- 1Department of Molecular Oncology, BC Cancer, Vancouver, BC, Canada
- 2Department of Pathology & Laboratory Medicine, University of British Columbia, Vancouver, BC, Canada
Ewing sarcoma (EwS) is a highly malignant bone and soft tissue tumor primarily affecting children and young adults. While most patients initially respond well to conventional front-line therapy, frequent metastasis results in poor 5-year overall survival rates for this disease. Accordingly, there is a critical need to develop better models to understand EwS metastasis. We and others previously used the ex vivo pulmonary metastasis assay (PuMA) to study lung metastasis in solid tumors including osteosarcoma (OS), but this technique has to date not been achievable for EwS. PuMA involves tail vein injection of fluorescent tumor cells into NOD-SCID mice, followed by their visualization in long-term cultures of tumor-bearing lung explants. Here we demonstrate successful implementation of PuMA for EwS cells using NOD-SCID-IL2 receptor gamma null (NSG) immunocompromised mice, which demonstrated high engraftment of EwS cell lines compared to NOD-SCID mice. This may be linked to immune permissiveness required by EwS cells, as increased basal cytotoxicity of EwS cells was observed in NOD-SCID compared to NSG lung sections, possibly due to the absence of natural killer (NK) cell activity in the latter. Together, our data demonstrate the utility of NSG mice for PuMA modeling of EwS lung metastasis.
Introduction
EwS is characterized in the majority of cases by the expression of EWSR1-FLI1 or EWSR1-ERG fusion proteins (1, 2). These oncoproteins function as chimeric transcription factors to regulate a broad range of candidate genes, leading to characteristic signatures of expression for these tumors (3–5). EwS tumors are highly metastatic, with dissemination occurring most commonly to lungs, bones, or bone marrow, with 20% of patients presenting with circulating EwS tumor cells at diagnosis (6). The 5-year overall survival of patients with metastasis at diagnosis or with recurrent disease remains dismal (7), highlighting the pressing need for extensive study into the mechanisms regulating EwS metastasis toward potential development of preventative therapeutic treatments. To facilitate the rapid progression of research into EwS metastasis, the development of cutting-edge methods that enable robust examination of in vivo tumorigenic behavior is essential.
The PuMA system has been used over the past 10 years to study tumor cell lung colonization, particularly OS (8–10). In PuMA experiments, green fluorescent protein (GFP), or other fluorescently-labeled tumor cells are injected via the tail vein into NOD-SCID mice. Growth of tumor cells is then monitored using fluorescence microscopy of lung sections that are cultured as ex vivo explants for several weeks. As such, PuMA recapitulates the arrest of tumor cells in the lung, and furthermore, allows for the direct quantification of metastatic cell growth in living lung tissues. Another major advantage over conventional metastasis models is that these studies can be performed without the need for whole animal studies, allowing for short-term temporal comparison of anti-metastatic effects of given interventions (e.g., candidate gene knockdown or specific drug treatments) in a 3D microenvironment (11–13).
The PuMA system has conventionally used the NOD-SCID immunocompromised mouse as the basis for ex vivo lung colonization studies (8–10). The NOD-scid IL2rγnull (NSG) mouse is a variant of NOD-SCID mice and was generated by introducing an X-linked IL2 receptor common gamma chain mutation to the NOD/Lt strain background carrying the SCID mutation (14). The interleukin 2 receptor gamma (IL2RG) gene produces the common gamma chain subunit, which serves as the signaling subunit for multiple cytokines (15). NOD-SCID and NSG mouse models share T and B cell depletion, loss of C5 complement, and impaired innate immunity (16). However, due to the absence of functional receptors for IL-2 and other cytokines, NSG mice are also deficient in NK cells (14). The NSG model has successfully been used to study metastasis of numerous different cancer types (16–18).
Despite the demonstrated utility of this experimental approach to the study of metastatic disease in OS, EwS cell lines have historically failed to grow in PuMA. In the present study, we set out to determine if alternative culture methods and a different mouse strain, namely NSG, would provide a lung microenvironment that is permissive for studying EwS metastatic growth in an adapted PuMA model. Our results demonstrate that PneumaCult™-ALI (PC medium) supports EwS cell survival and the maintenance of proper lung architecture in ex vivo cultures, in contrast to the B medium formulation used for conventional PuMA studies. In addition, we observe that in contrast to standard NOD-SCID mice, NSG mice are necessary for propagating EwS cells in the PuMA system. From these results we observe that enhanced immune permissiveness in NSG mice lacking NK cell activity may facilitate growth of EwS cells in the PuMA model compared to NOD-SCID mice, which have residual NK cells. Together, the results of this work demonstrate the novel adaptation of PuMA toward facilitating the critical study of metastatic disease in EwS.
Materials and Methods
Cell Lines and Reagents
The NK92 cell line was kindly provided by Dr. Dixie Mager. A673 and TC32 cells were purchased from ATCC and the plasmid for tdTomato stable expression was provided by Dr. John Ronald (Western University, Canada). eGFP-expressing MG63.3 cells were established by Ren et al. (9) and provided by Dr. Rosandra Kaplan (Pediatric Oncology Branch, National Cancer Institute). All cell lines were tested for mycoplasma on a regular basis using the LookOut Mycoplasma Detection Kit (Sigma).
PuMA
Studies were conducted under the UBC animal care certificate #A19-0143. PuMA assays were performed as described previously (8), with several modifications. Specifically, 1 × 106 viable A673 or TC32 (tdTomato+) cells were injected via tail-veins into either NOD-SCID or NSG 5–8 week-old female mice. Within 15–20 min of injection, mice were euthanized according to institutional animal care guidelines. A 1.2% agarose solution diluted 1:1 (v/v) in PneumaCult™-ALI (PC) basal media (STEMCELL technologies #05002) with 10x supplement (#05003) culture medium at 37°C was used to insufflate the lung via cannulation of the trachea with a Surflo Teflon IV Catheter 20G × 1.25” (Terumo Medical Canada Inc.). Lungs were then removed and placed in an ice-cold solution of PBS containing 100 U/mL penicillin and 100 μg/mL streptomycin and incubated at 4°C for 20-min. Transverse sections (1–2 mm in thickness) were made from each lobe and placed on a single 1.5 × 0.7-cm sterile Gelfoam (Pfizer) section pre-incubated overnight in a 6-well-plate with PC medium. For continuous culture, lung sections were incubated at 37°C in a humidified environment with 5% CO2. Every 48 h, culture medium was replaced with fresh PC.
Renal Subcapsular Implantation Model
Studies were conducted under the UBC animal care certificate #A19-0143. In brief, as described by Mendoza-Naranjo et al. (19), xenograft cell blocks for implantation were prepared using 1 × 106 viable TC32 EwS cells (tdTomato+) and implanted under the renal capsules of 6–8 week-old NSG immunocompromised male mice from the Animal Resource Center in the BC Cancer Research Center (n = 4). Animals were maintained according to UBC Animal Care Committee (ACC) regulations. Five weeks post-inoculation, mice were euthanized and lungs were collected for analysis.
Histology/Immunohistochemistry
Paraffin-embedded lung sections were sectioned and processed for standard H&E's or immunohistochemistry (IHC) using antibodies to CD99 (Abcam #ab27271) followed by ImmunoHistoMount (ImmunoBioscience #AR-6503-01) staining. Immunofluorescence was performed using primary mouse anti-CD99 antibody [HO36-1.1] (Abcam #ab212605) and anti-Granzyme B antibody [EPR22645-206] (Abcam #ab255598). Secondary anti-mouse Alexa 594 (Thermo Scientific #A11005) and anti-rabbit Alexa 488 (Thermo Scientific #A11008) antibodies were used for detection. Images were acquired using Zeiss LSM 800 Airyscan system controlled with Zen Blue software (version 2.6).
Fluorescent Imaging
Fluorescent images were acquired using a Colibri Observer Z1 microscope equipped with an Axiocam MRm and controlled using Zen Blue Software (version 3.1). Areas of fluorescence as a measure of metastatic burden were calculated using ImageJ and expressed as means of the fluorescent area in % area of each lung section across 10 individual sections. Fluorescent areas were normalized to day 0 and expressed as fold-changes. The Zeiss LSM 800 Airyscan system was used for confocal imaging using Zen Blue software (version 2.6).
In vitro NK Cytotoxicity Assay
NK92 cell viability was determined by Trypan blue (Sigma-Aldrich) and maintained at 91–93% across experiments. NK92 effector cells were added 24 h after A673, TC32, or MG63.3 target cell seeding at the indicated effector to target (E:T) ratios. After 24 h in co-culture, target cells were fixed and stained with crystal violet. Absorbance was quantified using SpectraMax i3 plate reader (Molecular Devices).
Results
While current in vivo xenograft models of EwS are effective for analyzing primary tumor growth, they often fail to allow assessment of metastatic disease before experimental endpoints are reached. The PuMA method facilitates the quantification and characterization of metastatic lesions in lung tissues over 14 days or more in a pathophysiologic setting, where interactions with lung epithelial cells and stromal elements are retained (8–10). PuMA has historically used NOD-SCID mice and so-called B medium for culturing lung explants, but we have observed these conditions to be incompatible with EwS cell growth in the PuMA model. We wondered whether this might be due to toxicity of EwS cells when cultured in B media, or the use of NOD-SCID mouse that have residual NK cell activity. We first compared EwS cell viability in B medium vs. a commercially available formulation called PneumaCult™-ALI (PC) medium designed for human airway epithelial cells cultured at the air-liquid interface (20). Using crystal violet staining, we observed that in contrast to B medium, EwS cells survived in PC medium (Figure 1A). Culture in PC medium was also observed to maintain pulmonary microarchitecture of both NOD-SCID and NSG mice over 14 days of PuMA ex vivo cultivation (Figure 1B).
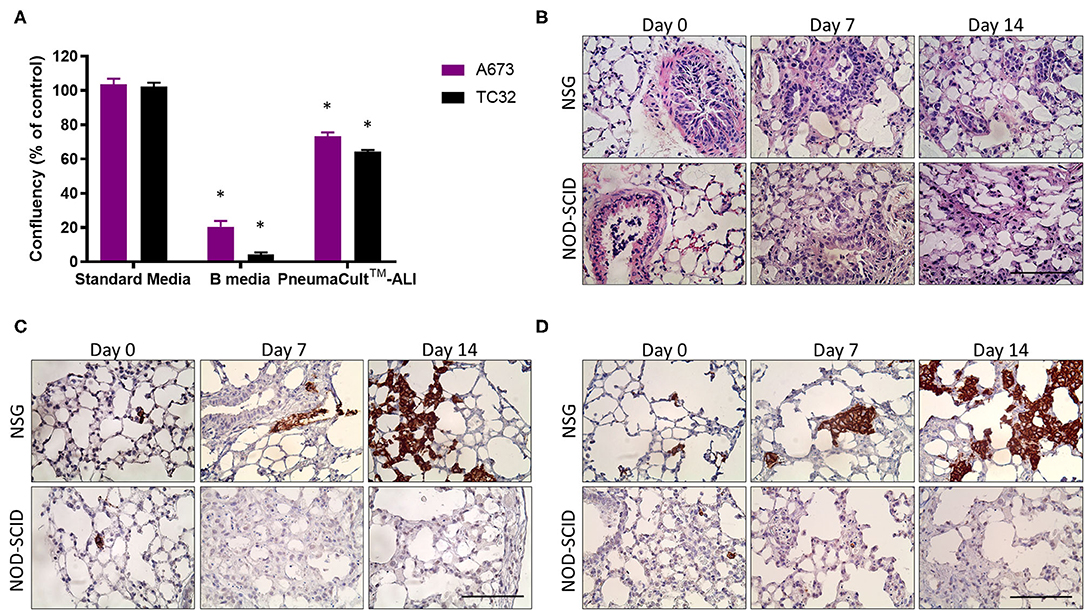
Figure 1. PneumaCult™-ALI [PC] medium maintains lung architecture during ex vivo growth. (A) Percentage of viable EwS cells cultured in B- and PC-medium relative to standard culture conditions for 96 h. Values reported represent the mean ± standard deviation (SD) of at least three independent experiments. Statistical analysis of differences between pairs was performed using a two-way ANOVA with a Tukey's post-hoc test (*p < 0.01). (B) H&E sections of NOD-SCID and NSG mouse lungs showing integrity of lung structures after 7 and 14 days. (C) Immunohistochemistry showing CD99 immunoreactive A673 and (D) TC32 cells in NOD-SCID and NSG mice lung slices at 0, 7, and 14 days post-injection. Three animals were included per group (n = 3) and a minimum of 10 lung tissue sections were analyzed per animal. Scale bars = 100 μm.
We next assessed the potential impact of residual NK activity in NOD-SCID mice on EwS cell survival in PuMA. To this end, tdTomato-labeled A673 and TC32 EwS cells were tested in PuMA experiments that used NOD-SCID or NSG mice, both carried out using PC medium for subsequent lung explant cultures. To assess lung colonization, the cell surface marker CD99 was used to detect EwS cells by IHC (21). CD99 positive A673 and TC32 cells were confirmed on Day 0 (Figures 1C,D), demonstrating that EwS tumor cells initially arrive in lungs in both strains (average area in μm2 = 8 and 18 for NSG and NOD/SCID, respectively). However, by 7 days of ex vivo lung culture, CD99 positive cells were only observed in NSG mice (Figures 1C,D). To quantify these findings, tdTomato fluorescence in A673 and TC32 PuMA lung sections were imaged over the same time period. As with CD99 staining, tdTomato-positive cells were observed in both strains at day 0, but only in NSG mice at day 7 and 14 time points of ex vivo growth (Figures 2A,B,D,E). Comparatively, MG63.3 OS cells were detected after 14 days in both mouse strains (Figures 2C,F). Lung lesions and microarchitecture observed for EwS cells in PuMA from NSG mice were comparable to in vivo results using renal subcapsular injection (Figures 1C,D, 2G). Together, this indicates that EwS cell lung colonization can be assessed using the PuMA model, but that this requires the use of PC medium and NSG mice.
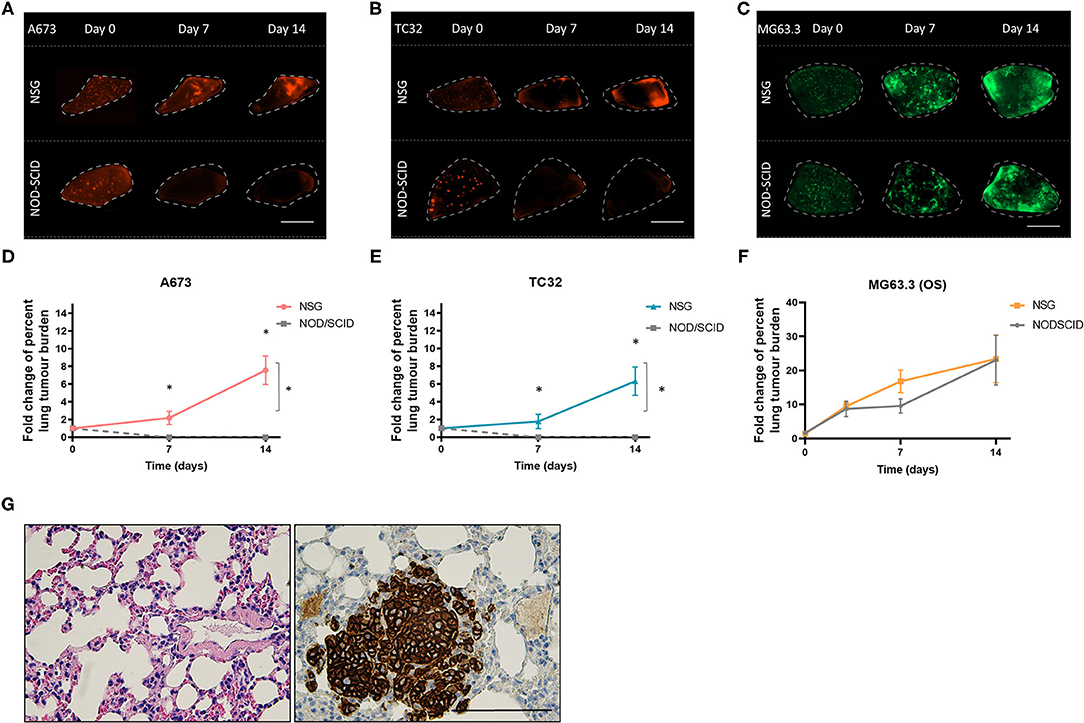
Figure 2. Ex vivo growth of EwS cells is maintained in NSG mice. Fluorescence images for A673 (A) TC32 (B) and MG63.3 (C) tdTomato+ EwS cells at day 0, 7, and 14 post-injection. Scale bars = 1 mm. Fold change in the percent metastatic tumor burden of A673 (D), TC32 (E), and (F) MG63.3 cells. Three animals were included per group (n = 3) and a minimum of 10 lung tissue sections were analyzed per animal. Results are shown as mean ± SD. Statistical assessment of differences was determined using two-way ANOVA tests with a Tukey's post-hoc test (*p < 0.01). (G) CD99 immunoreactive TC32 EwS cells in NSG mouse lung slices at 5 weeks after renal subcapsular implantation of EwS cells. Three animals were included per group (n = 3) and a minimum of 10 lung tissue sections were analyzed per animal. Scale bars = 100 μm.
To examine whether the ability of EwS cells to grow in lung explants of NSG but not NOD-SCID mice was potentially linked to selective NK cytotoxicity in the latter strain, we compared expression of the cytotoxic effector, Granzyme B in lung sections. Granzyme B is a serine protease secreted by cytotoxic T-lymphocytes and NK cells upon binding to target cells, leading to apoptosis of the latter (22). Immunofluorescence of PuMA lung sections from A673 EwS cells revealed the presence of Granzyme B in proximity to CD99-positive cells in NOD-SCID mice, but not NSG mice, at day 3 following tumor cell injection (Figure 3A). To validate that residual NK cells in NOD-SCID lungs might be sufficient to eliminate EwS but not OS cells, the human NK cell line NK92 (effector, E) was co-cultured with EwS or OS cells (tumor, T) at 1:1, 5:1, and 10:1 E:T ratios. After 24 h, the cytotoxic effects of the lowest E:T ratio of 1:1 in both EwS cell lines were significantly enhanced compared to cytotoxicity of MG63.3 cells (p = 0.01 and 0.03, respectively), although at higher E:T ratios, OS cells were also killed (Figure 3B).
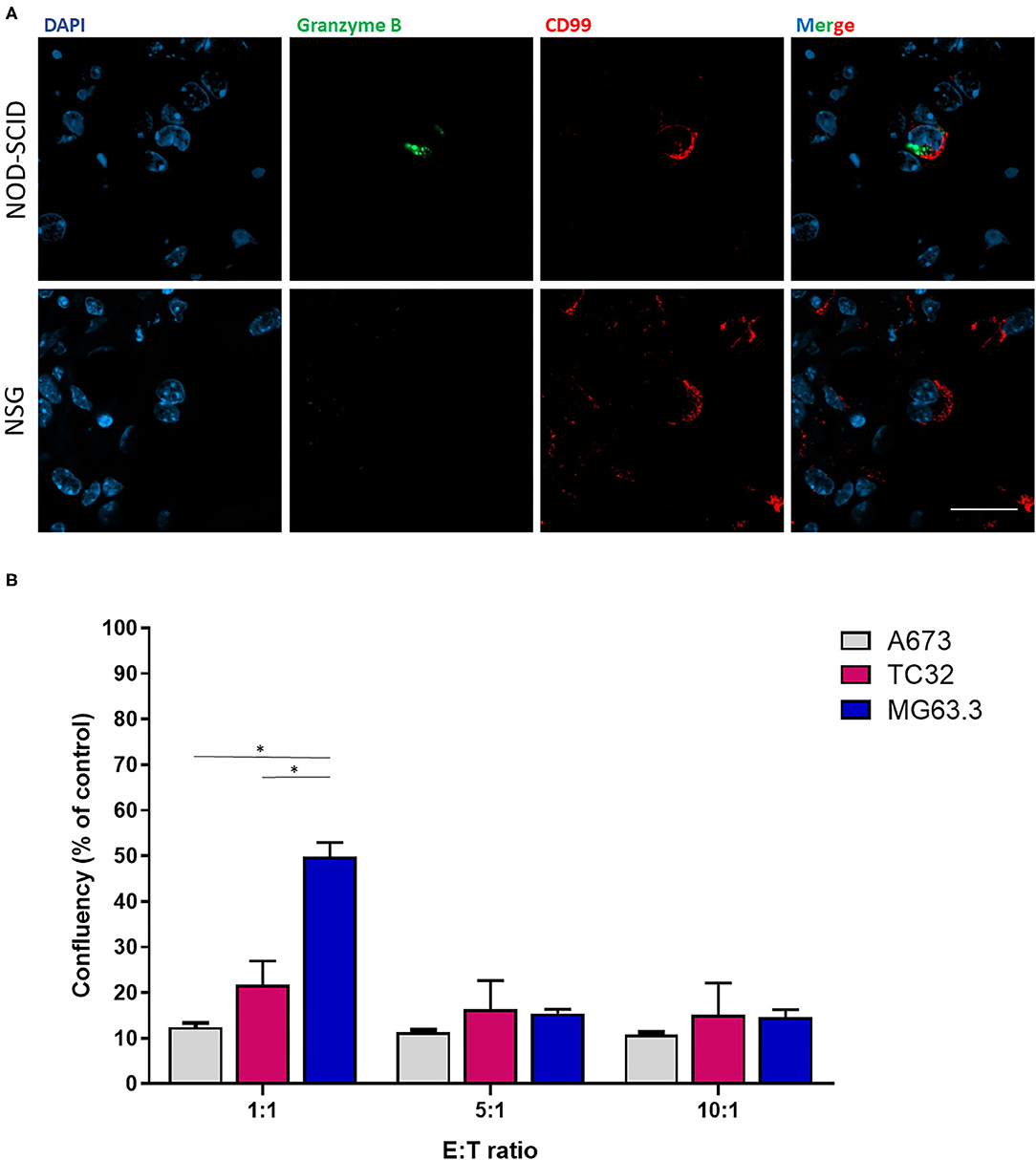
Figure 3. Effects of NK cells on ex vivo and in vitro EwS cell growth. (A) Granzyme B and CD99 co-staining in NOD-SCID and NSG mice at 3-days post-injection of EwS cells. Three animals were included per group (n = 3) and a minimum of 10 lung tissue sections were analyzed per animal. (B) NK92 cytotoxic effects on EwS compared to OS cells. Ratio-dependent cytotoxicity of NK92 cells was determined for A673, TC32, and MG63.3 cells. Bar graphs represent the mean ± SD of a minimum of three independent experiments. Statistical analysis of differences was calculated using a Student's t-test (*p < 0.01).
Discussion
PuMA has proven to be a very useful ex vivo model to study mechanisms of tumor cell lung colonization, particularly in OS (8–10). In a recent study, Morrow et al. document changes in enhancer usage in OS metastatic vs. primary tumor cells driven by the lung microenvironment (12). However, for unknown reasons the PuMA technique has to date not been applicable for EwS cells. In this study we set out to explore possible reasons, including whether the culture conditions and the mouse strains used for conventional PuMA modeling might hinder EwS cell survival. Our results demonstrate the successful adaptation of the PuMA system for studying EwS lung colonization, by substituting B medium for PC medium, and utilizing NSG mice rather than NOD-SCID mice as the host strain.
While NOD-SCID and NSG mouse models both are characterized by T and B cell depletion, loss of C5 complement, and impaired innate immunity, NSG mice are also deficient in NK cells due to the absence of functional receptors for IL-2 and other cytokines (16). Indeed, the beneficial effects of an anti-NK cell antibody before transplantation of human cells in NOD-SCID mice originally led to the development of the NSG model (23), which is associated with improved engraftment of a number of malignant cell types (16–18). Additionally, analysis of immune cell subsets and patient survival revealed that tumor-infiltrating activated NK cells confer prolonged overall survival for EwS patients (24). Previous studies also demonstrated that EwS cells are exquisitely sensitive to NK cell-mediated killing compared with OS and other tumor types (25–27). Our results show Granzyme B secretion in proximity to CD99 positive cells in NOD-SCID mice and significantly enhanced cytotoxic effects of the lowest NK92 E:T ratio of 1:1 in EwS cells compared to OS cells. Adoptive therapy using NK cells overexpressing the activating receptor NKG2D decreased the number of pulmonary metastatic nodules in an EwS NSG xenograft model (28), while in contrast, pharmacological upregulation of NKG2D led to functional NK cells that failed to infiltrate and reduce lung nodules in a nude mouse OS lung metastasis model (29). Together, these data point to NKG2D interactions with its ligands as at least partially explaining enhanced NK cell cytotoxicity in EwS. Accordingly, blocking NKG2D receptor considerably reduced NK cytotoxicity to EwS cells in previous studies (25, 27).
Our results therefore suggest that the immune permissiveness required by EwS cells may result from the lack of active NK cells in NSG mice and additional immune features that should be explored further. Adapting PuMA to NSG mice therefore provides a robust model to study EwS lung metastasis, facilitating the search for novel therapeutically approaches to reduce the burden of metastatic disease in EwS.
Data Availability Statement
The raw data supporting the conclusions of this article will be made available by the authors, without undue reservation.
Ethics Statement
The animal study was reviewed and approved by UBC Animal Care Committee (ACC).
Author Contributions
RS-R conceived the experiments, performed data analysis, and wrote the manuscript. ML and H-FZ performed experiments, performed data analysis, and contributed to the writing and editing of the manuscript. A-CD and CH performed data analysis and contributed to the writing and editing of the manuscript. PS oversaw the project, contributed to the interpretation of results, and co-wrote the manuscript. All authors contributed to the article and approved the submitted version.
Funding
This work was supported by a St. Baldrick's Foundation Martha's Better Ewing Sarcoma Treatment (BEST) grant to PS (grant #663113) and a Terry Fox Research Institute (grant #1082). This work was also supported in part by funds from the British Columbia Cancer Foundation (to PS).
Conflict of Interest
The authors declare that the research was conducted in the absence of any commercial or financial relationships that could be construed as a potential conflict of interest.
References
1. Delattre O, Zucman J, Plougastel B, Desmaze C, Melot T, Peter M, et al. Gene fusion with an ETS DNA-binding domain caused by chromosome translocation in human tumours. Nature. (1992) 359:162–5. doi: 10.1038/359162a0
2. Sorensen PH, Lessnick SL, Lopez-Terrada D, Liu XF, Triche TJ, Denny CT. A second Ewing's sarcoma translocation, t(21;22), fuses the EWS gene to another ETS-family transcription factor, ERG. Nat Genet. (1994) 6:146–51. doi: 10.1038/ng0294-146
3. Riggi N, Suva ML, Suva D, Cironi L, Provero P, Tercier S, et al. EWS-FLI-1 expression triggers a Ewing's sarcoma initiation program in primary human mesenchymal stem cells. Cancer Res. (2008) 68:2176–85. doi: 10.1158/0008-5472.CAN-07-1761
4. Tomazou EM, Sheffield NC, Schmidl C, Schuster M, Schonegger A, Datlinger P, et al. Epigenome mapping reveals distinct modes of gene regulation and widespread enhancer reprogramming by the oncogenic fusion protein EWS-FLI1. Cell Rep. (2015) 10:1082–95. doi: 10.1016/j.celrep.2015.01.042
5. Prieur A, Tirode F, Cohen P, Delattre O. EWS/FLI-1 silencing and gene profiling of Ewing cells reveal downstream oncogenic pathways and a crucial role for repression of insulin-like growth factor binding protein 3. Mol Cell Biol. (2004) 24:7275–83. doi: 10.1128/MCB.24.16.7275-7283.2004
6. Schleiermacher G, Peter M, Oberlin O, Philip T, Rubie H, Mechinaud F, et al. Increased risk of systemic relapses associated with bone marrow micrometastasis and circulating tumor cells in localized ewing tumor. J Clin Oncol. (2003) 21:85–91. doi: 10.1200/JCO.2003.03.006
7. Gaspar N, Hawkins DS, Dirksen U, Lewis IJ, Ferrari S, Le Deley MC, et al. Ewing sarcoma: current management and future approaches through collaboration. J Clin Oncol. (2015) 33:3036–46. doi: 10.1200/JCO.2014.59.5256
8. Mendoza A, Hong SH, Osborne T, Khan MA, Campbell K, Briggs J, et al. Modeling metastasis biology and therapy in real time in the mouse lung. J Clin Invest. (2010) 120:2979–88. doi: 10.1172/JCI40252
9. Ren L, Mendoza A, Zhu J, Briggs JW, Halsey C, Hong ES, et al. Characterization of the metastatic phenotype of a panel of established osteosarcoma cells. Oncotarget. (2015) 6:29469–81. doi: 10.18632/oncotarget.5177
10. Lizardo MM, Sorensen PH. Practical considerations in studying metastatic lung colonization in osteosarcoma using the pulmonary metastasis assay. J Vis Exp. (2018) 56332. doi: 10.3791/56332
11. Lizardo MM, Morrow JJ, Miller TE, Hong ES, Ren L, Mendoza A, et al. Upregulation of glucose-regulated protein 78 in metastatic cancer cells is necessary for lung metastasis progression. Neoplasia. (2016) 18:699–710. doi: 10.1016/j.neo.2016.09.001
12. Morrow JJ, Bayles I, Funnell APW, Miller TE, Saiakhova A, Lizardo MM, et al. Positively selected enhancer elements endow osteosarcoma cells with metastatic competence. Nat Med. (2018) 24:176–85. doi: 10.1038/nm.4475
13. Hong SH, Ren L, Mendoza A, Eleswarapu A, Khanna C. Apoptosis resistance and PKC signaling: distinguishing features of high and low metastatic cells. Neoplasia. (2012) 14:249–58. doi: 10.1593/neo.111498
14. Shultz LD, Lyons BL, Burzenski LM, Gott B, Chen X, Chaleff S, et al. Human lymphoid and myeloid cell development in NOD/LtSz-scid IL2R gamma null mice engrafted with mobilized human hemopoietic stem cells. J Immunol. (2005) 174:6477–89. doi: 10.4049/jimmunol.174.10.6477
15. Asao H, Okuyama C, Kumaki S, Ishii N, Tsuchiya S, Foster D, et al. Cutting edge: the common gamma-chain is an indispensable subunit of the IL-21 receptor complex. J Immunol. (2001) 167:1–5. doi: 10.4049/jimmunol.167.1.1
16. Puchalapalli M, Zeng X, Mu L, Anderson A, Hix Glickman L, Zhang M, et al. NSG mice provide a better spontaneous model of breast cancer metastasis than athymic (Nude) mice. PLoS ONE. (2016) 11:e0163521. doi: 10.1371/journal.pone.0163521
17. Quintana E, Piskounova E, Shackleton M, Weinberg D, Eskiocak U, Fullen DR, et al. Human melanoma metastasis in NSG mice correlates with clinical outcome in patients. Sci Transl Med. (2012) 4:159ra49. doi: 10.1126/scitranslmed.3004599
18. DeRose YS, Wang G, Lin YC, Bernard PS, Buys SS, Ebbert MT, et al. Tumor grafts derived from women with breast cancer authentically reflect tumor pathology, growth, metastasis and disease outcomes. Nat Med. (2011) 17:1514–20. doi: 10.1038/nm.2454
19. Mendoza-Naranjo A, El-Naggar A, Wai DH, Mistry P, Lazic N, Ayala FR, et al. ERBB4 confers metastatic capacity in Ewing sarcoma. EMBO Mol Med. (2013) 5:1087–102. doi: 10.1002/emmm.201202343
20. Nikolic MZ, Caritg O, Jeng Q, Johnson JA, Sun D, Howell KJ, et al. Human embryonic lung epithelial tips are multipotent progenitors that can be expanded in vitro as long-term self-renewing organoids. Elife. (2017) 6:e26575. doi: 10.7554/eLife.26575
21. Balamuth NJ, Womer RB. Ewing's sarcoma. Lancet Oncol. (2010) 11:184–92. doi: 10.1016/S1470-2045(09)70286-4
22. Lieberman J. The ABCs of granule-mediated cytotoxicity: new weapons in the arsenal. Nat Rev Immunol. (2003) 3:361–70. doi: 10.1038/nri1083
23. Yoshino H, Ueda T, Kawahata M, Kobayashi K, Ebihara Y, Manabe A, et al. Natural killer cell depletion by anti-asialo GM1 antiserum treatment enhances human hematopoietic stem cell engraftment in NOD/Shi-scid mice. Bone Marrow Transplant. (2000) 26:1211–6. doi: 10.1038/sj.bmt.1702702
24. Stahl D, Gentles AJ, Thiele R, Gutgemann I. Prognostic profiling of the immune cell microenvironment in Ewing s sarcoma family of tumors. Oncoimmunology. (2019) 8:e1674113. doi: 10.1080/2162402X.2019.1674113
25. Cho D, Shook DR, Shimasaki N, Chang YH, Fujisaki H, Campana D. Cytotoxicity of activated natural killer cells against pediatric solid tumors. Clin Cancer Res. (2010) 16:3901–9. doi: 10.1158/1078-0432.CCR-10-0735
26. Ahn YO, Weigel B, Verneris MR. Killing the killer: natural killer cells to treat Ewing's sarcoma. Clin Cancer Res. (2010) 16:3819–21. doi: 10.1158/1078-0432.CCR-10-1368
27. Verhoeven DH, de Hooge AS, Mooiman EC, Santos SJ, ten Dam MM, Gelderblom H, et al. NK cells recognize and lyse Ewing sarcoma cells through NKG2D and DNAM-1 receptor dependent pathways. Mol Immunol. (2008) 45:3917–25. doi: 10.1016/j.molimm.2008.06.016
28. Tong AA, Hashem H, Eid S, Allen F, Kingsley D, Huang AY. Adoptive natural killer cell therapy is effective in reducing pulmonary metastasis of Ewing sarcoma. Oncoimmunology. (2017) 6:e1303586. doi: 10.1080/2162402X.2017.1303586
Keywords: lung metastasis, Ewing sarcoma, osteosarcoma, PuMA, NSG mice, NK cells
Citation: Scopim-Ribeiro R, Lizardo MM, Zhang H-F, Dhez A-C, Hughes CS and Sorensen PH (2021) NSG Mice Facilitate ex vivo Characterization of Ewing Sarcoma Lung Metastasis Using the PuMA Model. Front. Oncol. 11:645757. doi: 10.3389/fonc.2021.645757
Received: 23 December 2020; Accepted: 22 February 2021;
Published: 22 March 2021.
Edited by:
Anat Erdreich-Epstein, Children's Hospital of Los Angeles, United StatesReviewed by:
Maria Tsokos, Beth Israel Deaconess Medical Center and Harvard Medical School, United StatesJoanna Kitlinska, Georgetown University, United States
Copyright © 2021 Scopim-Ribeiro, Lizardo, Zhang, Dhez, Hughes and Sorensen. This is an open-access article distributed under the terms of the Creative Commons Attribution License (CC BY). The use, distribution or reproduction in other forums is permitted, provided the original author(s) and the copyright owner(s) are credited and that the original publication in this journal is cited, in accordance with accepted academic practice. No use, distribution or reproduction is permitted which does not comply with these terms.
*Correspondence: Poul H. Sorensen, psorensen@bccrc.ca