- 1Breast Cancer Department, MD Anderson Cancer Center, Madrid, Spain
- 2Centro de Investigación Biomédica en Red de Cáncer (CIBERONC), Madrid, Spain
- 3International Breast Cancer Center (IBCC), Barcelona, Spain
- 4Medical Scientia Innovation Research (MedSIR), Barcelona, Spain
- 5Vall d’Hebron Institute of Oncology, Barcelona, Spain
- 6Biochemistry Department, Universidad Autónoma de Madrid (UAM), Instituto de Investigaciones Biomédicas ‘Alberto Sols’ (CSIC-UAM), IdiPaz, & Centro de Investigación Biomédica en Red de Cáncer (CIBERONC), Madrid, Spain
- 7MD Anderson International Foundation, Madrid, Spain
Breast cancer is the most common tumor in women worldwide, and an increasing public health concern. Knowledge of both protective and negative risk factors is essential for a better understanding of this heterogenous disease. We undertook a review of the recent literature and evaluated the relationship between obesity mediators and breast cancer development depending on menopausal status. Excess weight is now pandemic and has replaced tobacco as the main lifestyle-related risk factor for premature death. Although the prevalence of obesity/overweight has increased globally over the last 50 years, the potential harm attributable to excess fat has generally been underestimated. The relationship between overweight/obesity, breast cancer and overall risk appears to be highly dependent on menopausal status. Thus, obesity increases the risk of breast cancer in postmenopausal women but, conversely, it appears to be protective in premenopausal women. We evaluate the role of different clinical factors potentially involved in this seemingly contradictory relationship, including estrogen, mammogram density, adipokines, insulin-signaling pathway activation, and inflammatory status. A key focus of this review is to better understand the impact of body mass index and menopausal status on these clinical factors and, hence, provide some clarity into the inter-relationships involved in this controversial issue.
Introduction
The incidence of obesity has nearly tripled since 1975 and it is now considered one of the worst pandemics of the 21th century. In 2016, more than 1.9 billion people aged over 18 years were considered to be overweight, and >650 million were obese; representing 39% and 13% of the adult global population, respectively (1). The prevalence of obesity varies widely by country; for example countries such as Vietnam (2.1%) and Japan (4.4%) reporting low rates compared with 37.3% in the United States and the highest rates in Oceania (Nauru, 61%; Cook Islands 55%; and Samoa/Tonga, both 46%) (1, 2). Except for some regions in sub-Saharan Africa and Asia, more people are now obese than underweight (1); and projected trends for the next 10 years are disheartening, with an estimated 3.3 billion (58%) of the world adult population predicted to be overweight or obese by 2030 (3).
Obesity and overweight are generally associated with physical inactivity and a sedentary lifestyle. A survey of nearly 2 million participants in 2016 revealed that over a quarter (27.5%) of the global adult population failed to get enough physical activity with the highest prevalence (43%) in women, particularly in high-income countries (4). Such figures represent a setback for the global target set by the World Health Organisation (WHO) member states to reduce physical inactivity by 10% by 2025.
Paradoxical Effects of Body Mass Index on Breast Cancer Risk in Relation to Menopausal Status
Overweight is defined as a body mass index (BMI >25 kg/m2, whereas obesity is categorised as a BMI >30 kg/m2 and is considered to be a chronic disease (5, 6). The association between breast cancer risk and excess weight (overweight/obese) varies according to menopausal status (Table 1), with independent studies reporting a negative correlation between obesity and risk in premenopausal women (9–11). Indeed, a pooled analysis of 7 studies including a total of 337,819 women (4,385 of whom had invasive breast cancer) reported a risk ratio (RR) of 0.54 [95% confidence interval (95% CI): 0.34-0.85] for breast cancer risk when comparing premenopausal women with BMI >31 kg/m2 versus those with a BMI of ≤21 kg/m2 (7). Likewise, a separate meta-analysis involving 9 studies also demonstrated an inverse correlation between obesity and per unit increase in BMI with regards breast cancer risk in premenopausal women [RR: 0.98; 95% CI: 0.97-0.99] (8). Similar findings were reported in an independent meta-analysis consisting of 34 datasets of women (a total of >2.5 million women) in which breast cancer risk was investigated relative to BMI (12). In total, breast cancer was reported in 7,930 premenopausal and 23,909 postmenopausal women and, overall, breast cancer risk was shown to be reduced by approximately 8% per 5 kg/m2 BMI increase in premenopausal women [RR: 0.92; 95% CI: 0.88-0.97; p=0.001]. In contrast, the risk was increased in postmenopausal women [RR: 1.12; 95% CI: 1.08-1.16; p<0.0001] (12). This latter meta-analysis undertaken by Renehan and colleagues (2008) included prospective cohort and large observational studies, and provides a stronger level of evidence than earlier case-control reports.
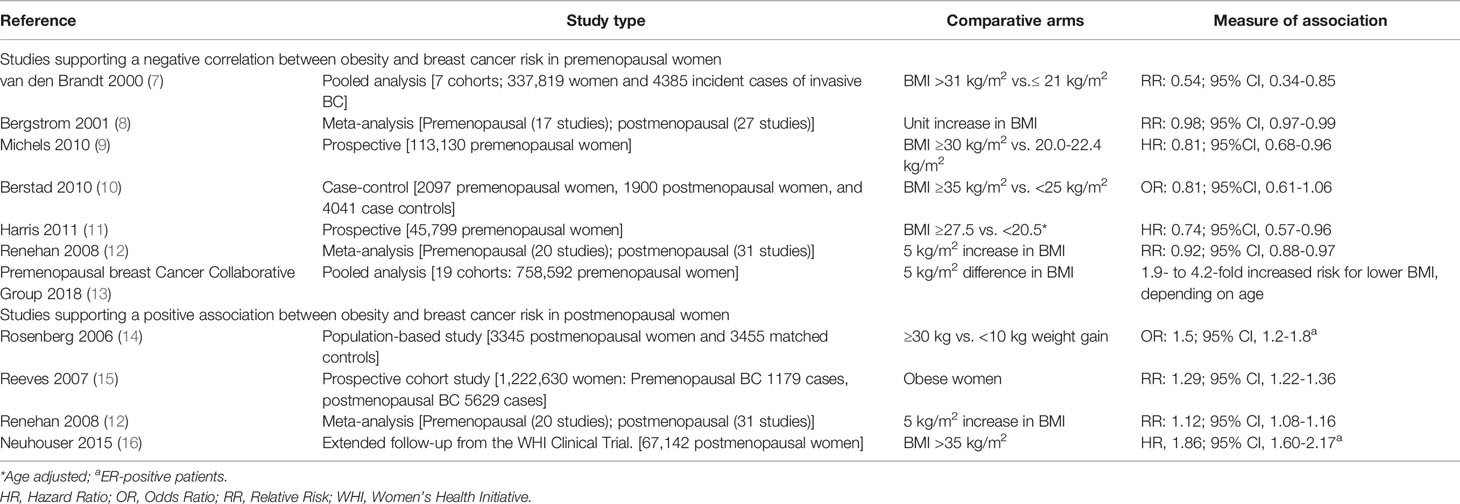
Table 1 Summary of large studies/analyses investigating the association of obesity with breast cancer (BC) according to menopausal status.
The largest, most recent study investigating the association of obesity with breast cancer risk came from The Premenopausal Breast Cancer Collaborative Group (13). This multicenter study used pooled individual-level data from 758,592 premenopausal women aged 18 to 54 years. Median follow-up was 9.3 years, during which time 13,082 cases of breast cancer were reported. The analysis revealed a significant 4.2-fold increased risk of breast cancer in underweight women versus women with obesity under 24 years, and a 1.9- to 2.5-fold increased risk in older patients. This led to an estimated 12% to 23% reduction in premenopausal breast cancer risk per 5 kg/m2 increase in BMI, depending on age. Moreover, estrogen-receptor (ER) and/or progesterone-receptor (PR) positive breast cancer was associated with BMI in all age groups, whereas hormone-receptor negative breast cancer was associated with BMI only in women aged 18-24 years. In this younger age group, the strength of the association between ER- and/or PR-receptor positive breast cancer and BMI (HR, 0.75; 95% CI, 0.70 – 0.81) was much stronger than that for ER- and PR-receptor negative breast cancer (HR, 0.85; 95% CI, 0.76 – 0.95). The lack of association of triple-negative breast cancer with BMI in women above 25 years-of-age was contrary to previous reports (17). The authors considered that the discrepancy possibly reflected differences in study design (case-controls versus prospective study); the former being more susceptible to potential selection bias.
Unlike premenopausal women, several studies and large meta-analyses have demonstrated a consistent, direct association between obesity and breast cancer risk in postmenopausal women. The Million Women Study followed 1.2 million UK women (45,037 with breast cancer) aged 50-64 years for a mean period of 5.4 years. The study identified an approximate 30% higher risk of breast cancer in postmenopausal women with obesity compared to women who were not obese (RR: 1.29; 95% CI: 1.22-1.36) (15). These findings are comparable to those of Renehan and colleagues reported above (postmenopausal breast cancer risk was positively associated with each 5 kg/m2 increase in BMI (RR: 1.12; p <0.0001) (12). The increased risk of breast cancer in postmenopausal women with obesity was greater in, and may be limited to, those not receiving menopausal hormone therapy. When factoring in hormone-receptor status, the association between BMI and postmenopausal breast cancer risk appeared to be restricted to ER-positive cases (14, 16).
Potential Mechanisms that Could Explain the Paradoxical Relationship Between Obesity and Breast Cancer
Why obesity is a risk factor for breast cancer in postmenopausal women, but appears to be protective during premenopause is clearly an important question. A better understanding of the mechanisms involved could provide significant clues to the underlying pathophysiology and have clinical implications for breast cancer prevention and treatment. Mapping the precise cellular and molecular landscape of breast tissue in the context of an evolving physiology has shed light into potential driving factors responsible for breast cancer risk and progression; including the role of serum estrogens, leptin and adiponectin imbalances, mammogram density, insulin deregulation pathways and chronic inflammation.
Difference in Estrogen Levels
Physiologically, estrogen production decreases during aging, with the natural consequence that postmenopausal women have lower blood levels of estrogenic hormones, such as estradiol, relative to premenopausal women. Estrogen synthesis in premenopausal women mainly localizes to the ovary, whereas in postmenopausal women estrogen production shifts to peripheral tissues. In postmenopausal women with obesity the main source of estrogen biosynthesis is adipose tissue.
The primary mediator of postmenopausal estrogen biosynthesis is aromatase (also known as CYP19A1, Cytochrome P450 Family 19 Subfamily A Member 1), an enzymatic complex found both in breast adipose and tumor tissues (18). Aromatase is responsible for the conversion of androgens produced by the adrenal cortex and the postmenopausal ovary into estrogens. This can lead to an increase in local estrogen levels by up to 10-fold in the endometrium and myometrium compared with that in breast tissue (19). Significantly, however, higher concentrations of estrogens were found in malignant breast tissue compared with non-malignant tissue.
Several meta-analyses of prospective studies have confirmed a relationship between increased serum estrogen levels and postmenopausal breast cancer (20–24). Although postmenopausal estradiol levels are lower overall, they have been shown to be higher in women with obesity compared with non-obese women (21 vs 12 pg/mL; with no overlap in 95% CI) (25). This contrasts with premenopausal data which found significantly lower estradiol levels (independent of age, race, and smoking) in women who were obese/overweight compared with women having normal bodyweight [32.8 pg/mL (95% CI: 30.6-35.2) vs. 39.8 pg/mL (95% CI: 37.0-42.8); p<0.001)] (25). Low estradiol levels in women with obesity may partly result from negative feedback in the hypothalamic-pituitary axis leading to a reduction in gonadotrophin secretion, and suppression of ovarian function and concomitant amenorrhea (26). Irregular menstruation and fewer ovulatory cycles may also result in a reduction in serum estradiol levels and this has been promulgated as contributory to the inverse association between obesity and breast cancer risk in premenopausal women (26).
Mammogram Density
Mammographic breast density (MBD) is defined by the proportion of glandular to fatty tissue and is usually classified using a scoring classification proposed by the American College of Radiology (ACR BI-RADS® Atlas, Breast Imaging Reporting and Data System) which describes 4 categories for breast density: a). almost entirely fatty; b). scattered fibroglandular tissue; c). heterogeneously dense fibroglandular tissue; and d). extremely dense fibroglandular tissue (27).
In women undergoing screening for breast cancer a high MBD poses two major challenges. Firstly, an elevated MBD may mask the presence of tumors within the dense tissue and diminish the detection sensitivity of screening mammography; and secondly, a high MBD is an independent risk factor for breast cancer (28). The magnitude of this association is 4.64-fold greater for the highest versus the lowest density categories. A low MBD associates with better breast cancer outcomes and lower risk of local recurrence without influencing the risk of metastasis or the mortality rates directly resulting from breast cancer. The strength of the association between MBD and breast cancer risk is greater than for most other established cancer risk factors, with the exception of age and some genetic factors (29).
The precise mechanisms underlying the positive association between elevated MBD and breast cancer risk remain poorly understood. It has been suggested that greater tissue density may be a consequence of increased epithelial cell concentrations, elevated levels of growth factors such as insulin-like growth factor-I (IGF-1), stromal fibrosis and/or epithelial hyperplasia; all of which represent potential breast cancer risk factors (28). Another plausible explanation is that there is higher aromatase expression in dense breast tissue compared to non-dense breast tissue (30). In addition, increased breast tissue density is associated with other well-established risk factors, including positive associations with hormonal replacement therapy, nulliparity, older age at first birth, and alcohol intake. Inverse associations have been linked to age, postmenopausal status and BMI (30).
In general, MBD correlates negatively with BMI; i.e., women who are obese or overweight have lower breast density than leaner women. The seemingly paradoxical relationship between obesity, breast density, and breast cancer risk lies in the fact that although adiposity has a positive correlation with absolute non-dense area and is a recognized risk factor for breast cancer, some reports indicate that absolute non-dense area correlates negatively with breast cancer risk (31). In this regard, Shieh et al. reported that elevated MBD was associated with an increased risk of ER-negative breast cancer independent of menopausal status or BMI in premenopausal women (32). Given that elevated breast density and overweight/obesity are both risk factors for ER-negative breast cancer, the authors hypothesize that they are likely to interact synergistically to augment the risk of ER-negative malignancy in younger women (32). In contrast, the study found no correlation between percent density and BMI in postmenopausal women. This result is consistent with the conclusions from the Nurses’ Health study, which reported an association between percent density and overall breast cancer in postmenopausal women, independent of BMI (33).
There is a tendency for MBD to decrease with age, while breast cancer incidence generally increases. This apparent contradiction fundamentally reflects that “true” breast tissue-age possibly results from the cumulative exposure to changing patterns of hormone and growth factor secretion and levels over time, rather than absolute age.
The Role of Adipocytokines
Breast tissue is primarily composed of adipose and fibrous tissue, and mammary glands. Adipose tissue acts as an energy reservoir, but it is also responsible for the secretion of a variety of metabolites, hormones, and cytokines, collectively known as adipokines, and in this regard it can be considered as a complex endocrine organ (34). In humans, >100 adipocytokines have been described to date, including resistin, leptin, adiponectin, visfatin, hepatocyte growth factor, tumor necrosis factor-alpha (TNF-α), heparin-binding epidermal growth factor-like growth factor, interleukin-6 (IL-6) (35), and nerve growth factor (NGF) and brain-derived neurotrophic factor (36). Among these, adiponectin and leptin have received special attention as molecular mediators in the association between obesity and breast cancer (37).
Adiponectin is a 30 kDa monomer, also known as adipocyte complement-related protein (Acrp30), which circulates in the plasma as low- and high-molecular weight multimers (38). Plasma concentrations are relatively high for a hormone and are usually within the range 2–20 μg/ml. In women, adiponectin levels correlate negatively with body weight, BMI, body fat, and serum leptin concentrations (38). Adiponectin has multiple physiological actions, including modulation of the AMP-activated protein kinase (AMPK) axis by mechanisms involving liver kinase B1 gene (LKB1), and mediated via two adiponectin receptors, AdipoR1 and AdipoR2 (39). Activation of AdipoR1 and AdipoR2 induces the formation of homodimeric and heterodimeric complexes and triggers an AMPK-mediated cascade that regulates cell proliferation, apoptosis, angiogenesis and cellular metabolism. Activated AMPK monitors cellular ‘AMP: ATP’ (adenosine triphosphate) and adenosine diphosphate ‘(ADP): ATP’ ratios, offseting ATP consumption and maintaining energy homeostasis. AMPK regulates several downstream targets including enzymes involved in the synthesis of fatty acids and triglycerides such as acetyl-CoA carboxylase (ACC) and fatty acid synthase (FAS), transcription factors, and other regulatory proteins. Adiponectin also exhibits strong insulin sensitizing and balancing activity, and reduced adiponectin levels enhance insulin signalling associated with neoplasia. In addition, AMPK activation negatively regulates mitogen-activated protein kinase (MAPK), phosphatidylinositol 3−kinase (PI3K)/protein kinase B (Akt) (PI3K/Akt), WNT-β-catenin, nuclear factor kappa-light-chain-enhancer of activated B cells (NF-κB), and JAK2/STAT3 pathways (40). AMPK-mediated inhibition of mTOR (mammalian target of rapamycin) signaling results in disregulated cell proliferation, inhibition of gluconeogenic gene transcription, and induction of enzymes engaged with fatty acid oxidation (41). In breast cancer cells, adiponectin increases serine–threonine liver kinase B1 (LKB1) expression, which can negatively impact cell proliferation, increase apoptosis, decrease aromatase activity, an reduce invasive capacity (42).
Current data in support of a direct involvement of adiponectin in breast cancer is controversial. A meta-analysis by Gu et al. showed that serum adiponectin levels were lower in breast cancer patients irrespective of menopausal status; however, no information on BMI was collected (43). In contrast, two previous meta-analyses identified a significant association between adiponectin levels and breast cancer risk in postmenopausal women (44, 45). The most recent meta-analysis on this topic by Yoon et al. reported that low adinopectin and high leptin levels represent high-risk factors for breast cancer, particularly in postmenopausal patients (46). Assessment of the role of serum adiponectin in breast cancer highlights a trend linking elevated adiponectin levels with reduced breast cancer risk when a combined analysis involving pre- and postmenopausal women was performed. However, the results appear to be driven mainly by a stronger association in the postmenopausal group. Unfortunately, similar studies investigating an association between adiponectin levels and BMI in breast cancer are lacking.
Another adipokine, leptin, also represents a key molecular mediator in the relationship between obesity and breast cancer. Leptin is a hormone predominantly produced by adipocytes in healthy and malignant tissue and is overexpressed in individuals who are obese/overweight. Serum levels in humans usually range between 5–50 ng/ml, but in individuals with obesity, concentrations can exceed 100 ng/mL (47).
Leptin displays pleiotropic effects and regulates adipose and breast tissue cell proliferation. Upon binding to its receptor ObR, leptin activates JAK2/STAT3, MAPK, and PI3K/Akt signaling pathways to promote cell proliferation, hence acting as a growth-factor in cancer and involved in regulating tumor development, growth, and metastasis (48, 49). Leptin is also able to induce aromatase activity, thus potentiating ER signaling in breast cancer cells, and this may play a role in tumor progression (50). In addition, leptin can activate ObR-expressing macrophages and stimulate secretion of multiple pro-inflammatory and proangiogenic cytokines such as IL-1, TNF-α, IL-6, IL-11 and nitric oxide to modulate macrophage phenotype (anti-inflammatory M2 and pro-inflammatory M1). Some studies have also indicated that leptin and ObR are overexpressed in primary and metastatic invasive ductal breast carcinoma cells, compared with non-cancer mammary tissue (51).
Several studies strongly support the hypothesis that serum leptin levels correlate with breast cancer occurrence and tumor behavior (52, 53). To better clarify this issue Pan et al. conducted a meta-analysis of 35 case-control and cohort studies (54). Their results confirmed an association between leptin levels and breast cancer risk, and the authors outlined two key conclusions from this meta-analysis: a strong relationship between breast cancer risk and higher leptin levels in patients who were overweight/obese and, secondly, elevated leptin levels in some but not all the studies was associated with breast cancer risk in postmenopausal but not in premenopausal women. However, a high degree of heterogeneity was observed among the studies included in the meta-analysis.
Insulin Signaling Pathway
Insulin is a peptide hormone synthesised by islet β-cells in the pancreas in response to elevations of glucose. Insulin regulates glucose uptake by muscle and adipose tissue and inhibits gluconeogenesis and glucose secretion by the liver. High insulin levels lead to an overload of glucose stores in liver and muscle. In addition to glucose homeostasis, insulin facilitates fatty acid transport, promotes lipogenesis, and inhibits lipolysis, thus contributing to the build-up of energy stores ready for mobilisation when insulin levels are low (e.g., during fasting) (55).
Under certain physiological and pathological conditions, cells may become less responsive to insulin and they eventually develop insulin resistance, causing both insulin and serum glucose levels to increase (3). Such outcomes may result from a combination of factors. For instance, endoplasmic reticulum stress, adiponectin reduction, leptin elevation, adipocyte death, macrophage infiltration and lipolysis can all contribute to the onset of chronic inflammation in obesity, and this is closely associated with marked insulin resistance and an increased likelihood of developing type 2 diabetes and other diseases (56, 57). A primary event in the transmission of inflammatory signals seems to be a reduction of functional insulin receptors and a blockage of insulin signaling pathways, and this has been hypothesized to lead to insulin resistance (58). Moreover, obesity may cause adipose tissue dysfunction, including secretion of abnormal levels of cytokines linked to insulin resistance, as well as impaired triglyceride storage and increased lipolysis. These abnormalities may in turn contribute to accumulation of non-esterified fatty acids (NEFAs) in the circulation and an overload of NEFAs by skeletal muscle and liver cells, which are liable to instigate decreased insulin responsiveness in these tissues. Increasing plasma NEFA levels might also contribute to a continuous loss of function of pancreatic β-cells, thereby causing inadequate insulin secretion (59).
When assessing type 2 diabetes as a risk factor for breast cancer the results appear to vary according to menopausal status. For example, a meta-analysis of 20 studies from nine different countries showed a 20% increase in breast cancer risk, independent of BMI, in postmenopausal women with diabetes compared with individuals who were not diabetic; significance was lost in premenopausal women (56). Likewise, a large prospective study by Michels et al. confirmed the positive relationship between type 2 diabetes and breast cancer risk (60). Interestingly, the study also found a negative trend between the two conditions in premenopausal women. Additionally, a positive association of plasma insulin and C-peptide with postmenopausal breast cancer risk has been reported, and this was independent of obesity status (at least in some studies) (56). In contrast, the situation in premenopausal women is unclear. The suggestion that relatively high circulating insulin levels may protect against breast cancer risk before the menopause is consistent with the apparently protective effect of diabetes observed by others authors; and it resembles closely the situation seen in obesity (61).
Insulin receptors are usually 6- to 10-fold overexpressed in breast cancer epithelial cells compared to healthy cells (62). Although primarily involved in the regulation of carbohydrate, lipid, and protein metabolism, insulin also acts as a growth factor, stimulating cell mitosis and migration, and inhibiting apoptosis (63). These effects may be potentiated under conditions of insulin resistance and concomitant impairment of insulin-regulated metabolic pathways. In general, insulin influences metabolic processes such as glucose transport through the PI3K pathway, whereas mitogenic stimuli involve the activation of Ras and the MAPK pathway.
Chronic Inflammation
White adipocyte tissue (WAT) comprises the largest energy storage and the major endo- and paracrine organ in the human body. WAT consists of a variety of cell types (e.g., white adipocytes, immune cells, fibroblasts and endothelial cells) and these support tissue homeostasis and insulin sensitivity. In healthy-weight individuals, adipose tissue is well vascularized and the microenvironment is rich in anti-inflammatory cytokines such as IL-4, IL-10 and IL-13. WAT contains a variety of type 2 immune cells, including alternatively activated (M2-like) macrophages, group 2 innate lymphoid cells, type 2 T helper (TH2) cells and IL-4-producing eosinophils. Thus, in healthy individuals the adipose tissue molecular milieu is largely comprised of anti-inflammatory immune cells and cytokines (64). Weight gain causes adipocytes to become hypertrophic and die, and this is a key event in the change to an obese adipose tissue microenvironment which is proinflammatory. Adipocyte death triggers innate immune responses which shift the immune milieu towards a type 1 (pro-inflammatory) state which is associated with the infiltration of leukocytes, including macrophages, as well as CD8-positive T lymphocytes and mast cells (65). The rupture of the cell membrane also facilitates the release of cellular contents [e.g., lipids, cytokines and damage-associated molecular by-products (e.g. fatty acids, ATP, reactive oxygen species, cholesterol and nucleic acids)] into the microenvironment (66). Free fatty acids (FFAs) stimulate multiple inflammatory signaling pathways, and activation of transcription factor NF-κB a key mediator in the immune response and adipose inflammation (67). These signals enhance recruitment and accumulation of macrophages in situ. Up to 90% of macrophages in the obese adipose tissue microenvironment participate in the development of adipocyte hypertrophy by encircling the dying adipocyte forming crown-like structures (CLS); a hallmark of inflammation in adipose tissue (68). Macrophages are the main source of type 1 cytokines (e.g. TNF-α, IFNγ, IL-1β and IL-6) as well as pro-inflammatory immune cells (granulocytes, group 1 innate lymphoid cells, B cells and CD8+ T cells), which act by preserving a state of chronic inflammation.
Iyengar et al. conducted a study in patients undergoing mastectomy, as treatment for (n=211) or to reduce the risk of (n=26) breast cancer, and evaluated WAT inflammation as manifested by CLS-B (crown-like structures in the breast) (69). Overall, breast WAT inflammation was independent of BMI in postmenopausal women (p=0.008), with CLS-B present in 58/93 (62%) postmenopausal versus 64/144 (44%) premenopausal women. Moreover, BMI correlated with CLS-B positivity; specifically, CLS-B was present in 43/48 (90%) obese, 39/73 (53%) overweight, and 40/116 (34%) healthy-weight (BMI <25Kg/m2) individuals (p<0.001). The results remained statistically significant in a multivariate model after adjusting for age, menopausal status, and use of non-steroidal anti-inflammatory drugs (NSAIDs) or statins (p<0.001). The authors noted that WAT inflammation and the presence of CLS-B is associated with elevated tissue levels of proinflammatory mediators and aromatase (the rate-limiting enzyme for estrogen biosynthesis), and this provides a plausible explanation for the paradoxical observation that hormone-dependent tumors are more common during the age-related menopausal transition when circulating estrogen levels have declined (69).
Both adipocytes and macrophages release pro-inflammatory mediators associated with WAT inflammation such as TNF-α, IL-1β, IL-6 and COX-2–derived prostaglandin E2, which can upregulate aromatase expression (70). Aromatase is produced by mesenchymal cells and fibroblasts surrounding lipid-filled adipocytes, rather than in the adipocytes themselves. Aromatase utilises circulating androgens as a substrate to produce estrogens, mainly estradiol, which can diffuse into tissues, especially breast adipose tissue where it can enter the breast duct and stimulate epithelial cell proliferation (71). WAT inflammation is also associated with elevated circulating levels of leptin, a well-established inducer of aromatase (72). In addition, breast WAT inflammation is paralleled by increased tissue levels of aromatase (Figure 1) (70).
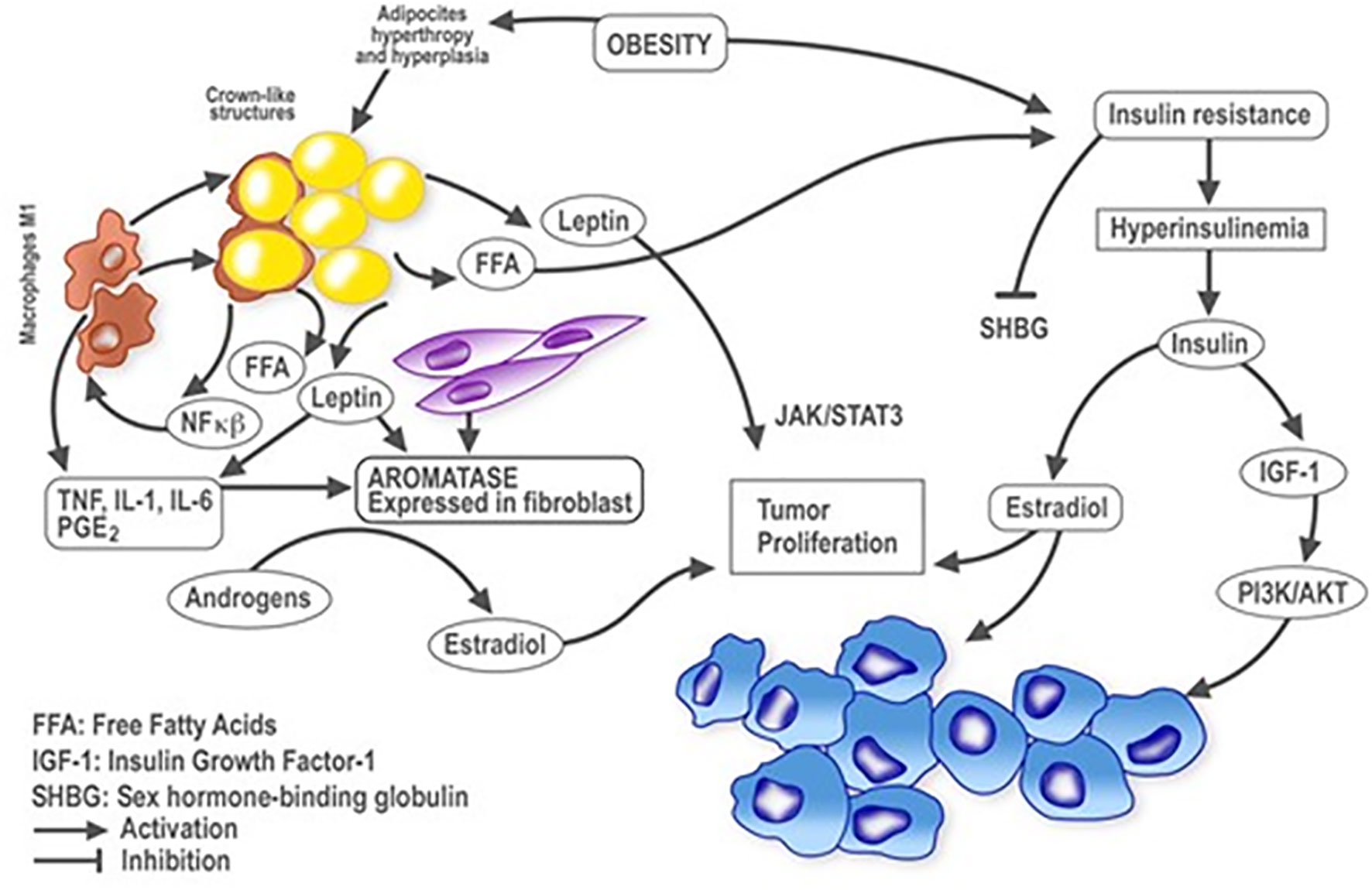
Figure 1 Plausible relationships between obesity and inflammation in breast cancer. Schematic representation of cellular and molecular events influenced by obesity and inflammation in the context of breast cancer development. Lines with arrowheads indicate activation; with flat ends, inhibition. NF-kB, nuclear factor kappa-light-chain-enhancer of activated B cells; TNFα, tumor necrosis factor alpha; IL, interleukin; PGE2, prostaglandin E2; FFA, free fatty acids; IGF-1, insulin growth factor 1; SHBG, sex hormone binding globulin.
Discussion
The precise mechanisms whereby obesity plays a protective role against breast cancer in premenopausal women, but represents a risk factor after the menopause remain elusive (Figure 2) (74). Chronic inflammation clearly influences the physiology of the breast differently depending on menopausal state, suggesting a relevant role for estrogens and adipokine-driven signaling pathways.
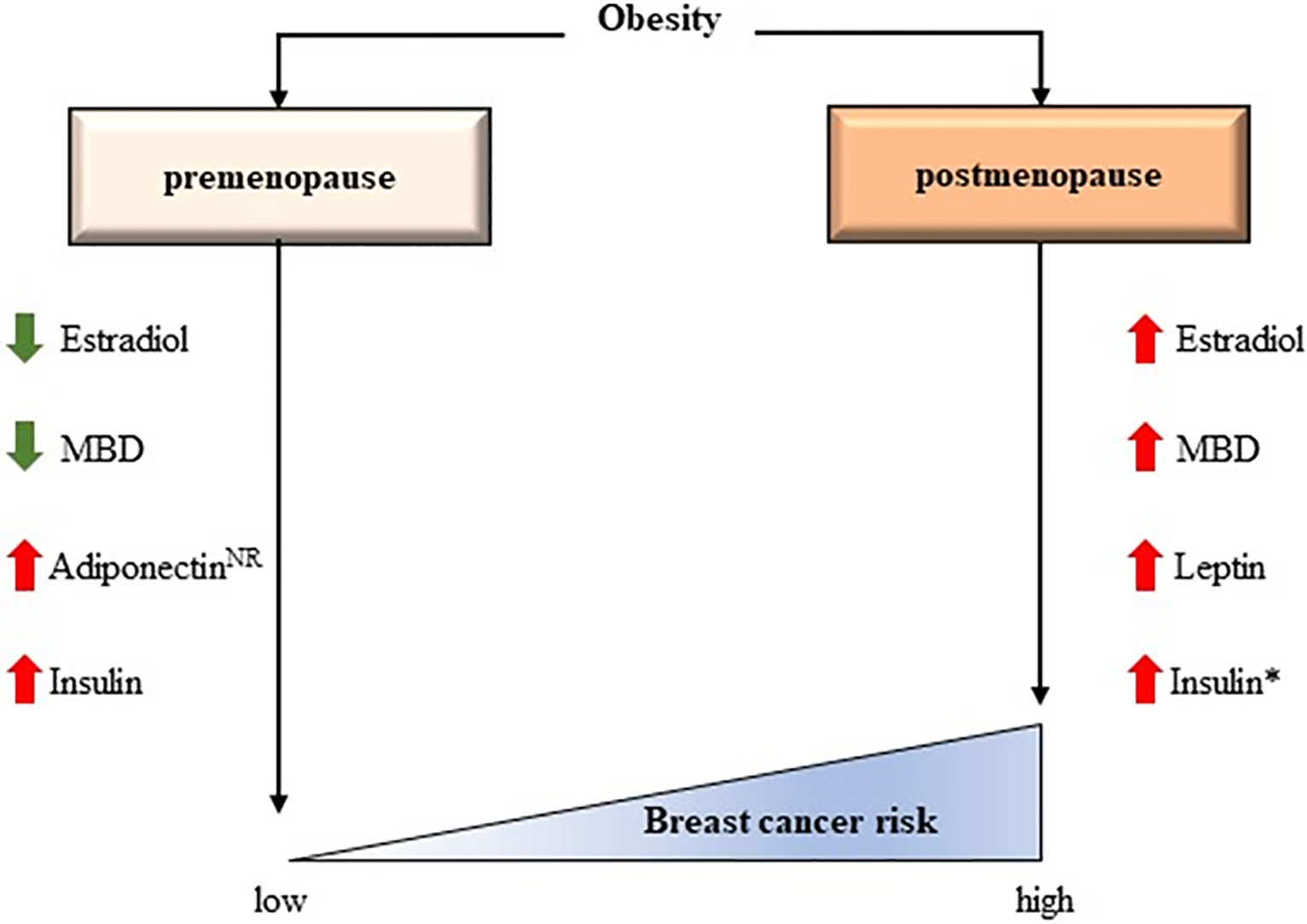
Figure 2 The controversial effects of obesity according to menopausal status. Some interactions have been confirmed while others have been hypothesized. MBD, Mammogram breast density; NR, Not clearly reported. *The effect of insulin in breast cancer risk could be independent of BMI (73).
It seems clear that the combination of oestrogens and obesity are able to protect against breast cancer, however the mechanism by which this interaction occurs is yet unknown. Zhao et al. conducted a study to address the impact of obesity in the breast according to menopausal status (75). Random fine needle aspirates (rFNAs) of breast tissue were collected from 57 premenopausal and 55 postmenopausal healthy women classified as normal-weight, overweight, and obese. Tissue samples were processed and subjected to TaqMan Low Density Array to determine the expression levels of 21 target genes. The study also measured breast tissue estradiol levels by liquid chromatography-tandem mass spectrometry and serum estradiol and follicle stimulating hormone (FSH) levels using radioimmunoassay and enzyme-linked immunosorbent assays, respectively. RPS6KB1 (an AMPK responsive gene for protein synthesis and cell growth), estrogen receptor α (encoded by the ESR1 gene) and its target gene GATA3, were significantly downregulated in rFNAs from premenopausal women with obesity. By contrast, levels of these biomarkers remained unchanged in relation to adiposity in postmenopausal women. On the other hand, prostaglandin-endoperoxide synthase 2 (PTGS2), cyclin D1 (CCND1), and TFF1 (an ESR1 target gene) were elevated in rFNAs from postmenopausal women with obesity. These results shed some light into mechanisms that potentially explain the different effects of obesity in the breast depending on menopausal status, hence contributing to our molecular understanding of breast biology in physiological and pathological situations.
Regarding adipokines, there exists a distinct relationship between leptin/adiponectin and breast cancer in postmenopausal women and such an association is far from clear in premenopausal women (73). According to Zhao and colleagues results, the protective role of obesity in premenopausal women could be favoured by activation of the AMPK signaling pathway and concomitant inhibition of proliferative stimuli via mTOR (75). Adiponectin may trigger activation of AMPK, which would explain the presence of high adiponectin levels in healthy, premenopausal women with obesity, but low levels in those with breast cancer. In relation to these observations, our group has initiated a prospective study in premenopausal women with and without breast cancer with a BMI of >25 kg/m2 (MDA-ADI-2020-01). The aim is to evaluate the blood levels of leptin and adiponectin between these two groups and to confirm the previously mentioned hypothesis: obese or overweight premenopausal women without breast cancer would have high levels of adiponectin and low levels of leptin despite a high BMI. By contrast, women with a high BMI and breast cancer would have high leptin levels and low adiponectin levels. If this hypothesis is confirmed, levels of adipokine and oestrogen in the blood could help in the prevention of breast cancer in premenopausal women.
In contrast to this hypothesis is the case-control study by Tworoger et al. showing a positive although non-significant association between adiponectin levels and breast cancer in premenopausal women; this suggests a differential role for the adipokine in breast tissue according to menopausal status (73).
Conclusions
Obesity can have a marked impact in women since it is associated with an increased risk for many chronic conditions such as diabetes and heart disease, as well as it increases the risk of malignancies such as breast cancer. Whilst most evidence suggests an inverse relationship between obesity and breast cancer risk during premenopause, no clinical strategy could contemplate weight-gain as a therapeutic approach taking into consideration the complications associated with excess fat and the risk of perpetuating a state of overweight through menopausal transition and into the menopause itself. The metabolic and cardiovascular risks associated with excessive weight would undoubtedly exceed any benefits achieved for breast cancer. Of greater importance is to research and fully understand the complex interplay between the various metabolic and hormonal factors involved, since this could potentially help define possible alternative treatment strategies. In an obese environment, the relationship between estrogens and adipokines and the activation of specific signaling pathways clearly differs between menopausal stages, and this is an area where future research can help determine the molecular/biochemical processes involved. Ultimately, a better knowledge of how obesity and adipose tissue inflammation interact with female sex hormones, and how these changes affect the pathogenesis of breast cancer, should enable us to develop new therapeutic strategies to reduce breast cancer risk and improve patient outcomes in individuals who are obese.
Author Contributions
LG-E and GM-B designed and wrote the manuscript. All authors provided editorial support and read the manuscript. All authors contributed to the article and approved the submitted version.
Funding
This research did not receive any specific grant from funding agencies in the public, commercial, or not-for-profit sectors.
Conflict of Interest
The authors declare that the research was conducted in the absence of any commercial or financial relationships that could be construed as a potential conflict of interest.
Publisher’s Note
All claims expressed in this article are solely those of the authors and do not necessarily represent those of their affiliated organizations, or those of the publisher, the editors and the reviewers. Any product that may be evaluated in this article, or claim that may be made by its manufacturer, is not guaranteed or endorsed by the publisher.
Acknowledgments
We would like to thank Dr Steve Clissold for an English language check of the manuscript. Editorial assistance was provided by Content Ed Net (Madrid, Spain).
References
1. World Health Organization. Obesity and Overweight. Available at: http://www.who.int/news-room/fact-sheets/detail/ (Accessed January 2021).
2. OECD. Obesity Update. (2017). Available at: https://www.oecd.org/els/health-systems/Obesity-Update-2017.pdf (Accessed January 2021).
3. Finkelstein EA, Khavjou OA, Thompson H, Trogdon JG, Pan L, Sherry B, et al. Obesity and Severe Obesity Forecasts Through 2030. Am J Prev Med (2012) 42(6):563–70. doi: 10.1016/j.amepre.2011.10.026
4. Guthold R, Stevens GA, Riley LM, Bull FC. Worldwide Trends in Insufficient Physical Activity From 2001 to 2016: A Pooled Analysis of 358 Population-Based Surveys With 1.9 Million Participants. Lancet Glob Health (2018) 6(10):e1077–86. doi: 10.1016/S2214-109X(18)30357-7
5. Collaboration PS. Body-Mass Index and Cause-Specific Mortality in 900 000 Adults: Collaborative Analyses of 57 Prospective Studies. Lancet (2009), 373(9669):1083–96. doi: 10.1016/S0140-6736(09)60318-4
6. Bray GA, Kim KK, Wilding JPH, World Obesity F. Obesity: A Chronic Relapsing Progressive Disease Process. A Position Statement of the World Obesity Federation. Obes Rev (2017) 18(7):715–23. doi: 10.1111/obr.12551
7. van den Brandt PA, Spiegelman D, Yaun SS, Adami HO, Beeson L, Folsom AR, et al. Pooled Analysis of Prospective Cohort Studies on Height, Weight, and Breast Cancer Risk. Am J Epidemiol (2000) 152(6):514–27. doi: 10.1093/aje/152.6.514
8. Bergstrom A, Pisani P, Tenet V, Wolk A, Adami HO. Overweight as an Avoidable Cause of Cancer in Europe. Int J Cancer (2001) 91(3):421–30. doi: 10.1002/1097-0215(200002)9999:9999<::AID-IJC1053>3.0.CO;2-T
9. Michels KB, Terry KL, Willett WC. Longitudinal Study on the Role of Body Size in Premenopausal Breast Cancer. Arch Intern Med (2006) 166(21):2395–402. doi: 10.1001/archinte.166.21.2395
10. Berstad P, Coates RJ, Bernstein L, Folger SG, Malone KE, Marchbanks PA, et al. A Case-Control Study of Body Mass Index and Breast Cancer Risk in White and African-American Women. Cancer Epidemiol Biomarkers Prev (2010) 19(6):1532–44. doi: 10.1158/1055-9965.EPI-10-0025
11. Harris HR, Willett WC, Terry KL, Michels KB. Body Fat Distribution and Risk of Premenopausal Breast Cancer in the Nurses’ Health Study II. J Natl Cancer Inst (2011) 103(3):273–8. doi: 10.1093/jnci/djq500
12. Renehan AG, Tyson M, Egger M, Heller RF, Zwahlen M. Body-Mass Index and Incidence of Cancer: A Systematic Review and Meta-Analysis of Prospective Observational Studies. Lancet (2008) 371(9612):569–78. doi: 10.1016/S0140-6736(08)60269-X
13. Group PBCC, Schoemaker MJ, Nichols HB, Wright LB, Brook MN, Jones ME, et al. Association of Body Mass Index and Age With Subsequent Breast Cancer Risk in Premenopausal Women. JAMA Oncol (2018) 4(11):e181771. doi: 10.1001/jamaoncol.2018.1771
14. Rosenberg LU, Einarsdottir K, Friman EI, Wedrén S, Dickman PW, Hall P, et al. Risk Factors for Hormone Receptor-Defined Breast Cancer in Postmenopausal Women. Cancer Epidemiol Biomarkers Prev (2006) 15(12):2482–8. doi: 10.1158/1055-9965.EPI-06-0489
15. Reeves GK, Pirie K, Beral V, Green J, Spencer E, Bull D, et al. Cancer Incidence and Mortality in Relation to Body Mass Index in the Million Women Study: Cohort Study. BMJ (2007) 335(7630):1134. doi: 10.1136/bmj.39367.495995.AE
16. Neuhouser ML, Aragaki AK, Prentice RL, Manson JE, Chlebowski R, Carty CL, et al. Overweight, Obesity, and Postmenopausal Invasive Breast Cancer Risk: A Secondary Analysis of the Women’s Health Initiative Randomized Clinical Trials. JAMA Oncol (2015) 1(5):611–21. doi: 10.1001/jamaoncol.2015.1546
17. Pierobon M, Frankenfeld CL. Obesity as a Risk Factor for Triple-Negative Breast Cancers: A Systematic Review and Meta-Analysis. Breast Cancer Res Treat (2013) 137(1):307–14. doi: 10.1007/s10549-012-2339-3
18. Miller WR. Aromatase and the Breast: Regulation and Clinical Aspects. Maturitas (2006) 54(4):335–41. doi: 10.1016/j.maturitas.2006.04.020
19. van Landeghem AA, Poortman J, Nabuurs M, Thijssen JH. Endogenous Concentration and Subcellular Distribution of Estrogens in Normal and Malignant Human Breast Tissue. Cancer Res (1985) 45(6):2900–6.
20. Thomas HV, Reeves GK, Key TJ. Endogenous Estrogen and Postmenopausal Breast Cancer: A Quantitative Review. Cancer Causes Control (1997) 8(6):922–8. doi: 10.1023/A:1018476631561
21. Key T, Appleby P, Barnes I, Reeves G, Endogenous H. Breast Cancer Collaborative G: Endogenous Sex Hormones and Breast Cancer in Postmenopausal Women: Reanalysis of Nine Prospective Studies. J Natl Cancer Inst (2002) 94(8):606–16. doi: 10.1093/jnci/94.8.606
22. Missmer SA, Eliassen AH, Barbieri RL, Hankinson SE. Endogenous Estrogen, Androgen, and Progesterone Concentrations and Breast Cancer Risk Among Postmenopausal Women. J Natl Cancer Inst (2004) 96(24):1856–65. doi: 10.1093/jnci/djh336
23. Kaaks R, Rinaldi S, Key TJ, Berrino F, Peeters PH, Biessy C, et al. Postmenopausal Serum Androgens, Oestrogens and Breast Cancer Risk: The European Prospective Investigation Into Cancer and Nutrition. Endocr Relat Cancer (2005) 12(4):1071–82. doi: 10.1677/erc.1.01038
24. Hankinson SE, Willett WC, Manson JE, Colditz GA, Hunter DJ, Spiegelman D, et al. Plasma Sex Steroid Hormone Levels and Risk of Breast Cancer in Postmenopausal Women. J Natl Cancer Inst (1998) 90(17):1292–9. doi: 10.1093/jnci/90.17.1292
25. Freeman EW, Sammel MD, Lin H, Gracia CR. Obesity and Reproductive Hormone Levels in the Transition to Menopause. Menopause (2010) 17(4):718–26. doi: 10.1097/gme.0b013e3181cec85d
26. Dowsett M, Folkerd E. Reduced Progesterone Levels Explain the Reduced Risk of Breast Cancer in Obese Premenopausal Women: A New Hypothesis. Breast Cancer Res Treat (2015) 149(1):1–4. doi: 10.1007/s10549-014-3211-4
27. D’Orsi CJ, Sickles EA, Mendelson EB, Morris EA. ACR BI-RADS Atlas: Breast Imaging Re-Porting and Data System. Reston, VA, USA: American College of Radiology (2013).
28. Boyd NF, Martin LJ, Yaffe MJ, Minkin S. Mammographic Density and Breast Cancer Risk: Current Understanding and Future Prospects. Breast Cancer Res (2011) 13(6):223. doi: 10.1186/bcr2942
29. McCormack VA, dos Santos Silva I. Breast Density and Parenchymal Patterns as Markers of Breast Cancer Risk: A Meta-Analysis. Cancer Epidemiol Biomarkers Prev (2006) 15(6):1159–69. doi: 10.1158/1055-9965.EPI-06-0034
30. Vachon CM, Sasano H, Ghosh K, Brandt KR, Watson DA, Reynolds C, et al. Aromatase Immunoreactivity Is Increased in Mammographically Dense Regions of the Breast. Breast Cancer Res Treat (2011) 125(1):243–52. doi: 10.1007/s10549-010-0944-6
31. Pettersson A, Graff RE, Ursin G, Santos Silva ID, McCormack V, Baglietto L, et al. Mammographic Density Phenotypes and Risk of Breast Cancer: A Meta-Analysis. J Natl Cancer Inst (2014) 106(5). doi: 10.1093/jnci/dju078
32. Shieh Y, Scott CG, Jensen MR, Norman AD, Bertrand KA, Pankratz VS, et al. Body Mass Index, Mammographic Density, and Breast Cancer Risk by Estrogen Receptor Subtype. Breast Cancer Res (2019) 21(1):48. doi: 10.1186/s13058-019-1129-9
33. Yaghjyan L, Colditz GA, Rosner B, Tamimi RM. Mammographic Breast Density and Breast Cancer Risk: Interactions of Percent Density, Absolute Dense, and Non-Dense Areas With Breast Cancer Risk Factors. Breast Cancer Res Treat (2015) 150(1):181–9. doi: 10.1007/s10549-015-3286-6
34. Chaldakov GN, Stankulov IS, Hristova M, Ghenev PI. Adipobiology of Disease: Adipokines and Adipokine-Targeted Pharmacology. Curr Pharm Des (2003) 9(12):1023–31. doi: 10.2174/1381612033455152
35. Cao H. Adipocytokines in Obesity and Metabolic Disease. J Endocrinol (2014) 220(2):T47–59. doi: 10.1530/JOE-13-0339
36. Sornelli F, Fiore M, Chaldakov GN, Aloe L. Adipose Tissue-Derived Nerve Growth Factor and Brain-Derived Neurotrophic Factor: Results From Experimental Stress and Diabetes. Gen Physiol Biophys (2009) 28:179–83.
37. Adriaenssens E, Vanhecke E, Saule P, Mougel A, Page A, Romon R, et al. Nerve Growth Factor Is a Potential Therapeutic Target in Breast Cancer. Cancer Res (2008) 68(2):346–51. doi: 10.1158/0008-5472.CAN-07-1183
38. Ryan AS, Berman DM, Nicklas BJ, Sinha M, Gingerich RL, Meneilly GS, et al. Plasma Adiponectin and Leptin Levels, Body Composition, and Glucose Utilization in Adult Women With Wide Ranges of Age and Obesity. Diabetes Care (2003) 26(8):2383–8. doi: 10.2337/diacare.26.8.2383
39. Yamauchi T, Kamon J, Ito Y, Tsuchida A, Yokomizo T, Kita S, et al. Cloning of Adiponectin Receptors That Mediate Antidiabetic Metabolic Effects. Nature (2003) 423(6941):762–9. doi: 10.1038/nature01705
40. Kim KY, Baek A, Hwang JE, Choi YA, Jeong J, Lee MS, et al. Adiponectin-Activated AMPK Stimulates Dephosphorylation of AKT Through Protein Phosphatase 2A Activation. Cancer Res (2009) 69(9):4018–26. doi: 10.1158/0008-5472.CAN-08-2641
41. Long YC, Zierath JR. AMP-Activated Protein Kinase Signaling in Metabolic Regulation. J Clin Invest (2006) 116(7):1776–83. doi: 10.1172/JCI29044
42. Taliaferro-Smith L, Nagalingam A, Zhong D, Zhou W, Saxena NK, Sharma D. LKB1 is Required for Adiponectin-Mediated Modulation of AMPK-S6K Axis and Inhibition of Migration and Invasion of Breast Cancer Cells. Oncogene (2009) 28(29):2621–33. doi: 10.1038/onc.2009.129
43. Gu L, Cao C, Fu J, Li Q, Li DH, Chen MY. Serum Adiponectin in Breast Cancer: A Meta-Analysis. Med (Baltimore) (2018) 97(29):e11433. doi: 10.1097/MD.0000000000011433
44. Liu LY, Wang M, Ma ZB, Yu LX, Zhang Q, Gao DZ, et al. The Role of Adiponectin in Breast Cancer: A Meta-Analysis. PloS One (2013) 8(8):e73183. doi: 10.1371/journal.pone.0073183
45. Ye J, Jia J, Dong S, Zhang C, Yu S, Li L, et al. Circulating Adiponectin Levels and the Risk of Breast Cancer: A Meta-Analysis. Eur J Cancer Prev (2014) 23(3):158–65. doi: 10.1097/CEJ.0b013e328364f293
46. Yoon YS, Kwon AR, Lee YK, Oh SW. Circulating Adipokines and Risk of Obesity Related Cancers: A Systematic Review and Meta-Analysis. Obes Res Clin Pract (2019) 13(4):329–39. doi: 10.1016/j.orcp.2019.03.006
47. Oksanen L, Ohman M, Heiman M, Kainulainen K, Kaprio J, Mustajoki P, et al. Markers for the Gene Ob and Serum Leptin Levels in Human Morbid Obesity. Hum Genet (1997) 99(5):559–64. doi: 10.1007/s004390050406
48. Tartaglia LA, Dembski M, Weng X, Deng N, Culpepper J, Devos R, et al. Identification and Expression Cloning of a Leptin Receptor, OB-R. Cell (1995) 83(7):1263–71. doi: 10.1016/0092-8674(95)90151-5
49. Barone I, Giordano C, Bonofiglio D, Ando S, Catalano S. Leptin, Obesity and Breast Cancer: Progress to Understanding the Molecular Connections. Curr Opin Pharmacol (2016) 31:83–9. doi: 10.1016/j.coph.2016.10.003
50. Catalano S, Marsico S, Giordano C, Mauro L, Rizza P, Panno ML, et al. Leptin Enhances, via AP-1, Expression of Aromatase in the MCF-7 Cell Line. J Biol Chem (2003) 278(31):28668–76. doi: 10.1074/jbc.M301695200
51. Garofalo C, Koda M, Cascio S, Sulkowska M, Kanczuga-Koda L, Golaszewska J, et al. Increased Expression of Leptin and the Leptin Receptor as a Marker of Breast Cancer Progression: Possible Role of Obesity-Related Stimuli. Clin Cancer Res (2006) 12(5):1447–53. doi: 10.1158/1078-0432.CCR-05-1913
52. Niu J, Jiang L, Guo W, Shao L, Liu Y, Wang L. The Association Between Leptin Level and Breast Cancer: A Meta-Analysis. PloS One (2013) 8(6):e67349. doi: 10.1371/journal.pone.0067349
53. Rodrigo C, Tennekoon KH, Karunanayake EH, De Silva K, Amarasinghe I, Wijayasiri A. Circulating Leptin, Soluble Leptin Receptor, Free Leptin Index, Visfatin and Selected Leptin and Leptin Receptor Gene Polymorphisms in Sporadic Breast Cancer. Endocr J (2017) 64(4):393–401. doi: 10.1507/endocrj.EJ16-0448
54. Pan H, Deng LL, Cui JQ, Shi L, Yang YC, Luo JH, et al. Association Between Serum Leptin Levels and Breast Cancer Risk: An Updated Systematic Review and Meta-Analysis. Med (Baltimore) (2018) 97(27):e11345. doi: 10.1097/MD.0000000000011345
55. Haeusler RA, McGraw TE, Accili D. Biochemical and Cellular Properties of Insulin Receptor Signalling. Nat Rev Mol Cell Biol (2018) 19(1):31–44. doi: 10.1038/nrm.2017.89
56. Larsson SC, Mantzoros CS, Wolk A. Diabetes Mellitus and Risk of Breast Cancer: A Meta-Analysis. Int J Cancer (2007) 121(4):856–62. doi: 10.1002/ijc.22717
57. Ye J, McGuinness OP. Inflammation During Obesity is Not All Bad: Evidence From Animal and Human Studies. Am J Physiol Endocrinol Metab (2013) 304(5):E466–77. doi: 10.1152/ajpendo.00266.2012
58. Rehman K, Akash MS. Mechanisms of Inflammatory Responses and Development of Insulin Resistance: How Are They Interlinked? J BioMed Sci (2016) 23(1):87. doi: 10.1186/s12929-016-0303-y
59. Kahn SE, Hull RL, Utzschneider KM. Mechanisms Linking Obesity to Insulin Resistance and Type 2 Diabetes. Nature (2006) 444(7121):840–6. doi: 10.1038/nature05482
60. Michels KB, Solomon CG, Hu FB, Rosner BA, Hankinson SE, Colditz GA, et al. Nurses’ Healths: Type 2 Diabetes and Subsequent Incidence of Breast Cancer in the Nurses’ Health Study. Diabetes Care (2003) 26(6):1752–8. doi: 10.2337/diacare.26.6.1752
61. Baron JA, Weiderpass E, Newcomb PA, Ho GY, Going SB, Beebe-Dimmer J, et al. Metabolic Disorders and Breast Cancer Risk (United States). Cancer Causes Control (2001) 12(10):875–80. doi: 10.1023/A:1013796112348
62. Papa V, Costantino A, Belfiore A. Insulin Receptor What Role in Breast Cancer? Trends Endocrinol Metab (1997) 8(8):306–12. doi: 10.1016/S1043-2760(97)00114-8
63. Mauro L, Naimo GD, Ricchio E, Panno ML, Ando S. Cross-Talk Between Adiponectin and IGF-IR in Breast Cancer. Front Oncol (2015) 5:157. doi: 10.3389/fonc.2015.00157
64. Quail DF, Dannenberg AJ. The Obese Adipose Tissue Microenvironment in Cancer Development and Progression. Nat Rev Endocrinol (2019) 15(3):139–54. doi: 10.1038/s41574-018-0126-x
65. Howe LR, Subbaramaiah K, Hudis CA, Dannenberg AJ. Molecular Pathways: Adipose Inflammation as a Mediator of Obesity-Associated Cancer. Clin Cancer Res (2013) 19(22):6074–83. doi: 10.1158/1078-0432.CCR-12-2603
66. Wen H, Gris D, Lei Y, Jha S, Zhang L, Huang MT, et al. Fatty Acid-Induced NLRP3-ASC Inflammasome Activation Interferes With Insulin Signaling. Nat Immunol (2011) 12(5):408–15. doi: 10.1038/ni.2022
67. Hotamisligil GS. Endoplasmic Reticulum Stress and the Inflammatory Basis of Metabolic Disease. Cell (2010) 140(6):900–17. doi: 10.1016/j.cell.2010.02.034
68. Cinti S, Mitchell G, Barbatelli G, Murano I, Ceresi E, Faloia E, et al. Adipocyte Death Defines Macrophage Localization and Function in Adipose Tissue of Obese Mice and Humans. J Lipid Res (2005) 46(11):2347–55. doi: 10.1194/jlr.M500294-JLR200
69. Iyengar NM, Morris PG, Zhou XK, Gucalp A, Giri D, Harbus MD, et al. Menopause Is a Determinant of Breast Adipose Inflammation. Cancer Prev Res (Phila) (2015) 8(5):349–58. doi: 10.1158/1940-6207.CAPR-14-0243
70. Subbaramaiah K, Morris PG, Zhou XK, Morrow M, Du B, Giri D, et al. Increased Levels of COX-2 and Prostaglandin E2 Contribute to Elevated Aromatase Expression in Inflamed Breast Tissue of Obese Women. Cancer Discov (2012) 2(4):356–65. doi: 10.1158/2159-8290.CD-11-0241
71. Simpson ER, Brown KA. Obesity and Breast Cancer: Role of Inflammation and Aromatase. J Mol Endocrinol (2013) 51(3):T51–9. doi: 10.1530/JME-13-0217
72. Brown KA, Simpson ER. Obesity and Breast Cancer: Progress to Understanding the Relationship. Cancer Res (2010) 70(1):4–7. doi: 10.1158/0008-5472.CAN-09-2257
73. Tworoger SS, Eliassen AH, Kelesidis T, Colditz GA, Willett WC, Mantzoros CS, et al. Plasma Adiponectin Concentrations and Risk of Incident Breast Cancer. J Clin Endocrinol Metab (2007) 92(4):1510–6. doi: 10.1210/jc.2006-1975
74. Vona-Davis L, Rose DP. Type 2 Diabetes and Obesity Metabolic Interactions: Common Factors for Breast Cancer Risk and Novel Approaches to Prevention and Therapy. Curr Diabetes Rev (2012) 8(2):116–30. doi: 10.2174/157339912799424519
Keywords: overweight, menopause, estrogen, mammogram, adipocytokine, insulin, obesity
Citation: García-Estévez L, Cortés J, Pérez S, Calvo I, Gallegos I and Moreno-Bueno G (2021) Obesity and Breast Cancer: A Paradoxical and Controversial Relationship Influenced by Menopausal Status. Front. Oncol. 11:705911. doi: 10.3389/fonc.2021.705911
Received: 06 May 2021; Accepted: 22 July 2021;
Published: 13 August 2021.
Edited by:
Assia Konsoulova, Complex Oncological Center (Burgas), BulgariaReviewed by:
George Nikov Chaldakov, Medical University of Varna, BulgariaAntonio Colantuoni, University of Naples Federico II, Italy
Copyright © 2021 García-Estévez, Cortés, Pérez, Calvo, Gallegos and Moreno-Bueno. This is an open-access article distributed under the terms of the Creative Commons Attribution License (CC BY). The use, distribution or reproduction in other forums is permitted, provided the original author(s) and the copyright owner(s) are credited and that the original publication in this journal is cited, in accordance with accepted academic practice. No use, distribution or reproduction is permitted which does not comply with these terms.
*Correspondence: Laura García-Estévez, bGdlc3RldmV6QG1kYW5kZXJzb24uZXM=; Gema Moreno-Bueno, Z21vcmVub0BpaWIudWFtLmVz