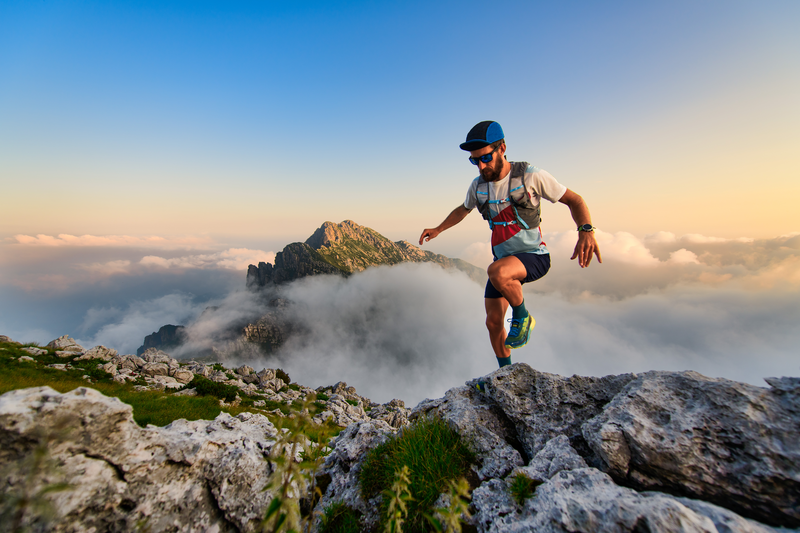
94% of researchers rate our articles as excellent or good
Learn more about the work of our research integrity team to safeguard the quality of each article we publish.
Find out more
SYSTEMATIC REVIEW article
Front. Oncol. , 29 April 2021
Sec. Thoracic Oncology
Volume 11 - 2021 | https://doi.org/10.3389/fonc.2021.680191
Background: The association between driver genes and the incidence of thromboembolic events (TEs) in patients diagnosed with non-small-cell lung cancer (NSCLC) needs to be quantified to guide clinical management.
Methods: We interrogated PubMed, Embase, Web of Science and Cochrane library databases for terms related to venous thromboembolism (VTE) and arterial thromboembolism (ATE) in patients diagnosed with non-small-cell lung cancer harboring driver genes. This search was conducted for studies published between 1 January, 2000 and 31 December, 2020. A random-effects meta-analysis was performed to analyze the pooled incidence and odds ratios of VTE in patients with different driver genes.
Results: Of the 2,742 citations identified, a total of 25 studies that included 21,156 patients met eligibility criteria. The overall pooled incidence of VTE in patients with driver genes was 23% (95% CI 18-29). Patients with ROS1 rearrangements had the highest incidence of VTE (37%, 95%CI 23-52). ALK rearrangements were associated with increased VTE risks (OR=2.08,95% CI 1.69-2.55), with the second highest incidence of VTE (27%, 95%CI 20-35). Both groups of patients with EGFR and KRAS mutations did not show a significantly increased risk for VTE (OR=1.33, 95% CI 0.75-2.34; OR=1.31, 95% CI 0.40-4.28).
Conclusions: ALK rearrangements were shown to be associated with increased VTE risks in patients diagnosed with non-small lung cancer, while there was no significant relation observed between VTE risks and EGFR or KRAS mutations in lung cancer patients.
Venous thromboembolisms (VTEs), which consist of deep vein thrombosis (DVT) and pulmonary embolisms (PEs), are a common complication associated with cancer, occurring in 5-10% of cancer patients. VTEs are also a major cause of morbidity and mortality (1, 2), increasing risks of death 3-5 times (3). Compared with other malignancies, lung cancer has been associated with an intermediate risk of VTE, especially during the first year following cancer diagnosis. The incidence of VTE in lung cancer patients is approximately 7-13% (1, 4). With prolonged survival, the aging of the cancer population and the introduction of thrombogenic anti-cancer treatments increase the incidence of VTE in cancer patients (2). In addition, patients diagnosed with lung cancer are at an increased risk for arterial thromboembolism (ATE), but the impact on the generation of ATE is less severe than that on the generation of VTE (5).
Molecular subtypes are highly relevant to the outcomes of patients with advanced or metastatic NSCLC, as targeted therapy has become a standard clinical management of patients with specific activating mutations in recent decades, and it can significantly improve the survival of patients. Guidelines recommend that all patients with advanced or metastatic NSCLC should be tested for targetable driver genes as a means to guide treatments (6, 7). Since different driver genes show heterogeneity in tumor biological behavior, treatment response and prognosis, driver genes may also manifest heterogeneity during thrombus generation. Many articles have suggested that ROS1 and ALK rearrangements cause a greater risk for VTE (8–10), but there are still some controversies for patients with EGFR, KRAS and other gene mutations (8, 11–15). Moreover, there is currently a lack of quantitative analysis determining the extent to which different driver genes affect VTE occurrence, as the incidence has been shown to vary between studies.
Since the primary prevention of VTE in cancer patients is not a validated management strategy, VTE risk assessment tools are necessary for efficient prevention (16). Although there are many clinical scoring systems incorporating known tumor-related VTE risk factors into the scoring system, these tools show poor performance in lung cancer patients (17, 18). Recently, two large random clinical trials investigating direct oral anticoagulants used the Khorana risk scoring system to screen patients in high risks of developing VTE. Results showed that every three VTE cases was prevented at the cost of a major bleeding event caused by thromboprophylaxis (19, 20). Therefore, a VTE risk assessment tool applicable for lung cancer patients is urgently needed. Incorporating positive driver genes into a risk assessment model may improve performance, and thus clarification of the correlation between driver genes and VTE risk is of great significance for VTE risk assessments. Consequently, we generated this systematic review and meta-analysis to evaluate the estimated incidence and risk of VTE in NSCLC patients with different driver genes.
According to the Preferred Reporting Items for Systematic Reviews and Meta-Analyses (PRISMA) statements, we identified relevant studies for meta-analysis and systematic review (Supplementary Table 1) (21). We performed a search of PubMed, Embase, Web of Science and Cochrane library databases for relevant articles written in English and published between 1 January, 2000 and 31 December, 2020. We used three groups of search terms including: (1) ‘lung cancer’, ‘lung neoplasms’ and ‘non-small-cell lung cancer’; (2) ‘EGFR’, ‘KRAS’, ‘ALK’, ‘ROS1’, ‘RET’, ‘MET’, ‘BRAF’, ‘MEK’, ‘PIK3CA’, ‘PTEN’, ‘FGFR1’, ‘HER2’ and ‘DDR2’; (3) ‘venous thromboembolism’, ‘deep vein thrombosis’, ‘pulmonary embolism’, ‘arterial thromboembolism’, ‘cerebral vascular accident’, ‘stroke’ and ‘myocardial infarction’ (specific search strategies are listed in Supplementary File 1). References of the included studies, published meta-analyses and systematic reviews were also assessed for further potential studies that could be included.
We included all full-text studies and abstracts with information on the incidence of VTE or ATE in lung cancer patients with confirmed driver genes. These studies included hospital-, population- and registry-based cohorts and case-control studies. The inclusion criteria were defined as follows: (1) patients diagnosed with pathologically confirmed NSCLC, (2) a confirmed gene status and (3) available data on VTEs or ATEs. Follow-up duration and treatment regimens were not restricted in any form. The exclusion criteria were defined as follows: (1) clinical trials and other studies on treatment safety (i.e., showing VTE or ATE as adverse events), were excluded due to their miscellaneous follow-up period which is usually not related to the time of cancer diagnosis, (2) case reports, case series, reviews, in vitro studies and animal studies. Three investigators independently assessed all trials for eligibility, and disagreements were resolved through consensus.
Two independent investigators reviewed titles and abstracts of potentially relevant studies and independently extracted study details using a standardized pilot-tested form. A third investigator reviewed all data entries. The following information from each eligible study was extracted, including: first author, study location, study design, sample size, pathological type, gene status, treatment regimens and follow-up duration. We extracted data on the incidence of VTE and ATE in NSCLC patients. Possible related factors for the development of VTE in NSCLC patients were also extracted when available, including age, sex, ethnicity, pathological type, cancer stage, smoking status, comorbidities, gene subtypes and treatment regimens.
The methodological quality of studies was assessed by two authors using the Newcastle-Ottawa quality assessment scale (22), which assigns 4 points for selection, 2 points for comparability and 3 points for outcomes.
The primary outcome was the occurrence of VTE, including events during the peri-diagnosis and treatment periods. VTE consists of DVT (symptomatic or asymptomatic) and PE. Secondary outcome was the incidence of ATE. ATE was defined as arterial thromboembolism, including but not limited to cerebral vascular accident and acute myocardial infarction.
Statistical analyses for overall risks of VTEs/ATEs were performed using Stata 14.0. Study heterogeneity was estimated using the χ2-based Q statistic and heterogeneity was considered statistically significant when I2 > 50%. Meta-analysis was performed using the Mantel-Haenszel random-effects model for estimates of the odds ratio (OR) and the inverse variance random-effects model for rate estimates. The association of potential risk factors with VTEs/ATEs was summarized as OR (95% confidence interval [CI]). Given that differences in follow-up periods, the inclusion of asymptomatic VTE, VTE composition, stage, pathological type, race, quality and publish year would affect the result, we performed subgroup analysis based on these factors. Publication bias was assessed through graphical visualization of funnel plots as well as using Begg’s and Egger’s tests. Sensitivity analysis of the primary outcome was conducted by sequential removal of each involved trial. A two-sided P value less than 0.05 was considered to be statistically significant.
We identified 2,742 reports and included 25 studies performed between January 1, 2000 and December 31, 2020 in the analysis. The process of identifying eligible studies for our systematic review and meta-analysis is shown in Figure 1. Characteristics of included studies are available in Table 1. 25 studies included 3 prospective cohorts, 20 retrospective cohorts and 2 retrospective case-control studies. Of the 25 studies, 22 studies reported VTEs, and 8 studies reported ATEs. 4 studies reported PEs or DVT events independently. The size of the studies varied between 26 and 4,752 participants. All included studies contained patients diagnosed with NSCLC or lung adenocarcinoma harboring driver genes including EGFR, ALK, ROS1, KRAS, MET and BRAF. All included studies were of good quality, as assessed using the Newcastle-Ottawa quality assessment scale for cohort and case-control studies (Supplementary Table 2).
The study included 21,156 patients of which 4,342 patients were confirmed to harbor driver gene mutations (2,080 EGFR mutated, 1,575 ALK rearranged, 340 KRAS mutated, 290 ROS1 rearranged, 31 BRAF mutated and 26 MET amplificated or exon 14 mutated; Clinical characteristics of each group with different driver genes were shown in Supplementary Table 3). Among the patients, 876 patients developed thromboembolic events, including 630 VTE in 2,882 patients with driver genes and 105 ATE events in 1,821 patients harboring driver genes. Pooled VTE incidence was 23% (95% CI 18-29). Patients with ROS1 rearrangements had the highest VTE incidence of 37% (95% CI 23-52), followed by ALK rearranged patients with an incidence of 27% (95%CI 20-35). VTE incidence in patients with EGFR mutations and KRAS mutations was 15% (95% CI 9-20) and 9% (95% CI 5-14), respectively (Figure 2). Furthermore, 10 studies were analyzed with negative controls as shown in Figure 3. Patients with ALK rearrangements showed significantly higher risks for VTE (OR=2.08,95% CI 1.69-2.55, P=0.000, I2 = 0.0%), while patients harboring EGFR (OR=1.33, 95% CI 0.75-2.34, P=0.328, I2 = 73.0%) and KRAS (OR=1.31, 95% CI 0.40-4.28, P=0.652, I2 = 82.0%) mutations showed no significant relation with the risk of VTE.
Subgroup analysis of VTE incidence in ALK rearranged or EGFR mutant patients (Figures 4, 5) was performed. Great consistency was shown in patients with ALK rearrangement. As for the VTE incidence in EGFR mutant patients, publish year and insufficient data may explain the heterogeneity of results. There were 5 included studies published before 2018, and 3 of them were conference abstracts with limited information. Other factors including the setting of the follow-up period, the inclusion of asymptomatic VTE, the composition of VTE, histology, stage, race and quality of studies were not significantly associated with the outcomes.
Figure 4 Subgroup analysis of VTE incidence in ALK rearranged patients. *Studies containing VTEs that occurred prior to diagnosis were also included; † Besides PE and DVT, venous thrombosis occurred at other sites were also included, including vena cava, neck vein, portal vein and other sites. No catheter-related events was included.
Figure 5 Subgroup analysis of VTE incidence in EGFR mutant patients. *Studies containing VTEs that occurred prior to diagnosis were also included.
Results of PEs in patients carrying driver genes showed similar trends. As shown in Figure 6A, Patients with ROS1 rearrangements had the highest PE incidence of 26% (95% CI 15-36), followed by ALK rearranged patients with an incidence of 20% (95%CI 14-26). PE incidence in patients with EGFR mutations was 8% (95% CI 2-14). Furthermore, as shown in Figure 6B, patients with ALK rearrangements showed significantly higher risks for PE (OR=1.71, 95% CI 1.28-2.28, P=0.000, I2 = 0.0%), while patients harboring EGFR mutations showed no association with the risk of PE (OR=1.19, 95% CI 0.60-2.36, P=0.614, I2 = 69.4%). Sensitivity analysis and publication bias are presented in supplementary figures. No significant publication bias was observed in funnel plots as well as the Egger’s and Begg’s test results.
Figure 6 The association of driver genes with PE events. (A) Pooled estimates for incidence of PE in patients with EGFR mutation, ALK rearrangement and ROS1 rearrangement. (B) Forest plot demonstrating the association of driver genes with PE events.
A total of 8 articles recorded ATE incidence in patients with different driver genes, as shown in Figure 7. Patients harboring ROS1 rearrangements showed higher incidence of ATE (7%, 95%CI 2-12), compared with that of ALK rearranged patients (4%, 95%CI 1-6). The results were similar to the ATE incidence observed in general lung cancer populations (41). There were only two studies available for risk estimates of ATE in patients with ALK rearrangement (9, 29), with a total of 5,289 patients (594 ALK rearranged). The results showed that ALK rearrangement did not significantly increase the risk of ATE (OR=0.92, 95% CI 0.44-1.92, P=0.823, I2 = 19.4%).
Even though recent articles indicate that both ROS1 and ALK rearrangements are associated with a higher risk for VTE, the incidence and relative risks reported vary between studies. In addition, there is controversy regarding the correlation between EGFR or KRAS mutations and VTE risks. In this systematic review and meta-analysis, we analyzed VTE incidence as well as the estimated risk for VTE in lung cancer patients with driver genes including ROS1, ALK, EGFR and KRAS. Patients with ROS1 and ALK rearrangements showed the highest incidence for VTE, and the risk of VTE in ALK rearranged patients was double of that observed in ALK wild type patients. Patients with KRAS mutations showed the lowest incidence for VTE among the analyzed driver genes, and both KRAS and EGFR mutations were proved no significant relation with VTE risks in patients with lung cancer. These findings suggest that incorporating ROS1 and ALK rearrangements into VTE risk assessment models may be beneficial for screening NSCLC patients with a high risk for VTE.
Among patients with driver gene mutations, the incidence of pooled VTE was 23% (95% CI 18-29), which was higher than the reported incidence of 7-13% in lung cancer patients (1, 4). This discrepancy may attribute to the difference of population compositions. In the general population, patients with ALK and ROS1 rearrangements are observed in 5% and 2% of NSCLC patients, respectively (42, 43). In the population with driver gene mutations studied in this article, patients with ALK rearrangements accounted for 29.6% while patients with ROS1 rearrangements accounted for 8.4%. Both of these proportions were much greater than what was observed in the general population. Given that patients with ROS1 and ALK rearrangements show longer survival rates compared to patients with other driver genes, several studies performed survival analyses to prove that the increased risk of VTE was not due to prolonged survival (8, 9, 28, 40). Consistent with previous studies, VTE that occurred in the peri-diagnostic period (one month prior to and after cancer diagnosis) accounted for 32%~45% (10, 23) and 23%~35% (9, 38, 40) of all VTEs in the ROS1 or ALK positive cohort, respectively.
There is no doubt that the major purpose of genetic testing is to guide treatment, and NSCLC with different driver genes has different optimal treatment options according to the guidelines (7). As the studies included in this meta-analysis provided limited treatment information (as shown in Supplementary Table 3), and preferred treatments for driven genes has been changing rapidly within years, it becomes quite difficult to diminish the treatment related bias through analysis. However, detailed information from several studies included confirmed the relatively higher risks of TE in NSCLC with ALK/ROS1 rearrangements regardless of the effects caused by treatments. When the timing of TE events was analyzed, 50%~59% of TE in ROS1 rearranged patients happened with naïve treatments (23, 31), while 37% of VTE in patients with ALK rearrangements occurred when patients received no treatments (38). In a study comparing the risk of TE between EGFR mutant and ALK rearranged patients, the incidence of TE before using TKI and the incidence of TE in patients who have never used TKI in patients with ALK rearrangements were significantly higher than those in patients with EGFR mutations (50% vs 17.2%; 90.9% vs 21.2%, respectively). The study also found that using TKI before thrombosis is a protective factor for TEs in ALK rearranged patients [HR=0.084 (95%CI 0.031-0.232)], which was not observed in EGFR mutant patients. This result suggests that the thrombotic predisposition of ALK rearrangement may be related to the gene alteration and derived kinase activity (32). In addition, a recent meta-analysis indicated that TKIs treating NSCLC with ALK/ROS1 rearrangements did not significantly increase the VTE risk compared with platinum-based chemotherapy by analyzing 6 randomized control studies (44). All the evidence supports that ALK/ROS1 rearrangements are associated with increased TE risks. None of the included studies mentioned immunotherapy in treatment modalities. With the advancement of treatments and the development of precise management for target genes in the future, the incidence of TE during clinical management in patients with different driver genes may change accordingly. However, putting aside the influence of treatments on TE events, it is unquestionable that driver genes play an important role in the occurrence of TE events.
The incidence of ATE in patients with lung cancer is approximately 6.3%-10.9% (5, 41). Studies have suggested that the risk of ATE may be the greatest during the peri-diagnosis period, which is similar to results in VTE studies (5, 45, 46). A recent study retrospectively analyzed an ALK positive cohort in NSCLC patients. Although the incidence of ATE was similar in patients with and without ALK rearrangements (5.0% vs 4.4%), ALK-positive patients showed a 3-fold greater risk than wild type patients using time-to-event analysis with relevant variables adjusted (9). Therefore, even though the incidence and risk estimate of ATE in patients with ALK or ROS1 rearrangement in our study were not significantly higher than that in the general lung cancer population, harboring driver genes such as ALK, may increase the risk of ATE generation in a short period after diagnosis with lung cancer. However, current research data for the relation between ATE and driver genes in cancer patients were not strong enough to support the hypothesis. More prospective studies with time-to-event information are expected.
Underlying molecular mechanisms of tumor genomic mutations affecting thrombosis are still unclear. Tissue factor (TF) is an important physiological trigger of coagulation. Upregulation in TF may contribute to the prethrombotic state linked to malignant tumors (47, 48). ALK rearrangements have been shown to be associated with high levels of TF (49). KRAS mutations have also been associated with increased TF expression levels in colorectal cancer and NSCLC (50–52). Moreover, it is known that inflammation plays an important role in thrombosis induction (53). Some studies on ALK-mutated lymphomas have shown that ALK rearrangements lead to increased STAT3 signal transduction, which is involved in downstream signaling of inflammatory cytokines (54). It has also been shown that ALK is important in the activation of NLRP3 inflammasomes in macrophages (55). From a macroscopic perspective, the thrombosis risk assessment model, ONKOTEV study included vascular or lymphatic macroscopic compression as one of the assessment criteria, suggesting that vascular or lymphatic compression may be related to increased VTE risk (56). Studies have shown that higher N stage is associated with an increased VTE risk (57). ALK and ROS1 are common fusion genes in NSCLC, with similar histological findings (58) and a higher tendency to lymph node metastasis (59, 60). In this study, patients with ALK rearrangements showed a higher risk of VTE, while patients with ROS1 rearrangements showed the highest incidence of VTE. Although the mechanism is not clear yet, the tendency of fusion genes to lymph node metastases may be related to increased risk of VTE.
In addition to driver genes, one study included in our study investigated the correlation between programmed cell death ligand 1 (PD-L1) expression and the risk of PE in NSCLC patients. PD-L1 expression is one of the important biomarkers to predict the potential benefit from anti-PD-1/PD-L1 treatment. The study showed that patients with PD-L1 expression in ≥1% tumor cells had a higher risk for PE [OR=1.798 (95%CI 1.137-2.201)], with an incidence of 15.4%, which indicated that PD-L1 expression may be a novel biomarker in prediction of TE in patients with NSCLC (35). Nichetti F et al. also found that high PD-L1 expression (≥50%) was one of the independent predisposing factors of TE during immune checkpoint inhibitor (ICI) treatments in patients with locally advanced or metastatic NSCLC [HR=2.55 (95% CI 1.05-6.19)] (61). However, another study in patients with glioma showed that PD-L1 expression was not related with the risk of VTE (62). At present, both clinical and fundamental researches on the association between PD-1/PD-L1 and TE are limited. With regards to the generation of ATE, it has been shown that the blockade of PD-1 pathway promotes the activation of proatherogenic T cell, thereby aggravating hyperlipidemia and accelerating the formation of atherosclerosis, which suggests that ICI may potentially foster ATEs (63, 64). As for VTEs, it has been reported that activated T cell subsets in vitro express functional TF on their cellular membranes (65). In addition, activated lymphocytes can release a variety of pro-inflammatory cytokines that promote the formation of hypercoagulable states as well (66). It is known that PD-L1 plays an important role in regulating T cell-mediated immune response (67). Therefore, when PD-1/PD-L1 pathway is blocked, the activated immune response may promote the occurrence of TE. However, current studies have indicated that the incidence of TE in patients receiving ICI did not increase significantly, and TE events are not considered as an ICIs drug related toxicity commonly (61, 68). Based on current results, we hypothesize that the risk of TE caused by the activated immune response may be more prominent in patients with high PD-L1 expression. PD-L1, as a potential biomarker for the risk of TE, may cover more pathological types other than lung adenocarcinoma, since driver genes are more common in patients with adenocarcinoma.
Despite these findings, there were also some limitations faced in this study. First, included studies varied in histological type, tumor staging, diagnostic methodology, follow-up duration and treatment. The existence of confounding factors may lead to significant heterogeneity for some gene mutations (I2>50%). Subgroup analysis was performed to analyze potential confounding factors. Second, for some gene mutations, such as ROS1, KRAS, MET and BRAF, there were few published studies, so the results for these mutations may have weaker power. Third, when calculating the estimated risk, the population of wild type was shown to be heterogeneous. For example, patients with EGFR wild type may harbor ROS1 and ALK rearrangements as well as other unknown gene mutations. Therefore, the calculated risk may show some deviation.
In conclusion, driver genes were associated with the risk of VTE in patients with NSCLC. Patients with ROS1 rearrangements showed the highest incidence of VTE with 37% (95%CI 23-52). ALK rearrangements caused approximately twice the risk for VTE, compared with patients with ALK wild type, and patients with ALK arrangements had the second highest incidence of VTE with 27% (95%CI 20-35). Patients with KRAS and EGFR mutations did not show a significant association with VTE risk in patients with NSCLC.
The original contributions presented in the study are included in the article/Supplementary Material. Further inquiries can be directed to the corresponding author.
JyiZ, MF, and XQ conceived of the study. MF obtained funding. The concept and design of this study were generated primarily by XQ. XQ, MF, and JyaZ collected data in the original studies. XQ and JyiZ performed the statistical analyses. XQ and MF interpreted the data and drafted the manuscript. MF and JyaZ revised the manuscript for important intellectual content. All authors contributed to the article and approved the submitted version.
This work was supported by grants received from the Health Commission of Zhejiang Province, China (grant number: 2020392137). The funding agencies played no role in the study design, participant recruitment, data collection, data analysis, data interpretation, manuscript preparation and the decision to submit the paper for publication.
The authors declare that the research was conducted in the absence of any commercial or financial relationships that could be construed as a potential conflict of interest.
The Supplementary Material for this article can be found online at: https://www.frontiersin.org/articles/10.3389/fonc.2021.680191/full#supplementary-material
AC, adenocarcinoma; ATE, arterial thromboembolism; DVT, deep vein thrombosis; HR, hazard ratio; ICI, immune checkpoint inhibitor; NSCLC, non-small-cell lung cancer; OR, odds ratio; PD-1, programmed cell death-1; PD-L1, programmed cell death ligand 1; PE, pulmonary embolism; TE, thromboembolic events; TF, tissue factor; VTE, venous thromboembolism.
1. Blom JW, Doggen CJM, Osanto S, Rosendaal FR. Malignancies, Prothrombotic Mutations, and the Risk of Venous Thrombosis. JAMA (2005) 293(6):715–22. doi: 10.1001/jama.293.6.715
2. Timp JF, Braekkan SK, Versteeg HH, Cannegieter SC. Epidemiology of Cancer-Associated Venous Thrombosis. Blood (2013) 122(10):1712–23. doi: 10.1182/blood-2013-04-460121
3. Sørensen HT, Mellemkjaer L, Olsen JH, Baron JA. Prognosis of Cancers Associated With Venous Thromboembolism. N Engl J Med (2000) 343(25):1846–50. doi: 10.1056/NEJM200012213432504
4. Ay C, Ünal UK. Epidemiology and Risk Factors for Venous Thromboembolism in Lung Cancer. Curr Opin Oncol (2016) 28(2):145–9. doi: 10.1097/CCO.0000000000000262
5. Yu J, Li A, Laureano M, Crowther M. Frequency of Arterial Thromboembolism in Populations With Malignancies: A Systematic Review. Thromb Res (2019) 184:16–23. doi: 10.1016/j.thromres.2019.10.004
6. König D, Savic Prince S, Rothschild SI. Targeted Therapy in Advanced and Metastatic Non-Small Cell Lung Cancer. an Update on Treatment of the Most Important Actionable Oncogenic Driver Alterations. Cancers (Basel) (2021) 13(4):804. doi: 10.3390/cancers13040804
7. Ettinger DS, Wood DE. NCCN Clinical Practice Guidelines in Oncology, in: Non-Small Cell Lung Cancer (2021). Available at NCCN.org (Accessed on 27 March 2021).
8. Ng TL, Smith DE, Mushtaq R, Patil T, Dimou A, Yang S, et al. ROS1 Gene Rearrangements are Associated With an Elevated Risk of Peridiagnosis Thromboembolic Events. J Thorac Oncol (2019) 14(4):596–605. doi: 10.1016/j.jtho.2018.12.001
9. Al-Samkari H, Leiva O, Dagogo-Jack I, Shaw A, Lennerz J, Iafrate AJ, et al. Impact of ALK Rearrangement on Venous and Arterial Thrombotic Risk in NSCLC. J Thoracic Oncol (2020) 15(9):1497–506. doi: 10.1016/j.jtho.2020.04.033
10. Chiari R, Ricciuti B, Landi L, Morelli AM, Delmonte A, Spitaleri G, et al. ROS1-Rearranged Non-Small-Cell Lung Cancer is Associated With a High Rate of Venous Thromboembolism: Analysis From a Phase II, Prospective, Multicenter, Two-Arms Trial (METROS). Clin Lung Cancer (2020) 21(1):15–20. doi: 10.1016/j.cllc.2019.06.012
11. Corrales-Rodriguez L, Soulières D, Weng X, Tehfe M, Florescu M, Blais N. Mutations in NSCLC and Their Link With Lung Cancer-Associated Thrombosis: A Case-Control Study. Thromb Res (2014) 133(1):48–51. doi: 10.1016/j.thromres.2013.10.042
12. Verso M, Chiari R, Mosca S, Franco L, Fischer M, Paglialunga L, et al. Incidence of Ct Scan-Detected Pulmonary Embolism in Patients With Oncogene-Addicted, Advanced Lung Adenocarcinoma. Thromb Res (2015) 136(5):924–7. doi: 10.1016/j.thromres.2015.09.006
13. Dou F, Li H, Zhu M, Liang L, Zhang Y, Yi J, et al. Association Between Oncogenic Status and Risk of Venous Thromboembolism in Patients With Non-Small Cell Lung Cancer. Respir Res (2018) 19(1):88. doi: 10.1186/s12931-018-0791-2
14. Wang J, Hu B, Li T, Miao J, Zhang W, Chen S, et al. The EGFR-Rearranged Adenocarcinoma is Associated With a High Rate of Venous Thromboembolism. Ann Trans Med (2019) 7(23):724. doi: 10.21037/atm.2019.12.24
15. Leiva O, Connors JM, Al-Samkari H. Impact of Tumor Genomic Mutations on Thrombotic Risk in Cancer Patients. Cancers (Basel) (2020) 12(7):1958. doi: 10.3390/cancers12071958
16. Phan M, John S, Casanegra AI, Rathbun S, Mansfield A, Stoner JA, et al. Primary Venous Thromboembolism Prophylaxis in Patients With Solid Tumors: A Meta-Analysis. J Thromb Thrombolysis (2014) 38(2):241–9. doi: 10.1007/s11239-013-1014-9
17. Mansfield AS, Tafur AJ, Wang CE, Kourelis TV, Wysokinska EM, Yang P. Predictors of Active Cancer Thromboembolic Outcomes: Validation of the Khorana Score Among Patients With Lung Cancer. J Thromb Haemostasis JTH (2016) 14(9):1773–8. doi: 10.1111/jth.13378
18. van Es N, Di Nisio M, Cesarman G, Kleinjan A, Otten H-M, Mahé I, et al. Comparison of Risk Prediction Scores for Venous Thromboembolism in Cancer Patients: A Prospective Cohort Study. Haematologica (2017) 102(9):1494–501. doi: 10.3324/haematol.2017.169060
19. Carrier M, Abou-Nassar K, Mallick R, Tagalakis V, Shivakumar S, Schattner A, et al. Apixaban to Prevent Venous Thromboembolism in Patients With Cancer. N Engl J Med (2019) 380(8):711–9. doi: 10.1056/NEJMoa1814468
20. Khorana AA, Soff GA, Kakkar AK, Vadhan-Raj S, Riess H, Wun T, et al. Rivaroxaban for Thromboprophylaxis in High-Risk Ambulatory Patients With Cancer. N Engl J Med (2019) 380(8):720–8. doi: 10.1056/NEJMoa1814630
21. Moher D, Liberati A, Tetzlaff J, Altman DG. Preferred Reporting Items for Systematic Reviews and Meta-Analyses: The PRISMA Statement. PloS Med (2009) 6(7):e1000097. doi: 10.1371/journal.pmed.1000097
22. Wells G, Shea B, O’Connell J. The Newcastle-Ottawa Scale (NOS) for Assessing the Quality of Nonrandomised Studies in Meta-Analyses. Ottawa Health Res Institute Web Site (2014) 7. doi: 10.2307/632432
23. Alexander M, Pavlakis N, John T, O’Connell R, Kao S, Hughes BGM, et al. A Multicenter Study of Thromboembolic Events Among Patients Diagnosed With ROS1-Rearranged Non-Small Cell Lung Cancer. Lung Cancer (2020) 142:34–40. doi: 10.1016/j.lungcan.2020.01.017
24. Azevedo S, Bei L, Cunha J, Oliveira C, Rodrigues A, Pousa I, et al. Anaplastic Lymphoma Kinase Fusion Oncogene Positive Non-Small Cell Lung Cancer-the Experience of an Institution. J Thoracic Oncol (2017) 12(1):S1179. doi: 10.1016/j.jtho.2016.11.1658
25. Berger N, Datta D, Tannenbaum S. Venous Thromboembolism and EGFR Mutation Status in Advanced Adenocarcinoma of Lung. Chest (2014) 146(4):1112–20. doi: 10.1378/chest.1977798
26. Davidsson E, Murgia N, Ortiz-Villalón C, Wiklundh E, Sköld M, Kölbeck KG, et al. Mutational Status Predicts the Risk of Thromboembolic Events in Lung Adenocarcinoma. Multidiscip Respir Med (2017) 12(1):16. doi: 10.1186/s40248-017-0097-0
27. Delmonte A, Mariotti M, Scarpi E, Ulivi P, Gavelli G, Calistri D, et al. Adenocarcinoma of the Lung and Venous Thromboembolic Events: A Monoinstitutional, Retrospective Evaluation. J Clin Oncol (2015) 33(15_suppl):e19149–e19149. doi: 10.1200/jco.2015.33.15_suppl.e19149
28. Dou F, Zhang Y, Yi J, Zhu M, Zhang S, Zhang D, et al. Association of ALK Rearrangement and Risk of Venous Thromboembolism in Patients With Non-Small Cell Lung Cancer: A Prospective Cohort Study. Thromb Res (2020) 186:36–41. doi: 10.1016/j.thromres.2019.12.009
29. Leader A, Icht O, Batat E, Rozovski U, Joseph L, Shochat T, et al. Incidence and Risk Factors of Arterial Thromboembolism in Non-Small Cell Lung Cancer. Blood (2019) 134(Supplement_1):3654. doi: 10.1182/blood-2019-128822
30. Lee Y-G, Kim I, Lee E, Bang S-M, Kang CH, Kim YT, et al. Risk Factors and Prognostic Impact of Venous Thromboembolism in Asian Patients With Non-Small Cell Lung Cancer. Thromb Haemostasis (2014) 111(6):1112–20. doi: 10.1160/TH13-11-0956
31. Muñoz-Unceta N, Zugazagoitia J, Manzano A, Jiménez-Aguilar E, Olmedo ME, Cacho JD, et al. High Risk of Thrombosis in Patients With Advanced Lung Cancer Harboring Rearrangements in ROS1. Eur J Cancer (2020) 141:193–8. doi: 10.1016/j.ejca.2020.10.002
32. Roopkumar J, Poudel SK, Gervaso L, Reddy CA, Velcheti V, Pennell NA, et al. Risk of Thromboembolism in Patients With ALK and EGFR-Mutant Lung Cancer: A Cohort Study. J Thromb Haemostasis JTH (2020) 19(3):822–9. doi: 10.1111/jth.15215
33. Shahzad H, Datta D. Correlation of EGFR Mutation Status to Venous Thromboembolism in Patients With Lung Adenocarcinoma. Am J Respir Crit Care Med (2017) 195:A4607. doi: 10.1164/ajrccm-conference.2017.B80-L
34. Shen Q, Dong X, Tang X, Zhou J. Risk Factors and Prognosis Value of Venous Thromboembolism in Patients With Advanced Non-Small Cell Lung Cancer: A Casecontrol Study. J Thoracic Dis (2017) 9(12):5068–74. doi: 10.21037/jtd.2017.11.116
35. Xiong W, Du H, Ding W, Sun J, Xu M, Guo X. The Association Between Pulmonary Embolism and the Cancer-Related Genomic Alterations in Patients With NSCLC. Respir Res (2020) 21(1):185. doi: 10.1186/s12931-020-01437-6
36. Yamazaki S, Okano T, Ishikawa R, Kobayashi K. Pulmonary Thromboembolism in Lung Cancer Patients and EGFR Mutations. Respirology (2013) 18:22. doi: 10.1111/resp.12183
37. Yang S, Yang L, Wu Y, Zhang C, Wang S, Ma N, et al. Anaplastic Lymphoma Kinase Rearrangement May Increase the Incidence of Venous Thromboembolism by Increasing Tissue Factor Expression in Advanced Lung Adenocarcinoma. Ann Trans Med (2020) 8(20):1307. doi: 10.21037/atm-20-6619
38. Zer A, Moskovitz M, Hwang DM, Hershko-Klement A, Fridel L, Korpanty GJ, et al. ALK-Rearranged Non–Small-Cell Lung Cancer is Associated With a High Rate of Venous Thromboembolism. Clin Lung Cancer (2017) 18(2):156–61. doi: 10.1016/j.cllc.2016.10.007
39. Zer A, Joseph L, Battat E, Shochat T, Leader A, Goldstein DA, et al. Venous Thromboembolism (VTE) in Patients With ALK-Rearranged Non-Small Cell Lung Cancer (ALK-LC): A Population-Based Cohort. J Clin Oncol (2019) 37:6608–6608. doi: 10.1200/JCO.2019.37.15_suppl.6608
40. Zugazagoitia J, Biosca M, Oliveira J, Olmedo ME, Dómine M, Nadal E, et al. Incidence, Predictors and Prognostic Significance of Thromboembolic Disease in Patients With Advanced ALK-Rearranged Non-Small Cell Lung Cancer. Eur Respir J (2018) 51(5):1702431. doi: 10.1183/13993003.02431-2017
41. Navi BB, Reiner AS, Kamel H, Iadecola C, Okin PM, Elkind MSV, et al. Risk of Arterial Thromboembolism in Patients With Cancer. J Am Coll Cardiol (2017) 70(8):926–38. doi: 10.1016/j.jacc.2017.06.047
42. Takeuchi K, Choi YL, Soda M, Inamura K, Togashi Y, Hatano S, et al. Multiplex Reverse Transcription-PCR Screening for EML4-ALK Fusion Transcripts. Clin Cancer Res (2008) 14(20):6618–24. doi: 10.1158/1078-0432.CCR-08-1018
43. Gainor JF, Shaw AT. Novel Targets in Non-Small Cell Lung Cancer: ROS1 and RET Fusions. Oncol (2013) 18(7):865–75. doi: 10.1634/theoncologist.2013-0095
44. Nichetti F, Lo Russo G, Prelaj A, Provenzano L, de Braud F, Cabiddu M, et al. ALK/ROS1 Rearrangements: A Real Hallmark for Thromboembolic Events in Cancer Patients? Thromb Res (2020) 194:176–7. doi: 10.1016/j.thromres.2020.06.041
45. Duarte CW, Lindner V, Francis SA, Schoormans D. Visualization of Cancer and Cardiovascular Disease Co-Occurrence With Network Methods. JCO Clin Cancer Inform (2017) 1:1–12. doi: 10.1200/CCI.16.00071
46. Navi BB, Reiner AS, Kamel H, Iadecola C, Okin PM, Tagawa ST, et al. Arterial Thromboembolic Events Preceding the Diagnosis of Cancer in Older Persons. Blood (2019) 133(8):781–9. doi: 10.1182/blood-2018-06-860874
47. Rickles FR, Patierno S, Fernandez PM. Tissue Factor, Thrombin, and Cancer. Chest (2003) 124(3 Suppl):58S–68S. doi: 10.1378/chest.124.3_suppl.58S
48. Rak J, Milsom C, Yu J. Tissue Factor in Cancer. Curr Opin Hematol (2008) 15(5):522–8. doi: 10.1097/MOH.0b013e3283063a3e
49. Sen Y, Tang H, Wang Q. ALK-Rearranged May Promote VTE by Increasing the Expression of TF in Advanced Lung Adenocarcinoma. J Thoracic Oncol (2017) 12(11):S1899–. doi: 10.1016/j.jtho.2017.09.671
50. Yu JL, May L, Lhotak V, Shahrzad S, Shirasawa S, Weitz JI, et al. Oncogenic Events Regulate Tissue Factor Expression in Colorectal Cancer Cells: Implications for Tumor Progression and Angiogenesis. Blood (2005) 105(4):1734–41. doi: 10.1182/blood-2004-05-2042
51. Regina S, Rollin J, Bléchet C, Iochmann S, Reverdiau P, Gruel Y. Tissue Factor Expression in Non-Small Cell Lung Cancer: Relationship With Vascular Endothelial Growth Factor Expression, Microvascular Density, and K-Ras Mutation. J Thoracic Oncol (2008) 3(7):689–97. doi: 10.1097/JTO.0b013e31817c1b21
52. Rao B, Gao Y, Huang J, Gao X, Fu X, Huang M, et al. Mutations of P53 and K-Ras Correlate TF Expression in Human Colorectal Carcinomas: TF Downregulation as a Marker of Poor Prognosis. Int J Colorectal Dis (2011) 26(5):593–601. doi: 10.1007/s00384-011-1164-1
53. De Caterina R, D’Ugo E, Libby P. Inflammation and Thrombosis - Testing the Hypothesis With Anti-Inflammatory Drug Trials. Thromb Haemostasis (2016) 116(6):1012–21. doi: 10.1160/TH16-03-0246
54. Crescenzo R, Abate F, Lasorsa E, Tabbo F, Gaudiano M, Chiesa N, et al. Convergent Mutations and Kinase Fusions Lead to Oncogenic STAT3 Activation in Anaplastic Large Cell Lymphoma. Cancer Cell (2015) 27(4):516–32. doi: 10.1016/j.ccell.2015.03.006
55. Zhang B, Wei W, Qiu J. ALK is Required for NLRP3 Inflammasome Activation in Macrophages. Biochem Biophys Res Commun (2018) 501(1):246–52. doi: 10.1016/j.bbrc.2018.04.226
56. Cella CA, Di Minno G, Carlomagno C, Arcopinto M, Cerbone AM, Matano E, et al. Preventing Venous Thromboembolism in Ambulatory Cancer Patients: The ONKOTEV Study. Oncol (2017) 22(5):601–8. doi: 10.1634/theoncologist.2016-0246
57. Li R, Hermann G, Baldini E, Chen A, Jackman D, Kozono D, et al. Advanced Nodal Stage Predicts Venous Thromboembolism in Patients With Locally Advanced Non-Small Cell Lung Cancer. Lung Cancer (Amsterdam Netherlands) (2016) 96:41–7. doi: 10.1016/j.lungcan.2016.03.004
58. Lee SE, Lee B, Hong M, Song J-Y, Jung K, Lira ME, et al. Comprehensive Analysis of RET and ROS1 Rearrangement in Lung Adenocarcinoma. Modern Pathol (2015) 28(4):468–79. doi: 10.1038/modpathol.2014.107
59. Wang R, Hu H, Pan Y, Li Y, Ye T, Li C, et al. RET Fusions Define a Unique Molecular and Clinicopathologic Subtype of Non-Small-Cell Lung Cancer. J Clin Oncol (2012) 30(35):4352–9. doi: 10.1200/JCO.2012.44.1477
60. Liu Z, Liang H, Lin J, Cai X, Pan Z, Liu J, et al. The Incidence of Lymph Node Metastasis in Patients With Different Oncogenic Driver Mutations Among T1 Non-Small-Cell Lung Cancer. Lung Cancer (Amsterdam Netherlands) (2019) 134:218–24. doi: 10.1016/j.lungcan.2019.06.026
61. Nichetti F, Ligorio F, Zattarin E, Signorelli D, Prelaj A, Proto C, et al. Is There an Interplay Between Immune Checkpoint Inhibitors, Thromboprophylactic Treatments and Thromboembolic Events? Mech Impact Non-Small Cell Lung Cancer Patients Cancers (Basel) (2019) 12(1):67. doi: 10.3390/cancers12010067
62. Mir Seyed Nazari P, Berghoff AS, Preusser M, Moik F, Posch F, Ricken G, et al. Association of Programmed Cell Death Ligand 1 and Circulating Lymphocytes With Risk of Venous Thromboembolism in Patients With Glioma. ESMO Open (2020) 5(3):e000647–e. doi: 10.1136/esmoopen-2019-000647
63. Bu D-x, Tarrio M, Maganto-Garcia E, Stavrakis G, Tajima G, Lederer J, et al. Impairment of the Programmed Cell Death-1 Pathway Increases Atherosclerotic Lesion Development and Inflammation. Arterioscler Thromb Vasc Biol (2011) 31(5):1100–7. doi: 10.1161/ATVBAHA.111.224709
64. Cochain C, Chaudhari SM, Koch M, Wiendl H, Eckstein H-H, Zernecke A. Programmed Cell Death-1 Deficiency Exacerbates T Cell Activation and Atherogenesis Despite Expansion of Regulatory T Cells in Atherosclerosis-Prone Mice. PLoS One (2014) 9(4):e93280–e. doi: 10.1371/journal.pone.0093280
65. De Palma R, Cirillo P, Ciccarelli G, Barra G, Conte S, Pellegrino G, et al. Expression of Functional Tissue Factor in Activated T-Lymphocytes in Vitro and in Vivo: A Possible Contribution of Immunity to Thrombosis? Int J Cardiol (2016) 218:188–95. doi: 10.1016/j.ijcard.2016.04.177
66. Joseph L, Fink LM, Hauer-Jensen M. Cytokines in Coagulation and Thrombosis: A Preclinical and Clinical Review. Blood Coagul Fibrinolysis (2002) 13(2):105–16. doi: 10.1097/00001721-200203000-00005
67. Kitano A, Ono M, Yoshida M, Noguchi E, Shimomura A, Shimoi T, et al. Tumour-Infiltrating Lymphocytes are Correlated With Higher Expression Levels of PD-1 and PD-L1 in Early Breast Cancer. ESMO Open (2017) 2(2):e000150–e. doi: 10.1136/esmoopen-2016-000150
Keywords: venous thromboembolism (VTE), arterial thromboembolism (ATE), non-small-cell lung cancer, ALK, ROS1, EGFR, KRAS
Citation: Qian X, Fu M, Zheng J, Zhou J and Zhou J (2021) Driver Genes Associated With the Incidence of Venous Thromboembolism in Patients With Non-Small-Cell Lung Cancer: A Systematic Review and Meta-Analysis. Front. Oncol. 11:680191. doi: 10.3389/fonc.2021.680191
Received: 13 March 2021; Accepted: 06 April 2021;
Published: 29 April 2021.
Edited by:
Umberto Malapelle, University of Naples Federico II, ItalyReviewed by:
Chunxia Su, Shanghai Pulmonary Hospital, ChinaCopyright © 2021 Qian, Fu, Zheng, Zhou and Zhou. This is an open-access article distributed under the terms of the Creative Commons Attribution License (CC BY). The use, distribution or reproduction in other forums is permitted, provided the original author(s) and the copyright owner(s) are credited and that the original publication in this journal is cited, in accordance with accepted academic practice. No use, distribution or reproduction is permitted which does not comply with these terms.
*Correspondence: Jianying Zhou, emp5aHpAemp1LmVkdS5jbg==
Disclaimer: All claims expressed in this article are solely those of the authors and do not necessarily represent those of their affiliated organizations, or those of the publisher, the editors and the reviewers. Any product that may be evaluated in this article or claim that may be made by its manufacturer is not guaranteed or endorsed by the publisher.
Research integrity at Frontiers
Learn more about the work of our research integrity team to safeguard the quality of each article we publish.