- 1Cancer Center, Renmin Hospital of Wuhan University, Wuhan, China
- 2Department of Nutrition, Changzheng Hospital, Second Military Medical University, Shanghai, China
- 3Cancer Center, Daping Hospital, Army Medical University (Third Military Medical University), Chongqing, China
- 4Geneplus-Beijing Institute, Beijing, China
- 5Department of Thoracic Surgery, Daping Hospital, Army Medical University (Third Military Medical University), Chongqing, China
Although immune checkpoint inhibitors (ICIs) have shown remarkable benefit for treatment of advanced non-small lung cancer (NSCLC), only a minority of patients can achieve durable responses and the most patients produce an ultra-rapid progressive disease. Here, we collected the availably published datasets and mined the determinants of response to immunotherapy on pathway levels. One hundred six NSCLC patients treated with immunotherapy were combined from Rizvi et al. and Hellman et al. studies (whole exon sequencing). Two independent validation datasets consisted of the MSKCC cohort (targeted sequencing) and data by Anagnostou and colleagues (whole exon sequencing). The Cancer Genome Atlas (TCGA) somatic mutation and gene expression data were applied to explore the immunobiology features. In the first combined cohort, we detected NOTCH pathway altered in 71% patients with durable clinical benefit (DCB) while only 36% among no durable benefit (NDB) (p = 0.005). Compared to NDB group, co-occurrence of NOTCH and at least two DDR (co-DDR) pathway was discovered in DCB group and contributed to a prolonged progression-free survival (PFS) [22.1 vs 3.6 months, p < 0.0001, HR, 0.34, 95% confidence interval (CI), 0.2–0.59]. In two independent datasets, co-occurrence of NOTCH+/co-DDR+ was also validated to be a better immunotherapy efficacy [Cohort 2: 13 vs 6 months, p = 0.034, HR, 0.55, 95% CI, 0.31–0.96; Cohort 3: 21 vs 11 months, p = 0.067, HR, 0.45, 95% CI, 0.18–1.1]. By analyzing TCGA cohort, we found patients with coexisting NOTCH+/co-DDR+ pathway had a higher TMB, more infiltration of CD4+T cells. Overall, co-occurrence of NOTCH and co-DDR pathway reflect a better immunotherapy efficacy in advanced NSCLC. This genomic predictor show promise in stratifying patients that suit for immunotherapy for future clinical practice.
Introduction
Immune checkpoint inhibitors (ICIs) has shown remarkable benefit for treatment of advanced non-small lung cancer (NSCLC) (1, 2). Nevertheless, only a limited patient population can generate durable responses after immunotherapy, while the majority of patients undergo inferior survival (3, 4). Therefore, there is an urgent need to stratify the patients who will benefit from ICIs.
A plethora of studies have shown biomarkers for predicting response to immunotherapy. The most heavily studied biomarker was programmed death-ligand 1 (PD-L1) expression correlated to efficacious immunotherapy (5–8), but subsequent trials proven a sizeable proportion of patients still achieve durable responses with PD-L1 negative (2, 9). Furthermore, some genomic markers have been reported for predicting the response to ICI, including tumor mutation burden (TMB) (10, 11), tumor neoantigen burden (TNB) (12, 13), and DNA repair alterations (14–16). Meanwhile, several studies explored the alterations on pathway level that correlates to ICI. Zhang et al. found NOTCH signaling related to better ICI efficacy (17). Wang et al. revealed co-mutations in DNA damage response (DDR) pathways could serve as predictors of response to ICB (18).
Due to the interactions and dependencies among different pathways, we supposed single pathway was not high enough to reflect the response of ICI. Therefore, we focused on the co-occurrent pathways to predict the superior survival outcomes after immunotherapy. Availably published datasets were collected from four studies. The first cohort was combined by data from Rizvi et al. and Hellman et al., which was used to identify the co-occurrent pathways correlated to ICI (15, 19). We further validated the co-occurrent pathways correlated to better efficacy after immunotherapy in two independent datasets (data from Anagnostou et al. and the MSKCC cohort) (20, 21). Using the somatic mutation and gene expression data from TCGA, we explored the immunobiology features about the co-occurrent pathway.
Materials and Methods
Datasets Used
We collected four publicly available datasets treated with immunotherapy (Supplementary Table 1). Thirty-four NSCLC patients sequenced by WES were downloaded from Rizvi et al. Another 75 patients with WES data were derived from Hellman et al. studies. Data from the two studies were merged as the first combined cohort. After excluding three patients lack of efficacy information, data from 106 patients was obtained. The second cohort was from Anagnostou et al., including 89 NSCLC patients treated with ICIs. Two patients were excluded because of lacking definitive response evaluation. For the third cohort, MSKCC cohort was downloaded from cBioPortal (http://www.cbioportal.org/study/summary?id=tmb_mskcc_2018). We also acquired the genome mutation from 1026 NSCLC patients and transcriptome expression data from 59 advanced NSCLC patients in TCGA.
Pathway Alterations
Genes involved in 10 oncogenic signaling pathways and eight DDR pathways were listed in Supplementary Table 2. The 10 canonical oncogenic signaling pathways were downloaded from (22) and included: cell cycle, Hippo signaling, Myc signaling, Notch signaling, oxidative stress response/Nrf2, PI-3-Kinase signaling, receptor-tyrosine kinase (RTK)/RAS/MAP-Kinase signaling, TGFb signaling, p53 and b-catenin/Wnt signaling. Eight DDR pathways analyzed were: mismatch repair (MMR); base excision repair (BER); check point factors (CPF); Fanconi anemia (FA); homologous recombination repair (HRR); nucleotide excision repair (NER); non-homologous end-joining (NHEJ); and DNA translesion synthesis (TLS) (23). We defined pathway alteration as at least one gene in this pathway mutated. Co-DDR was defined as two or more DDR pathway altered. Co-occurring alteration of NOTCH and DDR pathway was referred as NOTCH+/co-DDR+.
Immune Infiltration Analysis
SsGSEA was utilized to calculate the enrichment scores (ES) of immune cell types in the tumor microenvironment (24). Gene signatures of 28 immune cell types were downloaded from previous study (25). Tumors were further subclassified into different immune groups using the Euclidean distance and “ward.D” clustering. The expression levels of genes were first z-score normalized across all patients. Then we calculated the mean z-scores for each group and ranked in descending order. Based on the pre-ranked GSEA method, for each immune cell signature, we defined the q-value <10% and NES >0 as the enrichment, while the q-value <10% and NES <0 as the depletion.
Statistical Analyses
We performed univariable and multivariable Cox regression analyses to identify potential predictors of survival. Survival curves were estimated with the Kaplan-Meier product-limit method and compared by log-rank test. Comparisons of TMB, TNB, and expression levels between different groups were used with the Wilcoxon rank-sum test or t test. Enrichment analysis of gene function was calculated by GSEA with Benjamini-Hochberg correction for multiple hypothesis testing (q < 0.05). All statistical analyses were performed in the R statistical environment version 3.6.2.
Results
Pathway Alterations in NSCLC Treated With Immunotherapy
We collected WES data from 106 NSCLC patients treated with immunotherapy derived from published data as the discovery cohort (15, 19). Of these patients, 51 (48.1%) achieved durable clinical benefit and 55 (51.9%) had no response to the immunotherapy. The clinical features and efficacy outcomes were summarized in Table 1. To unravel the determinants of response to immunotherapy on pathway levels, we mapped all mutated genes to 10 oncogenic signaling pathways and eight DDR pathways (Supplementary Table 2, Figure 1). A tumor sample was considered as altered in a given pathway if one or more genes in this pathway contained non-synonymous mutations. Data from 99 patients succeeded to map to pathways that were further analyzed. The most frequently altered pathway was RTK/RAS in both DCB and NDB group (80 vs 82%), followed by HIPPO pathway (65% in DCB vs 52% in NDB). Notably, 71% patients with DCB had alteration in NOTCH pathway while only 36% in NDB group (p = 0.005). This was consistent with previous study that NOTCH signaling correlated with better ICI efficacy (17). More DDR-related pathways were altered in DCB. Co-mutations in DDR pathways (hereafter referred as co-DDR) have been reported to a better clinical benefit to ICI by Wang et al. (18), we also detected a higher prevalence of co-DDR pathway alteration in DCB group (69.3 vs 30%, p = 0.0002). Taken together, alterations on pathway level can reflect the divergent response in immunotherapy.
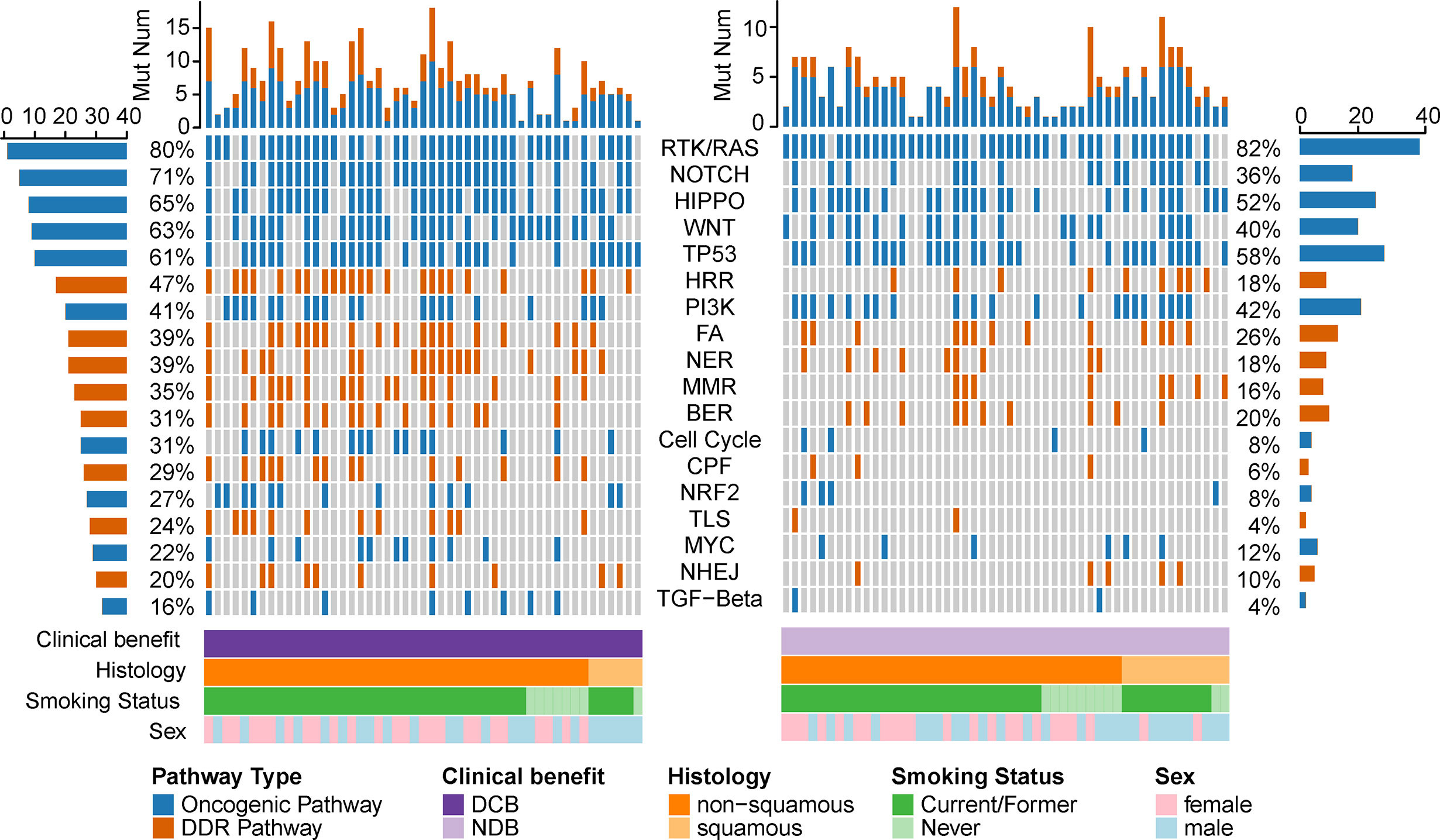
Figure 1 Pathway alterations in NSCLC with or without efficacious immunotherapy. Stacked plot showed the number of mutated pathway (histogram, top). Clinical characters are listed at the bottom of the figure. The prevalence of mutated pathways was calculated at left (in DCB group) and right (in NDB group). Orange represents the oncogenic pathway and blue stands for the DDR pathway.
Identification of NOTCH Co-occurring Pathways to Distinguish ICI Efficacy
Given that single pathway fails to adequately reveal the response of ICI, we applied a co-occurrence strategy to investigate how the NOTCH co-occurring pathways impacted the ICI efficacy. Comparing to NDB group, we identified the HIPPO and/or co-DDR pathway co-occurring with NOTCH pathway, which displayed a better clinical benefit (Figure 2A). After testing in univariate and multivariate cox regression for progressive free survival (PFS), only co-occurrence of NOTCH and co-DDR pathway proved to be an independently protective factor for PFS (Figure 2B, Supplementary Figure 1). Thus, 99 patients were further stratified into two groups according to the co-occurrent alteration of NOTCH and co-DDR pathways (hereafter referred as NOTCH+/co-DDR+). Altered genes for each pathway of NOCTH+/co-DDR+ were shown in Supplementary Figure 2. We defined the patients with NOTCH+/co-DDR+ as “GoodBenefit” (37 patients) and others were “BadBenefit” (62 patients). Patients grouped as “GoodBenefit” showed a longer PFS (22.1 vs 3.6 months, p < 0.0001, Figure 2C). Notably, co-occurrence of NOTCH+/co-DDR+ showed more prolonged PFS than single pathway (alteration either in NOTCH or co-DDR pathway) (Supplementary Figure 3A). In the NOTCH+/co-DDR+ group, we also detected higher tumor mutation burden (TMB, Figure 2D), higher tumor neoantigen burden (TNB, Figure 2E), more durable clinical benefit (p < 0.001, Figure 2F), and more improved objective response (p < 0.001, Figure 2G). These results were also observed when we stratified patients into the following three groups: CoPath (NOTCH+/co-DDR+) versus SinglePath (alterations in NOTCH or at least two DDR pathway) versus wild type (Supplementary Figures 3B–E). In summary, we identified NOTCH co-occurring with co-DDR pathways reflecting a better immunotherapy efficacy.
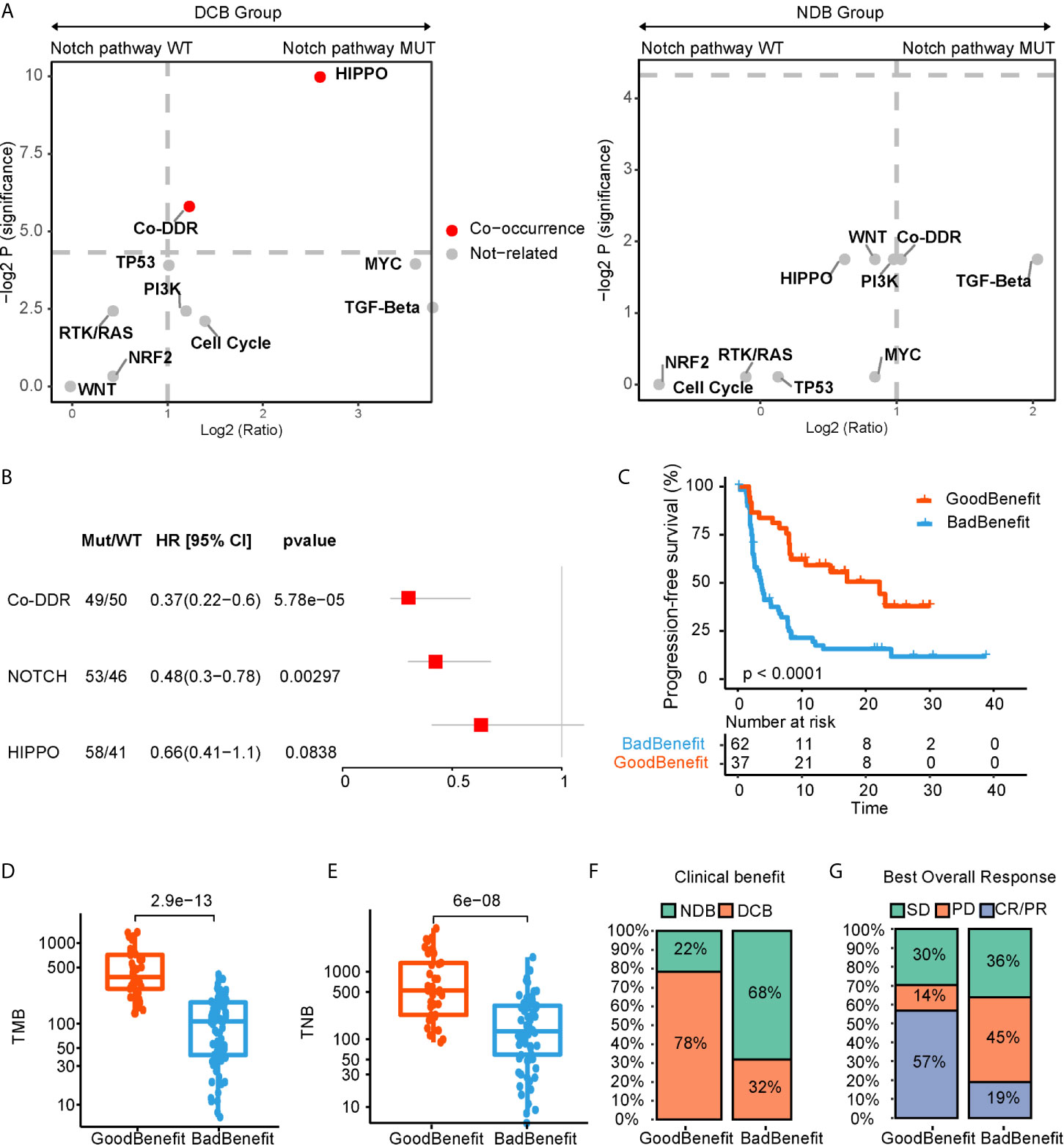
Figure 2 NOTCH co-occurring pathways that contributed to ICI efficacy. (A) log2 (odds ratio) and -log2 (p value) for enrichment of altered pathways co-occurred with and without NOTCH pathway. (B) Forest plot displaying univariate cox regression analyses for PFS of individually considered pathway. (C) Kaplan-Meier survival curves for PFS comparing “GoodBenefit” versus “BadBenefit.” (D, E) Boxplot of TMB (D) and TNB (E) in “GoodBenefit” versus “BadBenefit.” (F, G) Percentage of patients with clinical benefit evaluation (F) and objective response (G).
Independent Validation of the Model in Two Cohorts
To evaluate whether the co-occurrence of NOTCH+/co-DDR+ could serve as a potential predictor of immunotherapy efficacy, we crossed-validated our findings using two independent cohorts who received ICI therapies.
One validated dataset was from Anagnostou and colleagues (20), 87 NSCLC patients with definitive response evaluation were obtained. Using our stratification criteria, 26 patients were grouped into “GoodBenefit,” where 61 were classified as “BadBenefit.” Utilizing the univariate cox regression for PFS, “GoodBenefit” group showed a decreased risk of PFS (Figure 3A). Comparing to the group of “BadBenefit,” patients with co-existing pathways had longer PFS (13 vs 6 months, p = 0.034, HR, 0.55, 95% CI, 0.31–0.96) (Figure 3B). As expected, the TMB was significantly higher in “GoodBenefit” group than “BadBenefit” group (Figure 3C). When Comparing among the three groups (NOTCH+/co-DDR+ versus NOTCH or co-DDR occurrence versus Wildtype), co-occurrence of NOTCH/co-DDR also exhibited superior survival outcomes and the highest TMB (Supplementary Figures 4A, B).
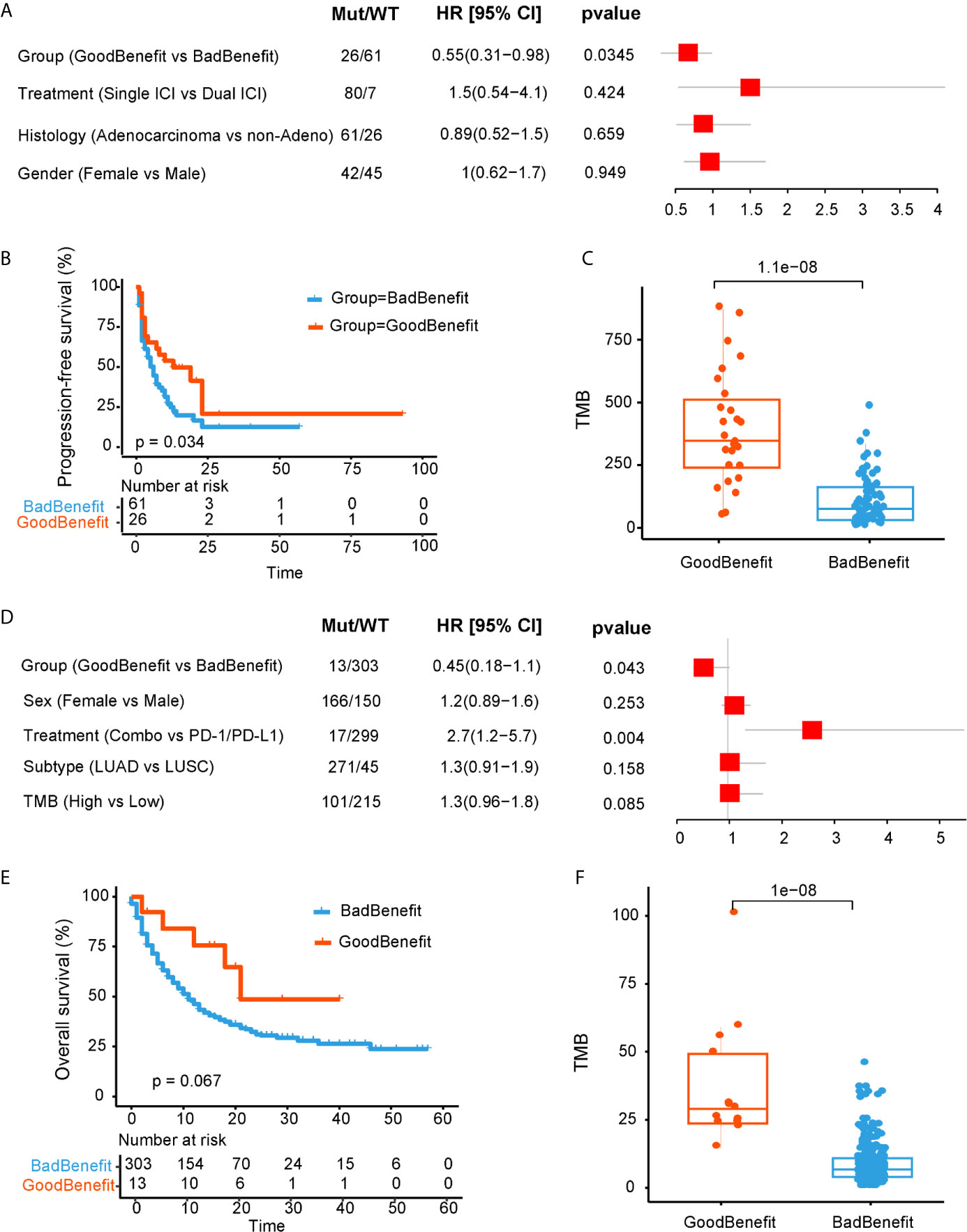
Figure 3 Validation of NOTCH/co-DDR co-occurring pathways to ICI efficacy in Anagnostou et al. cohort (A–C) and MSKCC cohort (D–F). (A) Forest plot displaying univariate cox regression analyses for PFS of individually considered pathway for Anagnostou et al. dataset. (B) Kaplan-Meier survival curves for PFS comparing “GoodBenefit” versus “BadBenefit.” (C) Boxplot of TMB in “GoodBenefit” versus “BadBenefit.” (D) Forest plot displaying univariate cox regression analyses for OS of individually considered pathway for MSKCC data. (E) Kaplan-Meier survival curves for OS comparing “GoodBenefit” versus “BadBenefit.” (F) Boxplot of TMB in “GoodBenefit” versus “BadBenefit.”.
For another independent cohort from MSKCC, 1,661 patients received immunotherapy by targeted sequencing, we retained the 316 NSCLC tissue [271 lung adenocarcinoma (LUAD) and 45 Lung squamous cell carcinoma (LUSC)] for further analyses (21). Co-occurrence of NOTCH+/co-DDR+ was found act as a protective factor (Figure 3D). Overall survival in co-occurrence group was prolonged, although did not reached the statistical significance (21 vs 11 months, p = 0.067, HR, 0.45, 95% CI, 0.181.1, Figure 3E). We speculated the limited genes from panel may underestimate the pathway alteration. In this cohort, targeted capture panel comprised of 341 and 410 genes, respectively, covering 10 oncogenic pathways and seven DDR pathways without TLS pathway. In addition, the TMB was significantly higher in “GoodBenefit” group (Figure 3F). When compared among the three groups, the co-occurrent group still performed improved OS prognostication and higher TMB (Supplementary Figures 4C, D).
Genomic Characteristics of NOTCH/co-DDR Co-occurrence in TCGA Cohort
We next explored the genomic characteristics in TCGA cohort according to our stratification criterion. One thousand twenty-six NSCLC WES data and clinical features were downloaded. All mutated genes were mapped to 18 canonical pathways. TMB in “GoodBenefit” group was significantly higher than “BadBenefit” group regardless of stage (Supplementary Figure 5A). Considering the predictor of NOTCH+/co-DDR+ was trained based on advanced NSCLC and without EGFR mutation. We filtered out the patients with early stage and EGFR alteration. Ultimately, 120 advanced NSCLC patients retained; 39.2% (47) patients were classified as “GoodBenefit” group while 60.8% (73) as “BadBenefit” group. This ratio of potential efficiency coincided with previous reports on NSCLC patients beneficial of ICIs delivery (26–29). In order to exclude NOTCH+/co-DDR+ as a potential prognostic factor, we performed the survival analyses in TCGA cohort treated with standard treatment. No significant difference of overall survival was detected between GoodBenefit versus BadBenefit group or among three groups (Figure 4A, Supplementary Figure 5B). This result demonstrated co-occurrent NOTCH+/co-DDR+ was not a prognostic factor per se, but can serve as predictor in condition of immunotherapy. Recent studies have shown the TMB was a predictor of the pathological response to immune checkpoint inhibitors treatment in advanced lung cancer patients, we next checked the TMB distribution in our groups. Comparing to wildtype, higher TMB was observed in “GoodBenefit” group (Figure 4B). In details, the TMB was significantly higher in co-occurrence group than those harbored single pathway (either NOCTH or co-DDR alteration) and wildtype (neither NOTCH nor co-DDR alteration) (Supplementary Figure 5C).
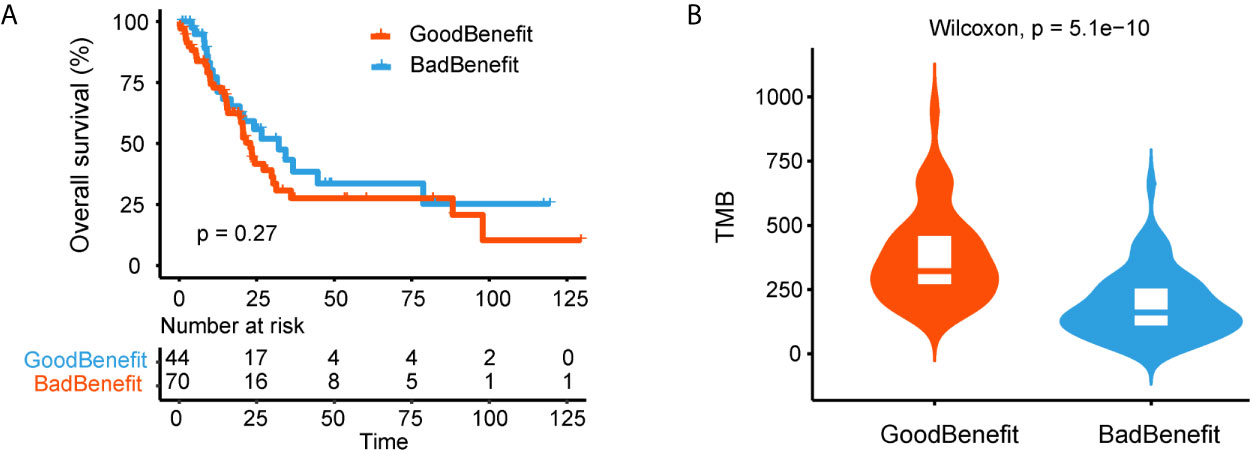
Figure 4 Genomic characteristics of NOTCH/co-DDR co-occurring pathways in TCGA cohort. (A) Kaplan-Meier survival curves for OS comparing “GoodBenefit” versus “BadBenefit.” (B) Violin plot of TMB between “GoodBenefit” versus “BadBenefit.”.
Immunobiology Features of NOTCH/co-DDR Co-occurrence
To further explore the immunobiology features of NOTCH+/co-DDR+ co-occurrence, we obtained 59 of 120 patients from TCGA whose RNA-seq data were available. The single sample gene set enrichment analysis (ssGSEA) method was employed to deconvolute the relative abundance of each immune cell type. By unsupervised clustering, we classified the 59 NSCLC into three clusters [immune-high (n = 27), immune-intermediate (n = 15), and immune-low (n = 17), Figure 5A]. Patients with NOTCH+/co-DDR+ co-occurrence had no preference to specific immune group (immune-high 14/27 vs immune-intermediate 3/15 vs immune-low 6/17, p = 0.12). Of note, CD4+ T cell were enriched in NOTCH+/co-DDR+ group (Figure 5B). We also found Interleukin 4 (IL-4), a quintessential T helper type 2 (Th2) cytokine produced by CD4+ T cells, expressed lower in “GoodBenefit” group (Figure 5C). TNFRSF18 was up-regulated in “GoodBenefit” group (Figure 5C), playing a role in promoting T effector cell activity by inducing proliferation and supporting survival in T cells, while also suppressing Treg activity (30). Another Tumor necrosis factor superfamily, TNFSF15 was downregulated in “GoodBenefit” group. TNFSF15 has been reported being an inhibitor of endothelial cell growth (31). Functional enrichment analyses revealed “GoodBenefit” group up-regulated growth factor receptor pathway, down-regulated DNA repair pathways and immune response pathways, this tumor context may contribute to the clinical benefit of ICI (Supplementary Figure 6).
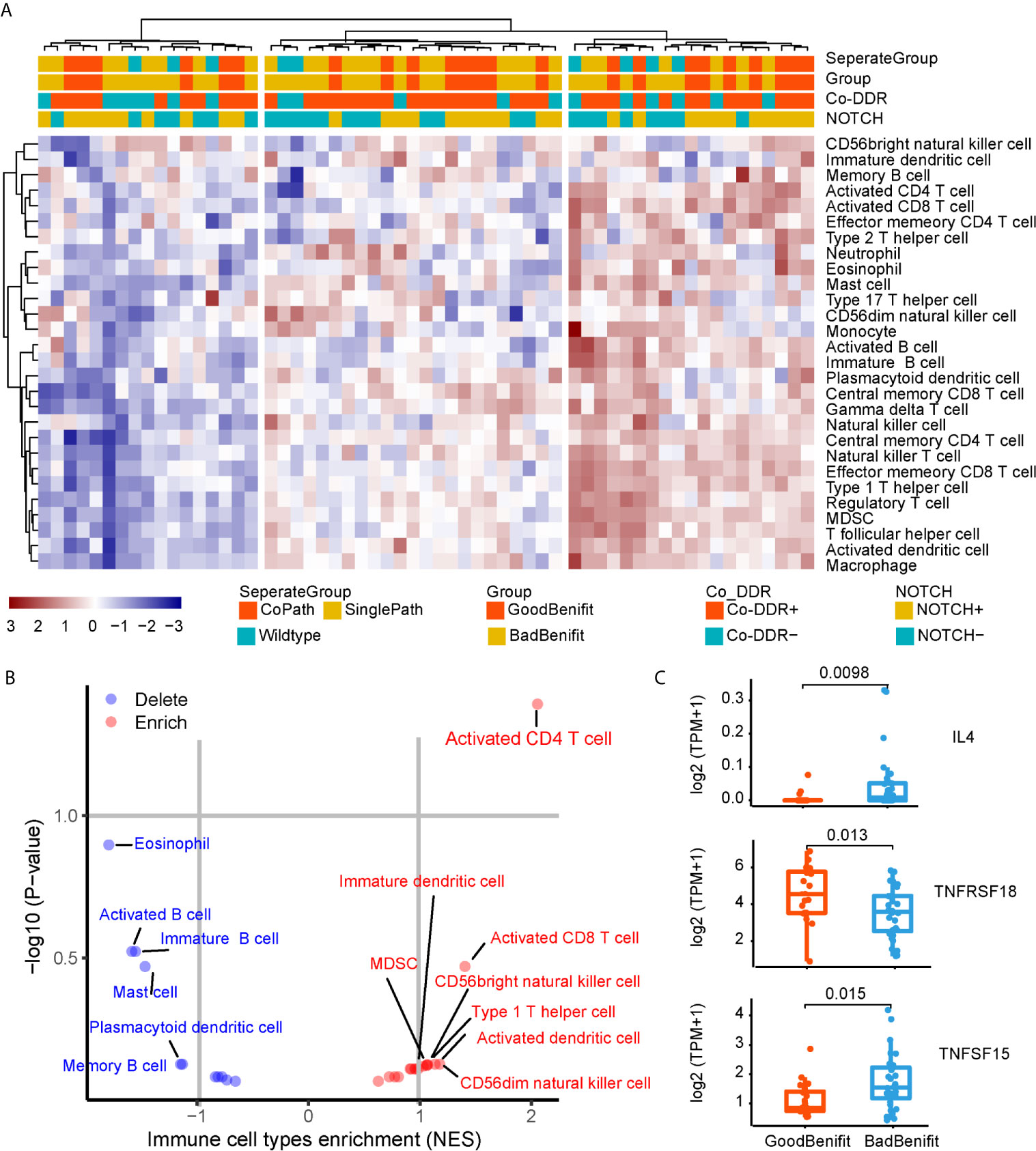
Figure 5 Immunobiology features of NOTCH/co-DDR co-occurrence. (A) Heatmap of immune infiltration in each patient. (B) Volcano plots for the enrichment (red) and depletion (blue) of immune cell types for GoodBenefit and BadBenefit. The expression levels of genes were first z-score normalized across all patients. Then we calculated the mean z-scores for each group and ranked in descending order. Based on the pre-ranked GSEA method, for each immune cell signature, we defined the q-value <10% and NES >0 as the enrichment, while the q-value <10% and NES <0 as the depletion. Immune cells with absolute NES greater than 1 were shown. (C) Normalized express of immune-related genes between Goodbenefit versus Badbenefit.
Discussion
In this study, we demonstrated the co-occurrence of NOTCH and co-DDR pathways can predict the superior survival outcomes after immunotherapy in advanced NSCLC. This phenomenon was first identified in one combined cohort and validated across another two independent cohorts. All the data we analyzed was performed WES, except one validated cohort from MSKCC (targeted sequencing). Using WES data was an advantage because it can cover enough genes of the pathway, overcoming the deficiency of targeted sequencing. To the best of our knowledge, this is the first report focusing on co-occurrence event on pathway level to explore the determinants of response to ICIs.
To explore whether there were dominant gene mutations in the co-occurring pathway, we depicted the co-occurring event on gene level involved in NOTCH and DDR pathways (Supplementary Figure 1). It can be observed that more alterations occurred in DCB group than NDB group. However, the overall mutation incidence on gene level was quite low. The most frequently mutated genes in NOTCH pathway were NOTCH1 (7%), NOTCH2 (7%), and JAG2 (7%). Meanwhile, low mutation rates of genes were observed in DDR pathway. Taken together, our data suggested that different genes converging to the co-occurring pathways effect the immunotherapy effect, rather than the specific gene of co-occurrence drives.
Although a single pathway of NOTCH or co-DDR has been reported as a predictor to ICI, we revealed co-occurrent NOTCH+/co-DDR+ pathway preferred in DCB and was more predictive to patients beneficial of ICIs delivery. In the first combined cohort, patients carrying co-mutations in NOTCH and co-DDR pathways had significantly longer PFS as compared to the SinglePath (alterations in NOTCH or at least two DDR pathway) or wild type, and the TMB was gradually decreasing. As for the validation dataset of cohort 2, we also observed the longer PFS in CoPath group than SinglePath, but there was no significant different in SinglePath versus Wild type. Thus, we speculated alteration in single pathway of NOTCH or co-DDR was incomplete correlate of immunotherapy.
Patients carrying alterations of NOTCH+/co-DDR+ pathway associated with increased TMB, TNB, and more infiltration of CD4+ T cell. Previous study has revealed that alterations in co-DDR pathway appeared to higher TMB and TNB (18). Since a higher mutation and neoantigen load could induce T cell-mediated antitumor response (32), we speculated that alterations in co-DDR contributed to more mutation and neoepitope, and further increased the likelihood of recognition by T cell. NOTCH signaling pathway has been reported as a pivotal role in regulating T cell modulation, differentiation, and activation (33, 34). In addition, previous study also reported NOTCH can control the fate of various T cell types, including Th1, Th2, and the regulatory T cells (35). In our result, we found co-occurrence of NOTCH/co-DDR tended to infiltrate more CD4+ T cell, but down-regulated the cytokine of Th2, suggesting more CD4 T cell that not secrete Th2 exist in NOTCH/co-DDR group. Moreover, recent studies have shown that NOTCH signaling is required for optimal T-cell-mediated anti-tumor immunity (36). Collectively, one potential explanation may be alteration of co-DDR pathway cause more mutations producing immunogenic neoantigens that are recognized and targeted by T cells and NOTCH pathway mediate more infiltration of CD4+ T cells and enhance effector T-cell activity. However, the underlying mechanism of co-ordination between NOTCH and co-DDR pathways and how these pathways shape together the microenvironment suit for immunotherapy should be further investigated in the future. Expanded data and more experiments are warranted to reveal the mechanism on how these pathways cross-talk to determine a better response to immunotherapy.
In conclusion, preliminary data from three cohorts showed evidence that co-mutations in NOTCH and co-DDR pathways indicated better immunotherapy efficacy. This genomic marker provided a new dimension to predict the superior survival outcomes in response to immunotherapy.
Data Availability Statement
The original contributions presented in the study are included in the article/Supplementary Material. Further inquiries can be directed to the corresponding authors.
Author Contributions
CC, CS, and ZZ conceived and designed the present study. ZZ, YYG, XS, and JB contributed equally to this work. ZZ and CC wrote the manuscript. ZZ, YYG, and XS collected and interpreted data. JB and WG performed the data analysis. JM, JL, and XX gave material support. JH, BZ, and YFG helped in improving the language and correcting grammar mistakes. All authors contributed to the article and approved the submitted version.
Funding
China Postdoctoral Science Foundation funded project (Grant #2014T70977). Hubei Province National Natural Science Foundation of China (Grant #2018CFB733).
Conflict of Interest
The authors declare that the research was conducted in the absence of any commercial or financial relationships that could be construed as a potential conflict of interest.
Supplementary Material
The Supplementary Material for this article can be found online at: https://www.frontiersin.org/articles/10.3389/fonc.2021.659321/full#supplementary-material
References
1. Reck M, Rodríguez-Abreu D, Robinson AG, Hui R, Csőszi T, Fülöp A, et al. Pembrolizumab Versus Chemotherapy for Pd-L1–Positive Non–Small-Cell Lung Cancer. N Engl J Med (2016) 375:1823–33. doi: 10.1056/NEJMoa1606774
2. Socinski MA, Jotte RM, Federico C, Francisco O, Daniil S, Naoyuki N, et al. Atezolizumab for First-Line Treatment of Metastatic Nonsquamous Nsclc. N Engl J Med (2018) 378:Nejmoa1716948–1716948. doi: 10.1056/NEJMoa1716948
3. Camidge DR, Doebele RC, Kerr KM. Comparing and Contrasting Predictive Biomarkers for Immunotherapy and Targeted Therapy of Nsclc. Nat Rev Clin Oncol (2019) 16:341–55. doi: 10.1038/s41571-019-0173-9
4. Gibney GT, Weiner LM, Atkins MB. Predictive Biomarkers for Checkpoint Inhibitor-Based Immunotherapy. Lancet Oncol (2016) 17:E542–542e551. doi: 10.1016/S1470-2045(16)30406-5
5. Garon EB, Rizvi NA, Hui R, Leighl N, Gandhi L. Pembrolizumab for the Treatment of Non–Small-Cell Lung Cancer. N Engl J Med (2015) 372:2018–28. doi: 10.1056/NEJMoa1501824
6. Chae YK, Pan A, Davis AA, Raparia K, Mohindra NA, Matsangou M, et al. Biomarkers for Pd-1/Pd-L1 Blockade Therapy In Non-Small-Cell Lung Cancer: Is Pd-L1 Expression a Good Marker for Patient Selection. Clin Lung Cancer (2016) 17:350–61. doi: 10.1016/J.Cllc.2016.03.011
7. Tumeh PC, Harview CL, Yearley JH, Shintaku IP, Taylor EJ, Robert L, et al. Pd-1 Blockade Induces Responses by Inhibiting Adaptive Immune Resistance. Nature (2014) 515:568–71. doi: 10.1038/Nature13954
8. Anagnostou V, Forde PM, White JR, Niknafs N, Hruban C, Naidoo J, et al. Dynamics of Tumor and Immune Responses During Immune Checkpoint Blockade in Non-Small Cell Lung Cancer. Cancer Res (2019) 79:1214–25. doi: 10.1158/0008-5472.Can-18-1127
9. Motzer RJ, Rini BI, Mcdermott DF, Redman BG, Kuzel TM, Harrison MR, et al. Nivolumab for Metastatic Renal Cell Carcinoma: Results of a Randomized Phase Ii Trial. J Clin Oncol (2014) 33:1430–7. doi: 10.1200/JCO.2014.59.0703
10. Hellmann MD, Callahan MK, Awad MM, Calvo E, Ascierto PA, Atmaca A, et al. Tumor Mutational Burden and Efficacy of Nivolumab Monotherapy and in Combination With Ipilimumab in Small-Cell Lung Cancer. Cancer Cell (2018) 33:853–61.e4. doi: 10.1016/j.ccell.2019.01.011
11. Carbone DP, Reck M, Paz-Ares L, Creelan B, Horn L, Steins M, et al. First-Line Nivolumab in Stage Iv or Recurrent Non-Small-Cell Lung Cancer. N Engl J Med (2017) 376:2415–26. doi: 10.1056/Nejmoa1613493
12. Van Allen EM, Miao D, Schilling B, Shukla SA, Blank C, Zimmer L, et al. Genomic Correlates of Response to Ctla-4 Blockade in Metastatic Melanoma. Science (2015) 350:207–11. doi: 10.1126/science.aad0095
13. Gubin MM, Zhang X, Schuster H, Caron E, Ward JP, Noguchi T, et al. Checkpoint Blockade Cancer Immunotherapy Targets Tumour-Specific Mutant Antigens. Nature (2014) 515:577–81. doi: 10.1038/Nature13988
15. Rizvi NA, Hellmann MD, Snyder A, Kvistborg P, Makarov V, Havel JJ, et al. Mutational Landscape Determines Sensitivity to Pd-1 Blockade in Non–Small Cell Lung Cancer. Science (2015) 348:124–8. doi: 10.1126/science.aaa1348
16. Bhangoo MS, Boasberg P, Mehta P, Elvin JA, Ali SM, Wu W, et al. Tumor Mutational Burden Guides Therapy in a Treatment Refractory Pole-Mutant Uterine Carcinosarcoma. Oncologist (2018) 23:518. doi: 10.1634/theoncologist.2017-0342
17. Zhang K, Hong X, Song Z, Xu Y, Liu L. IIdentification of Deleterious NOTCH Mutation as Novel Predictor to Efficacious Immunotherapy in NSCLC. Clin Cancer Res (2020) 26:3649–661. doi: 10.1158/1078-0432.CCR-19-3976
18. Wang Z, Zhao J, Wang G, Zhang F, Zhang Z, Zhang F, et al. Comutations in Dna Damage Response Pathways Serve as Potential Biomarkers for Immune Checkpoint Blockade. Cancer Res (2018) 78:6486–96. doi: 10.1158/0008-5472.Can-18-1814
19. Hellmann MD, Nathanson T, Rizvi H, Creelan BC, Sanchez-Vega F, Ahuja A, et al. Genomic Features of Response to Combination Immunotherapy in Patients With Advanced Non-Small-Cell Lung Cancer. Cancer Cell (2018) 33:843–52.e4. doi: 10.1016/j.ccell.2018.03.018
20. Anagnostou V, Niknafs N, Marrone K, Bruhm DC, White JR, Naidoo J, et al. Multimodal Genomic Features Predict Outcome of Immune Checkpoint Blockade in Non-Small-Cell Lung Cancer. Nat Cancer (2020) 1:99–111. doi: 10.1038/s43018-019-0008-8
21. Samstein RM, Lee C, Shoushtari AN, Hellmann MD, Shen R, Janjigian YY, et al. Tumor Mutational Load Predicts Survival After Immunotherapy Across Multiple Cancer Types. Nat Genet (2019) 51:202–6. doi: 10.1038/s41588-018-0312-8
22. Sanchez-Vega F, Mina M, Armenia J, Chatila WK, Luna A, La KC, et al. Oncogenic Signaling Pathways in the Cancer Genome Atlas. Cell (2018) 173:321–37.E10. doi: 10.1016/J.Cell.2018.03.035
23. Scarbrough PM, Weber RP, Iversen ES, Brhane Y, Amos CI, Kraft P, et al. a Cross-Cancer Genetic Association Analysis of the Dna Repair and Dna Damage Signaling Pathways for Lung, Ovary, Prostate, Breast, and Colorectal Cancer. Cancer Epidemiol Biomarkers Prev (2016) 25:193–200. doi: 10.1158/1055-9965.EPI-15-0649
24. Hnzelmann S, Castelo R, Guinney J. Gsva: Gene Set Variation Analysis for Microarray and Rna-Seq Data. BMC Bioinformatics (2013) 14:7–7. doi: 10.1186/1471-2105-14-7
25. Charoentong P, Finotello F, Angelova M, Mayer C, Efremova M, Rieder D, et al. Pan-Cancer Immunogenomic Analyses Reveal Genotype-Immunophenotype Relationships and Predictors of Response to Checkpoint Blockade. Cell Rep (2017) 18:248–62. doi: 10.1016/j.celrep.2016.12.019
26. Barlesi F, Vansteenkiste J, Spigel D, Ishii H, Garassino M, De Marinis F, et al. Avelumab versus docetaxel in patients with platinum-treated advanced non-small-cell lung cancer (JAVELIN Lung 200): An open-label, randomised, phase 3 study. Lancet Oncol (2018) 19:1468–79. doi: 10.1016/S1470-2045(18)30673-9
27. Borghaei H, Paz-Ares L, Horn L, Spigel DR, Steins M, Ready NE, et al. Nivolumab Versus Docetaxel in Advanced Nonsquamous Non–Small-Cell Lung Cancer. N Engl J Med (2015) 373:1627–39. doi: 10.1056/NEJMoa1507643
28. Garassino MC, Cho B, Kim J, Mazières J, Vansteenkiste J, Lena H, et al. Durvalumab as Third-Line or Later Treatment for Advanced Non-Small-Cell Lung Cancer (Atlantic): an Open-Label, Single-Arm, Phase 2 Study. Lancet Oncol (2018) 19:521–36. doi: 10.1016/S1470-2045(18)30144-X
29. Herbst RS, Baas P, Kim D, Felip E, Pérez-Gracia JL, Han J, et al. Pembrolizumab Versus Docetaxel for Previously Treated, Pd-L1-Positive, Advanced Non-Small-Cell Lung Cancer (Keynote-010): a Randomised Controlled Trial. Lancet (2016) 387:1540–50. doi: 10.1016/S0140-6736(15)01281-7
30. Mahoney KM, Rennert PD, Freeman GJ. Combination Cancer Immunotherapy and New Immunomodulatory Targets. Nat Rev Drug Discov (2015) 14:561–84. doi: 10.1038/nrd4591
31. Yu J, Tian S, Metheny-Barlow L, Chew LJ, Hayes AJ, Pan H, et al. Modulation of Endothelial Cell Growth Arrest and Apoptosis by Vascular Endothelial Growth Inhibitor. Circ Res (2001) 89:1161–7. doi: 10.1161/hh2401.101909
32. Keenan TE, Burke KP, Van Allen EM. Genomic Correlates of Response to Immune Checkpoint Blockade. Nat Med (2019) 25:1. doi: 10.1038/s41591-019-0382-x
33. Laky K, Fowlkes BJ. Notch Signaling in Cd4 and Cd8 T Cell Development. Curr Opin Immunol (2008) 20:197–202. doi: 10.1016/J.Coi.2008.03.004
34. Janghorban M, Xin L, Rosen JM, Zhang XH. Notch Signaling as a Regulator of the Tumor Immune Response: to Target or Not to Target. Front Immunol (2018) 9:1649:1649. doi: 10.3389/Fimmu.2018.01649
35. Luo X, Xu L, Li Y, Tan H. Notch Pathway Plays a Novel and Critical Role in Regulating Responses of T and Antigen-Presenting Cells in Agvhd. Front Immunol (2017) 33:169–81. doi: 10.1007/s10565-016-9364-7
Keywords: NOTCH pathway, DDR pathway, co-occurring mutations, immunotherapy, predictive biomarker, non-small cell lung cancer
Citation: Zhang Z, Gu Y, Su X, Bai J, Guan W, Ma J, Luo J, He J, Zhang B, Geng M, Xia X, Guan Y, Shen C and Chen C (2021) Co-Occurring Alteration of NOTCH and DDR Pathways Serves as Novel Predictor to Efficacious Immunotherapy in NSCLC. Front. Oncol. 11:659321. doi: 10.3389/fonc.2021.659321
Received: 27 January 2021; Accepted: 29 March 2021;
Published: 22 April 2021.
Edited by:
Meijuan Huang, Sichuan University, ChinaReviewed by:
Damini Chand, Canada’s Michael Smith Genome Sciences Centre, CanadaJianchun Duan, Chinese Academy of Medical Sciences and Peking Union Medical College, China
Copyright © 2021 Zhang, Gu, Su, Bai, Guan, Ma, Luo, He, Zhang, Geng, Xia, Guan, Shen and Chen. This is an open-access article distributed under the terms of the Creative Commons Attribution License (CC BY). The use, distribution or reproduction in other forums is permitted, provided the original author(s) and the copyright owner(s) are credited and that the original publication in this journal is cited, in accordance with accepted academic practice. No use, distribution or reproduction is permitted which does not comply with these terms.
*Correspondence: Cheng Shen, c2M5NDFAc2luYS5jb20=; Chuan Chen, c2lua3JpdmVyQDEyNi5jb20=
†These authors have contributed equally to this work