- 1Department of Radiation Oncology, Massachusetts General Hospital, Harvard Medical School, Boston, MA, United States
- 2Massachusetts General Hospital Cancer Center, Charlestown, MA, United States
Immunotherapy drugs have recently been approved by the Food and Drug Administration for the treatment of several genitourinary malignancies, including bladder cancer, renal cancer, and prostate cancer. Preclinical data and early clinical trial results suggest that immune checkpoint inhibitors can act synergistically with radiation therapy to enhance tumor cell killing at local irradiated sites and in some cases at distant sites through an abscopal effect. Because radiation therapy is commonly used in the treatment of genitourinary malignancies, there is great interest in testing the combination of immunotherapy with radiation therapy in these cancers to further improve treatment efficacy. In this review, we discuss the current evidence and biological rationale for combining immunotherapy with radiation therapy, as well as emerging data from ongoing and planned clinical trials testing the efficacy and tolerability of this combination in the treatment of genitourinary malignancies. We also outline outstanding questions regarding sequencing, dose fractionation, and biomarkers that remain to be addressed for the optimal delivery of this promising treatment approach.
Introduction
Prostate, bladder, and kidney/renal pelvis cancers rank fourth, seventh, and eighth, respectively, in estimated cancer-related deaths in the United States in 2020 (1). Radiation therapy is a well-established treatment modality for genitourinary malignancies, with clinical utility in the definitive, adjuvant, and palliative settings. In localized prostate cancer, for example, radiation therapy is a curative treatment option with survival outcomes that have been shown to be equivalent to those of radical prostatectomy (2). In bladder cancer, radiation is a critical part of bladder-preserving trimodality therapy, which has comparable outcomes to radical cystectomy in well-selected patients (3). Renal cell carcinoma has traditionally been considered relatively radioresistant, but recent advances in radiation delivery and image guidance technologies have led to the development of stereotactic body radiotherapy (SBRT), which enables the focal and conformal delivery of ablative radiation doses sufficient for the definitive treatment of primary renal cancer (4). In patients with metastatic cancer, palliative radiotherapy is frequently used to alleviate pain from bone metastases. In addition, emerging data suggests that in oligometastatic cancers with five or fewer metastatic lesions, the aggressive use of SBRT to ablate all sites of metastatic disease can lead to improved clinical outcomes (5, 6).
The last several years have also seen the rapid availability of immunotherapy drugs that increase overall survival in patients with a variety of cancers, including genitourinary malignancies (7). Immunotherapy utilizes the patient’s immune system to induce tumor cell killing and can be either active or passive in nature. Active immunotherapy directly targets tumor cells and includes antibody therapy and chimeric antigen receptor T-cell therapy. In contrast, passive immunotherapy enhances the ability of the immune system to eradicate tumor cells and includes checkpoint inhibitors and cytokines. Among these approaches, immune checkpoint inhibitors have shown some of the most promising clinical activity to date. Currently available checkpoint inhibitors target two immune checkpoints: PD-1/PD-L1, which modulates T-cell activity resulting in immune response inhibition (8), and CTLA-4, an immunoglobulin expressed by activated T cells that downregulates immune response (9). FDA-approved therapies that target these immune checkpoints include atezolizumab, durvalumab, pembrolizumab, nivolumab (PD-1/PD-L1), and ipilimumab (CTLA-4), among others (10, 11).
Recent data suggest that radiotherapy and immunotherapy may act synergistically, and there has been mounting excitement about the possibility of combining these modalities to further improve outcomes in patients with genitourinary cancers. In this review, we discuss the pre-clinical mechanistic rationale for combining radiotherapy with immunotherapy, as well as emerging data from ongoing and planned clinical trials testing the efficacy and tolerability of this combination in genitourinary malignancies.
Biological Rationale for Combining Radiotherapy and Immunotherapy
Radiotherapy Can Augment Immunotherapy
Several lines of evidence suggest that radiation can stimulate the tumor immune microenvironment, a concept that underlies a key rationale for combining radiotherapy with immunotherapy (12). In many cancers, the immune microenvironment becomes altered from a state of immune recognition/antagonism towards a state of immune escape, where the immune system becomes incapable of combatting the tumor (13). Biological changes commonly associated with immune escape include reduced MHC-class 1 expression, upregulated inhibitory ligands and cytokines, and increased numbers of myeloid-derived suppressor cells (14). Although the primary mechanism by which radiation causes local cell death is through the induction of DNA double-strand breaks (15), radiation has been shown to be immunogenic through the direct and indirect activation of innate and adaptive immune response (Figure 1) (16). Local cell death caused by radiation instigates the direct release of tumor antigens and promotes the priming and activation of cytotoxic T cells. In addition, radiation can promote the ability of antigen-presenting cells to present tumor antigens to naive T cells through the stimulation of calreticulin, a calcium-binding protein that promotes phagocytosis (17, 18). Conversely, radiation has also been found to downregulate the presence of CD47, a protein that signals down-regulation of phagocytosis (19). High radiation doses have been shown to increase MHC-1 expression, increasing the likelihood of tumor-specific peptide presentation by antigen-presenting cells to naïve T cells (20). This phenotype, in conjunction with increased expression of death receptors such as Fas, facilitates the immune system’s ability to kill tumor cells by enhancing the visibility of the tumor to cytotoxic T cells (21, 22). Moderate doses of radiation have also been shown to activate a type I interferon response in tumor cells through the sensing of cytoplasmic DNA derived from tumor micronuclei via the cGAS-STING pathway (23–26). Through these different processes, radiation therapy ultimately creates a proinflammatory microenvironment that instigates immune activation in a manner that may be synergistic with immunotherapy.
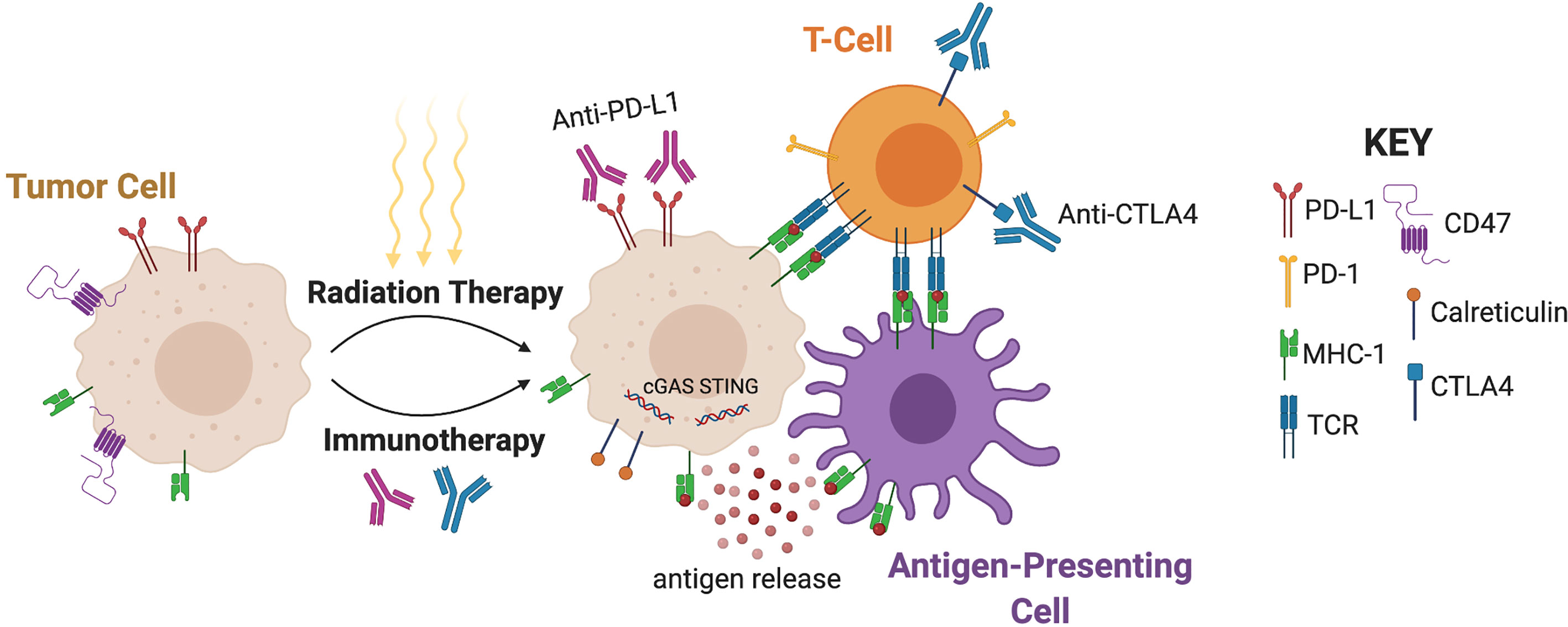
Figure 1 Mechanisms underlying synergy of radiotherapy and immunotherapy. Radiation promotes the ability of antigen-presenting cells to present tumor antigens to naive T cells through antigen release, stimulation of calreticulin, and downregulation of CD47. MHC-1 expression and the subsequent antigen presentation leads to interaction with T-Cell Receptors (TCR). Moderate doses of radiation also activate a type I interferon response through the sensing of cytoplasmic DNA via cGAS-STING. Radiation can upregulate PD-L1 and CTLA-4, and therefore immunotherapy can augment radiation efficacy by targeting these pathways. (Created with BioRender.com).
Immunotherapy May Augment Radiotherapy
Not all tumors will respond to radiation, despite administration of definitive doses. Although the reason for radioresistance remains unclear, one hypothesis is that immune-mediated mechanisms may be involved (27). It is important to note that although radiation can be immunogenic, it can also be immune-suppressive. Radiation can directly kill immune cells in or near the tumor through DNA double strand breaks and apoptotic cell death, which in turn may negatively impact T cells in peripheral circulation (28). For example, a retrospective study of prostate cancer patients treated with (N=36) or without (N=95) pelvic nodal irradiation demonstrated a higher risk of radiation-related lymphopenia with pelvic nodal irradiation (29). Indirectly, while activation of type 1 interferon through cGAS-STING induces recruitment of effector T cells and antigen presenting cells (30), it can also upregulate transforming growth factor β (TGF-β), which triggers an immune-suppressive environment (31–33). Radiation can also drive the recruitment of myeloid-derived suppressor cells (MDSCs) (34), which serve as critical mediators of immunosuppression and inhibit effector T cells as well as induce Tregs (35). Increased infiltration of Tregs into the tumor microenvironment through radiation can downregulate the immune response (36). As a result, radiation’s impact on MDSCs and T cells may promote tumor growth, local invasion, and subsequent metastases (37). Thus, therapies that counteract this effect by augmenting T-cell function may lead to improved control of the tumor (38). Radiation can also alter the balance of key immune checkpoint pathways including PD-L1 and CTLA-4. Radiation temporarily upregulates PD-L1 in mice with bladder cancer (39). The binding of the PD-L1 protein to the inhibitory checkpoint molecule PD-1 reduces the proliferation of antigen-specific T cells in lymph nodes (40). Similarly, radiation can upregulate the CTLA-4 receptor in T cells, leading to a downregulated immune response (41, 42). Thus, an important rationale for incorporating immunotherapy into radiotherapy regimens is to augment the efficacy of radiation by selectively targeting these immune suppressive effects.
Radiotherapy and Immunotherapy Are Synergistic
Compared to other cancer treatments, tumor response to immunotherapy is often slower and may result in transient increases in tumor burden, even in patients who have an effective immune response (43). Radiotherapy could potentially greatly reduce the growth of such tumors, thus enabling patients to respond to the immunotherapy for longer periods of time (44). In a similar vein, radiation can be used to prime the tumor for immunotherapy by increasing the susceptibility of tumor cells to immune-mediated treatment (45). Moreover, combining immune modulating agents and radiation may induce protective immunologic memory, which could prevent disease recurrence. Finally, reports in the literature suggest that combining immune checkpoint inhibitors and radiotherapy may result in increased frequency of the “abscopal effect,” the immunogenic cell killing of untreated distant tumors (46). Although the potential mechanism for the abscopal effect may include radiation-induced stimulation of systemic recognition of tumor-related antigens, the overall rarity of clinical cases necessitates further investigation (46, 47).
Clinical Evidence for Combining Radiotherapy and Immunotherapy
Non-Genitourinary Cancers
Several clinical studies have demonstrated a benefit for the combination of radiotherapy and immunotherapy in non-genitourinary cancers, as reviewed comprehensively elsewhere (44). For example, in lung cancer, the PACIFIC trial enrolled 709 non-small cell lung cancer (NSCLC) patients previously treated with platinum-based chemoradiation and randomized them in a 2:1 ratio to receive either adjuvant durvalumab or placebo. Treatment with durvalumab resulted in an increase in median progression-free survival and 2-year overall survival (66.3% vs 55.6%, P=0.005) (48, 49). In a secondary analysis of the KEYNOTE-001 phase 1 trial of pembrolizumab in NSCLC, patients who had previously received radiation therapy prior to receiving pembrolizumab experienced an increased median progression-free survival and overall survival compared to patients without previous radiotherapy (50). In the PEMBRO-RT Phase 2 randomized trial, 76 NSCLC patients received either pembrolizumab and SRBT (3 x 8 Gy within 7 days prior to the first cycle) or pembrolizumab alone. The study found that pembrolizumab preceded by SBRT resulted in a doubling of the overall response rate at 12 weeks (36% vs 18%, P=0.07) without any significant increase in toxicity, although this did not meet the prespecified endpoint for meaningful clinical benefit (51). Interestingly, subgroup analyses showed the largest benefit from the addition of radiation in patients with PD-L1 negative tumors.
Prostate Cancer
Although numerous clinical trials are investigating the combination of radiotherapy and immunotherapy in genitourinary cancers (Table 1), only a few randomized trials have been published to date with mature results. In prostate cancer, a multicenter phase 3 trial investigated the use of ipilimumab vs. placebo after bone-directed radiotherapy (8 Gy x 1 fraction) in 799 men with metastatic castration-resistant prostate cancer that progressed after docetaxel (63). Ipilimumab therapy was associated with a trend towards increased overall survival that was not statistically significant (P=0.053). However, subgroup analyses suggested that patients with favorable prognostic features such as the absence of visceral metastasis or anemia and normal alkaline phosphatase did have a significant improvement in survival with the addition of ipilimumab (63). In a phase 2 trial, 49 patients with oligometastatic hormone-sensitive prostate cancer were randomized to receive either the autologous cellular immunotherapy sipuleucel-T preceded by radiotherapy (30 Gy to a single metastatic site) or sipuleucel-T alone (52). Median progression-free survival was higher with the addition of radiotherapy (3.65 vs. 2.46 months, P=0.06), but this was not statistically significant. Overall, radiotherapy did not significantly enhance the humoral and cellular responses associated with sipuleucel-T.
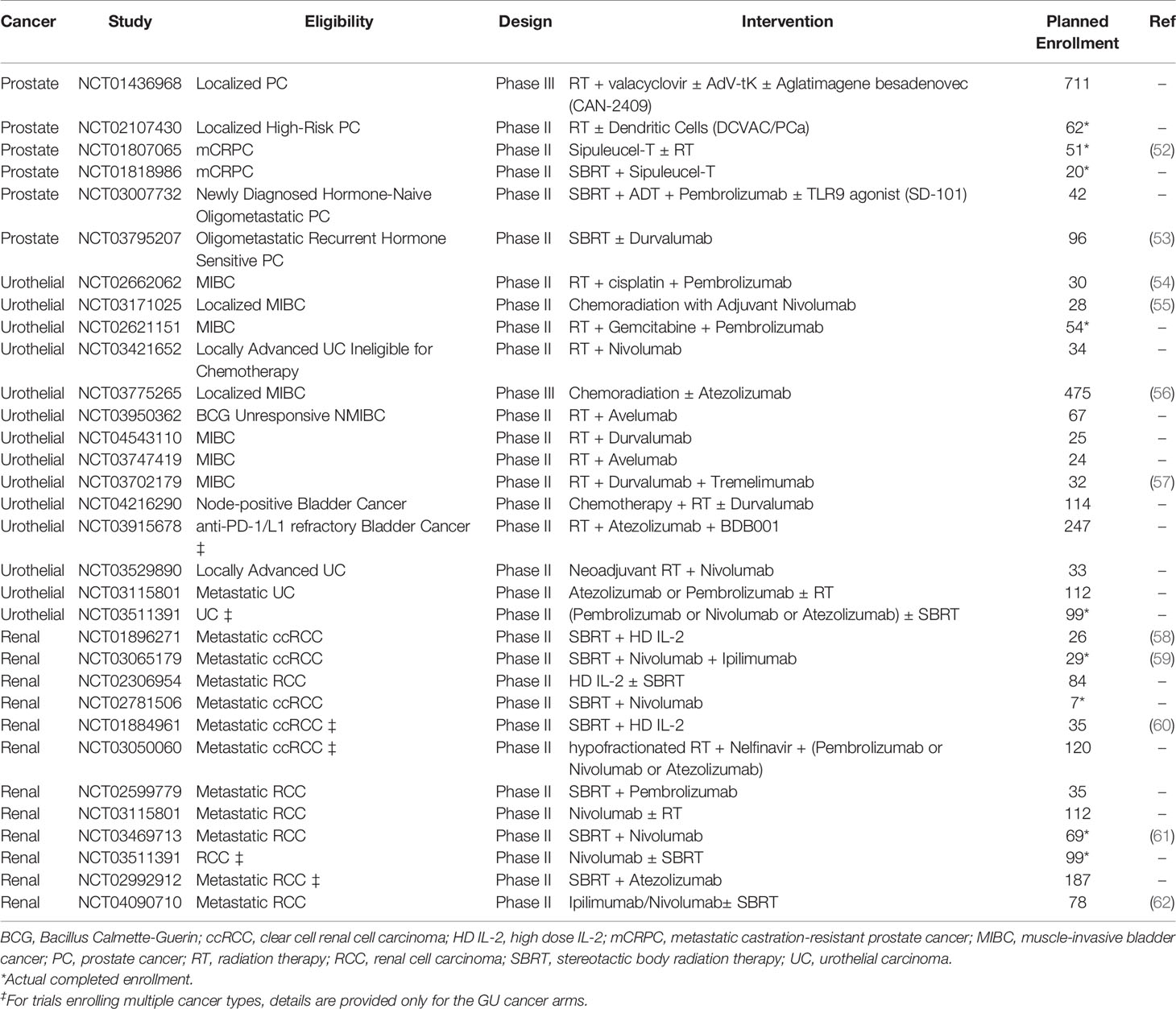
Table 1 Active Phase II and III clinical trials combining immunotherapy with radiation therapy in genitourinary cancers.
Although these clinical trials have not demonstrated a definite benefit for the addition of radiotherapy to immunotherapy, results from additional ongoing clinical trials in prostate cancer are pending, including those testing PD-1/PD-L1 checkpoint inhibitors in combination with radiotherapy (Table 1). For example, in an ongoing phase 2 study (NCT03795207), 96 oligometastatic prostate cancer patients are randomized to either SBRT with durvalumab or SBRT alone in a 2:1 manner. Durvalumab (1500 mg/cycle) is administered one month prior to SBRT (3 x 9 Gy or 3 x 11 Gy) and continued until progression with a maximum of 12 months. The primary endpoint of the trial is 2-year progression-free survival (53).
Kidney Cancer
Immune checkpoint inhibition has become a standard of care treatment for patients with metastatic renal cell carcinoma (RCC) (64, 65). Multiple clinical trials are currently evaluating whether the addition of radiotherapy to immunotherapy will further improve outcomes in this disease (Table 1). Early results have been presented for the RADVAX RCC single arm phase 2 trial (NCT03065179), in which 25 metastatic RCC patients received nivolumab and ipilimumab (N/I) with SBRT (50 Gy in 5 fractions) between the first and second doses of N/I (59). Partial responses were observed in 14/25 patients, for an objective response rate of 56%, which is higher than the expected response rate of 40%. The regimen was noted to have acceptable safety, although 10 (40%) patients required prednisone for immune-related adverse events. These results are encouraging for further investigation, although the study is limited by its small sample size and single-site design.
Preliminary results of the NIVES single arm phase 2 multicenter study (NCT03469713) have been presented recently, in which patients with metastatic RCC that progressed on up to two prior systemic therapies were treated with nivolumab for 6 months, in combination with SBRT (10 Gy x 3 fractions) to one metastatic lesion given 7 days after initiation of nivolumab (61). At a median follow-up of 15 months, the objective response rate was 17.4% (12/68 patients). Although tolerability was acceptable [most frequent grade 3/4 toxicities were diarrhea (5.8%), elevated amylase/lipase (4.3%), and fatigue (4.3%)], the study did not meet its primary endpoint of improving response rate to 40%.
Overall, the available results for combining immunotherapy with radiotherapy are mixed in RCC. Additional data from ongoing clinical trials are anticipated to clarify whether changing the timing or target site of SBRT will further improve outcomes. For example, to test the strategy of targeting the primary kidney lesion with SBRT rather than targeting metastases in this context, the CYTOSHRINK phase 2 trial (NCT04090710) will randomize up to 78 untreated advanced RCC patients to receive ipilimumab/nivolumab plus SBRT to the primary lesion (30-40 Gy in 5 fractions) between cycles 1 and 2, or ipilimumab/nivolumab alone (62).
Bladder Cancer
Although muscle-invasive bladder cancer has historically been treated with radical cystectomy, bladder-preserving trimodality therapy consisting of transurethral tumor resection, radiotherapy, and chemotherapy is now considered a standard treatment option according to consensus clinical guidelines (66, 67). Several clinical trials are examining the potential role of adding immunotherapy to further improve outcomes of these patients (Table 1). A phase Ib study (NCT02891161) demonstrated the safety of combining the anti-PD-L1 checkpoint inhibitor durvalumab with radiation therapy to the bladder (64.8 Gy in 36 fractions) in 6 patients with locally advanced bladder cancer, with no patients experienced dose limiting toxicity (68). A follow-up randomized phase 2 study (ECOG-ACRIN/NRG 8185; NCT04216290) is examining the addition of durvalumab to chemoradiation therapy in patients with clinically node-positive (N1-2) muscle-invasive bladder cancer. A large cooperative group randomized phase 3 study (SWOG/NRG 1806; NCT03775265) with a planned accrual of 475 patients is investigating the addition of the anti-PD-L1 inhibitor atezolizumab to chemoradiation in patients with localized muscle-invasive bladder cancer. Safety data from the first 73 patients of this study were recently presented, showing no grade 3 or higher immune-related adverse events to date (56). Another study is exploring the potential of this strategy for the management of non-muscle invasive bladder cancer, using the combination of radiotherapy with Bacillus Calmette-Guerin (BCG) and durvalumab (ADAPT-bladder; NCT03317158).
Considerations Surrounding Combining Radiotherapy and Immunotherapy
Sequencing
The optimal timing and sequencing of radiotherapy and immunotherapy for maximum efficacy of combination therapy remain unknown, although these may vary depending on tumor histology and type of immunotherapy (13). Interestingly, in a post-hoc analysis of the PACIFIC trial, patients who received durvalumab within 14 days after completing chemoradiation had better progression free survival than those who received durvalumab after 14 days, suggesting that immunotherapy should be started soon after radiation (69). Similarly, in a retrospective review of 758 patients with a range of cancer diagnoses who received radiotherapy and immunotherapy (either anti-CTLA-4 or anti-PD-1/PD-L1), patients who received concurrent therapy had better overall survival. Moreover, of those who received concurrent therapy, patients who received induction immunotherapy starting more than 30 days before radiation had improved overall survival compared to those who started less than 30 days before radiation (70). These studies suggest that careful consideration needs to be given to timing and sequencing of radiotherapy and immunotherapy in the design of clinical trials.
Dose and Fractionation
The optimal radiation dosing and fractionation strategy to maximize immunogenicity remains controversial. Most lines of evidence suggest that higher doses of radiation (>6-8 Gy per fraction) are more immunogenic than typical doses used in conventional fractionation (1.8-2 Gy per day) (71–73). Moderately high doses of 8-12 Gy seem to optimally activate the type I interferon response via cGAS/STING, while very high doses (20-30 Gy in 1 fraction) result in a decline in radiation-induced STING activation, in part due to negative feedback inhibition by Trex1 exonuclease which reduces accumulation of cytoplasmic DNA (24). Ultimately, the various fractionation schemes incorporated into ongoing clinical trials will yield insights into the optimal radiation dosing and fractionation needed for the effective combination with immunotherapy.
Biomarkers of Efficacy and Toxicity
The efficacy of immunotherapy varies greatly across patients and cancer types, and biomarkers that can identify the tumors that would be most responsive to specific immunotherapies are an area of active investigation (74). Candidate biomarkers of efficacy including PD-L1 expression, mutational burden, neoantigens, tumor infiltrating lymphocytes, and radiographic characteristics are under active study (75–79). Whether these same biomarkers will also predict responses to the combination of radiotherapy and immunotherapy remains an open question that should be actively addressed in ongoing and planned clinical trials.
There is also a need for biomarkers that can predict the occurrence of severe toxicity after the combination of immunotherapy and radiotherapy (80). Immune stimulatory drugs can cause immune-related adverse events (IrAEs) including fatigue, rash, skin disorders, and GI issues (81). Several large cohort studies (e.g. NCT03984318) are seeking to discover the underlying mechanisms responsible for severe IrAEs and identify predictive biomarkers. Biomarker candidate for IrAE prediction currently under investigation include cytokines, immune-cell subsets, autoantibodies, human leukocyte antigen haplotype, and radiomic characterization (82). Other studies are investigating the reduction of immunotherapy-related side effects through the use immunosuppressive drugs such as rituximab (anti-CD20) and tocilizumab (anti-IL-6) (NCT04375228). Radiotherapy is associated with its own set of toxicities, but can also cause adverse events similar to IrAEs through non-tumor specific antigens released into the tissue microenvironment by irradiation, potentially priming auto-reactive T cells to attack normal tissue (83). Predictors of these and other adverse events related to the combination of immunotherapy and radiotherapy need further study.
Conclusion
A growing body of preclinical and clinical evidence indicates a potential synergy between radiotherapy and immunotherapy, lending support for the combination of these two treatment approaches. Unanswered questions remain regarding the optimal sequencing of treatment, dose fractionation, and biomarkers of response and toxicity. Within genitourinary cancers, multiple clinical studies are ongoing with early indications of both promising as well as negative results, suggesting that specific details regarding the protocol by which treatment is delivered may impact the overall success of the approach. These efforts are exemplified by the SWOG/NRG 1806 phase 3 study testing the addition of atezolizumab to chemoradiation in muscle-invasive bladder cancer. Should these initial trials show promise, confirmatory trials may be necessary given increased FDA scrutiny of immunotherapy in light of recent voluntary withdrawal of drugs that received accelerated approval in bladder cancer (84). Continued research efforts are needed to fully evaluate and optimize this promising combination of radiotherapy and immunotherapy.
Author Contributions
JU, EK, and DM acquired, analyzed, and interpreted the data. JU, EK, and DM drafted and revised the manuscript for intellectual content. All authors contributed to the article and approved the submitted version.
Funding
This work was funded in part by the Cygnus Montanus Foundation founded by the Svanberg family and the Massachusetts General Hospital.
Conflict of Interest
The authors declare that the research was conducted in the absence of any commercial or financial relationships that could be construed as a potential conflict of interest.
Acknowledgments
The authors acknowledge past and present members of the DM laboratory for useful discussions.
References
1. Siegel RL, Miller KD, Jemal A. Cancer Statistics, 2020. CA Cancer J Clin (2020) 70:7–30. doi: 10.3322/caac.21590
2. Hamdy FC, Donovan JL, Lane JA, Mason M, Metcalfe C, Holding P, et al. 10-Year Outcomes After Monitoring, Surgery, or Radiotherapy for Localized Prostate Cancer. N Engl J Med (2016) 375:1415–24. doi: 10.1056/NEJMoa1606220
3. Kulkarni GS, Hermanns T, Wei Y, Bhindi B, Satkunasivam R, Athanasopoulos P, et al. Propensity Score Analysis of Radical Cystectomy Versus Bladder-Sparing Trimodal Therapy in the Setting of a Multidisciplinary Bladder Cancer Clinic. J Clin Oncol (2017) 35:2299–305. doi: 10.1200/JCO.2016.69.2327
4. Correa RJM, Louie AV, Zaorsky NG, Lehrer EJ, Ellis R, Ponsky L, et al. The Emerging Role of Stereotactic Ablative Radiotherapy for Primary Renal Cell Carcinoma: A Systematic Review and Meta-Analysis. Eur Urol Focus (2019) 5:958–69. doi: 10.1016/j.euf.2019.06.002
5. Palma DA, Olson R, Harrow S, Gaede S, Louie AV, Haasbeek C, et al. Stereotactic Ablative Radiotherapy for the Comprehensive Treatment of Oligometastatic Cancers: Long-Term Results of the SABR-COMET Phase II Randomized Trial. J Clin Oncol (2020) 38:2830–8. doi: 10.1200/JCO.20.00818
6. Phillips R, Shi WY, Deek M, Radwan N, Lim SJ, Antonarakis ES, et al. Outcomes of Observation vs Stereotactic Ablative Radiation for Oligometastatic Prostate Cancer: The ORIOLE Phase 2 Randomized Clinical Trial. JAMA Oncol (2020) 6:650–9. doi: 10.1001/jamaoncol.2020.0147
7. Sharma P, Siddiqui BA, Anandhan S, Yadav SS, Subudhi SK, Gao J, et al. The Next Decade of Immune Checkpoint Therapy. Cancer Discov (2021) 11:838–57. doi: 10.1158/2159-8290.CD-20-1680
8. Han Y, Liu D, Li L. PD-1/PD-L1 Pathway: Current Researches in Cancer. Am J Cancer Res (2020) 10:727–42.
9. Brunet JF, Denizot F, Luciani MF, Roux-Dosseto M, Suzan M, Mattei MG, et al. A New Member of the Immunoglobulin Superfamily–CTLA-4. Nature (1987) 328:267–70. doi: 10.1038/328267a0
10. Cameron F, Whiteside G, Perry C. Ipilimumab: First Global Approval. Drugs (2011) 71:1093–104. doi: 10.2165/11594010-000000000-00000
11. Pardoll DM. The Blockade of Immune Checkpoints in Cancer Immunotherapy. Nat Rev Cancer (2012) 12:252–64. doi: 10.1038/nrc3239
12. Wang Y, Liu ZG, Yuan H, Deng W, Li J, Huang Y, et al. The Reciprocity Between Radiotherapy and Cancer Immunotherapy. Clin Cancer Res (2019) 25:1709–17. doi: 10.1158/1078-0432.CCR-18-2581
13. Wang Y, Deng W, Li N, Neri S, Sharma A, Jiang W, et al. Combining Immunotherapy and Radiotherapy for Cancer Treatment: Current Challenges and Future Directions. Front Pharmacol (2018) 9:185. doi: 10.3389/fphar.2018.00185
14. Kalbasi A, June CH, Haas N, Vapiwala N. Radiation and Immunotherapy: A Synergistic Combination. J Clin Invest (2013) 123:2756–63. doi: 10.1172/JCI69219
15. G A, Hall EJ. Radiobiology for the Radiologist. Philadelphia, PA: Wolters Kluwer Health/Lippincott Williams & Wilkins (2012).
16. Jiang W, Chan CK, Weissman IL, Kim BYS, Hahn SM. Immune Priming of the Tumor Microenvironment by Radiation. Trends Cancer (2016) 2:638–45. doi: 10.1016/j.trecan.2016.09.007
17. Yuan H, Jiang W, von Roemeling CA, Qie Y, Liu X, Chen Y, et al. Multivalent Bi-Specific Nanobioconjugate Engager for Targeted Cancer Immunotherapy. Nat Nanotechnol (2017) 12:763–9. doi: 10.1038/nnano.2017.69
18. Obeid M, Tesniere A, Ghiringhelli F, Fimia GM, Apetoh L, Perfettini JL, et al. Calreticulin Exposure Dictates the Immunogenicity of Cancer Cell Death. Nat Med (2007) 13:54–61. doi: 10.1038/nm1523
19. Vermeer DW, Spanos WC, Vermeer PD, Bruns AM, Lee KM, Lee JH. Radiation-Induced Loss of Cell Surface CD47 Enhances Immune-Mediated Clearance of Human Papillomavirus-Positive Cancer. Int J Cancer (2013) 133:120–9. doi: 10.1002/ijc.28015
20. Reits EA, Hodge JW, Herberts CA, Groothuis TA, Chakraborty M, Wansley EK, et al. Radiation Modulates the Peptide Repertoire, Enhances MHC Class I Expression, and Induces Successful Antitumor Immunotherapy. J Exp Med (2006) 203:1259–71. doi: 10.1084/jem.20052494
21. Marincola FM, Jaffee EM, Hicklin DJ, Ferrone S. Escape of Human Solid Tumors From T-Cell Recognition: Molecular Mechanisms and Functional Significance. Adv Immunol (2000) 74:181–273. doi: 10.1016/s0065-2776(08)60911-6
22. Werner LR, Kler JS, Gressett MM, Riegert M, Werner LK, Heinze CM, et al. Transcriptional-Mediated Effects of Radiation on the Expression of Immune Susceptibility Markers in Melanoma. Radiother Oncol (2017) 124:418–26. doi: 10.1016/j.radonc.2017.08.016
23. Harding SM, Benci JL, Irianto J, Discher DE, Minn AJ, Greenberg RA. Mitotic Progression Following DNA Damage Enables Pattern Recognition Within Micronuclei. Nature (2017) 548:466–70. doi: 10.1038/nature23470
24. Vanpouille-Box C, Alard A, Aryankalayil MJ, Sarfraz Y, Diamond JM, Schneider RJ, et al. DNA Exonuclease Trex1 Regulates Radiotherapy-Induced Tumour Immunogenicity. Nat Commun (2017) 8:15618. doi: 10.1038/ncomms15618
25. Watson RO, Manzanillo PS, Cox JS, Extracellular M. Tuberculosis DNA Targets Bacteria for Autophagy by Activating the Host DNA-sensing Pathway. Cell (2012) 150:803–15. doi: 10.1016/j.cell.2012.06.040
26. Deng L, Liang H, Xu M, Yang X, Burnette B, Arina A, et al. STING-Dependent Cytosolic DNA Sensing Promotes Radiation-Induced Type I Interferon-Dependent Antitumor Immunity in Immunogenic Tumors. Immunity (2014) 41:843–52. doi: 10.1016/j.immuni.2014.10.019
27. Mukherjee B, McEllin B, Camacho CV, Tomimatsu N, Sirasanagandala S, Nannepaga S, et al. EGFRvIII and DNA Double-Strand Break Repair: A Molecular Mechanism for Radioresistance in Glioblastoma. Cancer Res (2009) 69:4252–9. doi: 10.1158/0008-5472.CAN-08-4853
28. Deschavanne PJ, Fertil B. A Review of Human Cell Radiosensitivity In Vitro. Int J Radiat Oncol Biol Phys (1996) 34:251–66. doi: 10.1016/0360-3016(95)02029-2
29. Schad MD, Dutta SW, Muller DM, Wijesooriya K, Showalter TN. Radiation-Related Lymphopenia After Pelvic Nodal Irradiation for Prostate Cancer. Adv Radiat Oncol (2019) 4:323–30. doi: 10.1016/j.adro.2019.01.005
30. Ishikawa H, Ma Z, Barber GN. STING Regulates Intracellular DNA-Mediated, Type I Interferon-Dependent Innate Immunity. Nature (2009) 461:788–92. doi: 10.1038/nature08476
31. Wrzesinski SH, Wan YY, Flavell RA. Transforming Growth Factor-Beta and the Immune Response: Implications for Anticancer Therapy. Clin Cancer Res (2007) 13:5262–70. doi: 10.1158/1078-0432.CCR-07-1157
32. Yang L, Pang Y, Moses HL. TGF-beta and Immune Cells: An Important Regulatory Axis in the Tumor Microenvironment and Progression. Trends Immunol (2010) 31:220–7. doi: 10.1016/j.it.2010.04.002
33. Trapani JA. The Dual Adverse Effects of TGF-beta Secretion on Tumor Progression. Cancer Cell (2005) 8:349–50. doi: 10.1016/j.ccr.2005.10.018
34. Liang H, Deng L, Hou Y, Meng X, Huang X, Rao E, et al. Host STING-dependent MDSC Mobilization Drives Extrinsic Radiation Resistance. Nat Commun (2017) 8:1736. doi: 10.1038/s41467-017-01566-5
35. Nakamura T, Ushigome H. Myeloid-Derived Suppressor Cells as a Regulator of Immunity in Organ Transplantation. Int J Mol Sci (2018) 19:23–57. doi: 10.3390/ijms19082357
36. Sharabi AB, Nirschl CJ, Kochel CM, Nirschl TR, Francica BJ, Velarde E, et al. Stereotactic Radiation Therapy Augments Antigen-Specific PD-1-Mediated Antitumor Immune Responses Via Cross-Presentation of Tumor Antigen. Cancer Immunol Res (2015) 3:345–55. doi: 10.1158/2326-6066.CIR-14-0196
37. Kumar V, Patel S, Tcyganov E, Gabrilovich DI. The Nature of Myeloid-Derived Suppressor Cells in the Tumor Microenvironment. Trends Immunol (2016) 37:208–20. doi: 10.1016/j.it.2016.01.004
38. Dovedi SJ, Adlard AL, Lipowska-Bhalla G, McKenna C, Jones S, Cheadle EJ, et al. Acquired Resistance to Fractionated Radiotherapy can be Overcome by Concurrent PD-L1 Blockade. Cancer Res (2014) 74:5458–68. doi: 10.1158/0008-5472.CAN-14-1258
39. Wu CT, Chen WC, Chang YH, Lin WY, Chen MF. The Role of PD-L1 in the Radiation Response and Clinical Outcome for Bladder Cancer. Sci Rep (2016) 6:19740. doi: 10.1038/srep19740
40. Chemnitz JM, Parry RV, Nichols KE, June CH, Riley JL. SHP-1 and SHP-2 Associate With Immunoreceptor Tyrosine-Based Switch Motif of Programmed Death 1 Upon Primary Human T Cell Stimulation, But Only Receptor Ligation Prevents T Cell Activation. J Immunol (2004) 173:945–54. doi: 10.4049/jimmunol.173.2.945
41. Syn NL, Teng MWL, Mok TSK, Soo RA. De-Novo and Acquired Resistance to Immune Checkpoint Targeting. Lancet Oncol (2017) 18:e731–41. doi: 10.1016/S1470-2045(17)30607-1
42. Persa E, Balogh A, Safrany G, Lumniczky K. The Effect of Ionizing Radiation on Regulatory T Cells in Health and Disease. Cancer Lett (2015) 368:252–61. doi: 10.1016/j.canlet.2015.03.003
43. Hodi FS, Ballinger M, Lyons B, Soria JC, Nishino M, Tabernero J, et al. Immune-Modified Response Evaluation Criteria in Solid Tumors (imRECIST): Refining Guidelines to Assess the Clinical Benefit of Cancer Immunotherapy. J Clin Oncol (2018) 36:850–8. doi: 10.1200/JCO.2017.75.1644
44. Jagodinsky JC, Harari PM, Morris ZS. The Promise of Combining Radiation Therapy With Immunotherapy. Int J Radiat Oncol Biol Phys (2020) 108:6–16. doi: 10.1016/j.ijrobp.2020.04.023
45. Demaria S, Bhardwaj N, McBride WH, Formenti SC. Combining Radiotherapy and Immunotherapy: A Revived Partnership. Int J Radiat Oncol Biol Phys (2005) 63:655–66. doi: 10.1016/j.ijrobp.2005.06.032
46. Demaria S, Ng B, Devitt ML, Babb JS, Kawashima N, Liebes L, et al. Ionizing Radiation Inhibition of Distant Untreated Tumors (Abscopal Effect) is Immune Mediated. Int J Radiat Oncol Biol Phys (2004) 58:862–70. doi: 10.1016/j.ijrobp.2003.09.012
47. Dewan MZ, Galloway AE, Kawashima N, Dewyngaert JK, Babb JS, Formenti SC, et al. Fractionated But Not Single-Dose Radiotherapy Induces an Immune-Mediated Abscopal Effect When Combined With anti-CTLA-4 Antibody. Clin Cancer Res (2009) 15:5379–88. doi: 10.1158/1078-0432.CCR-09-0265
48. Antonia SJ, Villegas A, Daniel D, Vicente D, Murakami S, Hui R, et al. Durvalumab After Chemoradiotherapy in Stage III Non-Small-Cell Lung Cancer. N Engl J Med (2017) 377:1919–29. doi: 10.1056/NEJMoa1709937
49. Antonia SJ, Villegas A, Daniel D, Vicente D, Murakami S, Hui R, et al. Overall Survival With Durvalumab After Chemoradiotherapy in Stage III NSCLC. N Engl J Med (2018) 379:2342–50. doi: 10.1056/NEJMoa1809697
50. Shaverdian N, Lisberg AE, Bornazyan K, Veruttipong D, Goldman JW, Formenti SC, et al. Previous Radiotherapy and the Clinical Activity and Toxicity of Pembrolizumab in the Treatment of non-Small-Cell Lung Cancer: A Secondary Analysis of the KEYNOTE-001 Phase 1 Trial. Lancet Oncol (2017) 18:895–903. doi: 10.1016/S1470-2045(17)30380-7
51. Theelen W, Peulen HMU, Lalezari F, van der Noort V, de Vries JF, Aerts J, et al. Effect of Pembrolizumab After Stereotactic Body Radiotherapy vs Pembrolizumab Alone on Tumor Response in Patients With Advanced non-Small Cell Lung Cancer: Results of the PEMBRO-RT Phase 2 Randomized Clinical Trial. JAMA Oncol (2019) 5(9):1276–82. doi: 10.1001/jamaoncol.2019.1478
52. Twardowski P, Wong JYC, Pal SK, Maughan BL, Frankel PH, Franklin K, et al. Randomized Phase II Trial of Sipuleucel-T Immunotherapy Preceded by Sensitizing Radiation Therapy and Sipuleucel-T Alone in Patients With Metastatic Castrate Resistant Prostate Cancer. Cancer Treat Res Commun (2019) 19:100116. doi: 10.1016/j.ctarc.2018.100116
53. Supiot S, Libois V, Guimas V, Rio E, Rolland F, Bompas E, et al. Prostate Cancer With Oligometastatic Relapse: Combining Stereotactic Ablative Radiotherapy and Durvalumab, a Randomized Phase II Trial (POSTCARD - GETUG-P13). J Clin Oncol (2019) 37:TPS5088–TPS5088. doi: 10.1200/JCO.2019.37.15_suppl.TPS5088
54. Weickhardt AJ, Foroudi F, Sengupta S, Galletta L, Herschtal A, Grimison PS, et al. Pembrolizumab and Chemoradiotherapy for Muscle Invasive Bladder Cancer: The ANZUP 1502 PCR-MIB Trial. J Clin Oncol (2018) 36:TPS531–1. doi: 10.1200/JCO.2018.36.6_suppl.TPS531
55. Gupta S, Maughan BL, Dechet CB, Lowrance WT, Neil BO, Kokeny KE, et al. NEXT: A Phase II, Open-Label Study of Nivolumab Adjuvant to Chemoradiation in Patients (Pts) With Localized Muscle Invasive Bladder Cancer. J Clin Oncol (2020) 38:TPS605–5. doi: 10.1200/JCO.2020.38.6_suppl.TPS605
56. Singh P, Efstathiou J, Tangen C, Jhavar S, Hahn N, Costello B, et al. INTACT (S/N1806) Phase III Randomized Trial of Concurrent Chemoradiotherapy With or Without Atezolizumab in Localized Muscle-Invasive Bladder Cancer: Safety Update on First 73 Patients. J Clin Oncol (2021) 39(suppl 6):abstr 428. doi: 10.1200/JCO.2021.39.6_suppl.428
57. Cuellar MA, Medina A, Girones R, Valderrama BP, Font A, Juan-fita M, et al. Phase II Trial of Durvalumab Plus Tremelimumab With Concurrent Radiotherapy as Bladder-Sparing Therapy in Patients With Localized Muscle Invasive Bladder Cancer: A SOGUG Study. J Clin Oncol (2020) 38:TPS5097–TPS5097. doi: 10.1200/JCO.2020.38.15_suppl.TPS5097
58. Hannan R, Ishihara D, Louder K, Ahn C, Margulis V, Arriaga YE, et al. Phase II Trial of High-Dose Interleukin-2 (IL-2) and Stereotactic Radiation Therapy (SABR) for Metastatic Clear Cell Renal Cell Carcinoma (ccRCC): Interim Analysis. J Clin Oncol (2016) 34:532–2. doi: 10.1200/jco.2016.34.2_suppl.532
59. Hammers HJ, Vonmerveldt D, Ahn C, Nadal RM, Drake CG, Folkert MR, et al. Combination of Dual Immune Checkpoint Inhibition (ICI) With Stereotactic Radiation (SBRT) in Metastatic Renal Cell Carcinoma (mRCC) (RADVAX RCC). J Clin Oncol (2020) 38:614–4. doi: 10.1200/JCO.2020.38.6_suppl.614
60. Ridolfi L, De Rosa F, Granato AM, Pancisi E, Bulgarelli J, Romeo A, et al. Radiotherapy as an Immunological Booster in Patients With Metastatic Melanoma or Renal Cell Carcinoma Treated With High-Dose Interleukin-2: Interim Analysis Data. J Clin Oncol (2015) 33:e14007–7. doi: 10.1200/jco.2015.33.15_suppl.e14007
61. Masini C, Iotti C, De Giorgi U, Salvatore Bellia R, Buti S, Salaroli F, et al. Nivoluman (NIVO) in Combination With Stereotactic Body Radiotherapy (SBRT) in Pretreated Patients (Pts) With Metastatic Renal Cell Carcinoma (mRCC): First Results of Phase II NIVES Study. J Clin Oncol (2020) 38:613–3. doi: 10.1200/JCO.2020.38.6_suppl.613
62. Lalani A-KA, Swaminath A, Pond GR, Kapoor A, Chu W, Bramson JL, et al. Phase II Trial of Cytoreductive Stereotactic Hypofractionated Radiotherapy With Combination Ipilimumab/Nivolumab for Metastatic Kidney Cancer (CYTOSHRINK). J Clin Oncol (2020) 38:TPS761–1. doi: 10.1200/JCO.2020.38.6_suppl.TPS761
63. Kwon ED, Drake CG, Scher HI, Fizazi K, Bossi A, van den Eertwegh AJ, et al. Ipilimumab Versus Placebo After Radiotherapy in Patients With Metastatic Castration-Resistant Prostate Cancer That had Progressed After Docetaxel Chemotherapy (CA184-043): A Multicentre, Randomised, Double-Blind, Phase 3 Trial. Lancet Oncol (2014) 15:700–12. doi: 10.1016/S1470-2045(14)70189-5
64. Motzer RJ, Escudier B, McDermott DF, George S, Hammers HJ, Srinivas S, et al. Nivolumab Versus Everolimus in Advanced Renal-Cell Carcinoma. N Engl J Med (2015) 373:1803–13. doi: 10.1056/NEJMoa1510665
65. Motzer RJ, Tannir NM, McDermott DF, Aren Frontera O, Melichar B, Choueiri TK, et al. Nivolumab Plus Ipilimumab Versus Sunitinib in Advanced Renal-Cell Carcinoma. N Engl J Med (2018) 378:1277–90. doi: 10.1056/NEJMoa1712126
66. Flaig TW, Spiess PE, Agarwal N, Bangs R, Boorjian SA, Buyyounouski MK, et al. Nccn Guidelines Insights: Bladder Cancer, Version 5.2018. J Natl Compr Canc Netw (2018) 16:1041–53. doi: 10.6004/jnccn.2018.0072
67. Chang SS, Bochner BH, Chou R, Dreicer R, Kamat AM, Lerner SP, et al. Treatment of Non-Metastatic Muscle-Invasive Bladder Cancer: AUA/ASCO/ASTRO/SUO Guideline. J Urol (2017) 198:552–9. doi: 10.1016/j.juro.2017.04.086
68. Joshi M, Tuanquin L, Kaag M, Zakharia Y, Liao J, Merrill S, et al. Phase Ib Study of Concurrent Durvalumab and Radiation Therapy (DUART) Followed by Adjuvant Durvalumab in Patients With Urothelial Cancer of the Bladder: BTCRC-GU15-023 Study. J Clin Oncol (2018) 36:455–5. doi: 10.1200/JCO.2018.36.6_suppl.455
69. Faivre-Finn C, Spigel DR, Senan S, Langer CJ, Raben D, Perez B, et al. Efficacy and Safety Evaluation Based on Time From Completion of Radiotherapy to Randomization With Durvalumab or Placebo in Pts From PACIFIC. Ann Oncol (2018) 29(Suppl. 8):viii488. doi: 10.1093/annonc/mdy291
70. Samstein R, Rimner A, Barker CA, Yamada Y. Combined Immune Checkpoint Blockade and Radiation Therapy: Timing and Dose Fractionation Associated With Greatest Survival Duration Among Over 750 Treated Patients. Int J Radiat Oncol Biol Phys (2017) 99:S129–30. doi: 10.1016/j.ijrobp.2017.06.303
71. Brooks ED, Schoenhals JE, Tang C, Micevic G, Gomez DR, Chang JY, et al. Stereotactic Ablative Radiation Therapy Combined With Immunotherapy for Solid Tumors. Cancer J (2016) 22:257–66. doi: 10.1097/PPO.0000000000000210
72. Lugade AA, Moran JP, Gerber SA, Rose RC, Frelinger JG, Lord EM. Local Radiation Therapy of B16 Melanoma Tumors Increases the Generation of Tumor Antigen-Specific Effector Cells That Traffic to the Tumor. J Immunol (2005) 174:7516–23. doi: 10.4049/jimmunol.174.12.7516
73. Schaue D, Ratikan JA, Iwamoto KS, McBride WH. Maximizing Tumor Immunity With Fractionated Radiation. Int J Radiat Oncol Biol Phys (2012) 83:1306–10. doi: 10.1016/j.ijrobp.2011.09.049
74. Shum B, Larkin J, Turajlic S. Predictive Biomarkers for Response to Immune Checkpoint Inhibition. Semin Cancer Biol (2021) 30(2019):998–1004. doi: 10.1016/j.semcancer.2021.03.036
75. Topalian SL, Hodi FS, Brahmer JR, Gettinger SN, Smith DC, McDermott DF, et al. Safety, Activity, and Immune Correlates of anti-PD-1 Antibody in Cancer. N Engl J Med (2012) 366:2443–54. doi: 10.1056/NEJMoa1200690
76. Herbst RS, Soria JC, Kowanetz M, Fine GD, Hamid O, Gordon MS, et al. Predictive Correlates of Response to the anti-PD-L1 Antibody MPDL3280A in Cancer Patients. Nature (2014) 515:563–7. doi: 10.1038/nature14011
77. Schumacher TN, Schreiber RD. Neoantigens in Cancer Immunotherapy. Science (2015) 348:69–74. doi: 10.1126/science.aaa4971
78. Lee Y, Auh SL, Wang Y, Burnette B, Wang Y, Meng Y, et al. Therapeutic Effects of Ablative Radiation on Local Tumor Require CD8+ T Cells: Changing Strategies for Cancer Treatment. Blood (2009) 114:589–95. doi: 10.1182/blood-2009-02-206870
79. Trebeschi S, Drago SG, Birkbak NJ, Kurilova I, Calin AM, Delli Pizzi A, et al. Predicting Response to Cancer Immunotherapy Using Noninvasive Radiomic Biomarkers. Ann Oncol (2019) 30:998–1004. doi: 10.1093/annonc/mdz108
80. Kang JH, Bluestone JA, Young A. Predicting and Preventing Immune Checkpoint Inhibitor Toxicity: Targeting Cytokines. Trends Immunol (2021) 42:293–311. doi: 10.1016/j.it.2021.02.006
81. Kumar V, Chaudhary N, Garg M, Floudas CS, Soni P, Chandra AB. Current Diagnosis and Management of Immune Related Adverse Events (irAEs) Induced by Immune Checkpoint Inhibitor Therapy. Front Pharmacol (2017) 8:49. doi: 10.3389/fphar.2017.00049
82. von Itzstein MS, Khan S, Gerber DE. Investigational Biomarkers for Checkpoint Inhibitor Immune-Related Adverse Event Prediction and Diagnosis. Clin Chem (2020) 66:779–93. doi: 10.1093/clinchem/hvaa081
83. Tang C, Jiang W, Yap TA. Efficacy and Toxic Effects of Cancer Immunotherapy Combinations-A Double-Edged Sword. JAMA Oncol (2018) 4:1116–7. doi: 10.1001/jamaoncol.2017.4606
84. Federal Drug Administration. FDA In Brief: FDA Oncologic Drugs Advisory Committee to Review Status of Six Indications Granted Accelerated Approval (2021). Available at: https://www.fda.gov/news-events/fda-brief/fda-brief-fda-oncologic-drugs-advisory-committee-review-status-six-indications-granted-accelerated (Accessed April 7, 2021).
Keywords: immunotherapy, radiation therapy, renal cancer, bladder cancer, prostate cancer, genitourinary cancer (GU cancer), radiotherapy, immune checkpoint inhibitor
Citation: Ukleja J, Kusaka E and Miyamoto DT (2021) Immunotherapy Combined With Radiation Therapy for Genitourinary Malignancies. Front. Oncol. 11:663852. doi: 10.3389/fonc.2021.663852
Received: 03 February 2021; Accepted: 22 April 2021;
Published: 10 May 2021.
Edited by:
Daphne Haas-Kogan, Brigham and Women’s Hospital and Harvard Medical School, United StatesReviewed by:
Andrew Zhuang Wang, University of North Carolina at Chapel Hill, United StatesEllen Ackerstaff, Memorial Sloan Kettering Cancer Center, United States
Trevor Royce, University of North Carolina at Chapel Hill, United States
Copyright © 2021 Ukleja, Kusaka and Miyamoto. This is an open-access article distributed under the terms of the Creative Commons Attribution License (CC BY). The use, distribution or reproduction in other forums is permitted, provided the original author(s) and the copyright owner(s) are credited and that the original publication in this journal is cited, in accordance with accepted academic practice. No use, distribution or reproduction is permitted which does not comply with these terms.
*Correspondence: David T. Miyamoto, dmiyamoto@mgh.harvard.edu
†These authors have contributed equally to this work and share first authorship