- 1Center for Childhood Cancer and Blood Diseases, Abigail Wexner Research Institute at Nationwide Children’s Hospital, Columbus, OH, United States
- 2Department of Medicine, University of Rochester School of Medicine and Dentistry, Rochester, NY, United States
- 3Department of Otolaryngology Head & Neck Surgery, Harvard Medical School, Massachusetts Eye and Ear, and Massachusetts General Hospital, Boston, MA, United States
- 4Division of Medicinal Chemistry and Pharmacognosy, The Ohio State University College of Pharmacy, Columbus, OH, United States
- 5Department of Pediatrics, The Ohio State University College of Medicine, Columbus, OH, United States
- 6Department of Otolaryngology-Head & Neck Surgery, The Ohio State University College of Medicine, Columbus, OH, United States
The neurofibromatosis syndromes, including NF1, NF2, and schwannomatosis, are tumor suppressor syndromes characterized by multiple nervous system tumors, particularly Schwann cell neoplasms. NF-related tumors are mainly treated by surgery, and some of them have been treated by but are refractory to conventional chemotherapy. Recent advances in molecular genetics and genomics alongside the development of multiple animal models have provided a better understanding of NF tumor biology and facilitated target identification and therapeutic evaluation. Many targeted therapies have been evaluated in preclinical models and patients with limited success. One major advance is the FDA approval of the MEK inhibitor selumetinib for the treatment of NF1-associated plexiform neurofibroma. Due to their anti-neoplastic, antioxidant, and anti-inflammatory properties, selected natural compounds could be useful as a primary therapy or as an adjuvant therapy prior to or following surgery and/or radiation for patients with tumor predisposition syndromes, as patients often take them as dietary supplements and for health enhancement purposes. Here we review the natural compounds that have been evaluated in NF models. Some have demonstrated potent anti-tumor effects and may become viable treatments in the future.
Neurofibromatosis (NF) Comprises Three Distinct Genetic Disorders That Cause Tumors to Grow Along Nerves
As a group of slowly progressive autosomal-dominant syndromes, NF is classified into neurofibromatosis type 1 (NF1), neurofibromatosis type 2 (NF2), and schwannomatosis (1). These syndromes typically present with neural tumors, which manifest in different locations depending upon their genetic etiology. Although the tumors frequently remain benign, they incur severe patient morbidity and occasionally exhibit malignant progression.
NF1, previously known as von Recklinghausen disease, affects ~1 in 3,000 individuals (2) and is caused by mutations of the NF1 gene on chromosome 17q11.2 (3–5). Although NF1 mutations can be inherited, the de novo mutation rate is relatively high (~42%). With 100% penetrance, NF1 patients are symptomatic, but with variable expression. First presenting with hyperpigmentation-like café-au-lait macules and freckling in afflicted patients, the hallmark of NF1 is the development of neurofibromas, benign tumors composed of an admixture of dysplastic Schwann cells and stromal cells, including fibroblasts, mast cells, and perineural cells embedded in a collagenous extracellular matrix (ECM). Nearly all NF1 patients develop cutaneous neurofibromas (CNFs, or dermal neurofibroma), which arise along superficial nerves and are confined to the cutaneous tissues (6). CNFs can cause itching and pain. About half of NF1 patients also develop more serious plexiform neurofibromas (PNFs) (7). These tumors occur deeper within the body and are more extensive, surrounding multiple nerve roots and causing pain and disfigurement. PNFs can undergo malignant progression, and 8-13% of PNFs in NF1 patients develop into highly-aggressive malignant peripheral nerve sheath tumors (MPNSTs) (8).
A fraction (15-20%) of NF1 patients develop optic pathway gliomas (OPGs, astrocytomas of the optic tract) (9). Patients with OPGs may present with visual disturbances and progressive vision loss. NF1 patients are also predisposed to other types of tumors, including gliomas, gastrointestinal stromal tumors, juvenile myelomonocytic leukemia, and glomus tumors (10). Although most NF1-related gliomas are classified as benign pilocytic astrocytomas, adult NF1 patients are at ~50-fold increased risk of developing malignant glioblastomas.
For current NF1 treatment, surgical excision of CNFs can be performed when symptomatic (6). Surgical removal of PNFs is more challenging because they are more diffuse and involve multiple nerve roots. A recent exciting development is the FDA approval of the MEK inhibitor selumetinib (Koselugo™) for the treatment of children with symptomatic, inoperable PNF based on the trial (ClinicalTrials.gov Identifier: NCT01362803) which demonstrated a 72% response rate in tumor volume shrinkage by ≥20% in NF1 children with PNFs (11). However, this is not curative as no subjects had complete tumor disappearance and tumors regrew particularly after dose reduction or cessation due to toxic effects, including diarrhea, weight gain, paronychia, skin ulceration, and elevated creatinine level. Identification of other targeted agents that synergize with selumetinib in eliminating PNFs should be sought. Several other MEK inhibitors, such as trametinib (Mekinist®; ClinicalTrials.gov Identifiers: NCT02124772 and NCT03741101) and cobimetinib (NCT02639546), are also being evaluated in children and adults with PNFs. Mirdametinib was recently reported to shrink the sizes of PNFs in adults and reduce tumor-associated pain (NCT02096471) (12), and a larger trial of this drug in children and adults with NF1 is currently recruiting (NCT03962543). Additionally, a topical gel formulation of the MEK inhibitor NFX-179 is being tested in NF1 patients with CNFs (NCT04435665).
Children with OPGs are routinely monitored for clinical progression by neuroimaging and visual acuity tests (13). Slow-growing gliomas causing minimal symptoms are usually not treated, but if the disease progresses, chemotherapy and occasionally, surgery is used to stabilize and reduce tumor burden. Radiation is contraindicated in NF1 patients with benign tumors due to the heightened risk of secondary malignancies. In a phase 2 clinical trial of selumetinib for pediatric low-grade glioma, sustained responses were seen in ~40% of NF1 patients (14), leading to a phase 3 trial comparing selumetinib to chemotherapy (ClinicalTrials.gov Identifier: NCT03871257). Due to highly aggressive behavior, MPNSTs are excised with wide margins (8). Radiation may be used following resection of large (≥5cm) tumors, and chemotherapy can be applied in a primary or adjuvant setting to treat unresectable or metastatic tumors. Despite these efforts, the local recurrence rate remains high (~32-65%), suggesting an urgent need for additional effective treatments (8, 15).
NF2 has an incidence of ~1 in 30,000 and has nearly complete penetrance (16). It is caused by mutations in the NF2 gene on chromosome 22q12.2 (17, 18). The hallmark of NF2 is bilateral vestibular schwannomas (VS). As the most common benign tumors of the cerebellopontine angle, 95% of VS are unilateral and occur sporadically. Occasionally, unilateral tumors are found in NF2 patients, especially when they are mosaic for NF2 loss. Like NF2-related VS, sporadic unilateral VS harbor NF2 mutations (19). Compared to sporadic VS, NF2-associated tumors display more aggressive behavior, with a propensity towards multifocal, rapid growth. Due to their intracranial location and proximity to cranial nerves, VS present with serious comorbidities, including hearing loss, tinnitus, balance dysfunction, facial weakness, and brainstem compression. NF2 is also associated with an increased incidence of cutaneous schwannomas.
Patients with NF2 also develop meningiomas and less commonly, spinal schwannomas, ependymomas, and astrocytomas (1, 16). Meningiomas are the most common brain tumors, and ~80% are benign (WHO grade I), whereas the remaining are atypical (grade II) and anaplastic (grade III) (20). In addition to NF2-related meningiomas, NF2 mutations are found in ~50% of sporadic meningiomas. Up to 60% of NF2 patients develop meningiomas, mostly benign and often multiple, which are associated with disease severity and increased mortality (21). These tumors cause significant morbidity, including cranial nerve palsy, seizures, and brainstem compression, which may lead to paralysis, aspiration pneumonia, and death.
The current standard-of-care for NF2-related tumors is surgery (1, 16, 19). Radiosurgery, such as stereotactic γ-knife radiation, may be used, especially for unresectable tumors. However, it must be weighed against the risk of causing malignant transformation of benign tumors and inducing second-site malignancies. Also, surgery may not be recommended if the tumor is located in critical structures or if there is high tumor burden (21). Currently, an FDA-approved medical therapy is not available for NF2 patients, although a few targeted therapies have been evaluated (1). Bevacizumab (Avastin®), a monoclonal antibody that neutralizes vascular endothelial growth factor (VEGF), is administered off-label to patients with NF2 and progressive VS, with ~36-41% of the patients experiencing durable hearing improvement and reduced tumor volume (22, 23). However, intravenous administration of bevacizumab is needed, and its adverse effect profile, including hypertension and proteinuria, may preclude long-term use for some patients. Also, meningiomas are unresponsive to bevacizumab (24). Another targeted drug, the kinase inhibitor brigatinib, is currently in a phase 2 clinical trial for NF2-related tumors (ClinicalTrials.gov Identifier: NCT04374305).
Schwannomatosis manifests as multiple schwannomas on nerves throughout the body but without involvement of vestibular nerves (1, 25). The true incidence of schwannomatosis is unknown but thought to be similar to NF2. Schwannomatosis usually occurs sporadically, although familial or mosaic cases occasionally occur. The most common familial mutations occur in either the SNF5/SMARCB1/INI1 (Switch/Sucrose Non-Fermentable chromatin remodeling complex subunit-5/SWI/SNF-related, Matrix-associated, Actin-dependent Regulator of Chromatin, subfamily-B, member-1/INtegrase Interactor 1) gene on chromosome 22q11.23 or the LZTR1 (Leucine Zipper-like Transcriptional Regulator 1) gene on chromosome 22q11.21; both are located near the NF2 locus (26, 27). Interestingly, schwannomatosis-related schwannomas also exhibit NF2 inactivation. It is currently thought that familial schwannomatosis is inherited through a “three-event, four-hit” process where inactivating mutations occur in the NF2 gene located on the same chromosome as the germline-mutated LZTR1 or SMARCB1 allele. Loss of the remaining normal copy of chromosome 22q results in biallelic loss-of-heterozygosity (1, 16). However, some patients with schwannomatosis do not have LZTR1 or SNF5/SMARCB1/INI1 mutations, suggesting the presence of another tumor suppressor gene on chromosome 22q in schwannomatosis development.
Patients with schwannomatosis are often diagnosed at age over 30 and frequently present with chronic debilitating pain. As an FDA-approved drug is also not available, surgery is considered for symptomatic patients. As pain is the major symptom experienced by patients with schwannomatosis, the anti-nerve growth factor (NGF) neutralizing monoclonal antibody tanezumab is being tested in a phase 2 trial for pain alleviation (ClinicalTrials.gov Identifier: NCT04163419). Schwannomatosis is also associated with an increased risk of meningioma (1, 16). Unlike NF2, schwannomatosis patients with germline loss of SMARCB1 have a higher risk of developing MPNSTs.
Signaling Pathways Affected in NF-Associated Tumors
The NF1 gene encodes neurofibromin, a Ras-GAP (GTPase-activating protein) which stimulates the intrinsic GTPase activity of Ras, converting it from the active GTP-bound to inactive GDP-bound state (9, 28). Therefore, NF1-deficient cells consistently exhibit excessive levels of Ras-GTP and activation of its downstream signaling, including the Raf/MEK/ERK mitogen-activated protein kinase (MAPK) and phosphoinositide-3-kinase (PI3K)/AKT/mammalian target of rapamycin (mTOR) pathways (Figure 1). Activated ERKs phosphorylate several effectors important for cell proliferation, including components of the protein translation apparatus, cell-cycle proteins, transcription factors, other kinases, and phosphatases. Activated AKT signals multiple downstream targets, promoting cell growth, survival, and motility (29). Through phosphorylation of mTOR, AKT also promotes protein translation. The mTOR protein functions as a component in two multi-protein complexes, mTORC1 and mTORC2, with overlapping protein compositions but distinct cellular functions (30). By integrating various extracellular and intracellular signals, including growth factor receptor signaling and the levels of ATP, oxygen, and nutrients, mTORC1 regulates ribosome biogenesis and protein translation. The mTORC2 complex is insensitive to nutrient levels but is responsive to growth factor-mediated activation of PI3K. Paradoxically, mTORC1 negatively regulates the activity of mTORC2 via direct inhibition or through S6-kinase. Acute inhibition of mTORC1 by rapamycin and rapalogs can cause feedback activation of mTORC2, which then phosphorylates AKT on serine-473, leading to restoration of PI3K/AKT signaling.
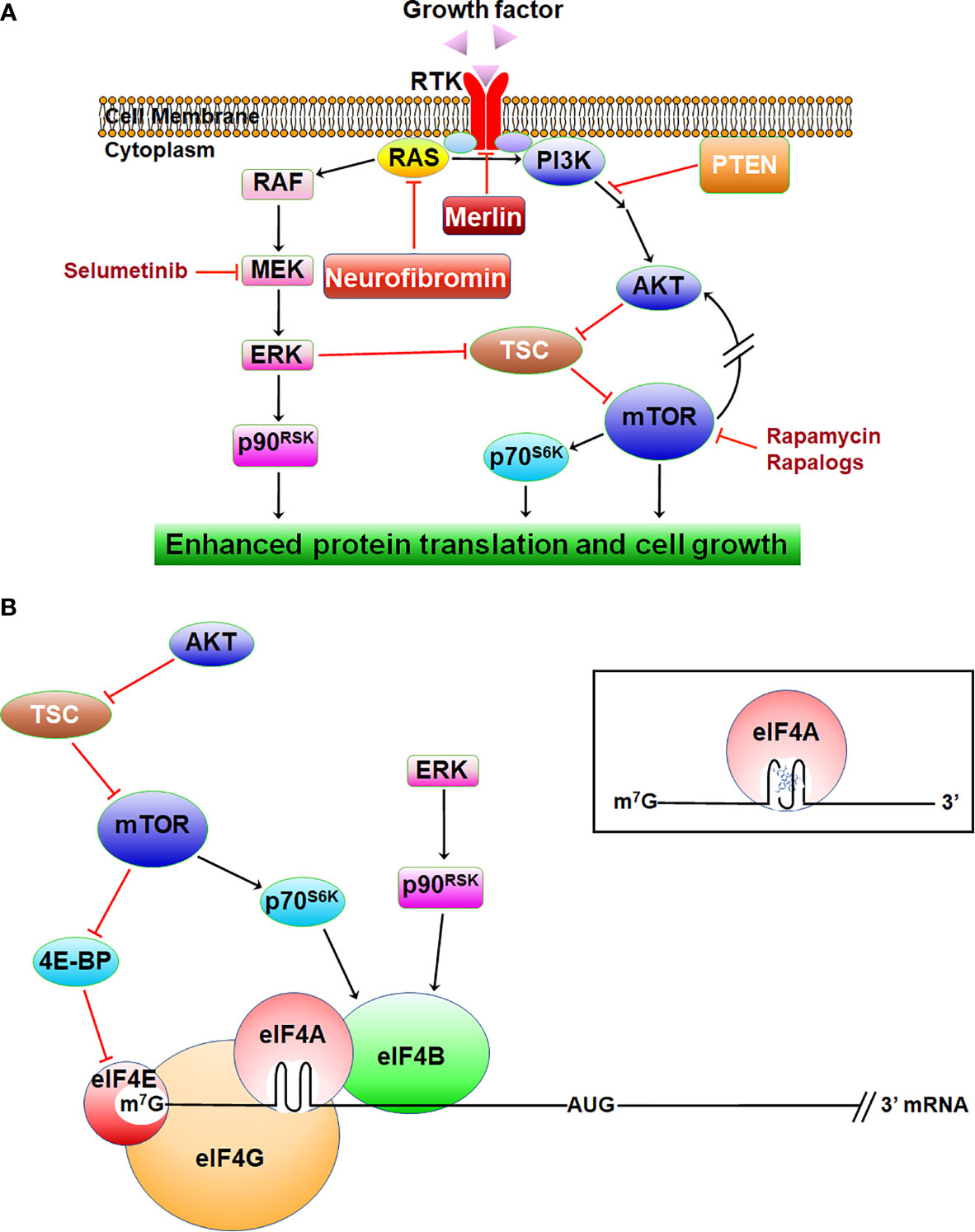
Figure 1 The signaling pathways inhibited by neurofibromin and merlin. (A) NF-related tumors exhibit activated Ras/RAF/MEK/ERK and PI3K/AKT/mTOR, leading to enhanced protein translation and growth. (B) Activated ERK and AKT/mTOR enhance translation via eIF4B, which stimulates eIF4A activity. AKT/mTOR also promotes translation via eIF4E. Inset: rocaglamide locks eIF4A onto the structured 5’-UTR of mRNA.
Neurofibromas express several RTK ligands, including neuregulin/heregulin, insulin-like growth factor-1 (IGF-1), and hepatocyte growth factor (HGF) (31). NF1-related PNFs can progress to atypical neurofibromatous neoplasms of uncertain biological potential (ANNUBP), which are hypothesized to be precursor lesions of MPNST. In addition to NF1 loss, ANNUBP often harbor deletions of CDKN2A/B (Cyclin-Dependent Kinase Inhibitor 2A/B). Additionally, MPNSTs frequently acquire mutations in SUZ12 or EED, subunits of the polycomb repressive complex 2 (PRC2) for chromatin remodeling. Loss of PRC2 enhances Ras-driven gene transcription (32). Also, MPNSTs often overexpress several RTKs, including epidermal growth factor receptor (EGFR), HGF receptor (HGFR or MET), platelet-derived growth factor receptor (PDGFR), and insulin-like growth factor-1 receptor (IGF-1R), potentially resulting in autocrine signaling. These aberrantly-activated RTKs can initiate Ras-independent survival signals through mediators, such as the STAT (signal transducers and activators of transcription) transcription factors (33).
The NF2 gene encodes merlin for moesin, ezrin, and radixin-like protein (17, 18), which shares similarity to the protein 4.1, ezrin, radixin, and moesin (FERM) family of membrane-associated proteins that link cell-surface receptors to the actin cytoskeleton (34). Loss of merlin results in defective cell-cell adhesion via destabilizing adherens junctions, which correlates with abnormal activation of focal adhesion kinase (FAK), a downstream target of ECM-binding integrins and MET (35). Merlin negatively regulates ligand-induced internalization and recycling of multiple RTKs, such as EGFR and PDGFR (34) (Figure 1A). Cells lacking merlin also show elevated levels of several other RTKs, including IGF-1R, MET, and the EGFR family members ErbB2 and ErbB3 (36, 37). These RTKs activate ERKs and AKT/mTOR, promoting translation and cell growth. Through binding to angiomotin, merlin inhibits p21-activated kinases (PAKs), and PAKs reciprocally phosphorylate and inactivate merlin (38–40). Merlin-deficient cells display deregulated PAK and enhanced invasiveness.
Additionally, merlin suppresses the Hippo/large tumor suppressor kinase 1/2 (LATS1/2)/Yes-associated protein (YAP) pathway (41, 42). Merlin inhibits proteasomal degradation of LATS1/2 by interacting with the E3 ubiquitin ligase CRL4DCAF1 in the nucleus (43). Therefore, merlin deficiency is associated with enhanced YAP-dependent gene transcription and cell proliferation. Further, loss of merlin resulted in mTORC1 activation, leading to phosphorylation of ribosomal protein S6 and enhanced cap-dependent protein synthesis via inhibition of the eIF4E-binding protein (4E-BP) (44, 45). NF2-deficient cells also display mTORC2 activation. Inhibition of mTORC1 by rapamycin amplifies AKT activation by mTORC2 (46, 47).
The molecular mechanisms by which inactivation of the LZTR1 or SMARCB1/INI1 gene in addition to the NF2 gene causes schwannomatosis are not understood. Mutations in LZTR1 also occur in several cancer types, including ~22% glioblastomas (48). Introducing LZTR1 to LZTR1-mutated glioblastoma cells decreases cyclin A and polo-like kinase 1 expression, indicating the importance of LZTR1 in cell cycle progression. As a member of the BTB-kelch superfamily, LZTR1 acts as an adaptor for cullin 3 (Cul3)-containing E3 ubiquitin ligases (27) and facilitates polyubiquitination and degradation of Ras (49). Cul3-containing ubiquitin ligases promote cell differentiation, and LZTR1-depleted Schwann cells have gene expression signatures consistent with a demyelinated proliferative phenotype. Additionally, recent evidence indicates that LZTR1-mutant schwannomatosis schwannomas exhibit deregulated VEGF receptor, ErbB3, and ERK signaling (25). SMARCB1 is a component of the SWI/SNF chromatin remodeling complex, which affects the accessibility of genes to transcription factors and RNA polymerases (50). Loss of SMARCB1 function is associated with deregulated Hedgehog/GLI and WNT/β-catenin signaling. Additionally, SWI/SNF antagonizes PRC function, and SMARCB1 deficiency results in PRC placing repressive histone methylation marks on other tumor suppressor genes, such as CDKN2A (51).
It should be emphasized that although NF1-, NF2-, and schwannomatosis-related tumors have distinct genetic origins, they share downstream activation of several molecular targets (1, 2, 6, 16, 25, 52). Collectively, these deregulated signaling pathways due to tumor suppressor loss in NF-associated tumors provide several targets for therapeutic development.
Unique Challenges for Developing Medical Therapies for NF
Neurofibromatoses are systemic, life-long diseases with diverse manifestations. Drug safety and high efficacy must be carefully considered for these patients. Due to their anatomical locations, routine biopsies of NF-associated tumors may not be possible. Determination of appropriate endpoints and identification of noninvasive biomarkers to monitor drug pharmacodynamics and predict patient response are of great importance. Owing to their benign nature, NF-associated tumors exhibit variable growth patterns. NF1-related PNFs tend to rapidly expand during childhood but develop a more indolent growth pattern in adults. NF2-associated VS grow slowly at one-to-two millimeters per year with occasional increases in growth rates. Therefore, reduction in tumor volume may not be an ideal endpoint, and other metrics, such as time to progression, hearing loss, and pain, should be considered for drug efficacy (53–55). NF2 and schwannomatosis are rare diseases, and patients may be difficult to recruit to clinical trials unless the drugs are effective and well tolerated. For most NF-associated tumors, medication may need to be taken for years. The dosing regimen should be as simple as possible to aid in patient compliance, with a daily oral tablet being ideal. In addition, patients being recruited to clinical trials should be asked whether they are taking any drugs or supplements as there could be toxicities when combining over-the-counter (OTC) with investigational agents.
From identification of targets deregulated in NF-related tumors using traditional and systems biology approaches (56–59), it is anticipated that additional targeted compounds will be successful. The evaluation of these targeted drugs has been the focus of several recent reviews. Below, we summarize the current research on natural compounds in NF-associated tumors (Table 1).
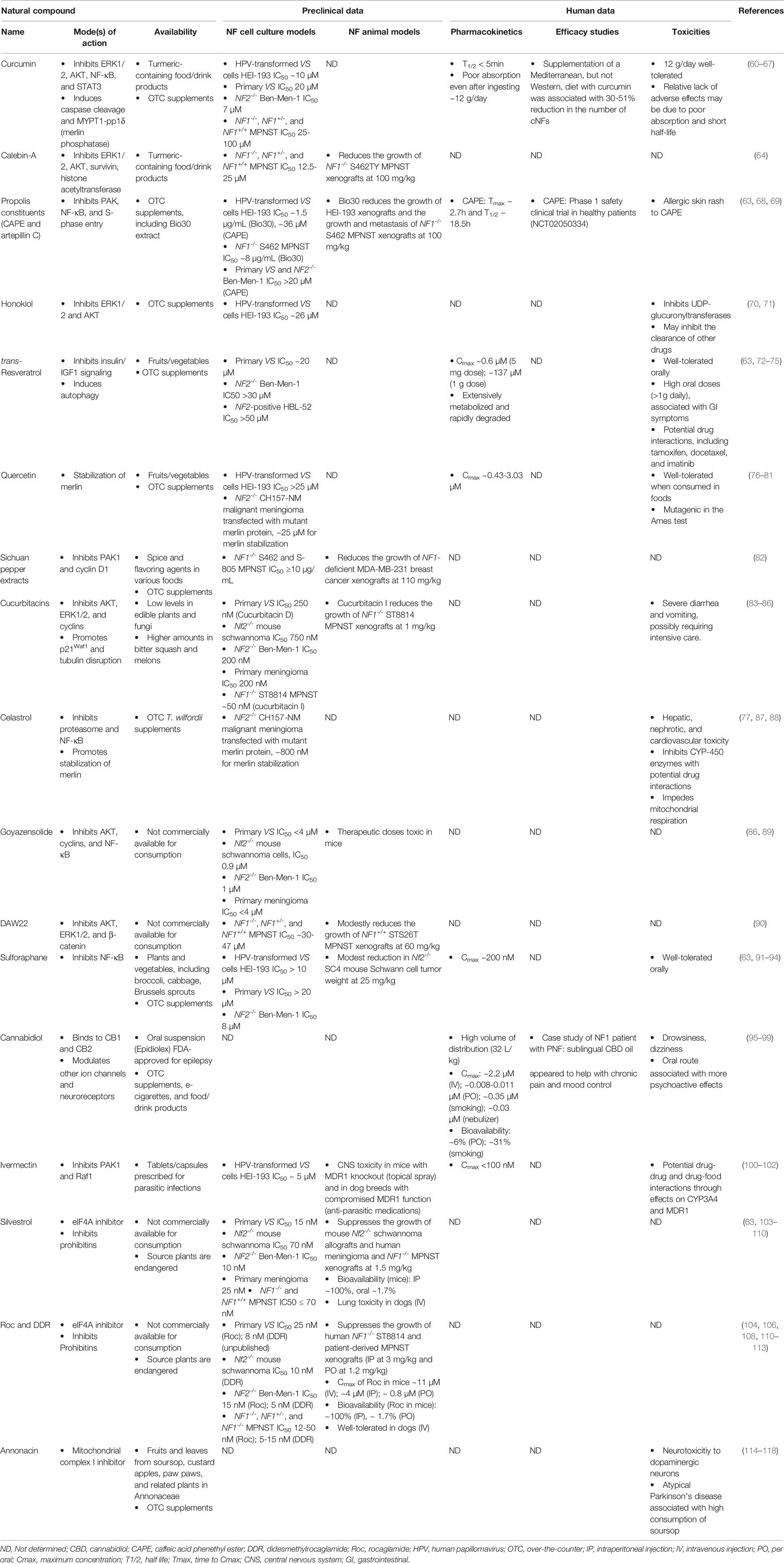
Table 1 The natural compounds evaluated in NF-related models and their mechanisms of action, preclinical data, and human evaluation.
Natural Compounds for Preventative Purposes and Potential Treatments of NF
Natural compounds from terrestrial microbes, higher plants, and marine organisms have been studied as cancer chemotherapeutic agents for several decades. Over the last 40 years, ~50% of FDA-approved drugs are natural products, natural product derivatives, or synthesized compounds based on pharmacophores originally identified in natural products (119). For example, the DNA-intercalating anti-neoplastic agents anthracyclines are made by Streptomyces bacteria. The microtubule-disruptor Taxol® (paclitaxel) was originally isolated from the bark of Pacific yew. Due to the slow-growing nature of this plant, Taxol is manufactured semi-synthetically from a precursor or produced by plant cell culture. The topoisomerase I inhibitor camptothecin was obtained from Camptotheca acuminata (happy tree). Several camptothecin analogs, including topotecan (Hycamtin®) and irinotecan (Camptosar®), have been synthesized. Trabectedin (Yondelis®, ecteinascidin 743, ET-743), which interferes with transcription and related processes, was discovered from extracts of the sea squirt Ecteinascidia turbinata and later found to come from Candidatus Endoecteinascidia frumentensis, a γ-proteobacterium living in symbiosis with the sea squirt. Since natural compounds tend to have diverse structural complexity and may inhibit molecules previously thought to be untargetable, the U.S. National Cancer Institute has divisions focused on drug discovery within the natural product space aiming to identify agents that inhibit difficult targets. Also, natural compounds have served valuable roles as probes to delineate the signaling pathways important for cell growth (103).
In addition to anti-neoplastic activity, natural compounds may possess antioxidant and anti-inflammatory properties and are particularly attractive as adjunct therapies for patients with tumor predisposition syndromes, including NF. Due to limited treatment options, NF patients often take them as dietary supplements for health enhancement purposes. Although much research has been conducted to evaluate anti-tumor activities of natural products, most studies only report their in vitro effects. Sometimes high doses are used, which may not be achievable or even desirable in vivo. At high concentrations, small molecules may cause off-target effects, unwanted redox activities, and anomalous plasma membrane permeability. Some natural dietary supplements are labeled as safe with health benefits, but these claims have not been validated. Many supplements are crude extracts, containing a mixture of compounds with the active components unknown, and lot-to-lot variability could contribute to irreproducible results. Compound sourcing may affect the activity of natural products. Also, some supplements may have dangerous contaminants, such as heavy metals, or may not contain the claimed ingredients. Therefore, dietary supplements should be carefully verified for overall chemical composition and safety (120). For purified single-chemical entity natural products that may be developed into new therapies for NF, it is important that their biological activities be carefully investigated and that they be treated as tractable hits, defined as compounds with rational structure-activity relationships. Here we summarize the natural compounds (Figure 2) that have been evaluated in NF-related tumor cell and animal models.
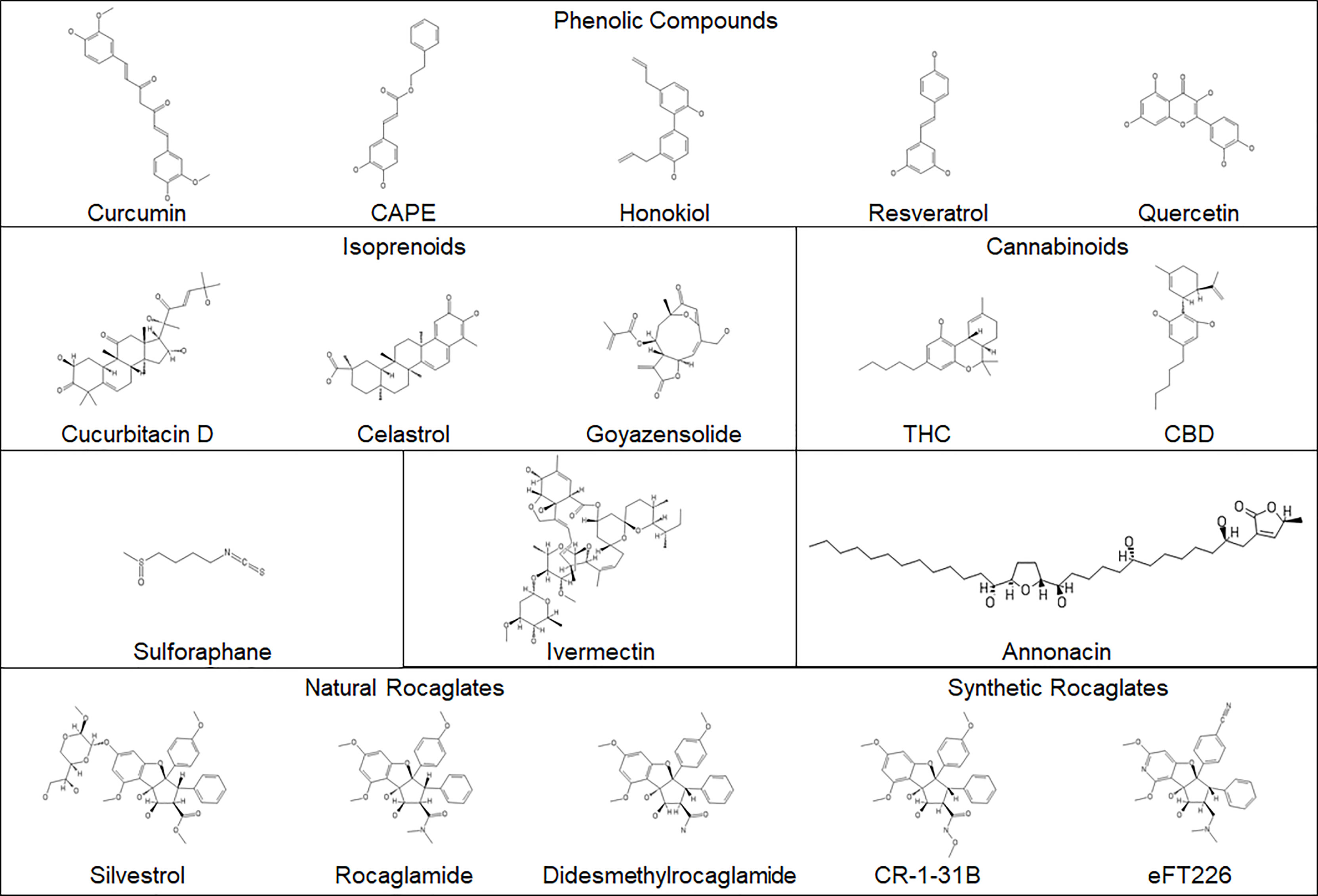
Figure 2 The structures of the natural compounds evaluated in NF-associated disease. Synthetic rocaglates are included for comparison.
Phenolic Compounds
Characterized by the presence of one or more phenol functional groups, these compounds comprise flavonoids, usually found in herbs, citrus fruits, and other plants, and non-flavonoids, also present in plants and including the curcuminoids in turmeric (121). However, phenolic compounds may be difficult to develop into drugs as many nonspecifically perturb cell membrane and alter protein function. The sections below summarize several phenolic compounds that have been evaluated in NF-related tumor models.
(i) Curcumin and other curcuminoids. The diarylheptanoid curcumin is isolated from turmeric (Curcuma longa) and comprises 2-5% of the plant rhizome. Turmeric is commonly used in Indian cooking and is a component in Ayurvedic medicine for treating infections, inflammation, and other chronic conditions. Curcumin possesses anti-inflammatory properties through inhibition of the NF-κB pathway and suppression of phospho-STAT3 in cancer cells at relatively high IC50 (50% inhibitory concentration; ~20µM) (60, 61). However, curcumin has also been shown to induce apoptosis by increasing pro-inflammatory oxidative damage. Using HEI-193, a human papillomavirus oncogene-transformed NF2-associated VS cell line, curcumin inhibits colony formation at ~10µM by downregulating the ERK, AKT, and NF-κB pathways while increasing free radical-mediated apoptosis (62) (Table 1). These results suggest cell context-dependent effects of curcumin. Also, curcumin enhances the expression of heat shock protein 70 (HSP70), a molecular chaperone associated with drug resistance. Combining curcumin with the pan-HSP inhibitor KNK437 yields synergistic growth inhibition in HEI-193 cells. The use of HEI-193 as an NF2-related cell model is of concern as viral oncogene transformation alters growth behavior of benign schwannoma cells. Other NF2-associated schwannoma models should be evaluated to confirm the findings.
Previously, we found that the IC50 value of curcumin was ~20µM in primary VS cells (63) and ~7µM in NF2-deficient Ben-Men-1 meningioma cells. Curcumin also inhibits proliferation of NF1-deficient MPNST cells at IC50 values of 25-100µM (64). A related curcuminoid, calebin-A, reduces cell growth at IC50 values ranging from 12.5-25µM and decreases the levels of phospho-AKT and survivin in the same MPNST cell lines. Calebin-A shows modest anti-tumor activity in an NF1-deficient MPNST xenograft model when dosed at 100mg/kg (Table 1). A dietary study in a small number of NF1 patients fed a Mediterranean diet supplemented with curcumin reported a 30-51% reduction in the number of CNFs, while a Western diet with the same supplement did not show any tumor inhibition (65). A larger study with well-defined objective outcomes is required to draw firm conclusions about the efficacy of curcumin in NF1 patients.
Curcumin has a short half-life in aqueous solution (<5 minutes), making it imperative that experiments be rigorously controlled to ensure the observed treatment effects are due to curcumin but not its degradation products (66). The instability of curcumin along with poor bioavailability may explain why many early trials did not show any clinical benefits. To increase bioavailability, alternative delivery approaches have been sought, including protein nanoparticles, liposomal formulations, and erythrocytes coated with porous nanoparticles (66, 67). It remains to be seen if these strategies could improve the efficacy of curcumin.
(ii) Propolis constituents. Also called bee glue, propolis is found in beehives and has been used since the ancient Egyptian civilization for its anti-inflammatory, anti-bacterial, and wound-healing properties. It comprises an admixture of different chemicals with wide variability in composition depending upon factors, such as the regional flora, climate, and preparation method (68). Therefore, studies using crude propolis extracts are highly liable to lot-to-lot variabilities in their biological effects. Chinese red propolis inhibits VEGF expression and contains at least 12 components, including the phenolic ester, caffeic acid phenethyl ester (CAPE), and the flavonoid, kaempferol. Turkish propolis induces G1 arrest and apoptosis in cancer cells and contains six major constituents, including caffeic acid and CAPE. Polish propolis inhibits S-phase entry, decreases cell viability, and contains multiple flavonoids. Bio30 is a water-miscible extract of New Zealand propolis, which contains multiple phenolic compounds including CAPE, and suppresses the growth of HEI-193 schwannoma and NF1-deficient S462 MPNST cells (69) (Table 1). Among these propolis specimens, a common constituent is CAPE. The IC50 of this compound in HEI-193 schwannoma cells was ~36 µM. We also found that CAPE has little growth-inhibitory activity in VS and meningioma cells at doses <=20µM (63). It is not known whether it is possible to achieve this high concentration of CAPE in humans.
(iii) Honokiol. As a biphenolic lignan from the bark of Magnolia trees, honokiol is commonly consumed as an ingredient in herbal tea preparations for treating anxiety in Asia and possesses anti-tumor activity (70). In HEI-193 schwannoma cells, honokiol inhibits proliferation by decreasing phospho-AKT and ERK1/2 at an IC50 of ~26µM (71) (Table 1). However, this compound has not been tested in other NF2- or NF1-related models. Also, honokiol crosses the blood-brain barrier, but its oral bioavailability and plasma half-life are low (70). Several approaches, including encapsulating honokiol in nanoparticles or liposomes, are being used to enhance these properties.
(iv) trans-Resveratrol. This stilbenoid was isolated originally from white hellebore, but is found widely in various fruits and nuts, including grapes, apples, and pistachios (72). It has beneficial metabolic effects in mouse models of diet-induced diabetes by negatively modulating insulin and IGF-1 signaling. It inhibits cell proliferation by reducing the levels of cyclin D, Hippo-YAP, and β-catenin. In addition, resveratrol at doses of 5mg or less per day reduces the number of adenomas by ~40% in a mouse model of high-fat diet-induced colorectal carcinoma by promoting autophagy, while a higher dose of ~1g per day shows less inhibitory effects. These results suggest that resveratrol may have chemo-preventive effects (73). Pharmacokinetic studies indicate that a single dose of resveratrol of 5mg or 1g reaches peak plasma concentrations of 0.6 and 137µM, respectively. It is generally accepted that resveratrol at doses ≤1g can be taken long-term, but higher doses (e.g., 2.5 and 5 g) can cause gastrointestinal symptoms (74). In human VS cells and NF2-deficient Ben-Men-1 cells, resveratrol exhibits moderate antiproliferative activity at doses up to 20µM (63). In NF2-expressing HBL-52 meningioma cells, the effective dose of resveratrol to induce apoptosis is >50µM (75). Therefore, additional studies in NF-related animal models are needed to determine if resveratrol exhibits anti-tumor effects at tolerable doses.
(v) Quercetin. The pigmented pentahydroxylated flavone quercetin is widely found in oaks (genus Quercus), herbs, fruits, and vegetables. It induces apoptosis at a relatively high dose and moderately reduces cancer cell growth (76). Quercetin inhibits the growth of HEI-193 schwannoma cells and stabilizes the expression of mutant merlin proteins in NF2-deficient CH157-NM malignant meningioma cells at doses ≥25µM (77); however, these doses are not possible to be reached in humans (Table 1). Additionally, it is known to aggregate and promiscuously bind to proteins, making it less therapeutically effective and potentially toxic (78–81). The known toxic effects of quercetin include mutagenicity, prooxidant activity, and mitochondrial toxicity.
(vi) Sichuan pepper extracts. The Sichuan peppercorns harvested from the seeds of the aromatic spiny shrub Zanthoxylum piperitum are used in East Asian cuisine and traditional Chinese medicine. Peppercorn extracts inhibit proliferation of NF1-deficient MPNST and MDA-MB-231 breast cancer cells by reducing PAK1 activation and cyclin D1 levels (82) (Table 1). These extracts also suppress MDA-MB-231 xenografts, but resistant cell populations rapidly developed during treatment. Moreover, the constituents in Sichuan pepper extracts responsible for the antiproliferative effects have not been determined.
Isoprenoids
Terpenoids and steroids are isoprene-derived, with terpenes being a class of naturally-occurring hydrocarbons originally named for their discovery in turpentine, a resin distilled from conifer sap. Isoprenoids are the largest group of natural products. Although they are mainly found in plants, some classes, such as steroids, are common in animals. In plants, terpenoids are often found as aromatic compounds that play important roles in signal transduction and act as a defense against herbivores (122). With great structural diversity, terpenoids are made up of repeating units of the C5-hydrocarbon isoprene and classified based on the number and structural organization of isoprene units. Although the words terpenes and terpenoids are often used interchangeably, terpenoids properly refer to modified terpenes with additional oxygenated functional groups.
(i) Cucurbitacin. Originally isolated from the squash family (Cucurbitaceae), cucurbitacins are tetracyclic triterpenoids with a steroidal skeleton (83). These compounds are also found in several other plants and mushrooms. They often have glycosidic linkages and are classified into multiple variants according to their side chains. Cucurbitacins are contained in traditional Asian remedies for treating viral diseases and inflammatory conditions. They exhibit antiproliferative effects by disrupting microtubule polymerization. Also, they inhibit cancer cell growth by decreasing phospho-AKT and phospho-ERK, increasing the levels of p21WAF1, and promoting apoptosis (84). Cucurbitacin I effectively inhibits proliferation of NF1-null MPNST cells and induces apoptosis by decreasing STAT3 signaling (85) (Table 1). We showed that cucurbitacin D has growth-inhibitory activity against Nf2-null mouse schwannoma and human Ben-Men-1 cells at sub-micromolar IC50 concentrations by decreasing the expression of cyclins and phospho-AKT, leading to G2/M arrest (86). Despite their anti-tumor activity, cucurbitacins may cause gastrointestinal toxicity and have a low therapeutic index, which hamper their further clinical development (84).
(ii) Celastrol. Originally isolated from Tripterygium wilfordii (thunder god vine), celastrol (tripterine) is a pentacyclic triterpenoid, which exhibits anti-obesity, antioxidant, anti-inflammatory, and anti-tumor effects (87). It inhibits NF-κB signaling and multiple other pathways and reduces proliferation and invasion of cancer cells with an IC50 of ~2µM. Celastrol impedes degradation of the merlin protein in malignant meningioma cells carrying a missense mutation in the NF2 gene at ~1µM (77) (Table 1). However, celastrol has a problematic ortho-quinone methide functional group that possesses redox activity and is reactive promiscuously with the sulfur nucleophiles present in the active sites of several enzymes, including metabolic coenzymes, needed by normal cells. These features may explain the serious adverse effects on hepatic, renal, reproductive, and cardiovascular systems reported after consuming T. wilfordii supplements (88).
(iii) Goyazensolide. Isolated from Piptocoma rufescens (velvetshrub) and other members of the sunflower family (Asteraceae), goyazensolide is a sesquiterpene lactone. It was identified initially as an anti-schistosomal agent and has antiproliferative activity by inhibiting NF-κB expression and inducing apoptosis (89). We found that goyazensolide suppresses proliferation of Nf2-/- mouse schwannoma cells and NF2-deficient human meningioma cells at IC50 doses of ~1µM and less effectively in primary human VS and meningioma cells (86) (Table 1). These growth-suppressive effects appear to be due to decreased expression of AKT and cyclins, followed by G2/M arrest. Unfortunately, goyazensolide was too toxic in mice at therapeutic doses for further development.
(iv) DAW22. This sesquiterpene coumarin was isolated from the roots of Ferula ferulaeoides, a member of the carrot family (Apiaceae). It is antiproliferative and pro-apoptotic in sporadic and NF1-associated MPNST cells but at relatively high IC50 doses (30-47µM) (90) (Table 1). Also, it only modestly reduces tumor growth in NF1-expressing MPNST-bearing mice at the dose of 60mg/kg. The effects of DAW22 in NF2-related tumor cell and animal models have not been investigated.
Sulforaphane
Frequently found as a glycosidic precursor in cruciferous vegetables of the mustard family (Brassicaceae), sulforaphane is a sulfur-containing member of the isothiocyanate compound class (91). It has anti-inflammatory and anti-neoplastic activities in several types of cancer cells, partly via inhibiting NF-κB. In HEI-193 schwannoma cells, sulforaphane has growth-inhibitory effects at IC50 >10µM (92) (Table 1). We also found that it inhibited the growth of primary VS and meningioma cells at IC50 >20µM (63). Due to its short half life (93), the plasma concentrations of sulforaphane in humans peak at ~200nM (94). These results suggest that it may not be very potent against NF2-related tumors. However, sulforaphane is generally safe (93), improved formulation and delivery methods will be required to reach a therapeutic level.
Cannabinoids
Cannabis sativa, called marijuana or hemp, is used in traditional Chinese and Ayurvedic medicine, but in the West, more well-known for recreational purposes. Although Cannabis sativa varieties synthesize >100 different cannabinoids, two compounds, Δ9-tetrahydrocannabinol (THC) and cannabidiol (CBD), are primarily studied for their clinical effects. Cannabis extracts and individual cannabinoids are increasingly used in patients suffering from glaucoma, neuropathic pain, and cancer (95, 96). THC is known for its appetite stimulation and psychoactive properties and is a Schedule 1-controlled substance. Dronabinol (Marinol®, Syndros™), a synthetic THC analog, is FDA-approved for treating HIV-induced appetite loss and chemotherapy-related nausea and vomiting. Nabiximols (Sativex®), a 1:1 THC : CBD extract, is approved in ~30 countries. for multiple sclerosis-related symptoms. Cannabinoids may have anti-neoplastic activity through their effects on endocannabinoid receptors (97). The synthetic THC analog, WIN-55212-2, is an endocannabinoid receptor agonist and induces G1 cell cycle arrest (123). In some contexts, cannabinoids may be pro-tumorigenic as 100-300nM THC enhances DNA synthesis in cancer cells by endocannabinoid receptor-mediated EGFR transactivation (124). However, it should be noted that overdoses of THC may cause acute intoxication, tachycardia, aboulia, and psychosis. Also, THC and related analogs have not been assessed in NF-related cell and animal models.
In contrast, CBD is non-psychoactive and has analgesic, anxiolytic, and anticonvulsant properties (95, 96). In the U.S., chemically-synthesized CBD is legally sold as an over-the-counter supplement. Clinical trials suggest that CBD is overall well-tolerated with drowsiness and dizziness being the main adverse effects (96) (Table 1). The FDA approved Epidiolex®, a plant-derived CBD, to treat Lennox-Gastaut and Dravet syndromes, two rare forms of severe epilepsy. In addition, CBD may have helped control neuropathic pain and mood disorders in an NF1 patient with PNFs (98). A larger study is needed to confirm these findings. CBD preparations are sold in a wide variety of formulations, and the purity and safety of different products are unclear. Additional rigorous clinical examinations should be conducted to validate analgesic qualities, pharmacokinetics, and long-term safety (99).
Ivermectin
The anti-parasitic avermectins were originally isolated from Streptomyces avermitilis. Subsequently, a semi-synthetic derivative of avermectin, ivermectin, was developed for veterinary use to treat parasite infestations and was later approved by the FDA to treat river blindness and other nematode infections in humans (100, 101). Ivermectin is well tolerated and only causes mild toxicity even when taking ten times the FDA-approved dose. It has anti-tumor activity in various types of cancer. It inhibits proliferation of HEI-193 schwannoma cells at an IC50 of ~5µM by blocking PAK1 and decreasing phospho-Raf (102) (Table 1). However, the FDA-approved therapeutic dose of ivermectin in humans only reaches plasma concentrations of <100nM (100). Also, ivermectin is a substrate for the multidrug resistance 1 (MDR1) transporter, which prevents the drug from reaching high concentrations in the brain (100, 101). Thus, this drug is not likely to be effective against NF2-related VS and meningiomas.
Silvestrol, Rocaglamide, and Didesmethylrocaglamide
Rocaglates, also called flavaglines, are a large family of cyclopenta[b]benzofurans synthesized by tropical trees of the Aglaia genus in the mahogany family (Meliaceae) (104). Among this group of natural compounds, rocaglamide (also known as rocaglamide A or RocA; Figure 2) was first found to possess antileukemic activity (111) but was not further characterized biologically for some years due to its scarcity. Subsequently, a few other rocaglates with anti-proliferative activity were identified, including silvestrol which was the first flavagline with an unusual sugar-like dioxanyl ring discovered in Aglaia foveolata (105). Silvestrol inhibits proliferation of a variety of cancer cell lines at low nanomolar concentrations similar to camptothecin and paclitaxel (104). Acting as inhibitors of the eukaryotic translation initiation factor 4A (eIF4A), an RNA helicase (106), silvestrol and rocaglamide bind eIF4A and lock it onto purine-rich sequences in the 5’-untranslated region (UTR) of certain mRNAs, leading to translation inhibition (107, 112) (Figure 1B). Rocaglates may also bind to prohibitins, resulting in inhibition Raf/ERK signaling (108).
We have shown that MPNST, VS, and meningioma tumors frequently overexpress the eIF4F components, including eIF4A (63, 109). Genetic depletion of eIF4A via RNA interference and pharmacological inhibition by silvestrol effectively suppress proliferation of NF2-deficient tumor and NF1-deficient MPNSTs cells (Table 1). As an eIF4A inhibitor, silvestrol reduces the protein levels of multiple cyclins and oncogenic kinases, including AKT, ERK, and FAK, leading to G2/M arrest and apoptosis. Also, it profoundly suppresses tumor growth of Nf2-/- schwannomas and NF1-/- MPNSTs. However, a toxicology study in dogs revealed that silvestrol caused lung damage (https://dtp.cancer.gov/publications/silvestrol_rocaglamide_studies.pdf). Consequently, its further clinical development was suspended (103).
By side-by-side comparing 10 silvestrol-related rocaglates lacking the dioxanyl ring (110), we identified rocaglamide and didesmethylrocaglamide (also called RocB) with growth-inhibitory activity comparable to silvestrol in MPNST, schwannoma, and meningioma cells at low nanomolars (113) (Table 1). Both rocaglamide and didesmethylrocaglamide reduce expression of multiple oncogenic kinases IGF-1R, AKT, and ERKs while simultaneously inducing DNA damage response, caspase cleavage, and apoptosis. Interestingly, rocaglamide exhibited 50% oral bioavailability and was not susceptible to multi-drug resistance-1 efflux. When delivered by oral gavage or intraperitoneal injection, rocaglamide potently suppressed tumor growth in an orthotopic MPNST model. Most importantly, rocaglamide was well tolerated in mice and did not induce pulmonary toxicity in dogs. Furthermore, both rocaglamides exhibited strong anti-tumor effects against other sarcomas, including osteosarcoma, Ewing sarcoma, and rhabdomyosarcoma. These results warrant a clinical trial to evaluate these rocaglamides in patients with sarcomas and those afflicted by NF. It should also be mentioned that the synthetic rocaglates have also been developed that retain the core scaffold responsible for eIF4A inhibition, while incorporating side chain modifications to optimize pharmacokinetic and pharmacodynamic properties. One such compound, (-)-CR-1-31B prolongs survival of mice bearing pancreatic adenocarcinoma allografts (125). Another synthetic rocaglate-like compound, eFT226 (zotatifin), has anti-tumor activity against several fibroblast growth factor receptor- and ErbB2-driven cancers (126) and has recently entered a phase 1/2 clinical trial in patients with K-Ras- or RTK-driven advanced solid tumors (ClinicalTrials.gov Identifier: NCT04092673).
Annonacin
Also called guyabano or graviola, soursop is the fruit of Annona muricata, a member of the custard apple family (Annonaceae). With its pleasant aroma, soursop is used to make juices and as a flavoring agent (Table 1). Extracts from fruits and leaves of Annona muricata are reported to have anti-tumor activity against multiple tumor types, including anecdotally shrinking NF2-associated VS (114–116). One active component with anti-proliferative activity in soursop is annonacin, an acetogenin. However, this compound inhibits mitochondrial complex I, elicits severe neurotoxic effects, and excessive consumption of Annona plants and supplements are associated with atypical Parkinson’s disease (117). These serious adverse effects prevent it from further development as an anti-tumor agent (118).
Additionally, several other natural compounds may be of interest to NF patients. Silibinin, the main flavonolignan component in the extract of milk thistle (Silybum marianum) seeds, is used to treat hepatotoxicity caused by poisoning from the death cap mushroom Amanita phalloides. It inhibits lung cancer cell proliferation by suppressing AKT and ERK activation (127). Gingerol, an alkylphenol found in ginger, decreases the growth of breast cancer cells by lowering the expression of EGFR and β1-integrin (128). Shikonin, a naphthoquinone pigment found in the root of Lithospermum erythrorhizon in the borage family (Boraginaceae), is used in traditional Chinese medicine for treating inflammatory diseases. It suppresses leukemia cell growth by decreasing phospho-AKT and ERKs (129). Angelica sinensis, commonly known as dong quai (danggui, dang’ui), is an herb in the carrot family (Apiaceae) used in traditional Asian medicine for reproductive disorders. An active component in dong quai is the γ-lactone N-butylidenephthalide, which inhibits proliferation of gastric carcinoma cells by increasing the levels of REDD1 (regulated in development and DNA damage responses 1), a negative regulator of the mTOR pathway (130). Genistein is an isoflavone found in soy-based foods, such as soymilk. It induces apoptosis, reduces tumor vascularity, and suppresses metastasis by inhibiting cyclins and AKT activation (131). Epigallocatechin gallate is the most abundant catechin ester in green tea (Camellia sinensis). It decreases phosphorylation of PI3K and AKT and reduces IGF-1R levels in some cancer cells (132). Since these natural compounds inhibit the signaling pathways frequently activated in NF-associated tumors, it will be interesting to see whether they have anti-tumor effects in these tumors.
Conclusion
NF are characterized by multiple nervous system tumors and other non-tumoral manifestations. Surgery and/or radiation are the standard of care to control the tumor burden but often incur significant morbidities. The recent approval of the MEK inhibitor selumetinib to treat NF1-associated PNF suggests that medications targeting specific NF signaling pathways can be successful. However, combining selumetinib with other targeted agent(s) will be needed to achieve a cure. Although not FDA-approved, bevacizumab is used off-label for NF2 patients, with some of them experiencing tumor reduction and improved hearing. Recently, using traditional and systems biology approaches (56–59), several targeted compounds and compound combinations with anti-tumor effects in NF-related models have been identified, and some are being evaluated in humans.
Natural compounds have been investigated as potential cancer therapies for several decades, and many are on the WHO’s List of Essential Medicines. Many patients with NF-related tumors take natural products as supplements in the hope of reducing tumor growth. While several natural compounds can inhibit signal transduction pathways deregulated in NF-associated tumors (Figures 1 and 2), most have only been tested in cell culture models and exhibit high IC50 values that may not be achievable in vivo (Table 1). In some cases, the cell culture and animal models used do not accurately reflect the pathogenesis of NF tumors. Therefore, the published data should be interpreted cautiously, with patients consulting their physicians before taking any natural compounds. Of the natural compounds that demonstrated potent anti-tumor activity in both NF-related models, the eIF4A inhibitors rocaglamide and didesmethylrocaglamide effectively block the expression multiple oncogenic kinases with good bioavailability and toxicity profiles and are expected to enter clinical trials in the future.
Author Contributions
AA: project conception and manuscript writing. JO: project conception and manuscript writing, DW: project conception. AK: project conception, and L-SC: project conception and supervision and manuscript writing and authorship. All authors contributed to the article and approved the submitted version.
Funding
This study was supported by grants from the Galloway Family, Advocure NF2, CancerFree KIDS, Sunbeam Foundation, NF2 Biosolutions, Department of Defense W81XWH-18-1-0547, and National Institute of Neurological Disorders and Stroke R01NS113854 to L-SC, the Department of Defense W81XWH-14-1-0167 to DW, and National Cancer Institute P01CA125066 to AK and P30CA16058 to The OSU Comprehensive Cancer Center. The funder was not involved in the study design, collection, analysis, interpretation of data, the writing of this article or the decision to submit it for publication.
Conflict of Interest
L-SC and AK: Patent: U.S. Provisional Application No. 19/55304 Anticancer rocaglamide derivatives. DW: Consultant: CereXis.
The remaining authors declare that the research was conducted in the absence of any commercial or financial relationships that could be construed as a potential conflict of interest.
Publisher’s Note
All claims expressed in this article are solely those of the authors and do not necessarily represent those of their affiliated organizations, or those of the publisher, the editors and the reviewers. Any product that may be evaluated in this article, or claim that may be made by its manufacturer, is not guaranteed or endorsed by the publisher.
References
1. Plotkin SR, Wick A. Neurofibromatosis and Schwannomatosis. Semin Neurol (2018) 38(1):73–85. doi: 10.1055/s-0038-1627471
2. Evans DGR, Salvador H, Chang VY, Erez A, Voss SD, Schneider KW, et al. Cancer and Central Nervous System Tumor Surveillance in Pediatric Neurofibromatosis 1. Clin Cancer Res (2017) 23(12):e46–53. doi: 10.1158/1078-0432.CCR-17-0589
3. Viskochil D, Buchberg AM, Xu G, Cawthon RM, Stevens J, Wolff RK, et al. Deletions and a Translocation Interrupt a Cloned Gene at the Neurofibromatosis Type 1 Locus. Cell (1990) 62(1):187–92. doi: 10.1016/0092-8674(90)90252-A
4. Wallace MR, Marchuk DA, Andersen LB, Letcher R, Odeh HM, Saulino AM, et al. Type 1 Neurofibromatosis Gene: Identification of a Large Transcript Disrupted in Three NF1 Patients. Science (1990) 249(4965):181–6. doi: 10.1126/science.2134734
5. Xu GF, O’Connell P, Viskochil D, Cawthon R, Robertson M, Culver M, et al. The Neurofibromatosis Type 1 Gene Encodes a Protein Related to GAP. Cell (1990) 62(3):599–608. doi: 10.1016/0092-8674(90)90024-9
6. Chamseddin BH, Le LQ. Management of Cutaneous Neurofibroma: Current Therapy and Future Directions. Neurooncol Adv (2019) 2(Suppl 1):i107–16. doi: 10.1093/noajnl/vdz034
7. Staedtke V, Bai R-Y, Blakeley JO. Cancer of the Peripheral Nerve in Neurofibromatosis Type 1. Neurotherapeutics (2017) 14(2):298–306. doi: 10.1007/s13311-017-0518-y
8. Kim A, Stewart DR, Reilly KM, Viskochil D, Miettinen MM, Widemann BC. Malignant Peripheral Nerve Sheath Tumors State of the Science: Leveraging Clinical and Biological Insights Into Effective Therapies. Sarcoma (2017) 2017:7429697. doi: 10.1155/2017/7429697
9. Costa ADA, Gutmann DH. Brain Tumors in Neurofibromatosis Type 1. Neurooncol Adv (2020) 2(Supplement 1):i85–97. doi: 10.1093/noajnl/vdz040
10. Laycock-van Spyk S, Thomas N, Cooper DN, Upadhyaya M. Neurofibromatosis Type 1-Associated Tumours: Their Somatic Mutational Spectrum and Pathogenesis. Hum Genomics (2011) 5(6):623–90. doi: 10.1186/1479-7364-5-6-623
11. Gross AM, Wolters PL, Dombi E, Baldwin A, Whitcomb P, Fisher E, et al. Selumetinib in Children With Inoperable Plexiform Neurofibromas. N Engl J Med (2020) 382(15):1430–42. doi: 10.1056/NEJMoa1912735
12. Weiss BD, Wolters PL, Plotkin SR, Widemann B, Tonsgard JH, Blakeley J, et al. NF106: A Neurofibromatosis Clinical Trials Consortium Phase II Trial of the MEK Inhibitor Mirdametinib (PD-0325901) in Adolescents and Adults With NF1-Related Plexiform Neurofibromas. J Clin Oncol (2021) 39(7):797–806. doi: 10.1200/JCO.20.02220
13. de Blank PMK, Fisher MJ, Liu GT, Gutmann DH, Listernick R, Ferner RE, et al. Optic Pathway Gliomas in Neurofibromatosis Type 1: An Update: Surveillance, Treatment Indications, and Biomarkers of Vision. J Neuroophthalmol (2017) 37(Suppl 1):S23–32. doi: 10.1097/WNO.0000000000000550
14. Fangusaro J, Onar-Thomas A, Young Poussaint T, Wu S, Ligon AH, Lindeman N, et al. Selumetinib in Paediatric Patients With BRAF-Aberrant or Neurofibromatosis Type 1-Associated Recurrent, Refractory, or Progressive Low-Grade Glioma: A Multicentre, Phase 2 Trial. Lancet Oncol (2019) 20(7):1011–22. doi: 10.1016/S1470-2045(19)30277-3
15. Wu LMN, Lu QR. Therapeutic Targets for Malignant Peripheral Nerve Sheath Tumors. Future Neurol (2019) 14(1):FNL7. doi: 10.2217/fnl-2018-0026
16. Evans DGR, Salvador H, Chang VY, Erez A, Voss SD, Druker H, et al. Cancer and Central Nervous System Tumor Surveillance in Pediatric Neurofibromatosis 2 and Related Disorders. Clin Cancer Res (2017) 23(12):e54–61. doi: 10.1158/1078-0432.CCR-17-0590
17. Rouleau GA, Merel P, Lutchman M, Sanson M, Zucman J, Marineau C, et al. Alteration in a New Gene Encoding a Putative Membrane-Organizing Protein Causes Neuro-Fibromatosis Type 2. Nature (1993) 363(6429):515–21. doi: 10.1038/363515a0
18. Trofatter JA, MacCollin MM, Rutter JL, Murrell JR, Duyao MP, Parry DM, et al. A Novel Moesin-, Ezrin-, Radixin-Like Gene Is a Candidate for the Neurofibromatosis 2 Tumor Suppressor. Cell (1993) 72(5):791–800. doi: 10.1016/0092-8674(93)90406-G
19. Welling DB, Packer MD, Akhmametyeva EM, Chang L-S. The Biology and Genetics of Vestibular Schwannomas. In: Bambakidis N, Megerian C, Spetzler R, editors. Surgery of the Cerebellopontine Angle. Hamilton, Ontario, CA: B.C. Decker, Inc (2008). p. 119–34.
20. Kruchko C, Ostrom QT, Gittleman H, Barnholtz-Sloan JS. The CBTRUS Story: Providing Accurate Population-Based Statistics on Brain and Other Central Nervous System Tumors for Everyone. Neuro Oncol (2018) 20(3):295–8. doi: 10.1093/neuonc/noy006
21. Goutagny S, Kalamarides M. Meningiomas and Neurofibromatosis. J Neuro-Oncol (2010) 99(3):341–7. doi: 10.1007/s11060-010-0339-x
22. Plotkin SR, Duda DG, Muzikansky A, Allen J, Blakeley J, Rosser T, et al. Multicenter, Prospective, Phase II and Biomarker Study of High-Dose Bevacizumab as Induction Therapy in Patients With Neurofibromatosis Type 2 and Progressive Vestibular Schwannoma. J Clin Oncol (2019) 37(35):3446–54. doi: 10.1200/JCO.19.01367
23. Blakeley JO, Ye X, Duda DG, Halpin CF, Bergner AL, Muzikansky A, et al. Efficacy and Biomarker Study of Bevacizumab for Hearing Loss Resulting From Neurofibromatosis Type 2–Associated Vestibular Schwannomas. J Clin Oncol (2016) 34(14):1669–75. doi: 10.1200/JCO.2015.64.3817
24. Nunes FP, Merker VL, Jennings D, Caruso PA, di Tomaso E, Muzikansky A, et al. Bevacizumab Treatment for Meningiomas in NF2: A Retrospective Analysis of 15 Patients. PloS One (2013) 8(3):e59941. doi: 10.1371/journal.pone.0059941
25. Mansouri S, Suppiah S, Mamatjan Y, Paganini I, Liu JC, Karimi S, et al. Epigenomic, Genomic, and Transcriptomic Landscape of Schwannomatosis. Acta Neuropathol. (2021) 141(1):101–16. doi: 10.1007/s00401-020-02230-x
26. Smith MJ, Isidor B, Beetz C, Williams SG, Bhaskar SS, Richer WW, et al. Mutations in LZTR1 Add to the Complex Heterogeneity of Schwannomatosis. Neurology (2015) 84(2):141–7. doi: 10.1212/WNL.0000000000001129
27. Kehrer-Sawatzki H, Farschtschi S, Mautner V-F, Cooper DN. The Molecular Pathogenesis of Schwannomatosis, a Paradigm for the Co-Involvement of Multiple Tumour Suppressor Genes in Tumorigenesis. Hum Genet (2017) 136(2):129–48. doi: 10.1007/s00439-016-1753-8
28. Karnoub AE, Weinberg RA. Ras Oncogenes: Split Personalities. Nat Rev Mol Cell Biol (2008) 9(7):517–31. doi: 10.1038/nrm2438
29. Goncalves MD, Hopkins BD, Cantley LC. Phosphatidylinositol 3-Kinase, Growth Disorders, and Cancer. N Engl J Med (2018) 379(21):2052–62. doi: 10.1056/NEJMra1704560
30. Laplante M, Sabatini DM. mTOR Signaling in Growth Control and Disease. Cell (2012) 149(2):274–93. doi: 10.1016/j.cell.2012.03.017
31. Lemberg KM, Wang J, Pratilas CA. From Genes to -Omics: The Evolving Molecular Landscape of Malignant Peripheral Nerve Sheath Tumor. Genes (2020) 11(6):691. doi: 10.3390/genes11060691
32. De Raedt T, Beert E, Pasmant E, Luscan A, Brems H, Ortonne N, et al. PRC2 Loss Amplifies Ras-Driven Transcription and Confers Sensitivity to BRD4-Based Therapies. Nature (2014) 514(7521):247–51. doi: 10.1038/nature13561
33. Rad E, Tee AR. Neurofibromatosis Type 1: Fundamental Insights Into Cell Signalling and Cancer. Semin Cell Dev Biol (2016) 52:39–46. doi: 10.1016/j.semcdb.2016.02.007
34. Casaletto JB, McClatchey AI. Spatial Regulation of Receptor Tyrosine Kinases in Development and Cancer. Nat Rev Cancer. (2012) 12(6):387–400. doi: 10.1038/nrc3277
35. Fernandez-Valle C, Tang Y, Ricard J, Rodenas-Ruano A, Taylor A, Hackler E, et al. Paxillin Binds Schwannomin and Regulates Its Density-Dependent Localization and Effect on Cell Morphology. Nat Genet (2002) 31(4):354–62. doi: 10.1038/ng930
36. Lallemand D, Manent J, Couvelard A, Watilliaux A, Siena M, Chareyre F, et al. Merlin Regulates Transmembrane Receptor Accumulation and Signaling at the Plasma Membrane in Primary Mouse Schwann Cells and in Human Schwannomas. Oncogene (2009) 28(6):854–65. doi: 10.1038/onc.2008.427
37. Bush ML, Burns SS, Oblinger J, Davletova S, Chang L-S, Welling DB, et al. Treatment of Vestibular Schwannoma Cells With ErbB Inhibitors. Otol Neurotol (2012) 33(2):244–57. doi: 10.1097/MAO.0b013e31823e287f
38. Kissil JL, Wilker EW, Johnson KC, Eckman MS, Yaffe MB, Jacks T. Merlin, the Product of the Nf2 Tumor Suppressor Gene, Is an Inhibitor of the P21-Activated Kinase, Pak1. Mol Cell (2003) 12(4):841–9. doi: 10.1016/S1097-2765(03)00382-4
39. Morrison H, Sperka T, Manent J, Giovannini M, Ponta H, Herrlich P. Merlin/neurofibromatosis Type 2 Suppresses Growth by Inhibiting the Activation of Ras and Rac. Cancer Res (2007) 67(2):520–7. doi: 10.1158/0008-5472.CAN-06-1608
40. Yi C, Troutman S, Fera D, Stemmer-Rachamimov A, Avila JL, Christian N, et al. A Tight Junction-Associated Merlin-Angiomotin Complex Mediates Merlin’s Regulation of Mitogenic Signaling and Tumor Suppressive Functions. Cancer Cell (2011) 19(4):527–40. doi: 10.1016/j.ccr.2011.02.017
41. Hamaratoglu F, Willecke M, Kango-Singh M, Nolo R, Hyun E, Tao C, et al. The Tumour-Suppressor Genes NF2/Merlin and Expanded Act Through Hippo Signalling to Regulate Cell Proliferation and Apoptosis. Nat Cell Biol (2006) 8(1):27–36. doi: 10.1038/ncb1339
42. Zhao B, Li L, Lei Q, Guan K-L. The Hippo–YAP Pathway in Organ Size Control and Tumorigenesis: An Updated Version. Genes Dev (2010) 24(9):862–74. doi: 10.1101/gad.1909210
43. Li W, You L, Cooper J, Schiavon G, Pepe-Caprio A, Zhou L, et al. Merlin/NF2 Suppresses Tumorigenesis by Inhibiting the E3 Ubiquitin Ligase CRL4(DCAF1) in the Nucleus. Cell (2010) 140(4):477–90. doi: 10.1016/j.cell.2010.01.029
44. James MF, Han S, Polizzano C, Plotkin SR, Manning BD, Stemmer-Rachamimov A, et al. NF2/merlin Is a Novel Negative Regulator of mTOR Complex 1, and Activation of Mtorc1 Is Associated With Meningioma and Schwannoma Growth. Mol Cell Biol (2009) 29(15):4250–61. doi: 10.1128/MCB.01581-08
45. López-Lago MA, Okada T, Murillo MM, Socci N, Giancotti FG. Loss of the Tumor Suppressor Gene NF2, Encoding Merlin, Constitutively Activates Integrin-Dependent Mtorc1 Signaling. Mol Cell Biol (2009) 29(15):4235–49. doi: 10.1128/MCB.01578-08
46. Beauchamp RL, James MF, DeSouza PA, Wagh V, Zhao W-N, Jordan JT, et al. A High-Throughput Kinome Screen Reveals Serum/Glucocorticoid-Regulated Kinase 1 as a Therapeutic Target for NF2-Deficient Meningiomas. Oncotarget (2015) 6(19):16981–97. doi: 10.18632/oncotarget.4858
47. Angus SP, Oblinger JL, Stuhlmiller TJ, DeSouza PA, Beauchamp RL, Witt L, et al. EPH Receptor Signaling as a Novel Therapeutic Target in NF2-Deficient Meningioma. Neuro Oncol (2018) 20(9):1185–96. doi: 10.1093/neuonc/noy046
48. Frattini V, Trifonov V, Chan JM, Castano A, Lia M, Abate F, et al. The Integrated Landscape of Driver Genomic Alterations in Glioblastoma. Nat Genet (2013) 45(10):1141–9. doi: 10.1038/ng.2734
49. Steklov M, Pandolfi S, Baietti MF, Batiuk A, Carai P, Najm P, et al. Mutations in LZTR1 Drive Human Disease by Dysregulating RAS Ubiquitination. Science (2018) 362(6419):1177–82. doi: 10.1126/science.aap7607
50. Hodges C, Kirkland JG, Crabtree GR. The Many Roles of BAF (mSWI/SNF) and PBAF Complexes in Cancer. Cold Spring Harb Perspect Med (2016) 6(8):a026930. doi: 10.1101/cshperspect.a026930
51. Mittal P, Roberts CWM. The SWI/SNF Complex in Cancer - Biology, Biomarkers and Therapy. Nat Rev Clin Oncol (2020) 17(7):435–48. doi: 10.1038/s41571-020-0357-3
52. Brosseau J-P, Liao C-P, Le LQ. Translating Current Basic Research Into Future Therapies for Neurofibromatosis Type 1. Br J Cancer. (2020) 123(2):178–86. doi: 10.1038/s41416-020-0903-x
53. Widemann BC, Dombi E, Gillespie A, Wolters PL, Belasco J, Goldman S, et al. Phase 2 Randomized, Flexible Crossover, Double-Blinded, Placebo-Controlled Trial of the Farnesyltransferase Inhibitor Tipifarnib in Children and Young Adults With Neurofibromatosis Type 1 and Progressive Plexiform Neurofibromas. Neuro Oncol (2014) 16(5):707–18. doi: 10.1093/neuonc/nou004
54. Plotkin SR, Ardern-Holmes SL, Barker FG, Blakeley JO, Evans DG, Ferner RE, et al. Hearing and Facial Function Outcomes for Neurofibromatosis 2 Clinical Trials. Neurology (2013) 81(21 Suppl 1):S25–32. doi: 10.1212/01.wnl.0000435746.02780.f6
55. Wolters PL, Martin S, Merker VL, Tomsgard JH, Solomon SE, Baldwin A, et al. Patient-Reported Outcomes of Pain and Physical Functioning in Neurofibromatosis Clinical Trials. Neurology (2016) 87(7 Suppl 1):S4–12. doi: 10.1212/WNL.0000000000002927
56. Ferrer M, Gosline SJC, Stathis M, Zhang X, Guo X, Guha R, et al. Pharmacological and Genomic Profiling of Neurofibromatosis Type 1 Plexiform Neurofibroma-Derived Schwann Cells. Sci Data (2018) 5(1):180106. doi: 10.1038/sdata.2018.106
57. Synodos for NF2 Consortium, Allaway R, Angus SP, Beauchamp RL, Blakeley JO, Bott M, et al. Traditional and Systems Biology Based Drug Discovery for the Rare Tumor Syndrome Neurofibromatosis Type 2. PloS One (2018) 13(6):e0197350. doi: 10.1371/journal.pone.0197350
58. Terribas E, Fernández M, Mazuelas H, Fernández-Rodríguez J, Biayna J, Blanco I, et al. KIF11 and KIF15 Mitotic Kinesins Are Potential Therapeutic Vulnerabilities for Malignant Peripheral Nerve Sheath Tumors. Neurooncol Adv (2020) 2(Suppl 1):i62–74. doi: 10.1093/noajnl/vdz061
59. Chang L-S, Oblinger JL, Smith AE, Ferrer M, Angus SP, Hawley E, et al. Brigatinib Causes Tumor Shrinkage in Both NF2-Deficient Meningioma and Schwannoma Through Inhibition of Multiple Tyrosine Kinases But Not ALK. PloS One (2021) 16(7):e0252048. doi: 10.1371/journal.pone.0252048
60. Peng Y, Zheng J, Zhou Y, Li J. Characterization of a Novel Curcumin Analog P1 as Potent Inhibitor of the NF-κb Signaling Pathway With Distinct Mechanisms. Acta Pharmacol Sin (2013) 34(7):939–50. doi: 10.1038/aps.2013.2
61. Siddiqui FA, Prakasam G, Chattopadhyay S, Rehman AU, Padder RA, Ansari MA, et al. Curcumin Decreases Warburg Effect in Cancer Cells by Down-Regulating Pyruvate Kinase M2 via mTOR-Hif1α Inhibition. Sci Rep (2018) 8(1):1–9. doi: 10.1038/s41598-018-25524-3
62. Angelo LS, Wu JY, Meng F, Sun M, Kopetz S, McCutcheon IA, et al. Combining Curcumin (Diferuloylmethane) and Heat Shock Protein Inhibition for Neurofibromatosis 2 Treatment: Analysis of Response and Resistance Pathways. Mol Cancer Ther (2011) 10(11):2094–103. doi: 10.1158/1535-7163.MCT-11-0243
63. Oblinger JL, Burns SS, Akhmametyeva EM, Huang J, Pan L, Ren Y, et al. Components of the Eif4f Complex Are Potential Therapeutic Targets for Malignant Peripheral Nerve Sheath Tumors and Vestibular Schwannomas. Neuro Oncol (2016) 18(9):1265–77. doi: 10.1093/neuonc/now032
64. Lee M-J, Tsai Y-J, Lin M-Y, You H-L, Kalyanam N, Ho C-T, et al. Calebin-A Induced Death of Malignant Peripheral Nerve Sheath Tumor Cells by Activation of Histone Acetyltransferase. Phytomedicine (2019) 57:377–84. doi: 10.1016/j.phymed.2019.01.001
65. Esposito T, Schettino C, Polverino P, Allocca S, Adelfi L, D-Amico A, et al. Synergistic Interplay Between Curcumin and Polyphenol-Rich Foods in the Mediterranean Diet: Therapeutic Prospects for Neurofibromatosis 1 Patients. Nutrients (2017) 9(7):783. doi: 10.3390/nu9070783
66. Nelson KM, Dahlin JL, Bisson J, Graham J, Pauli GF, Walters MA. The Essential Medicinal Chemistry of Curcumin. J Med Chem (2017) 60(5):1620–37. doi: 10.1021/acs.jmedchem.6b00975
67. Xie X, Wang H, Williams GR, Yang Y, Zheng Y, Wu J, et al. Erythrocyte Membrane Cloaked Curcumin-Loaded Nanoparticles for Enhanced Chemotherapy. Pharmaceutics (2019) 11(9):429. doi: 10.3390/pharmaceutics11090429
68. Tran TD, Ogbourne SM, Brooks PR, Sánchez-Cruz N, Medina-Franco JL, Quinn RJ. Lessons From Exploring Chemical Space and Chemical Diversity of Propolis Components. Int J Mol Sci (2020) 21(14):4988. doi: 10.3390/ijms21144988
69. Demestre M, Messerli SM, Celli N, Shahhossini M, Kluwe L, Mautner V, et al. CAPE (Caffeic Acid Phenethyl Ester)-Based Propolis Extract (Bio 30) Suppresses the Growth of Human Neurofibromatosis (NF) Tumor Xenografts in Mice. Phytother Res (2009) 23(2):226–30. doi: 10.1002/ptr.2594
70. Ong CP, Lee WL, Tang YQ, Yap WH. Honokiol: A Review of Its Anticancer Potential and Mechanisms. Cancers (2020) 12(1):48. doi: 10.3390/cancers12010048
71. Lee JD, Lee JY, Baek BJ, Lee BD, Koh YW, Lee W-S, et al. The Inhibitory Effect of Honokiol, A Natural Plant Product, on Vestibular Schwannoma Cells. Laryngoscope (2012) 122(1):162–6. doi: 10.1002/lary.21781
72. Berretta M, Bignucolo A, Di Francia R, Cornello F, Facchini G, Ceccarelli M, et al. Resveratrol in Cancer Patients: From Bench to Bedside. Int J Mol Sci (2020) 21(8):2945. doi: 10.3390/ijms21082945
73. Cai H, Scott E, Kholghi A, Andreadi C, Rufini A, Karmokar A, et al. Cancer Chemoprevention: Evidence of a Nonlinear Dose Response for the Protective Effects of Resveratrol in Humans and Mice. Sci Transl Med (2015) 7(298):298ra117–298ra117. doi: 10.1126/scitranslmed.aaa7619
74. Brown VA, Patel KR, Viskaduraki M, Crowell JA, Perloff M, Booth TD, et al. Repeat Dose Study of the Cancer Chemopreventive Agent Resveratrol in Healthy Volunteers: Safety, Pharmacokinetics, and Effect on the Insulin-Like Growth Factor Axis. Cancer Res (2010) 70(22):9003–11. doi: 10.1158/0008-5472.CAN-10-2364
75. Hu S, Wei W, Yuan J, Cheng J. Resveratrol Inhibits Proliferation in HBL-52 Meningioma Cells. Onco Targets Ther (2019) 12:11579–86. doi: 10.2147/OTT.S228513
76. Hashemzaei M, Delarami Far A, Yari A, Heravi RE, Tabrizian K, Taghdisi SM, et al. Anticancer and Apoptosis−Inducing Effects of Quercetin In Vitro and In Vivo. Oncol Rep (2017) 38(2):819–28. doi: 10.3892/or.2017.5766
77. Yang C, Asthagiri AR, Iyer RR, Lu J, Xu DS, Ksendzovsky A, et al. Missense Mutations in the NF2 Gene Result in the Quantitative Loss of Merlin Protein and Minimally Affect Protein Intrinsic Function. Proc Natl Acad Sci USA (2011) 108(12):4980–5. doi: 10.1073/pnas.1102198108
78. McGovern SL, Shoichet BK. Kinase Inhibitors: Not Just for Kinases Anymore. J Med Chem (2003) 46(8):1478–83. doi: 10.1021/jm020427b
79. Guo Y, Mah E, Bruno RS. Quercetin Bioavailability Is Associated With Inadequate Plasma Vitamin C Status and Greater Plasma Endotoxin in Adults. Nutrition (2014) 30(11):1279–86. doi: 10.1016/j.nut.2014.03.032
80. Chen R, Lin J, Hong J, Han D, Zhang AD, Lan R, et al. Potential Toxicity of Quercetin: The Repression of Mitochondrial Copy Number via Decreased POLG Expression and Excessive TFAM Expression in Irradiated Murine Bone Marrow. Toxicol Rep (2014) 1:450–8. doi: 10.1016/j.toxrep.2014.07.014
81. Resende FA, Vilegas W, Dos Santos LC, Varanda EA. Mutagenicity of Flavonoids Assayed by Bacterial Reverse Mutation (Ames) Test. Molecules (2012) 17(5):5255–68. doi: 10.3390/molecules17055255
82. Hirokawa Y, Nheu T, Grimm K, Mautner V, Maeda S, Yoshida M, et al. Sichuan Pepper Extracts Block the PAK1/Cyclin D1 Pathway and the Growth of NF1-Deficient Cancer Xenograft in Mice. Cancer Biol Ther (2006) 5(3):305–9. doi: 10.4161/cbt.5.3.2404
83. Jing S, Zou H, Wu Z, Ren L, Zhang T, Zhang J, et al. Cucurbitacins: Bioactivities and Synergistic Effect With Small-Molecule Drugs. J Func Foods. (2020) 72:104042. doi: 10.1016/j.jff.2020.104042
84. Lee DH, Iwanski GB, Thoennissen NH. Cucurbitacin: Ancient Compound Shedding New Light on Cancer Treatment. Sci World J (2010) 10:413–8. doi: 10.1100/tsw.2010.44
85. Banerjee S, Byrd JN, Gianino SM, Harpstrite SM, Rodriguez FJ, Tuskan RG, et al. The Neurofibromatosis Type 1 Tumor Suppressor Controls Cell Growth by Regulating Signal Transducer and Activator of Transcription-3 Activity In Vitro and In Vivo. Cancer Res (2010) 70(4):1356–66. doi: 10.1158/0008-5472.CAN-09-2178
86. Spear SA, Burns SS, Oblinger JL, Ren Y, Pan L, Kinghorn AD, et al. Natural Compounds as Potential Treatments of NF2-Deficient Schwannoma and Meningioma: Cucurbitacin D and Goyazensolide. Otol Neurotol (2013) 34(8):1519–27. doi: 10.1097/MAO.0b013e3182956169
87. Hou W, Liu B, Xu H. Celastrol: Progresses in Structure-Modifications, Structure-Activity Relationships, Pharmacology and Toxicology. Eur J Med Chem (2020) 189:112081. doi: 10.1016/j.ejmech.2020.112081
88. Du X, Nyagblordzro M, An L, Guo X, Du L, Wang Y, et al. Pharmacokinetic and Toxicological Characteristics of Tripterigium Glycosides and Their Derivatives. Curr Drug Metab (2018) 19(7):605–27. doi: 10.2174/1389200219666180302152752
89. Muñoz-Acuña U, Shen Q, Ren Y, Lantvit DD, Wittwer JA, Kinghorn AD, et al. Goyazensolide Induces Apoptosis in Cancer Cells In Vitro and In Vivo. Int J Cancer Res (2013) 9(2):36–53. doi: 10.3923/ijcr.2013.36.53
90. Li X-X, Zhang S-J, Chiu AP, Lo LH, Huang J, Rowlands DK, et al. Targeting of AKT/ERK/CTNNB1 by DAW22 as a Potential Therapeutic Compound for Malignant Peripheral Nerve Sheath Tumor. Cancer Med (2018) 7(9):4791–800. doi: 10.1002/cam4.1732
91. Kamal MM, Akter S, Lin C-N, Nazzal S. Sulforaphane as an Anticancer Molecule: Mechanisms of Action, Synergistic Effects, Enhancement of Drug Safety, and Delivery Systems. Arch Pharm Res (2020) 43(4):371–84. doi: 10.1007/s12272-020-01225-2
92. Kim BG, Fujita T, Stankovic KM, Welling DB, Moon IS, Choi JY, et al. Sulforaphane, a Natural Component of Broccoli, Inhibits Vestibular Schwannoma Growth In Vitro and In Vivo. Sci Rep (2016) 6:1–10. doi: 10.1038/srep36215
93. Mangla B, Javed S, Sultan MH, Kumar P, Kohli K, Najmi A, et al. Sulforaphane: A Review of Its Therapeutic Potentials, Advances in Its Nanodelivery, Recent Patents, and Clinical Trials. Phytother Res (2021) 1–19. doi: 10.1002/ptr.7176
94. Langston-Cox A, Anderson D, Creek DJ, Palmer K, Wallace EM, Marshall SA. Measuring Sulforaphane and Its Metabolites in Human Plasma: A High Throughput Method. Molecules (2020) 25(4):829. doi: 10.3390/molecules25040829
95. Montero-Oleas N, Arevalo-Rodriguez I, Nuñez-González S, Viteri-García A, Simancas-Racines D. Therapeutic Use of Cannabis and Cannabinoids: An Evidence Mapping and Appraisal of Systematic Reviews. BMC Complement Med Ther (2020) 20(1):12. doi: 10.1186/s12906-019-2803-2
96. Urits I, Gress K, Charipova K, Habib K, Lee D, Lee C, et al. Use of Cannabidiol (CBD) for the Treatment of Chronic Pain. Best Pract Res Clin Anaesthesiol. (2020) 34(3):463–77. doi: 10.1016/j.bpa.2020.06.004
97. Kovalchuk O, Kovalchuk I. Cannabinoids as Anticancer Therapeutic Agents. Cell Cycle (2020) 19(9):961–89. doi: 10.1080/15384101.2020.1742952
98. Hegazy O, Platnick H. Cannabidiol (CBD) for Treatment of Neurofibromatosis-Related Pain and Concomitant Mood Disorder: A Case Report. Cureus (2019) 11(12):e6312. doi: 10.7759/cureus.6312
99. Millar SA, Stone NL, Yates AS, O’Sullivan SE. A Systematic Review on the Pharmacokinetics of Cannabidiol in Humans. Front Pharmacol (2018) 9:1365. doi: 10.3389/fphar.2018.01365
100. González Canga A, Sahagún Prieto AM, Diez Liébana MJ, Fernández Martínez N, Sierra Vega M, García Vieitez JJ. The Pharmacokinetics and Interactions of Ivermectin in Humans—A Mini-Review. AAPS J (2008) 10(1):42–6. doi: 10.1208/s12248-007-9000-9
101. Dowling P. Pharmacogenetics: It’s Not Just About Ivermectin in Collies. Can Vet J (2006) 47(12):1165–8.
102. Hashimoto H, Messerli SM, Sudo T, Maruta H. Ivermectin Inactivates the Kinase PAK1 and Blocks the PAK1-Dependent Growth of Human Ovarian Cancer and NF2 Tumor Cell Lines. Drug Discov Ther (2009) 3(6):243–6.
103. Kinghorn AD, Blanco EJC DE, Lucas DM, Rakotondraibe HL, Orjala J, Soejarto DD, et al. Discovery of Anticancer Agents of Diverse Natural Origin. Anticancer Res (2016) 36(11):5623–37. doi: 10.21873/anticanres.11146
104. Pan L, Woodard JL, Lucas DM, Fuchs JR, Kinghorn AD. Rocaglamide, Silvestrol and Structurally Related Bioactive Compounds From Aglaia Species. Nat Prod Rep (2014) 31(7):924–39. doi: 10.1039/C4NP00006D
105. Hwang BY, Su B-N, Chai H, Mi Q, Kardono LBS, Afriastini JJ, et al. Silvestrol and Episilvestrol, Potential Anticancer Rocaglate Derivatives From Aglaia Silvestris. J Org Chem (2004) 69(10):3350–8. doi: 10.1021/jo040120f
106. Sadlish H, Galicia-Vazquez G, Paris CG, Aust T, Bhullar B, Chang L, et al. Evidence for a Functionally Relevant Rocaglamide Binding Site on the Eif4a-RNA Complex. ACS Chem Biol (2013) 8(7):1519–27. doi: 10.1021/cb400158t
107. Wolfe AL, Singh K, Zhong Y, Drewe P, Rajasekhar VK, Sanghvi VR, et al. RNA G-Quadruplexes Cause Eif4a-Dependent Oncogene Translation in Cancer. Nature (2014) 513(7516):65–70. doi: 10.1038/nature13485
108. Thuaud F, Ribeiro N, Nebigil CG, Désaubry L. Prohibitin Ligands in Cell Death and Survival: Mode of Action and Therapeutic Potential. Chem Biol (2013) 20(3):316–31. doi: 10.1016/j.chembiol.2013.02.006
109. Oblinger JL, Burns SS, Huang J, Pan L, Ren Y, Shen R, et al. Overexpression of Eif4f Components in Meningiomas and Suppression of Meningioma Cell Growth by Inhibiting Translation Initiation. Exp Neurol (2018) 299(Pt B):299–307. doi: 10.1016/j.expneurol.2017.06.015
110. Pan L, Acuña UM, Li J, Jena N, Ninh TN, Pannell CM, et al. Bioactive Flavaglines and Other Constituents Isolated From Aglaia Perviridis. J Nat Prod (2013) 76(3):394–404. doi: 10.1021/np3007588
111. King ML, Chiang C-C, Ling H-C, Fujita E, Ochiai M, McPhail AT. X-Ray Crystal Structure of Rocaglamide, a Novel Antileukemic 1h-Cyclopenta[B]Benzofuran From Aglaia Elliptifolia. J Chem Soc Chem Commun (1982) 20):1150–1. doi: 10.1039/c39820001150
112. Iwasaki S, Iwasaki W, Takahashi M, Sakamoto A, Watanabe C, Shichino Y, et al. The Translation Inhibitor Rocaglamide Targets a Bimolecular Cavity Between Eif4a and Polypurine RNA. Mol Cell (2019) 73(4):738–48.e9. doi: 10.1016/j.molcel.2018.11.026
113. Chang L-S, Oblinger JL, Burns SS, Huang J, Anderson LW, Hollingshead MG, et al. Targeting Protein Translation by Rocaglamide and Didesmethylrocaglamide to Treat MPNST and Other Sarcomas. Mol Cancer Ther (2020) 19(3):731–41. doi: 10.1158/1535-7163.MCT-19-0809
114. Ecosystems Research and Development Bureau. Guyabano (Annona Muricata Linnaeus). Department Environ Natural Resources Res Inf Ser Ecosystems (2014) 26(3):1–13. Available at: http://erdb.denr.gov.ph/files/publications/rise/r_v26n3.pdf.
115. Moghadamtousi SZ, Fadaeinasab M, Nikzad S, Mohan G, Ali HM, Kadir HA. Annona Muricata (Annonaceae): A Review of Its Traditional Uses, Isolated Acetogenins and Biological Activities. Int J Mol Sci (2015) 16(7):15625–58. doi: 10.3390/ijms160715625
116. GoodByeNf. Neurofibromatosis Network, Inspire. In: Good Bye NF - Neurofibromatosis (2014). Available at: https://www.inspire.com/groups/neurofibromatosis-network/discussion/good-bye-nf/ (Accessed 2/12/2021).
117. Lannuzel A, Höglinger GU, Champy P, Michel PP, Hirsch EC, Ruberg M. Is Atypical Parkinsonism in the Caribbean Caused by the Consumption of Annonacae? J Neural Transm Suppl (2006) 70):153–7. doi: 10.1007/978-3-211-45295-0_24
118. Cancer Research UK. Graviola (Soursop) (2018). Available at: https://www.cancerresearchuk.org/about-cancer/cancer-in-general/treatment/complementary-alternative-therapies/individual-therapies/graviola (Accessed 2/12/2021).
119. Newman DJ, Cragg GM. Natural Products as Sources of New Drugs Over the Nearly Four Decades From 01/1981 to 09/2019. J Nat Prod (2020) 83(3):770–803. doi: 10.1021/acs.jnatprod.9b01285
120. Shipkowski KA, Betz JM, Birnbaum LS, Bucher JR, Coates PM, Hopp DC, et al. Naturally Complex: Perspectives and Challenges Associated With Botanical Dietary Supplement Safety Assessment. Food Chem Toxicol (2018) 118:963–71. doi: 10.1016/j.fct.2018.04.007
121. Perrone L, Sampaolo S, Melone MAB. Bioactive Phenolic Compounds in the Modulation of Central and Peripheral Nervous System Cancers: Facts and Misdeeds. Cancers (2020) 12(2):454. doi: 10.3390/cancers12020454
122. Pichersky E, Raguso RA. Why do Plants Produce So Many Terpenoid Compounds? New Phytol (2018) 220(3):692–702. doi: 10.1111/nph.14178
123. Sarfaraz S, Afaq F, Adhami VM, Malik A, Mukhtar H. Cannabinoid Receptor Agonist-Induced Apoptosis of Human Prostate Cancer Cells LNCaP Proceeds Through Sustained Activation of ERK1/2 Leading to G1 Cell Cycle Arrest. J Biol Chem (2006) 281(51):39480–91. doi: 10.1074/jbc.M603495200
124. Hart S, Fischer OM, Ullrich A. Cannabinoids Induce Cancer Cell Proliferation via Tumor Necrosis Factor α-Converting Enzyme (TACE/ADAM17)-Mediated Transactivation of the Epidermal Growth Factor Receptor. Cancer Res (2004) 64(6):1943–50. doi: 10.1158/0008-5472.CAN-03-3720
125. Chan K, Robert F, Oertlin C, Kapeller-Libermann D, Avizonis D, Gutierrez J, et al. Eif4a Supports an Oncogenic Translation Program in Pancreatic Ductal Adenocarcinoma. Nat Commun (2019) 10(1):5151. doi: 10.1038/s41467-019-13086-5
126. Ernst JT, Thompson PA, Nilewski C, Sprengeler PA, Sperry S, Packard G, et al. Design of Development Candidate Eft226, A First in Class Inhibitor of Eukaryotic Initiation Factor 4a RNA Helicase. J Med Chem (2020) 63(11):5879–955. doi: 10.1021/acs.jmedchem.0c00182
127. Chen P-N, Hsieh Y-S, Chiou H-L, Chu S-C. Silibinin Inhibits Cell Invasion Through Inactivation of Both PI3K-Akt and MAPK Signaling Pathways. Chem Biol Interact (2005) 156(2-3):141–50. doi: 10.1016/j.cbi.2005.08.005
128. Martin ACBM, Fuzer AM, Becceneri AB, da Silva JA, Tomasin R, Denoyer D, et al. [10]-Gingerol Induces Apoptosis and Inhibits Metastatic Dissemination of Triple Negative Breast Cancer In Vivo. Oncotarget (2017) 8(42):72260–71. doi: 10.18632/oncotarget.20139
129. Zhao Q, Assimopoulou AN, Klauck SM, Damianakos H, Chinou I, Kretschmer N, et al. Inhibition of C-MYC With Involvement of ERK/JNK/MAPK and AKT Pathways as a Novel Mechanism for Shikonin and Its Derivatives in Killing Leukemia Cells. Oncotarget (2015) 6(36):38934–51. doi: 10.18632/oncotarget.5380
130. Liao K-F, Chiu T-L, Huang S-Y, Hsieh T-F, Chang S-F, Ruan S-P, et al. Anti-Cancer Effects of Radix Angelica Sinensis (Danggui) and N-Butylidenephthalide on Gastric Cancer: Implications for REDD1 Activation and mTOR Inhibition. CPB (2018) 48(6):2231–46. doi: 10.1159/000492641
131. Spagnuolo C, Russo GL, Orhan IE, Habtemariam S, Daglia M, Sureda A, et al. Genistein and Cancer: Current Status, Challenges, and Future Directions. Adv Nutr (2015) 6(4):408–19. doi: 10.3945/an.114.008052
Keywords: neurofibromatosis (NF), signaling pathway, targeted therapy, natural compounds, eIF4A inhibitors, rocaglamide, didesmethylrocaglamide, protein translation
Citation: Amaravathi A, Oblinger JL, Welling DB, Kinghorn AD and Chang L-S (2021) Neurofibromatosis: Molecular Pathogenesis and Natural Compounds as Potential Treatments. Front. Oncol. 11:698192. doi: 10.3389/fonc.2021.698192
Received: 20 April 2021; Accepted: 01 September 2021;
Published: 17 September 2021.
Edited by:
Fabrizio Martelli, National Institute of Health (ISS), ItalyReviewed by:
Suresh Kumar Kalangi, Amity University Gurgaon, IndiaChristine Pratilas, Johns Hopkins University, United States
Copyright © 2021 Amaravathi, Oblinger, Welling, Kinghorn and Chang. This is an open-access article distributed under the terms of the Creative Commons Attribution License (CC BY). The use, distribution or reproduction in other forums is permitted, provided the original author(s) and the copyright owner(s) are credited and that the original publication in this journal is cited, in accordance with accepted academic practice. No use, distribution or reproduction is permitted which does not comply with these terms.
*Correspondence: Long-Sheng Chang, Long-Sheng.Chang@nationwidechildrens.org
†These authors have contributed equally to this work