA Molecules Existing as Highly Base-Paired Rod-Like Structures. Proc Natl Acad Sci U S A (1976) 73:3852–6. doi: 10.1073/pnas.73.11.3852
20. Memczak S, Jens M, Elefsinioti A, Torti F, Krueger J, Rybak A, et al. Circular RNAs are a Large Class of Animal RNAs With Regulatory Potency. Nature (2013) 495:333–8. doi: 10.1038/nature11928
21. Salzman J, Chen RE, Olsen MN, Wang PL, Brown PO. Cell-Type Specific Features of Circular RNA Expression. PloS Genet (2013) 9:e1003777. doi: 10.1371/journal.pgen.1003777
22. Zhang Y, Zhang XO, Chen T, Xiang JF, Yin QF, Xing YH, et al. Circular Intronic Long Noncoding RNAs. Mol Cell (2013) 51:792–806. doi: 10.1016/j.molcel.2013.08.017
23. Wang Y, Zhang Y, Wang P, Fu X, Lin W. Circular RNAs in Renal Cell Carcinoma: Implications for Tumorigenesis, Diagnosis, and Therapy. Mol Cancer (2020) 19:149. doi: 10.1186/s12943-020-01266-7
24. Enuka Y, Lauriola M, Feldman ME, Sas-Chen A, Ulitsky I, Yarden Y. Circular RNAs are Long-Lived and Display Only Minimal Early Alterations in Response to a Growth Factor. Nucleic Acids Res (2016) 44:1370–83. doi: 10.1093/nar/gkv1367
25. Jeck WR, Sorrentino JA, Wang K, Slevin MK, Burd CE, Liu J, et al. Circular RNAs are Abundant, Conserved, and Associated With ALU Repeats. RNA (2013) 19:141–57. doi: 10.1261/rna.035667.112
26. Zhou T, Xie X, Li M, Shi J, Zhou JJ, Knox KS, et al. Rat BodyMap Transcriptomes Reveal Unique Circular RNA Features Across Tissue Types and Developmental Stages. RNA (2018) 24:1443–56. doi: 10.1261/rna.067132.118
27. Zhang Z, Yang T, Xiao J. Circular RNAs: Promising Biomarkers for Human Diseases. EBioMedicine (2018) 34:267–74. doi: 10.1016/j.ebiom.2018.07.036
28. Vargas AJ, Harris CC. Biomarker Development in the Precision Medicine Era: Lung Cancer as a Case Study. Nat Rev Cancer (2016) 16:525–37. doi: 10.1038/nrc.2016.56
29. Song M, Xia L, Sun M, Yang C, Wang F. Circular RNA in Liver: Health and Diseases. Adv Exp Med Biol (2018) 1087:245–57. doi: 10.1007/978-981-13-1426-1_20
30. Altesha MA, Ni T, Khan A, Liu K, Zheng X. Circular RNA in Cardiovascular Disease. J Cell Physiol (2019) 234:5588–600. doi: 10.1002/jcp.27384
31. Arnaiz E, Sole C, Manterola L, Iparraguirre L, Otaegui D, Lawrie CH. CircRNAs and Cancer: Biomarkers and Master Regulators. Semin Cancer Biol (2019) 58:90–9. doi: 10.1016/j.semcancer.2018.12.002
32. Fang Y, Wang X, Li W, Han J, Jin J, Su F, et al. Screening of Circular RNAs and Validation of Circankrd36 Associated With Inflammation in Patients With Type 2 Diabetes Mellitus. Int J Mol Med (2018) 42:1865–74. doi: 10.3892/ijmm.2018.3783
33. Hanan M, Soreq H, Kadener S. CircRNAs in the Brain. RNA Biol (2017) 14:1028–34. doi: 10.1080/15476286.2016.1255398
34. Kristensen LS, Hansen TB, Veno MT, Kjems J. Circular RNAs in Cancer: Opportunities and Challenges in the Field. Oncogene (2018) 37:555–65. doi: 10.1038/onc.2017.361
35. Fernandez PL, Hernandez L, Farre X, Campo E, Cardesa A. Alterations of Cell Cycle-Regulatory Genes in Prostate Cancer. Pathobiology (2002) 70:1–10. doi: 10.1159/000065998
36. Xue J, Liu Y, Luo F, Lu X, Xu H, Liu X, et al. Circ100284, via miR-217 Regulation of EZH2, is Involved in the Arsenite-Accelerated Cell Cycle of Human Keratinocytes in Carcinogenesis. BBA-Mol Basis. Dis (2017) 1863:753–63. doi: 10.1016/j.bbadis.2016.12.018
37. Lu L, Luo F, Liu Y, Liu X, Shi L, Lu X, et al. Posttranscriptional Silencing of the lncRNA MALAT1 by miR-217 Inhibits the Epithelial-Mesenchymal Transition via Enhancer of Zeste Homolog 2 in the Malignant Transformation of HBE Cells Induced by Cigarette Smoke Extract. Toxicol Appl Pharmacol (2015) 289:276–85. doi: 10.1016/j.taap.2015.09.016
38. Chen DL, Zhang DS, Lu YX, Chen LZ, Zeng ZL, He MM, et al. microRNA-217 Inhibits Tumor Progression and Metastasis by Downregulating EZH2 and Predicts Favorable Prognosis in Gastric Cancer. Oncotarget (2015) 6:10868–79. doi: 10.18632/oncotarget.3451
39. Zhang N, Nan A, Chen L, Li X, Jia Y, Qiu M, et al. Circular RNA Circsatb2 Promotes Progression of non-Small Cell Lung Cancer Cells. Mol Cancer (2020) 19:101. doi: 10.1186/s12943-020-01221-6
40. Tian L, Cao J, Jiao H, Zhang J, Ren X, Liu X, et al. CircRASSF2 Promotes Laryngeal Squamous Cell Carcinoma Progression by Regulating the miR-302b-3p/IGF-1R Axis. Clin Sci (Lond) (2019) 133:1053–66. doi: 10.1042/CS20190110
41. Lu J, Wang YH, Yoon C, Huang XY, Xu Y, Xie JW, et al. Circular RNA Circ-RanGAP1 Regulates VEGFA Expression by Targeting miR-877-3p to Facilitate Gastric Cancer Invasion and Metastasis. Cancer Lett (2020) 471:38–48. doi: 10.1016/j.canlet.2019.11.038
42. Yang H, Zhang H, Yang Y, Wang X, Deng T, Liu R, et al. Hypoxia Induced Exosomal circRNA Promotes Metastasis of Colorectal Cancer via Targeting GEF-H1/RhoA Axis. Theranostics (2020) 10:8211–26. doi: 10.7150/thno.44419
43. Huang XY, Huang ZL, Huang J, Xu B, Huang XY, Xu YH, et al. Exosomal circRNA-100338 Promotes Hepatocellular Carcinoma Metastasis via Enhancing Invasiveness and Angiogenesis. J Exp Clin Cancer Res (2020) 39:20. doi: 10.1186/s13046-020-1529-9
44. Chen X, Chen RX, Wei WS, Li YH, Feng ZH, Tan L, et al. PRMT5 Circular RNA Promotes Metastasis of Urothelial Carcinoma of the Bladder Through Sponging miR-30c to Induce Epithelial-Mesenchymal Transition. Clin Cancer Res (2018) 24:6319–30. doi: 10.1158/1078-0432.CCR-18-1270
45. Byron SA, Van Keuren-Jensen KR, Engelthaler DM, Carpten JD, Craig DW. Translating RNA Sequencing Into Clinical Diagnostics: Opportunities and Challenges. Nat Rev Genet (2016) 17:257–71. doi: 10.1038/nrg.2016.10
46. Li Y, Zheng Q, Bao C, Li S, Guo W, Zhao J, et al. Circular RNA is Enriched and Stable in Exosomes: A Promising Biomarker for Cancer Diagnosis. Cell Res (2015) 25:981–4. doi: 10.1038/cr.2015.82
47. Bahn JH, Zhang Q, Li F, Chan TM, Lin X, Kim Y, et al. The Landscape of microRNA, Piwi-Interacting RNA, and Circular RNA in Human Saliva. Clin Chem (2015) 61:221–30. doi: 10.1373/clinchem.2014.230433
48. Memczak S, Papavasileiou P, Peters O, Rajewsky N. Identification and Characterization of Circular RNAs As a New Class of Putative Biomarkers in Human Blood. PloS One (2015) 10:e0141214. doi: 10.1371/journal.pone.0141214
49. Zhang X, Yuan X, Shi H, Wu L, Qian H, Xu W. Exosomes in Cancer: Small Particle, Big Player. J Hematol Oncol (2015) 8:83. doi: 10.1186/s13045-015-0181-x
50. Li P, Chen S, Chen H, Mo X, Li T, Shao Y, et al. Using Circular RNA as a Novel Type of Biomarker in the Screening of Gastric Cancer. Clin Chim Acta (2015) 444:132–6. doi: 10.1016/j.cca.2015.02.018
51. Meng S, Zhou H, Feng Z, Xu Z, Tang Y, Li P, et al. CircRNA: Functions and Properties of a Novel Potential Biomarker for Cancer. Mol Cancer (2017) 16:94. doi: 10.1186/s12943-017-0663-2
52. Su M, Xiao Y, Ma J, Tang Y, Tian B, Zhang Y, et al. Circular RNAs in Cancer: Emerging Functions in Hallmarks, Stemness, Resistance and Roles as Potential Biomarkers. Mol Cancer (2019) 18(1):90. doi: 10.1186/s12943-019-1002-6
53. Sheng R, Li X, Wang Z, Wang X. Response to Comments on Circular RNAs and Their Emerging Roles as Diagnostic and Prognostic Biomarkers in Ovarian Cancer. Cancer Lett (2020) 476:183–4. doi: 10.1016/j.canlet.2020.02.006
54. Yu J, Ding WB, Wang MC, Guo XG, Xu J, Xu QG, et al. Plasma Circular RNA Panel to Diagnose Hepatitis B Virus-Related Hepatocellular Carcinoma: A Large-Scale, Multicenter Study. Int J Cancer (2020) 146:1754–63. doi: 10.1002/ijc.32647
55. Lin J, Cai D, Li W, Yu T, Mao H, Jiang S, et al. Plasma Circular RNA Panel Acts as a Novel Diagnostic Biomarker for Colorectal Cancer. Clin Biochem (2019) 74:60–8. doi: 10.1016/j.clinbiochem.2019.10.012
56. Li T, Shao Y, Fu L, Xie Y, Zhu L, Sun W, et al. Plasma Circular RNA Profiling of Patients With Gastric Cancer and Their Droplet Digital RT-PCR Detection. J Mol Med (2017) 96:85. doi: 10.1007/s00109-017-1600-y
57. Huang M, He YR, Liang LC, Huang Q, Zhu ZQ. Circular RNA Hsa_Circ_0000745 may Serve as a Diagnostic Marker for Gastric Cancer. World J Gastroenterol (2017) 23:6330–8. doi: 10.3748/wjg.v23.i34.6330
58. Sun H, Tang W, Rong D, Jin H, Fu K, Zhang W, et al. Hsa_circ_0000520, a Potential New Circular RNA Biomarker, Is Involved in Gastric Carcinoma. Cancer Biomark (2018) 21:299–306. doi: 10.3233/CBM-170379
59. Chen S, Li T, Zhao Q, Xiao B, Guo J. Using Circular RNA Hsa_Circ_0000190 as a New Biomarker in the Diagnosis of Gastric Cancer. Clin Chim Acta (2017) 466:167–71. doi: 10.1016/j.cca.2017.01.025
60. Li WH, Song YC, Zhang H, Zhou ZJ, Xie X, Zeng QN, et al. Decreased Expression of Hsa_circ_00001649 in Gastric Cancer and Its Clinical Significance. Dis Markers (2017) 2017:4587698. doi: 10.1155/2017/4587698
61. Zhao Q, Chen S, Li T, Xiao B, Zhang X. Clinical Values of Circular RNA 0000181 in the Screening of Gastric Cancer. J Clin Lab Anal (2018) 32:e22333. doi: 10.1002/jcla.22333
62. Tan S, Gou Q, Pu W, Guo C, Yang Y, Wu K, et al. Circular RNA F-circEA Produced From EML4-ALK Fusion Gene as a Novel Liquid Biopsy Biomarker for Non-Small Cell Lung Cancer. Cell Res (2018) 28:693–5. doi: 10.1038/s41422-018-0033-7
63. Tang W, Fu K, Sun H, Rong D, Wang H, Cao H. CircRNA Microarray Profiling Identifies a Novel Circulating Biomarker for Detection of Gastric Cancer. Mol Cancer (2018) 17:137. doi: 10.1186/s12943-018-0888-8
64. Lu J, Zhang PY, Xie JW, Wang JB, Lin JX, Chen QY, et al. Hsa_circ_0000467 Promotes Cancer Progression and Serves as a Diagnostic and Prognostic Biomarker for Gastric Cancer. J Clin Lab Anal (2019) 33:e22726. doi: 10.1002/jcla.22726
65. Pan B, Qin J, Liu X, He B, Wang X, Pan Y, et al. Identification of Serum Exosomal Hsa-Circ-0004771 as a Novel Diagnostic Biomarker of Colorectal Cancer. Front Genet (2019) 10:1096. doi: 10.3389/fgene.2019.01096
66. Shuai M, Hong J, Huang D, Zhang X, Tian Y. Upregulation of circRNA_0000285 Serves as a Prognostic Biomarker for Nasopharyngeal Carcinoma and is Involved in Radiosensitivity. Oncol Lett (2018) 16:6495–501. doi: 10.3892/ol.2018.9471
67. Li Z, Yanfang W, Li J, Jiang P, Peng T, Chen K, et al. Tumor-Released Exosomal Circular RNA PDE8A Promotes Invasive Growth via the miR-338/MACC1/MET Pathway in Pancreatic Cancer. Cancer Lett (2018) 432:237–50. doi: 10.1016/j.canlet.2018.04.035
68. Jafari Ghods F. Circular RNA in Saliva. Adv Exp Med Biol (2018) 1087:131–9. doi: 10.1007/978-981-13-1426-1_11
69. Zhao SY, Wang J, Ouyang SB, Huang ZK, Liao L. Salivary Circular RNAs Hsa_Circ_0001874 and Hsa_Circ_0001971 as Novel Biomarkers for the Diagnosis of Oral Squamous Cell Carcinoma. Cell Physiol Biochem (2018) 47:2511–21. doi: 10.1159/000491624
70. Shao Y, Li J, Lu R, Li T, Yang Y, Xiao B, et al. Global Circular RNA Expression Profile of Human Gastric Cancer and its Clinical Significance. Cancer Med (2017) 6:1173–80. doi: 10.1002/cam4.1055
71. Li Q, Shao Y, Zhang X, Zheng T, Miao M, Qin L, et al. Plasma Long Noncoding RNA Protected by Exosomes as a Potential Stable Biomarker for Gastric Cancer. Tumour Biol (2015) 36:2007–12. doi: 10.1007/s13277-014-2807-y
72. Kong S, Yang Q, Tang C, Wang T, Shen X, Ju S. Identification of Hsa_Circ_0001821 as a Novel Diagnostic Biomarker in Gastric Cancer via Comprehensive Circular RNA Profiling. Front Genet (2019) 10:878. doi: 10.3389/fgene.2019.00878
73. Zhang X, Wang S, Wang H, Cao J, Huang X, Chen Z, et al. Circular RNA Circnrip1 Acts as a microRNA-149-5p Sponge to Promote Gastric Cancer Progression via the AKT1/mTOR Pathway. Mol Cancer (2019) 18:20. doi: 10.1186/s12943-018-0935-5
74. Zhu X, Wang X, Wei S, Chen Y, Chen Y, Fan X, et al. Hsa_Circ_0013958: A Circular RNA and Potential Novel Biomarker for Lung Adenocarcinoma. FEBS J (2017) 284:2170–82. doi: 10.1111/febs.14132
75. Luo YH, Yang YP, Chien CS, Yarmishyn AA, Ishola AA, Chien Y, et al. Plasma Level of Circular RNA Hsa_Circ_0000190 Correlates With Tumor Progression and Poor Treatment Response in Advanced Lung Cancers. Cancers (Basel) (2020) 12(7):1740. doi: 10.3390/cancers12071740
76. Fan CM, Wang JP, Tang YY, Zhao J, He SY, Xiong F, et al. Circman1a2 Could Serve as a Novel Serum Biomarker for Malignant Tumors. Cancer Sci (2019) 110:2180–8. doi: 10.1111/cas.14034
77. Zhou X, Liu HY, Wang WY, Zhao H, Wang T. Hsa_circ_0102533 Serves as a Blood-Based Biomarker for Non-Small-Cell Lung Cancer Diagnosis and Regulates Apoptosis In Vitro. Int J Clin Exp Pathol (2018) 11:4395–404.
78. Hang D, Zhou J, Qin N, Zhou W, Ma H, Jin G, et al. A Novel Plasma Circular RNA circFARSA is a Potential Biomarker for Non-Small Cell Lung Cancer. Cancer Med (2018) 7:2783–91. doi: 10.1002/cam4.1514
79. Li L, Li W, Chen N, Zhao H, Xu G, Zhao Y, et al. FLI1 Exonic Circular RNAs as a Novel Oncogenic Driver to Promote Tumor Metastasis in Small Cell Lung Cancer. Clin Cancer Res (2019) 25:1302–17. doi: 10.1158/1078-0432.CCR-18-1447
80. Yang F, Liu DY, Guo JT, Ge N, Zhu P, Liu X, et al. Circular RNA Circ-LDLRAD3 as a Biomarker in Diagnosis of Pancreatic Cancer. World J Gastroenterol (2017) 23:8345–54. doi: 10.3748/wjg.v23.i47.8345
81. Zhang X, Zhou H, Jing W, Luo P, Qiu S, Liu X, et al. The Circular RNA Hsa_Circ_0001445 Regulates the Proliferation and Migration of Hepatocellular Carcinoma and May Serve as a Diagnostic Biomarker. Dis Markers (2018) 2018:3073467. doi: 10.1155/2018/3073467
82. Wang G, Liu W, Zou Y, Wang G, Deng Y, Luo J, et al. Three Isoforms of Exosomal Circptgr1 Promote Hepatocellular Carcinoma Metastasis via the Mir449a-MET Pathway. EBioMedicine (2019) 40:432–45. doi: 10.1016/j.ebiom.2018.12.062
83. Lei B, Zhou J, Xuan X, Tian Z, Zhang M, Gao W, et al. Circular RNA Expression Profiles of Peripheral Blood Mononuclear Cells in Hepatocellular Carcinoma Patients by Sequence Analysis. Cancer Med (2019) 8:1423–33. doi: 10.1002/cam4.2010
84. Huang XY, Huang ZL, Zhang PB, Huang XY, Huang J, Wang HC, et al. CircRNA-100338 Is Associated With mTOR Signaling Pathway and Poor Prognosis in Hepatocellular Carcinoma. Front Oncol (2019) 9:392. doi: 10.3389/fonc.2019.00392
85. Li Y, Zang H, Zhang X, Huang G. Exosomal Circ-ZNF652 Promotes Cell Proliferation, Migration, Invasion and Glycolysis in Hepatocellular Carcinoma via miR-29a-3p/GUCD1 Axis. Cancer Manage Res (2020) 12:7739–51. doi: 10.2147/CMAR.S259424
86. Yin WB, Yan MG, Fang X, Guo JJ, Xiong W, Zhang RP. Circulating Circular RNA Hsa_Circ_0001785 Acts as a Diagnostic Biomarker for Breast Cancer Detection. Clin Chim Acta (2018) 487:363–8. doi: 10.1016/j.cca.2017.10.011
87. Li Y, Li C, Xu R, Wang Y, Li D, Zhang B. A Novel Circfmn2 Promotes Tumor Proliferation in CRC by Regulating the miR-1182/hTERT Signaling Pathways. Clin Sci (Lond) (2019) 133:2463–79. doi: 10.1042/CS20190715
88. Feng W, Gong H, Wang Y, Zhu G, Xue T, Wang Y, et al. Circift80 Functions as a ceRNA of miR-1236-3p to Promote Colorectal Cancer Progression. Mol Ther.-Nucleic Acids (2019) 18:375–87. doi: 10.1016/j.omtn.2019.08.024
89. Hon KW, Ab-Mutalib NS, Abdullah NMA, Jamal R, Abu N. Extracellular Vesicle-Derived Circular RNAs Confers Chemoresistance in Colorectal Cancer. Sci Rep (2019) 9:16497. doi: 10.1038/s41598-019-53063-y
90. Li XN, Wang ZJ, Ye CX, Zhao BC, Huang XX, Yang L. Circular RNA circVAPA is Up-Regulated and Exerts Oncogenic Properties by Sponging miR-101 in Colorectal Cancer. Biomed Pharmacother (2019) 112:108611. doi: 10.1016/j.biopha.2019.108611
91. Wang YM, Huang LM, Li DR, Shao JH, Xiong SL, Wang CM, et al. Hsa_circ_0101996 Combined With Hsa_Circ_0101119 in Peripheral Whole Blood can Serve as the Potential Biomarkers for Human Cervical Squamous Cell Carcinoma. Int J Clin Exp Pathol (2017) 10:11924–31.
92. Wei L, Chaoqin Z, Jun J, Peng L, Baoxia C, Chunyan J, et al. Characterization of Hsa_Circ_0004277 as a New Biomarker for Acute Myeloid Leukemia via Circular RNA Profile and Bioinformatics Analysis. Int J Mol Sci (2017) 18:597–. doi: 10.3390/ijms18030597
93. Ping L, Jian-Jun C, Chu-Shu L, Guang-Hua L, Ming Z. High Circ_100053 Predicts a Poor Outcome for Chronic Myeloid Leukemia and Is Involved in Imatinib Resistance. Oncol Res (2019). doi: 10.3727/096504018X15412701483326
94. Yang C, Wei Y, Yu L, Xiao Y. Identification of Altered Circular RNA Expression in Serum Exosomes From Patients With Papillary Thyroid Carcinoma by High-Throughput Sequencing. Med Sci Monit (2019) 25:2785–91. doi: 10.12659/MSM.915658
95. Zhu K, Niu L, Wang J, Wang Y, Zhou J, Wang F, et al. Circular RNA Hsa_Circ_0000885 Levels Are Increased in Tissue and Serum Samples From Patients With Osteosarcoma. Med Sci Monit (2019) 25:1499–505. doi: 10.12659/MSM.914899
96. Xu H, Gong Z, Shen Y, Fang Y, Zhong S. Circular RNA Expression in Extracellular Vesicles Isolated From Serum of Patients With Endometrial Cancer. Epigenomics (2018) 10:187–97. doi: 10.2217/epi-2017-0109
97. Li T, Sun X, Chen L. Exosome Circ_0044516 Promotes Prostate Cancer Cell Proliferation and Metastasis as a Potential Biomarker. J Cell Biochem (2020) 121:2118–26. doi: 10.1002/jcb.28239
98. Xu J, Ji L, Liang Y, Wan Z, Zheng W, Song X, et al. CircRNA-SORE Mediates Sorafenib Resistance in Hepatocellular Carcinoma by Stabilizing YBX1. Signal Transduction Tar (2020) 5:298. doi: 10.1038/s41392-020-00375-5
99. Wang X, Zhang H, Yang H, Bai M, Ning T, Deng T, et al. Exosome-Delivered circRNA Promotes Glycolysis to Induce Chemoresistance Through the miR-122-PKM2 Axis in Colorectal Cancer. Mol Oncol (2020) 14:539–55. doi: 10.1002/1878-0261.12629
100. Yan L, Liu G, Cao H, Zhang H, Shao F. Hsa_circ_0035483 Sponges hsa-miR-335 to Promote the Gemcitabine-Resistance of Human Renal Cancer Cells by Autophagy Regulation. Biochem Biophys Res Commun (2019) 519:172–8. doi: 10.1016/j.bbrc.2019.08.093
101. Xin R, Gao Y, Gao Y, Wang R, Kadash-Edmondson KE, Liu B, et al. Isocirc Catalogs Full-Length Circular RNA Isoforms in Human Transcriptomes. Nat Commun (2021) 12:266. doi: 10.1038/s41467-020-20459-8
102. Zhang J, Hou L, Zuo Z, Ji P, Zhang X, Xue Y, et al. Comprehensive Profiling of Circular RNAs With Nanopore Sequencing and CIRI-Long. Nat Biotechnol (2021) 39(7):836–45. doi: 10.1038/s41587-021-00842-6
103. Liu Y, Zhang X, Liu M, Xu F, Zhang Q, Zhang Y, et al. Direct Detection of circRNA in Real Samples Using Reverse Transcription-Rolling Circle Amplification. Anal Chim Acta (2020) 1101:169–75. doi: 10.1016/j.aca.2019.12.027
104. Sykes PJ, Neoh SH, Brisco MJ, Hughes E, Condon J, Morley AA. Quantitation of Targets for PCR by Use of Limiting Dilution. Biotechniques (1992) 13:444–9. doi: 10.3109/10520299209110042
105. Liu C, Kannisto E, Yu G, Yang Y, Reid ME, Patnaik SK, et al. Non-Invasive Detection of Exosomal MicroRNAs via Tethered Cationic Lipoplex Nanoparticles (tCLN) Biochip for Lung Cancer Early Detection. Front Genet (2020) 11:258. doi: 10.3389/fgene.2020.00258
106. Cho S, Yang HC, Rhee WJ. Simultaneous Multiplexed Detection of Exosomal microRNAs and Surface Proteins for Prostate Cancer Diagnosis. Biosens Bioelectron (2019) 146:111749. doi: 10.1016/j.bios.2019.111749
107. Ramot Y, Rotkopf S, Gabai RM, Zorde Khvalevsky E, Muravnik S, Marzoli GA, et al. Preclinical Safety Evaluation in Rats of a Polymeric Matrix Containing an siRNA Drug Used as a Local and Prolonged Delivery System for Pancreatic Cancer Therapy. Toxicol Pathol (2016) 44:856–65. doi: 10.1177/0192623316645860
108. D’Cruz OJ, Qazi S, Hwang L, Ng K, Trieu V. Impact of Targeting Transforming Growth Factor Beta-2 With Antisense OT-101 on the Cytokine and Chemokine Profile in Patients With Advanced Pancreatic Cancer. Onco Targets Ther (2018) 11:2779–96. doi: 10.2147/OTT.S161905
109. He AT, Liu J, Li F, Yang BB. Targeting Circular RNAs as a Therapeutic Approach: Current Strategies and Challenges. Signal Transduct Target Ther (2021) 6:185. doi: 10.1038/s41392-021-00569-5
110. Meganck RM, Borchardt EK, Castellanos Rivera RM, Scalabrino ML, Wilusz JE, Marzluff WF, et al. Tissue-Dependent Expression and Translation of Circular RNAs With Recombinant AAV Vectors In Vivo. Mol Ther Nucleic Acids (2018) 13:89–98. doi: 10.1016/j.omtn.2018.08.008
111. Chen YG, Chen R, Ahmad S, Verma R, Kasturi SP, Amaya L, et al. N6-Methyladenosine Modification Controls Circular RNA Immunity. Mol Cell (2019) 76:96–109.e109. doi: 10.1016/j.molcel.2019.07.016
112. Draz MS, Fang BA, Zhang P, Hu Z, Gu S, Weng KC, et al. Nanoparticle-Mediated Systemic Delivery of siRNA for Treatment of Cancers and Viral Infections. Theranostics (2014) 4:872–92. doi: 10.7150/thno.9404
113. Oliveira ACN, Fernandes J, Goncalves A, Gomes AC, Oliveira M. Lipid-Based Nanocarriers for siRNA Delivery: Challenges, Strategies and the Lessons Learned From the DODAX: MO Liposomal System. Curr Drug Targets (2019) 20:29–50. doi: 10.2174/1389450119666180703145410
114. Hsiao KY, Lin YC, Gupta SK, Chang N, Yen L, Sun HS, et al. Noncoding Effects of Circular RNA CCDC66 Promote Colon Cancer Growth and Metastasis. Cancer Res (2017) 77:2339–50. doi: 10.1158/0008-5472.Can-16-1883
115. Zeng K, Chen X, Xu M, Liu X, Hu X, Xu T, et al. CircHIPK3 Promotes Colorectal Cancer Growth and Metastasis by Sponging miR-7. Cell Death Dis (2018) 9:417. doi: 10.1038/s41419-018-0454-8
116. Li Z, Huang C, Bao C, Chen L, Lin M, Wang X, et al. Exon-Intron Circular RNAs Regulate Transcription in the Nucleus. Nat Struct Mol Biol (2015) 22:256–64. doi: 10.1038/nsmb.2959
117. Piwecka M, Glazar P, Hernandez-Miranda LR, Memczak S, Wolf SA, Rybak-Wolf A, et al. Loss of a Mammalian Circular RNA Locus Causes miRNA Deregulation and Affects Brain Function. Science (2017) 357(6357):eaam8526. doi: 10.1126/science.aam8526
118. Strutt SC, Torrez RM, Kaya E, Negrete OA, Doudna JA. RNA-Dependent RNA Targeting by CRISPR-Cas9. Elife (2018) 7:e32724. doi: 10.7554/eLife.32724
119. Vitiello M, Tuccoli A, Poliseno L. Long Non-Coding RNAs in Cancer: Implications for Personalized Therapy. Cell Oncol (Dordr) (2015) 38:17–28. doi: 10.1007/s13402-014-0180-x
120. Penaud-Budloo M, Francois A, Clement N, Ayuso E. Pharmacology of Recombinant Adeno-Associated Virus Production. Mol Ther Methods Clin Dev (2018) 8:166–80. doi: 10.1016/j.omtm.2018.01.002
121. Chen W, Quan Y, Fan S, Wang H, Liang J, Huang L, et al. Exosome-Transmitted Circular RNA Hsa_Circ_0051443 Suppresses Hepatocellular Carcinoma Progression. Cancer Lett (2020) 475:119–28. doi: 10.1016/j.canlet.2020.01.022
122. Zhang Y, Tang K, Chen L, Du M, Qu Z. Exosomal CircGDI2 Suppresses Oral Squamous Cell Carcinoma Progression Through the Regulation of MiR-424-5p/SCAI Axis. Cancer Manage Res (2020) 12:7501–14. doi: 10.2147/CMAR.S255687
123. Judge AD, Robbins M, Tavakoli I, Levi J, Hu L, Fronda A, et al. Confirming the RNAi-Mediated Mechanism of Action of siRNA-Based Cancer Therapeutics in Mice. J Clin Invest (2009) 119:661–73. doi: 10.1172/JCI37515
124. Zhao Q, Liu J, Deng H, Ma R, Liao JY, Liang H, et al. Targeting Mitochondria-Located circRNA SCAR Alleviates NASH via Reducing mROS Output. Cell (2020) 183:76–93 e22. doi: 10.1016/j.cell.2020.08.009
125. Du WW, Fang L, Yang W, Wu N, Awan FM, Yang Z, et al. Induction of Tumor Apoptosis Through a Circular RNA Enhancing Foxo3 Activity. Cell Death Differ (2017) 24:357–70. doi: 10.1038/cdd.2016.133
126. Wang F, Zuroske T, Watts JK. RNA Therapeutics on the Rise. Nat Rev Drug Discov (2020) 19:441–2. doi: 10.1038/d41573-020-00078-0
127. Szabo L, Salzman J. Detecting Circular RNAs: Bioinformatic and Experimental Challenges. Nat Rev Genet (2016) 17:679–92. doi: 10.1038/nrg.2016.114
128. Kristensen LS, Andersen MS, Stagsted LVW, Ebbesen KK, Hansen TB, Kjems J. The Biogenesis, Biology and Characterization of Circular RNAs. Nat Rev Genet (2019) 20:675–91. doi: 10.1038/s41576-019-0158-7
129. Hardwick SA, Deveson IW, Mercer TR. Reference Standards for Next-Generation Sequencing. Nat Rev Genet (2017) 18:473–84. doi: 10.1038/nrg.2017.44
130. Dou Y, Cha DJ, Franklin JL, Higginbotham JN, Jeppesen DK, Weaver AM, et al. Circular RNAs are Down-Regulated in KRAS Mutant Colon Cancer Cells and can be Transferred to Exosomes. Sci Rep (2016) 6:37982. doi: 10.1038/srep37982
Keywords: cancer diagnosis, liquid biopsy, circRNA, biomarker, exosome
Citation: Zhang Y, Wang Y, Su X, Wang P and Lin W (2021) The Value of Circulating Circular RNA in Cancer Diagnosis, Monitoring, Prognosis, and Guiding Treatment. Front. Oncol. 11:736546. doi: 10.3389/fonc.2021.736546
Received: 05 July 2021; Accepted: 23 September 2021;
Published: 14 October 2021.
Edited by:
Shanchun Guo, Xavier University of Louisiana, United StatesReviewed by:
Junyun Wang, Beijing Institute of Genomics (CAS), ChinaWuQiang Fan, Massachusetts General Hospital, United States
Copyright © 2021 Zhang, Wang, Su, Wang and Lin. This is an open-access article distributed under the terms of the Creative Commons Attribution License (CC BY). The use, distribution or reproduction in other forums is permitted, provided the original author(s) and the copyright owner(s) are credited and that the original publication in this journal is cited, in accordance with accepted academic practice. No use, distribution or reproduction is permitted which does not comply with these terms.
*Correspondence: Weiqiang Lin, wpyyy@126.com; Ping Wang, wpyyy@126.com
†These authors have contributed equally to this work