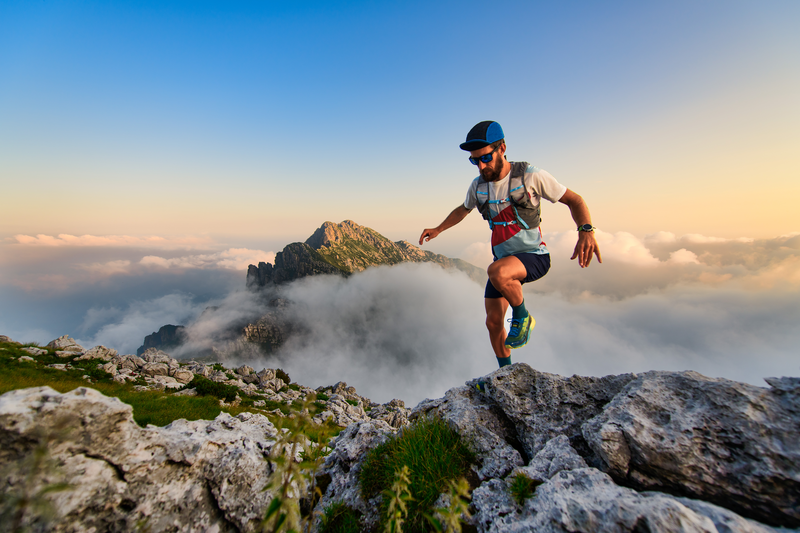
95% of researchers rate our articles as excellent or good
Learn more about the work of our research integrity team to safeguard the quality of each article we publish.
Find out more
ORIGINAL RESEARCH article
Front. Oncol. , 28 May 2021
Sec. Cancer Immunity and Immunotherapy
Volume 11 - 2021 | https://doi.org/10.3389/fonc.2021.663612
This article is part of the Research Topic Immunotherapy with Checkpoint Inhibitors for Non-small Cell Lung Cancer, Colon Cancer and Esophageal Cancer View all 48 articles
Background: In recent years, immune checkpoint inhibitors (ICIs) in combination with chemotherapy have increased survival in patients with advanced non-small cell lung cancer (NSCLC). Vascular endothelial growth factor (VEGF), which plays a key role in tumor angiogenesis, is an immunological modulator; therefore, it is expected that anti-VEGF therapy in combination with ICIs enhances the antitumor effect of ICIs. In the present study, we investigated the impact of VEGF inhibition on clinical outcomes of NSCLC patients, including the efficacy of ICI treatment.
Methods: A total of 105 patients with advanced NSCLC who had been treated with ICIs were retrospectively analyzed to examine the relationship between the history of treatment with anti-VEGF agents and the clinical outcomes with ICI monotherapy.
Results: Patients who had received anti-VEGF therapy prior to ICIs showed shortened progression-free survival of ICI treatment and a decreased overall response rate to ICI treatment. By contrast, anti-VEGF therapy after ICI treatment was associated with increased survival, especially in patients who had also received anti-VEGF therapy prior to ICI therapy.
Conclusions: These retrospective observations suggest that anti-VEGF therapy prior to ICIs might be a negative predictor of response to ICIs. The sequence of anti-VEGF therapy might play a role in its ability to predict survival in NSCLC patients. Further investigation is warranted to identify the role of VEGF inhibition in altering clinical outcomes after immunotherapy.
Lung cancer is the leading cause of cancer-related death worldwide (1). Novel strategies for treating advanced lung cancer have progressed in recent years. One strategy has been the successful treatment of non-small cell lung cancer (NSCLC) with angiogenesis inhibitors. In addition, cancer immunotherapies, including programmed cell death protein 1 (PD-1)/programmed death ligand 1 (PD-L1) and cytotoxic T lymphocyte-associated antigen 4 (CTLA-4) checkpoint inhibitors, are being developed as promising alternative strategies for treating patients with advanced NSCLC. Several phase III clinical trials have shown improved outcomes with these immune checkpoint inhibitors (ICIs), such as prolonged survival and a more durable treatment response (2–7). However, as with chemotherapy and molecular-targeted therapy, some patients show intrinsic resistance to ICIs, and a majority of patients treated with ICIs develop acquired resistance.
The tumor microenvironment (TME), which consists of blood vessels, stromal cells, fibroblasts, signaling molecules, immune cells, and extracellular matrix components, is controlled by extracellular signals released from tumors. Vascular endothelial growth factor (VEGF), which is produced by tumor cells and surrounding stromal cells, promotes angiogenesis by binding VEGF receptor (VEGFR) on vascular endothelial cells. VEGF is also a potential modulator of the innate immune response. VEGF/VEGFR signaling promotes evasion of the antitumor immune response through multiple mechanisms, including suppression of dendritic-cell maturation (8), reducing cytotoxic T lymphocyte activity via production of inflammatory cytokines and upregulation of immune checkpoint expression (9, 10), activation of regulatory T cells (Tregs) (11), and accumulation of myeloid-derived suppressor cells (MDSCs) (12). High levels of pretreatment serum VEGF are associated with poor response to immunotherapy in malignant melanoma (13). Further, in a preclinical model, VEGF/VEGFR blockade restores immunosuppressive alterations caused by VEGF production, and combination of VEGF/VEGFR blockade with immunotherapy improves the antitumor immune response (14).
Bevacizumab, which is a recombinant, humanized monoclonal antibody that binds all isoforms of VEGF-A, shows favorable outcomes in combination with chemotherapy in NSCLC patients (15). Additionally, a previous study demonstrated that combination of bevacizumab with the CTLA-4 inhibitor ipilimumab enhanced infiltration of CD8+ T-cells compared to ipilimumab monotherapy in malignant melanoma (16). In recent years, some clinical study demonstrated that combination of anti-VEGF agents and ICIs prolongs survival compared to anti-VEGF alone in multiple carcinomas such as renal cell carcinoma and hepatocellular carcinoma (17, 18). The IMpower150 trial demonstrated significant improvement in survival in non-squamous NSCLC patients receiving atezolizumab, bevacizumab, carboplatin, and paclitaxel compared to patients receiving bevacizumab, carboplatin, and paclitaxel (19). On the other hand, a previous study demonstrated that prior anti-VEGF therapy was a negative predictive factor for the treatment efficacy of ICIs in metastatic renal cell carcinoma (20). However, studies in NSCLC patients who had received immunotherapy indicated a survival benefit of ICIs following chemotherapy combined with an angiogenesis inhibitor (21–23). Therefore, the impact of anti-VEGF therapy on the efficacy of immunotherapy is not fully understood in NSCLC patients. In this study, we conducted a retrospective investigation focused on the impact of VEGF inhibition on clinical outcomes in patients with advanced NSCLC treated with ICIs.
We retrospectively analyzed the medical records of NSCLC patients who received ICIs from February 2016 to December 2017 at five institutions in Japan: the University Hospital Kyoto Prefectural University of Medicine (Kyoto, Japan), the Japanese Red Cross Kyoto Daiichi Hospital (Kyoto, Japan), the Japanese Red Cross Kyoto Daini Hospital (Kyoto, Japan), the Matsushita Memorial Hospital (Osaka, Japan), and the Uji-Tokushukai Medical Center (Kyoto, Japan). In total, 105 patients met the following inclusion criteria: 1) histologically or cytologically proven NSCLC; 2) age ≥ 20 years; 3) administered more than two cycles of anti-PD-1 antibodies (nivolumab or pembrolizumab); and 4) received systemic chemotherapy, including cytotoxic chemotherapy or molecular-targeted therapy, prior to ICI treatment. Patients were excluded if they met any of the following criteria: 1) patients who have uncontrolled serious infections, uncontrolled cardiovascular disease, and metabolic disorders, including diabetes mellitus; 2) patients who were pregnant or nursing; and 3) patients who were regarded as unsuitable for this study by the investigators.
The study was conducted in accordance with the Declaration of Helsinki. All patients provided written or electronic informed consent. The authors are accountable for all aspects of the work in ensuring that questions related to the accuracy or integrity of any part of the work are appropriately investigated and resolved. Each hospital study protocol was approved by the ethics committee of the respective hospital. We used the Response Evaluation Criteria in Solid Tumors version 1.1 for evaluation of treatment efficacy.
Survival curves were calculated according to the Kaplan-Meier method. Gehan-Breslow-Wilcoxon method was used to compare survival. Overall survival (OS) was defined as the interval from the initial day of treatment to death from any cause. Progression-free survival (PFS) was defined as the interval from the initial day of treatment to disease progression or death. Categorical variables, such as the overall response rate (ORR), were compared using Fisher’s exact test. Cox proportional hazards models were used for multivariate analysis of PFS and OS, and logistic regression models were used for multivariate analysis of the ORR. For all analyses, a p-value less than 0.05 indicated statistical significance.
Statistical analyses were performed using GraphPad Prism8 (GraphPad Software, San Diego, CA, USA) and EZR statistical software version 1.50 (Saitama Medical Center, Jichi Medical University, Saitama, Japan), which is a graphical user interface for R (The R Foundation for Statistical Computing, Vienna, Austria). More precisely, it is a modified version of R commander designed to add statistical functions frequently used in biostatistics.
The data cutoff date for the survival analysis was May 1, 2020. The median duration of follow-up for surviving participants was 36.8 months (range, 4.1-51.6 months). Of the 105 NSCLC patients treated with ICIs, 35 (33%) were treated with anti-VEGF agents before ICIs (pre-anti-VEGF) and 70 (67%) patients were not treated with anti-VEGF agents before ICIs (no pre-anti-VEGF) (Figure 1). The characteristics of the two groups are listed in Table 1. Non-squamous cell carcinoma was more common in the pre-anti-VEGF group than in the no pre-anti-VEGF group, and radiation therapy was less common in the pre-anti-VEGF group. Other factors, including the occurrence of immune related adverse events and peripheral blood findings, did not differ between the two groups (Table 1, Supplementary Table 1).
Figure 1 Flow diagram of patients included in the analysis. VEGF, vascular endothelial growth factor; ICI, immune checkpoint inhibitor.
To investigate prognostic factors related to clinical outcomes, we conducted univariate and multivariate analyses for ORR, PFS and OS. The ORR of ICI treatment was significantly lower in the pre-anti-VEGF group than that in the no pre-anti-VEGF group in univariate analysis (17% and 33%, respectively; p = 0.014) (Figure 2). Multivariate analysis showed that a treatment history of anti-VEGF agents was independently associated with a poor response to ICI treatment [OR: odds ratio 0.30 (95% CI: 0.09-0.96), p = 0.043] (Table 2). In the univariate analysis of PFS and OS, patients whose Eastern Cooperative Oncology Group-performance status (ECOG-PS) scores were ≥2 had significantly poorer PFS and OS than other patients (p = 0.039 and p < 0.001, respectively) (Supplementary Table 2). Multivariate analysis revealed that worse ECOG-PS and a treatment history of anti-VEGF agents were independently associated with poor PFS after immunotherapy [HR 2.26 (95% CI: 1.20-4.29), p = 0.012 and HR 1.83 (95% CI: 1.05-3.20), p = 0.033, respectively] (Table 2). By contrast, a treatment history of anti-VEGF agents was not significantly associated with OS [HR 1.47 (95% CI: 0.76-2.82), p = 0.249] (Table 2). Furthermore, propensity score matched analysis was performed to balance the characteristics between groups with or without anti-VEGF therapeutic history. After matching, the mean propensity scores for pre-anti-VEGF and no-anti-VEGF were 0.444 ± 0.162 and 0.444 ± 0.162, respectively (p = 1) (Supplemnetary Table 3). Pre-anti-VEGF group showed marginally shorten PFS of ICI treatment in the full cohort, however, this tendency was emphasized in the property score matched cohort (Figure 3).
Figure 2 ORR to ICIs according to anti-VEGF treatment prior to ICI treatment. ORR, overall response rate; ICI, immune checkpoint inhibitor; VEGF, vascular endothelial growth factor.
Figure 3 Progression-free survival ICIs according to anti-VEGF treatment prior to ICI treatment. (A) Kaplan-Meier analysis of PFS after ICI treatment according to history of treatment with anti-VEGF agents in the full cohort. (B) Kaplan-Meier analysis of PFS in the propensity score-matched cohort (1:1, n=28 /group). ICI, immune checkpoint inhibitor; VEGF, vascular endothelial growth factor; PFS, progression-free survival.
To further reveal the impact of VEGF inhibition on patient outcomes, we examined the effect of the sequence of anti-VEGF therapy related to ICI treatment. Among the 98 patients who discontinued ICI treatment, previous treatment with anti-VEGF agents (n = 34) showed a trend toward prolonged OS compared to no previous treatment with anti-VEGF agents (n = 64) [median OS 20.1 months (95% CI 12.7-26.6) and 11.0 months (95% CI 6.6-15.5), respectively; p = 0.216] and post-ICI survival time [HR: 0.68 (95% CI: 0.42-1.09), p = 0.027] (Figures 4A, B). Patients who received anti-VEGF agents after ICIs tended to have longer post-ICI survival than those who did not receive anti-VEGF therapy after ICIs [HR 0.66 (95% CI 0.34-1.29), p = 0.195] (Figure 4C). Furthermore, a therapeutic history of anti-VEGF therapy prior to ICIs was associated with a favorable prognosis after ICIs among patients who received anti-VEGF therapy after ICIs [HR: 0.57 (95% CI: 0.14-2.24), p = 0.044) (Figure 4D).
Figure 4 Impact of anti-VEGF therapy on survival when administered pre- or post-ICI therapy. (A) Swimmer plot for patients who discontinued ICIs (n = 98) categorized by whether they received anti-VEGF therapy prior to ICIs. (B) Kaplan-Meier analysis of survival from ICI cessation according to history of treatment with anti-VEGF agents. Gehan-Breslow-Wilcoxon method was used to compare survival. (C) Kaplan-Meier analysis of survival from ICI cessation in patients who received salvage chemotherapy after ICIs (n = 58) according to post-ICI anti-VEGF treatment. (D) Kaplan-Meier analysis of survival from ICI cessation in patients who received post-ICI anti-VEGF therapy (n = 16) according to therapeutic history of anti-VEGF agents prior to ICIs. Gehan-Breslow-Wilcoxon method was used to compare survival. VEGF, vascular endothelial growth factor; OS, overall survival; CI, confidence interval; ICI, immune checkpoint inhibitor; HR, hazard ratio; MST, median survival time.
This retrospective study revealed the impact of the sequence of anti-VEGF inhibition on clinical outcomes related to ICI treatment in NSCLC patients. Anti-VEGF therapy prior to ICIs was shown to be a negative predictor of response to ICI treatment. The sequence of anti-VEGF therapy might play a role in its ability to predict survival in NSCLC patients. Thus, it is important to establish a therapeutic strategy that maximizes the therapeutic effect of ICIs and anti-VEGF therapy.
Currently, immunotherapy is widely used in multiple cancers including lung cancer, and the multiple biomarkers related to the efficacy of ICI treatment have recently emerged, such as PD-L1 expression in tumor and/or immune cells and tumor mutation burden (5, 24, 25). Previous studies have reported that the profile of immune cells in the TME or circulating peripheral blood might be a predictor of the therapeutic effect of immunotherapy (26–30). Tumor-infiltrating CD8+T cells, which exert an effector function in the TME, are associated with favorable clinical outcomes in patients receiving immunotherapy, whereas accumulation of immunosuppressive cells, such as MDSCs and Tregs, which are major mediators of immune tolerance, correlates with poor prognosis (26–28). These immune-related cells are regulated by molecules secreted by TME components and negatively regulate the function of effector T-cells. They can also inhibit the differentiation and maturation of dendritic cells (29, 30).
VEGF, a molecule secreted by tumor and stromal cells, plays pivotal roles in the TME. The TME is characterized by hypoperfusion, hypoxia, acidity, and high interstitial fluid pressure through tumor vascularization, which can lead to tumor growth and drug resistance. Anti-VEGF agents reverse these changes; therefore, combination treatment with anti-VEGF agents can be expected to enhance the therapeutic effect of ICIs. Previous studies have identified mechanisms of intrinsic resistance to immunotherapy related to previous treatment with anti-angiogenic therapies (31–33). One such mechanism is that anti-VEGF therapy induces compensatory upregulation of VEGF-independent angiogenic factors, which contribute to the production of an immunosuppressive TME. VEGF/VEGFR2 is a major signaling axis driving tumor angiogenesis, and blockade of this pathway induces upregulation of VEGF-independent compensatory pro-angiogenic factors, including fibroblast growth factor (FGF) 2, platelet-derived growth factor, and angiopoietin-1 and 2 (31). In preclinical models, bone marrow-derived fibrocyte-like cells, which are induced by anti-VEGF therapy, contribute to resistance to anti-VEGF therapy by upregulating FGF2, and the activated FGF/FGF receptor pathway is associated with non-T-cell inflamed tumors that show resistance to immunotherapy (32, 33). These previous studies suggest that multiple pro-angiogenic factors might be upregulated after VEGF inhibition, leading to attenuation of the antitumor immune response following ICI treatment. Another potential mechanism is that VEGF/VEGFR2 signaling, which is suppressed by anti-VEGF therapy, is immediately reactivated after withdrawal of anti-VEGF agents and returns to baseline, thereby inducing rapid revascularization (34).
In this study, anti-VEGF treatment prior to ICI treatment was associated with poor response to ICIs; however, salvage chemotherapy with anti-VEGF agents after ICI treatment increased survival. These observations support this second hypothesis and suggest that blockade of VEGF/VEGFR signaling might be reversible and that it is essential for patient survival after ICIs and prior anti-VEGF therapy. Additionally, pharmacodynamic analysis revealed that anti-PD-1 antibody remained bound even 2 months after infusion (35). Therefore, previous ICI treatment may improve the effect of any subsequent therapy, including anti-VEGF therapy. Further investigation is warranted to determine the optimal treatment strategy and sequence for ICIs and anti-VEGF therapy.
This study has several limitations. First, this was a retrospective study, so the cohort had a limited sample size and may be subject to patient selection bias. Second, additional analyses using peripheral blood or tumor samples, such as changes in secreted angiogenic factors and phenotypes of immune systems, were not performed, and the precise mechanisms of resistance to ICI were not elucidated. Third, PD-L1 expression, which is a predictive biomarker of ICI response, was measured in only some cases. Finally, the study does not examine the current standard regimens for advanced NSCLC: platinum-based chemotherapy combined with ICIs and angiogenesis inhibitors. Optimal treatment strategies that maximize the therapeutic effect of ICIs and anti-VEGF therapies should be investigated in future studies.
In conclusion, our study demonstrated poorer response to immunotherapy in patients previously treated with anti-VEGF agents than in patients not previously treated with anti-VEGF agents. However, salvage chemotherapy with anti-VEGF agents after ICI failure was associated with prolonged survival. These results suggest that the interventional sequence and maintenance of VEGF inhibition may be important and contribute to clinical outcomes in patients with advanced NSCLC. Further investigation is needed to determine the optimal treatment strategy for immunotherapy and anti-VEGF therapy.
The original contributions presented in the study are included in the article/Supplementary Material. Further inquiries can be directed to the corresponding author.
The studies involving human participants were reviewed and approved by Kyoto Prefectural University of Medicine. The patients/participants provided their written informed consent to participate in this study.
Conception and design: KeT and TadY. Administrative support: TakY and KoT. Provision of study materials or patients: SS, TT, TakY, YC, YM, MI, YK, and JU. Collection and assembly of data: KeT, AO, NK, RT, and MT. Data analysis and interpretation: KeT and TadY. All authors contributed to the article and approved the submitted version.
This work was supported by research grants from JSPS KAKENHI [Grant Numbers 20K22820 (to KeT) and 19K08608 (to TadY)].
TadY received commercial research grants from Pfizer, Ono Pharmaceutical, Chugai Pharmaceutical, and Takeda Pharmaceutical Company Limited. JU received research grants from Eli Lilly Japan K.K., AstraZeneca K.K., and Boehringer Ingelheim Japan. KoT received research grants from Chugai-Roche and Ono Pharmaceutical and personal fees from AstraZeneca, Chugai-Roche, MSD-Merck, Eli Lilly, Boehringer Ingelheim, and Daiichi Sankyo.
The remaining authors declare that the research was conducted in the absence of any commercial or financial relationships that could be construed as a potential conflict of interest.
We would like to thank the patients, their families, and all investigators involved in this study. We would also like to thank Editage (www.editage.com) for English language editing.
The Supplementary Material for this article can be found online at: https://www.frontiersin.org/articles/10.3389/fonc.2021.663612/full#supplementary-material
PD-1, programmed cell death 1; PD-L1, programmed death ligand 1; CTLA-4, cytotoxic T lymphocyte-associated antigen 4; NSCLC, non-small cell lung cancer; ICI, immune checkpoint inhibitor; TME, tumor microenvironment; VEGF: vascular endothelial growth factor; VEGFR, vascular endothelial growth factor receptor; Treg, regulator T cell; MDSC, myeloid-derived suppressor cell; OS, overall survival; PFS, progression-free survival; ORR, overall response rate; ECOG-PS, Eastern Cooperative Oncology Group-performance status; CI, confidence interval; HR, hazard ratio; FGF, fibroblast growth factor.
1. Miller KD, Sauer AG, Ortiz AP, Fedewa SA, Pinheiro PS, Tortolero-Luna G, et al. Cancer Statistics for Hispanics/Latinos, 2018. CA Cancer J Clin (2018) 68:425–45. doi: 10.3322/caac.21494
2. Antonia SJ, Villegas A, Daniel D, Vicente D, Murakami S, Hui R, et al. Durvalumab After Chemoradiotherapy in Stage III non-Small-Cell Lung Cancer. N Engl J Med (2017) 377:1919–29. doi: 10.1056/NEJMoa1709937
3. Borghaei H, Paz-Ares L, Horn L, Spigel DR, Steins M, Ready NE, et al. Nivolumab Versus Docetaxel in Advanced Nonsquamous non-Small-Cell Lung Cancer. N Engl J Med (2015) 373:1627–39. doi: 10.1056/NEJMoa1507643
4. Brahmer J, Reckamp KL, Baas P, Crinò L, Eberhardt WEE, Poddubskaya E, et al. Nivolumab Versus Docetaxel in Advanced Squamous-Cell non-Small-Cell Lung Cancer. N Engl J Med (2015) 373:123–35. doi: 10.1056/NEJMoa1504627
5. Herbst RS, Baas P, Kim DW, Felip E, Pérez-Gracia JL, Han JY, et al. Pembrolizumab Versus Docetaxel for Previously Treated, PD-L1-positive, Advanced non-Small-Cell Lung Cancer (KEYNOTE-010): A Randomised Controlled Trial. Lancet (2016) 387:1540–50. doi: 10.1016/S0140-6736(15)01281-7
6. Reck M, Rodríguez-Abreu D, Robinson AG, Hui R, Csőszi T, Fülöp A, et al. Pembrolizumab Versus Chemotherapy for PD-L1-positive non-Small-Cell Lung Cancer. N Engl J Med (2016) 375:1823–33. doi: 10.1056/NEJMoa1606774
7. Rittmeyer A, Barlesi F, Waterkamp D, Park K, Ciardiello F, von Pawel J, et al. Atezolizumab Versus Docetaxel in Patients With Previously Treated non-Small-Cell Lung Cancer (OAK): A Phase 3, Open-Label, Multicentre Randomised Controlled Trial. Lancet (2017) 389:255–65. doi: 10.1016/S0140-6736(16)32517-X
8. Oyama T, Ran S, Ishida T, Nadaf S, Kerr L, Carbone DP, et al. Vascular Endothelial Growth Factor Affects Dendritic Cell Maturation Through the Inhibition of Nuclear Factor-Kappa B Activation in Hemopoietic Progenitor Cells. J Immunol (1998) 160:1224–32.
9. Martino EC, Misso G, Pastina P, Costantini S, Vanni F, Gandolfo C, et al. Immune-Modulating Effects of Bevacizumab in Metastatic non-Small-Cell Lung Cancer Patients. Cell Death Discov (2016) 2:16025. doi: 10.1038/cddiscovery.2016.25
10. Voron T, Colussi O, Marcheteau E, Pernot S, Nizard M, Pointet AL, et al. VEGF-a Modulates Expression of Inhibitory Checkpoints on CD8+ T Cells in Tumors. J Exp Med (2015) 212:139–48. doi: 10.1084/jem.20140559
11. Terme M, Pernot S, Marcheteau E, Sandoval F, Benhamouda N, Colussi O, et al. VEGFA-VEGFR Pathway Blockade Inhibits Tumor-Induced Regulatory T-cell Proliferation in Colorectal Cancer. Cancer Res (2013) 73:539–49. doi: 10.1158/0008-5472.CAN-12-2325
12. Huang Y, Goel S, Duda DG, Fukumura D, Jain RK. Vascular Normalization as an Emerging Strategy to Enhance Cancer Immunotherapy. Cancer Res (2013) 73:2943–8. doi: 10.1158/0008-5472.CAN-12-4354
13. Yuan J, Zhou J, Dong Z, Tandon S, Kuk D, Panageas KS, et al. Pretreatment Serum VEGF is Associated With Clinical Response and Overall Survival in Advanced Melanoma Patients Treated With Ipilimumab. Cancer Immunol Res (2014) 2:127–32. doi: 10.1158/2326-6066.CIR-13-0163
14. Courau T, Nehar-Belaid D, Florez L, Levacher B, Vazquez T, Brimaud F, et al. TGF-β and VEGF Cooperatively Control the Immunotolerant Tumor Environment and the Efficacy of Cancer Immunotherapies. JCI Insight (2016) 1:e85974. doi: 10.1172/jci.insight.85974
15. Sandler A, Gray R, Perry MC, Brahmer J, Schiller JH, Dowlati A, et al. Paclitaxel-Carboplatin Alone or With Bevacizumab for non-Small-Cell Lung Cancer. N Engl J Med (2006) 355:2542–50. doi: 10.1056/NEJMoa061884
16. Hodi FS, Lawrence D, Lezcano C, Wu X, Zhou J, Sasada T, et al. Bevacizumab Plus Ipilimumab in Patients With Metastatic Melanoma. Cancer Immunol Res (2014) 2:632–42. doi: 10.1158/2326-6066.CIR-14-0053
17. Finn RS, Qin S, Ikeda M, Galle PR, Ducreux M, Kim TY, et al. Atezolizumab plus Bevacizumab in Unresectable Hepatocellular Carcinoma. N Engl J Med (2020) 382:1894–905. doi: 10.1056/NEJMoa1915745
18. Choueiri TK, Powles T, Burotto M, Escudier B, Bourlon MT, Zurawski B, et al. Nivolumab plus Cabozantinib versus Sunitinib for Advanced Renal-Cell Carcinoma. N Engl J Med (2021) 384:829–41. doi: 10.1056/NEJMoa2026982
19. Socinski MA, Jotte RM, Cappuzzo F, Orlandi F, Stroyakovskiy D, Nogami N, et al. Atezolizumab for First-Line Treatment of Metastatic Nonsquamous NSCLC. N Engl J Med (2018) 378:2288–301. doi: 10.1056/NEJMoa1716948
20. Jeyakumar G, Kim S, Bumma N, Landry C, Silski C, Suisham S, et al. Neutrophil Lymphocyte Ratio and Duration of Prior Anti-Angiogenic Therapy as Biomarkers in Metastatic RCC Receiving Immune Checkpoint Inhibitor Therapy. J Immunother Cancer (2017) 5:82. doi: 10.1186/s40425-017-0287-5
21. Yoshimura A, Yamada T, Okuma Y, Kitadai R, Takeda T, Kanematsu T, et al. Retrospective Analysis of Docetaxel in Combination With Ramucirumab for Previously Treated non-Small Cell Lung Cancer Patients. Transl Lung Cancer Res (2019) 8:450–60. doi: 10.21037/tlcr.2019.08.07
22. Harada D, Takata K, Mori S, Kozuki T, Takechi Y, Moriki S, et al. Previous Immune Checkpoint Inhibitor Treatment to Increase the Efficacy of Docetaxel and Ramucirumab Combination Chemotherapy. Anticancer Res (2019) 39:4987–93. doi: 10.21873/anticanres.13688
23. Shiono A, Kaira K, Mouri A, Yamaguchi O, Hashimoto K, Uchida T, et al. Improved Efficacy of Ramucirumab Plus Docetaxel After Nivolumab Failure in Previously Treated non-Small Cell Lung Cancer Patients. Thorac Cancer (2019) 10:775–81. doi: 10.1111/1759-7714.12998
24. Le DT, Uram JN, Wang H, Bartlett BR, Kemberling H, Eyring AD, et al. PD-1 Blockade in Tumors With Mismatch-Repair Deficiency. N Engl J Med (2015) 372:2509–20. doi: 10.1056/NEJMoa1500596
25. Rizvi NA, Hellmann MD, Snyder A, Kvistborg P, Makarov V, Havel JJ, et al. Cancer Immunology. Mutational Landscape Determines Sensitivity to PD-1 Blockade in non-Small Cell Lung Cancer. Science (2015) 348:124–8. doi: 10.1126/science.aaa1348
26. Donnem T, Hald SM, Paulsen EE, Richardsen E, Al-Saad S, Kilvaer TK, et al. Stromal CD8+ T-Cell Density—a Promising Supplement to TNM Staging in non-Small Cell Lung Cancer. Clin Cancer Res (2015) 21:2635–43. doi: 10.1158/1078-0432.CCR-14-1905
27. Martens A, Wistuba-Hamprecht K, Foppen MG, Yuan J, Postow MA, Wong P, et al. Baseline Peripheral Blood Biomarkers Associated With Clinical Outcome of Advanced Melanoma Patients Treated With Ipilimumab. Clin Cancer Res (2016) 22:2908–18. doi: 10.1158/1078-0432.CCR-15-2412
28. Martens A, Wistuba-Hamprecht K, Yuan J, Postow MA, Wong P, Capone M, et al. Increases in Absolute Lymphocytes and Circulating CD4+ and CD8+ T Cells are Associated With Positive Clinical Outcome of Melanoma Patients Treated With Ipilimumab. Clin Cancer Res (2016) 22:4848–58. doi: 10.1158/1078-0432.CCR-16-0249
29. Jain RK. Normalization of Tumor Vasculature: An Emerging Concept in Antiangiogenic Therapy. Science (2005) 307:58–62. doi: 10.1126/science.1104819
30. Pilon-Thomas S, Kodumudi KN, El-Kenawi AE, Russell S, Weber AM, Luddy K, et al. Neutralization of Tumor Acidity Improves Antitumor Responses to Immunotherapy. Cancer Res (2016) 76:1381–90. doi: 10.1158/0008-5472.CAN-15-1743
31. Gacche RN. Compensatory Angiogenesis and Tumor Refractoriness. Oncogenesis (2015) 4:e153. doi: 10.1038/oncsis.2015.14
32. Mitsuhashi A, Goto H, Saijo A, Trung VT, Aono Y, Ogino H, et al. Fibrocyte-Like Cells Mediate Acquired Resistance to Anti-Angiogenic Therapy With Bevacizumab. Nat Commun (2015) 6:8792. doi: 10.1038/ncomms9792
33. Sweis RF, Spranger S, Bao R, Paner GP, Stadler WM, Steinberg G, et al. Molecular Drivers of the non-T-cell-inflamed Tumor Microenvironment in Urothelial Bladder Cancer. Cancer Immunol Res (2016) 4:563–8. doi: 10.1158/2326-6066.CIR-15-0274
34. Yang Y, Zhang Y, Iwamoto H, Hosaka K, Seki T, Andersson P, et al. Discontinuation of anti-VEGF Cancer Therapy Promotes Metastasis Through a Liver Revascularization Mechanism. Nat Commun (2016) 7:12680. doi: 10.1038/ncomms12680
35. Brahmer JR, Drake CG, Wollner I, Powderly JD, Picus J, Sharfman WH, et al. Phase I Study of Single-Agent Anti-Programmed Death-1 (MDX-1106) in Refractory Solid Tumors: Safety, Clinical Activity, Pharmacodynamics, and Immunologic Correlates. J Clin Oncol (2010) 28:3167–75. doi: 10.1200/JCO.2009.26.7609
Keywords: immune checkpoint inhibitors, immunotherapy, anti-VEGF therapy, non-small cell lung cancer, treatment response
Citation: Tanimura K, Yamada T, Omura A, Shiotsu S, Kataoka N, Takeda T, Taniguchi R, Yamada T, Takeuchi M, Chihara Y, Morimoto Y, Iwasaku M, Kaneko Y, Uchino J and Takayama K (2021) The Impact of VEGF Inhibition on Clinical Outcomes in Patients With Advanced Non-Small Cell Lung Cancer Treated With Immunotherapy: A Retrospective Cohort Study. Front. Oncol. 11:663612. doi: 10.3389/fonc.2021.663612
Received: 05 February 2021; Accepted: 16 April 2021;
Published: 28 May 2021.
Edited by:
Udo S. Gaipl, University Hospital Erlangen, GermanyReviewed by:
Jian-Guo Zhou, University of Erlangen Nuremberg, GermanyCopyright © 2021 Tanimura, Yamada, Omura, Shiotsu, Kataoka, Takeda, Taniguchi, Yamada, Takeuchi, Chihara, Morimoto, Iwasaku, Kaneko, Uchino and Takayama. This is an open-access article distributed under the terms of the Creative Commons Attribution License (CC BY). The use, distribution or reproduction in other forums is permitted, provided the original author(s) and the copyright owner(s) are credited and that the original publication in this journal is cited, in accordance with accepted academic practice. No use, distribution or reproduction is permitted which does not comply with these terms.
*Correspondence: Tadaaki Yamada, dGF5YW1hZGFAa290by5rcHUtbS5hYy5qcA==
Disclaimer: All claims expressed in this article are solely those of the authors and do not necessarily represent those of their affiliated organizations, or those of the publisher, the editors and the reviewers. Any product that may be evaluated in this article or claim that may be made by its manufacturer is not guaranteed or endorsed by the publisher.
Research integrity at Frontiers
Learn more about the work of our research integrity team to safeguard the quality of each article we publish.