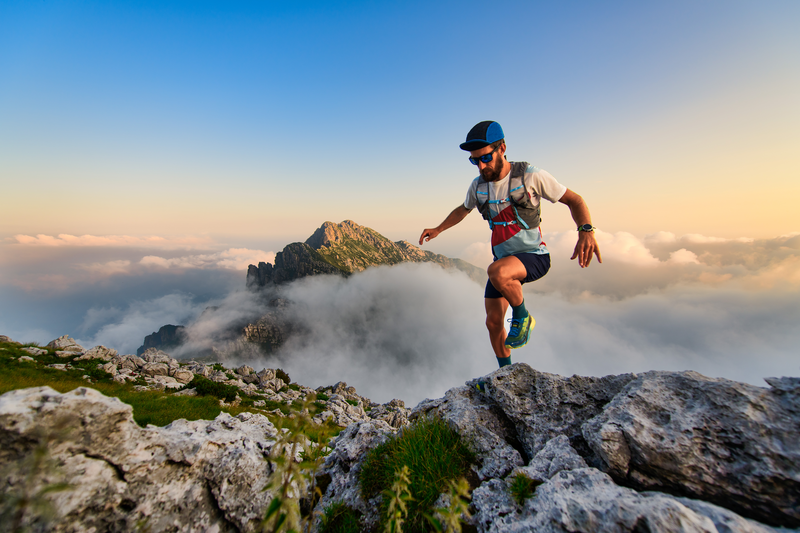
94% of researchers rate our articles as excellent or good
Learn more about the work of our research integrity team to safeguard the quality of each article we publish.
Find out more
ORIGINAL RESEARCH article
Front. Oncol. , 14 September 2021
Sec. Molecular and Cellular Oncology
Volume 11 - 2021 | https://doi.org/10.3389/fonc.2021.622626
This article is part of the Research Topic Liquid Biopsy View all 27 articles
Introduction: Desmoid tumor (DT) is a rare neoplasm with high local recurrence rates, composed of fibroblastic cells that are characterized by the expression of key molecules, including the intermediate filament vimentin, cyclooxygenase-2 (COX-2), and nuclear β-catenin, and lack of epithelial markers. Circulating tumor cells (CTCs) isolated from the peripheral blood of patients with sarcomas and other neoplasms can be used as early biomarkers of tumor invasion and dissemination. Moreover, CTCs can also re-colonize their tumors of origin through a process of “tumor self-seeding.”
Objectives: We aimed to identify CTCs in the peripheral blood of patients with DT and evaluate their expression of β-catenin, transforming growth factor receptor I (TGF-βRI), COX-2, and vimentin proteins.
Material and Methods: We conducted a prospective study of patients with initial diagnosis or relapsed DT with measurable disease. Blood samples from each patient were processed and filtered by ISET® (Rarecells, France) for CTC isolation and quantification. The CTC expression of β-catenin, COX-2, TGF-βRI, and vimentin was analyzed by immunocytochemistry (ICC).
Results: A total of 18 patients were included, and all had detectable CTCs. We found a concordance of β-catenin expression in both CTCs and primary tumors in 42.8% (6/14) of cases by using ICC and immunohistochemistry, respectively.
Conclusions: Our study identified a high prevalence of CTCs in DT patients. Concordance of β-catenin expression between primary tumor and CTCs brings new perspectives to assess the dynamics of CTCs in the blood compartment, opening new avenues for studying the biology and behavior of DT. In addition, these results open the possibility of using CTCs to predict DT dynamics at the time of disease progression and treatment. Further studies with larger sample sizes are needed to validate our findings.
Desmoid tumor (DT) is a rare non-metastasizing mesenchymal neoplasm that can show aggressive local behavior and thereby can impact the functionality and quality of life of the patients (1, 2). DTs are typically diagnosed in adults (35–40 years) and are more common in women of reproductive age (3, 4). They can affect any anatomic site but are commonly localized in the extremities, abdominal wall, and abdominal mesentery (5). The clinical outcomes of DTs are unpredictable, with some progressing to large-sized tumors with infiltration and destruction of adjacent vital structures, and others showing spontaneous regression (6). Nevertheless, reliable and validated predictive factors regarding DT evolution are still lacking (7).
The initial management of asymptomatic patients with non-life-threatening DTs is often watch-and-wait, as up to 20% will regress spontaneously (8). If treatment is indicated, options include surgery, radiation, or systemic therapy with tyrosine-kinase inhibitors or chemotherapy (6, 8). Due to the infiltrative nature of the disease, post-surgical recurrence rates can exceed 50% of cases, especially for extra-abdominal desmoids, which reinforce observation as an adequate strategy (9).
Histologically, DTs are composed of fibroblastic cells characterized on immunohistochemistry (IHC) by the expression of intermediate filament vimentin, along with cyclooxygenase-2 (COX-2) and nuclear β-catenin, and lack of epithelial markers expression (1, 10, 11). Approximately 90% of DTs are sporadic, while the remaining cases are usually related to familial adenomatous polyposis (FAP), specifically Gardner syndrome (12). In both cases, mutations that activate the Wnt/β-catenin signaling pathway are likely to play a significant role in tumorigenesis (13). Wnt/β-catenin signaling is involved in numerous processes, such as the control of gene expression and regulation of cell adhesion and polarity (14). In addition, approximately 85% of DTs are related to mutations in the exon 3 of the beta-catenin encoding gene CTNNB1 (15). CTNNB1 mutations of DTs generally occur at codons 41 or 45, with p.T41A (threonine to alanine), p.S45F (serine to phenylalanine), and p.S45P (serine to proline) being the most frequent ones (15–21).
Used as a new tool for diagnosing and monitoring cancer, liquid biopsies have received increasing attention. Using a simple blood test has enormous implications for the diagnosis of cancer, so it is possible to avoid invasive tissue biopsies in the future and obtain a similar result from the circulating tumor cell (CTC) test (22).
CTCs play a central role on tumor dissemination and metastasis, which are ultimately responsible for most cancer deaths. Cancer cells can enter circulation years before a tumor is diagnosed. The majority of cells die, and only a minor fraction contains viable metastatic precursors that infiltrate organs and survive for eventual relapse (23–25). The presence of CTCs and/or circulating tumor microemboli (CTMs) in the peripheral blood of patients can be early markers of tumor invasion and spread (26). The dissemination of cancer cells from a primary tumor is conventionally viewed as a unidirectional process that culminates with the metastatic colonization of distant organs. CTCs and CTMs are associated with poor prognosis in several carcinomas. Furthermore, CTCs can colonize their tumors of origin through a process of “tumor self-seeding” (27). In contrast to carcinomas, few studies have examined CTCs and CTMs in mesenchymal tumor patients (28), and to the best of our knowledge, there are no reports of CTC detection in cases of DT.
Therefore, the aims of this study were to determine whether CTCs could be detected in DT patients and analyze their expression of mesenchymal proteins [vimentin, transforming growth factor receptor I (TGF-βRI)], as well as β-catenin and COX-2.
This was a prospective and descriptive study, conducted with DT patients treated at the A.C. Camargo Cancer Center, São Paulo, Brazil, between June 2017 and October 2019. Peripheral blood samples were obtained after written informed consent. CTCs were analyzed by ISET® assay (Rarecells Diagnostics, Paris, France). This study was approved by the local Research Ethics Board (REB protocol 2427/17). Inclusion criteria were age >18 years, diagnosis of DT, presence of measurable disease, and a negative medical history of recent surgical procedures or trauma. Patients with FAP were excluded.
Blood samples (8 ml) were drawn in EDTA tubes (BD Vacutainer®) with immediate gentle agitation after blood collection. If samples were not processed immediately after phlebotomy, the tubes were left on a blood homogenizer at room temperature for up to 4 h. The isolation by size of epithelial tumor cells (ISET) assay was performed as described previously (29). The samples were processed on the platform according to the manufacturer’s instructions. Eight milliliter of whole blood (1 ml per spot) was topped up to 80 ml with erythrocyte lysis buffer containing 0.02% formaldehyde, incubated for 10 min at room temperature, and filtered through an 8-µm pore membrane. To preserve cell integrity, filtration pressure was optimized at −10 kPa. The membrane was then washed once with phosphate-buffered saline 1×. After processing, membranes were dried, wrapped in aluminum foil, and stored at −20°C until use.
The spot membranes were subjected to immunocytochemistry (ICC) in 24-well plates to evaluate protein expression. We made single [one protein analyzed, stained with DAB (Dako™)] or double (two proteins analyzed, stained with DAB and magenta chromogen) ICC assays. Before ICC reactions, antigen retrieval was performed using Antigen Retrieval Solution (Dako™). Cells in the ISET spots were then hydrated with tris-buffered saline (TBS) 1× for 20 min and permeabilized with TBS + Triton X-100 for 5 min. Endogenous peroxides were blocked with 3% hydrogen peroxide and kept in the dark for 15 min. The spots were incubated with antibodies diluted in TBS supplemented with 10% fetal calf serum. For single staining, antibody staining was visualized with DAB (Dako™). To amplify the antibody signal for double staining, the spots were incubated with Envision G/2 Doublestain System, Rabbit/Mouse (Dako™), followed by a 10-min incubation with magenta chromogen to visualize the second antibody. Cells were stained with hematoxylin and analyzed by light microscopy (BX61-Olympus).
Negative and positive controls were added for each ICC staining. For positive controls, blood from healthy subjects was spiked with A549 and U-87 cell lines. According to the Human Protein Atlas (http://www.proteinatlas.org/), the A549 cell line expresses both β-catenin and TGF-βRI, whereas the U-87 cell line expresses both COX-2 and vimentin. These cell lines were acquired from ATCC® HTB-43™. For negative controls, A549 and U-87 cell lines spiked in healthy blood were not incubated with the primary antibody, to avoid cross-reactivity (Supplementary Figure 1). For protein expression analysis, cells were classified according to their staining characteristics. The absence of staining was considered negative, while staining of the nucleus or cytoplasm was classified as positive, as expected for each antibody. We did not perform statistical analysis to evaluate protein expression, progression-free survival, or overall survival due to the rarity of events in these patients and the short follow-up interval.
Recently, it was shown that the limit of detection of ISET is 1 CTC/10 ml of whole blood and that despite its high sensitivity, the distribution of CTCs in membrane spots is not uniform (30). Thus, we tested all eight spots fixed with 0.02% formaldehyde to ensure the observation of all isolated CTCs.
Eighteen patients were enrolled in this study, including two males and 16 females, with median age at the time of recruitment of 43.5 years (18–65 years). DTs were diagnosed in the lower extremities of 27.7% of patients. Clinical and pathological characteristics were obtained from medical records and are summarized in Table 1.
CTCs were detected in all patients. Eight spots were tested for each patient. Two spots were tested for β-catenin and vimentin expression (double staining), two were single-stained for either COX-2 or TGFβ-RI, and the last spots were stained with hematoxylin for CTC visualization. In addition, the estimated CTC count was made considering the average count of the eight spots evaluated. The average number of CTCs detected by ISET® was 2.6 CTC/ml (0.5–13 CTCs/ml). One CTM, a cluster of three or more CTCs, was found in one patient. Quantification and characterization of CTCs are presented in Table 2, Figure 1, and Supplementary Figure 2.
Figure 1 (A–F) CTCs isolated from patients with DTs. Cells visualized with hematoxylin. We can observe pleomorphic desmoid tumor CTCs with all cytopathological features: nucleus size ≥ 12 µm, hyperchromatic and irregular nucleus, visible presence of cytoplasm, and a high nucleus–cytoplasm ratio (31). White asterisk: leukocytes. Black asterisk: membrane spots. Images were taken at ×600 magnification using a light microscope (Research System Microscope BX61—Olympus, Tokyo, Japan) coupled to a digital camera (SC100—Olympus, Tokyo, Japan). CTCs, circulating tumor cells; DTs, desmoid tumors.
CTCs of 11 patients (61.1%) expressed β-catenin. Immunohistochemical analysis of primary tumors revealed that 11 patients were positive for β-catenin (Figure 2) and three were negative, and the results of four patients were not reported. Five patients expressed β-catenin in both CTCs and primary tumors, and one who tested negative in the primary tumor also tested negative in CTCs. Two patients were positive for β-catenin expression in CTCs and negative in primary tumors (Table 2). Because i) IHC is the gold standard method for β-catenin detection in primary tumors and ii) primary tumor β-catenin expression was available in the medical records of only 14 patients, we found a concordance rate between primary tumors and CTCs of 42.8% (6 concordant/14 samples). As concordance, we considered simultaneous positive or negative expression of a given protein in both CTCs and primary tumors. Among the 18 patients evaluated for protein expression in CTCs, vimentin was observed in five patients (27.7%) (Figure 3), while nine were positive for TGFβ-RI (50%) and seven for COX-2 (38.8%).
Figure 2 (A) Immunocytochemistry of CTC with β-catenin antibody counterstaining with DAB. (B–F) Immunocytochemistry of CTC with β-catenin antibody and counterstaining with magenta chromogen. We can observe different degrees of staining. (A, B) β-Catenin highly positive staining in a patient. (C, D) β-Catenin middle staining in a patient. (E, F) β-Catenin weakly positive staining in a patient. Black asterisk: membrane spots. Black arrows: β-catenin. CTC, circulating tumor cell.
Figure 3 Immunocytochemistry for vimentin. (A, B) Immunocytochemistry of CTC with vimentin antibody and counterstaining with DAB. (A) High staining. (B) Weak staining. (C) Desmoid tumor CTMs observed in the blood filtered using the ISET, stained with vimentin and counterstained with DAB chromogen. (D) Leukocytes from desmoid tumor patient. Black asterisk: membrane spots. Black arrows: vimentin expression. White asterisk: leukocytes. Keys: size comparison between a leukocyte and a spot. CTC, circulating tumor cell; CTMs, circulating tumor microemboli; ISET, isolation by size of epithelial tumor cells.
Although it was our first study with DT, we have extensive experience with ISET, as we have been working with this method for a long time, with many published papers (29, 32–40) including two with sarcomas (29, 41). In the first paper with sarcoma (29), we tested the sensitivity of the method, as we were the first group to test ISET for non-solid tumors, and we proved, with cell line, that its sensitivity was high, even for mesenchymal cells. After spiking 25, 50, 100, and 150 HT1080 cells (derived from human fibrosarcoma), in triplicate, in 1 ml of blood from healthy donors and filtering the blood using ISET, we found mean numbers of 25, 54, 100, and 155 cells, respectively. The numbers found were not identical to the numbers of cells spiked because tumor cells were counted in a counting chamber, by dilution, and not one by one. In this study, we also filtered blood samples from 30 healthy donors in ISET and found no CTCs.
Since DTs do not metastasize, it is apparently counterintuitive to find CTCs in the bloodstream of these patients. However, our study showed that CTCs can be detected and isolated from DT patients even from relatively low volumes of blood samples. This finding suggests that CTCs might be playing a different role on the DT biology. That allowed us to hypothesize that CTCs can be working as vehicles that reseed the primary tumor, which could potentiate its aggressiveness. CTCs are characterized by cytomorphological features that include nucleus size ≥12 µm; hyperchromatic and irregular nuclei; visible presence of cytoplasm; and a high nucleus–cytoplasm ratio (31). We defined CTCs as cells displaying all the aforementioned characteristics, and we also observed CTC pleomorphism (Figure 1 and Supplementary Figure 2). Morphologic changes resulting in more rounded or “fibroblastoid” CTCs were most likely acquired during in vitro cell handling or by the filtering process. In Supplementary Figure 3, examples of macrophages isolated from the blood of patients with DT can be appreciated, demonstrating the morphological differences between CTCs and macrophages.
The high prevalence of CTCs in our patient population might be explained by the self-seeding hypothesis. It has been proposed that self-seeding can accelerate tumor growth, angiogenesis, and stromal recruitment through seed-derived factors (27). Therefore, tumor self-seeding might explain the relationships among anaplasia, tumor size, vascularity, and prognosis and also suggests that local recurrences may result from seeding of disseminated cells following complete tumor excision (1, 42, 43).
CTMs were isolated from a single patient (Figure 3 and Table 2) and were positive for β-catenin and vimentin expressions. Interestingly, it was in a 57-year-old woman whose diagnosis was in 2013 with the blood collection in this study made in September 2019 and who has not yet progressed. She has received only one treatment line (tamoxifen + celecoxib). Knowledge regarding CTMs, also called CTC clusters, has evolved over the last decade, and increasing evidence suggests that they play an essential role on the pathophysiology of metastasis (44). Preclinical and clinical studies exploiting other cancers have demonstrated that CTMs are associated with increased metastatic potential and poor prognosis (36, 45–48). Hayashi et al. detected CTMs in sarcoma patients using CellSieve™, a low-pressure microfiltration device. Our group previously isolated CTMs in sarcoma patients using ISET® but did not address DTs (41). Taking this into account, together with our current data, it is reasonable to hypothesize that the inability of DTs to metastasize can be explained in part by their failure to generate CTMs (as we rarely found CTMs in our cohort of 18 patients). However, this hypothesis needs to be interpreted cautiously, due to the small sample size of our cohort.
β-Catenin is broadly used for DT diagnosis. In line with this, we thought that this protein could also be expressed in CTCs. Analysis of β-catenin protein expression in CTCs showed 35.7% of concordance with primary tumors (considering both i) patients from whom reports of the primary tumor were available and ii) those patients in whom the spots analyzed for β-catenin did not present CTCs). β-Catenin is encoded by the CTNNB1 gene in humans and is involved in cell signaling, acting primarily as a transcription factor. It has an essential role on developmental biology and cell adhesion. Mutations and overexpression of this gene are associated with several types of cancers, such as lung, breast, ovarian, endometrial, hepatocellular, and colorectal carcinomas (19–21, 49). We also identified vimentin expression in the CTCs of five patients (27.7%). Vimentin is required for plasticity of mesenchymal cells under normal physiological conditions and migration of cancer cells that have undergone epithelial/mesenchymal transition. In a previous study of sarcoma CTCs conducted by our group, we detected vimentin in CTCs of three patients in a cohort of 11 (29).
TGF-β is a secreted cytokine that regulates cell migration, differentiation, and proliferation (50). Depending on its expression level, TGF‐β has both proangiogenic and antiangiogenic properties. Angiogenic factors (vascular endothelial growth factor and basic fibroblast growth factor) increase TGF‐β expression when its levels are low. At high levels, TGF-β rebuilds basement membrane and inhibits the growth of endothelial cells and smooth muscle cells (51, 52). In this study, curiously, nine patients (50%) were positive for TGFβ-RI expression in CTCs. In another study by our group (37), TGF-βRI expression was associated with poor prognosis of locally advanced head and neck cancer. The identification of TGF-βRI in CTCs opens paths for the comprehensive exploitation of this pathway in DT.
COX enzymes play important roles on human physiology and various pathological conditions (53, 54). There is evidence for the critical involvement of COX-2 in many pathologies, including cancer (55, 56). Here, we also found a high frequency of DT patients expressing COX-2 in CTCs. Considering that DTs do not form metastases but show aggressive local invasion, it is possible that COX-2 plays an essential role on this process, which needs to be further investigated.
In general, we found that β-catenin was highly expressed in CTCs. Except for patient #6, we also found that at least one marker was expressed by CTCs across the whole cohort analyzed in this study, suggesting that evolution of protein expression in CTCs might be used as a biomarker that allows the non-invasive diagnosis of DT patients.
In this study, we showed that CTCs are composed of highly heterogeneous cell populations with very different phenotypes. This difference in phenotypes makes the identification of CTCs challenging, due to their similarities with other cells of the immune system, such as giant monocytes and micromegakaryocytes. However, training in analysis with the help of cytopathological criteria can overcome these difficulties. Laget et al. (30) described results that consistently show the feasibility of isolating live and fixed tumor cells with a lower limit of detection (LLOD) of a cancer cell per 10 ml of blood and an LLOD sensitivity ranging from 83% to 100%. Those results demonstrated that ISET® allows highly sensitive and impartial isolation of fixed tumor cells from blood for reliable identification of CTCs, as well as the development of immuno-molecular studies. Here, we were able to demonstrate the expression of mesenchymal proteins in CTCs by ICC, which are probably involved in tumorigenesis process.
To conclude, our study opens the prospect of using CTCs to predict desmoid dynamics throughout the course of the disease. In addition, it demonstrates that DTs release CTCs, opening new avenues for studying the biology of this tumor and improving our understanding of its high local relapse rates without distant metastasis. Additional studies with larger sample sizes should be conducted to validate our findings and explore the mechanism of DT development and progression.
The datasets presented in this study can be found in online repositories. The names of the repository/repositories and accession number(s) can be found in the article/Supplementary Material.
The studies involving human participants were reviewed and approved by AC Camargo Cancer Center. The patients/participants provided their written informed consent to participate in this study.
AB: design, data analysis and interpretation, manuscript writing and collection and/or assembly of data. FC, MS and FP: clinical survey/review and writing. EA and AR: collection and/or assembly of data. TM: English review. CM: conception/design and writing of the clinical part of the manuscript. LC: conception/design, data analysis and interpretation, manuscript writing, final approval of manuscript. All authors contributed to the article and approved the submitted version.
We thank the National Institute for Science and Tecnology in Oncogenomics and Therapeutic Innovation (INCT) for financial support for this study. ACB had a PhD fellowship from São Paulo Research Foundation FAPESP (2019/18100-8).
The authors declare that the research was conducted in the absence of any commercial or financial relationships that could be construed as a potential conflict of interest.
All claims expressed in this article are solely those of the authors and do not necessarily represent those of their affiliated organizations, or those of the publisher, the editors and the reviewers. Any product that may be evaluated in this article, or claim that may be made by its manufacturer, is not guaranteed or endorsed by the publisher.
The Supplementary Material for this article can be found online at: https://www.frontiersin.org/articles/10.3389/fonc.2021.622626/full#supplementary-material
Supplementary Figure 1 | (A, B) Positive control, A549 cell line “spiked” in healthy blood and stained for β-catenin and TGF β-RI respectively. (C, D) Positive control, U-87 cell line “spiked” in healthy blood and stained for COX-2 and Vimentin respectively. (E) Negative control, A-549 cell line “spiked” in healthy blood and negative for β-catenin. Cells were analyzed by light microscopy (BX61-Olympus).
Supplementary Figure 2 | (A–I) Haematoxylin stained cells. Examples of isolated desmoid tumor CTCs with characteristic cytomorphological features (nucleus size ≥ 12 µm, hyperchromatic and irregular nucleus, visible presence of cytoplasm, and a high nucleus–cytoplasm ratio (31). White asterisk: leukocytes. Black asterisks: ISET membrane pore.
Supplementary Figure 3 | (A) Leukocytes from desmoid tumor patient. (B, C) Examples of macrophages isolated from the blood of patients with desmoid tumor. In (B), a macrophage stained with COX-2. In (C), a macrophage visualized with haematoxylin.
1. Wu C, Nik-Amini S, Nadesan P, Stanford WL, Alman BA. Aggressive Fibromatosis (Desmoid Tumor) Is Derived From Mesenchymal Progenitor Cells. Cancer Res (2010) 70(19):7690–8. doi: 10.1158/0008-5472.CAN-10-1656
2. Ratan R, Roland CL BA. Desmoid Fibromatosis: Management in an Era of Increasing Options. Curr Oncol Rep (2021) 23(4):41. doi: 10.1007/s11912-021-01026-w
3. de Camargo VP, Keohan ML, D’Adamo DR, Antonescu CR, Brennan MF, Singer S, et al. Clinical Outcomes of Systemic Therapy for Patients With Deep Fibromatosis (Desmoid Tumor). Cancer (2010) 116(9):2258–65. doi: 10.1002/cncr.25089
4. Penel N, Coindre J-M, Bonvalot S, Italiano A, Neuville A, Le Cesne A, et al. Management of Desmoid Tumours: A Nationwide Survey of Labelled Reference Centre Networks in France. Eur J Cancer (2016) 58:90–6. doi: 10.1016/j.ejca.2016.02.008
5. Fiore M, MacNeill A, Gronchi A, Colombo C. Desmoid-Type Fibromatosis. Surg Oncol Clin N Am (2016) 25(4):803–26. doi: 10.1016/j.soc.2016.05.010
6. Garcia-Ortega DY, Martín-Tellez KS, Cuellar-Hubbe M, Martínez-Said H, Álvarez-Cano A, Brener-Chaoul M, et al. Desmoid-Type Fibromatosis. Cancers (Basel) (2020) 12(7):1851. doi: 10.3390/cancers12071851
7. Penel N, Chibon F, Salas S. Adult Desmoid Tumors: Biology, Management and Ongoing Trials. Curr Opin Oncol (2017) 29(4):268–74. doi: 10.1097/CCO.0000000000000374
8. Group DTW The Management of Desmoid Tumours: A Joint Global Consensus-Based Guideline Approach for Adult and Paediatric Patients. Eur J Cancer (2020) 127:96–107. doi: 10.1016/j.ejca.2019.11.013
9. Peng PD, Hyder O, Mavros MN, Turley R, Groeschl R, Firoozmand A, et al. Management and Recurrence Patterns of Desmoids Tumors: A Multi-Institutional Analysis of 211 Patients. Ann Surg Oncol (2012) 19(13):4036–42. doi: 10.1245/s10434-012-2634-6
10. Kotiligam D, Lazar AJF, Pollock RE, Lev D. Desmoid Tumor: A Disease Opportune for Molecular Insights. Histol Histopathol (2008) 23(1):117–26. doi: 10.14670/HH-23.117
11. Owens CL, Sharma R, Ali SZ. Deep Fibromatosis (Desmoid Tumor). Cancer (2007) 111(3):166–72. doi: 10.1002/cncr.22689
12. Koskenvuo L, Ristimäki A, Lepistö A. Comparison of Sporadic and FAP-Associated Desmoid-Type Fibromatoses. J Surg Oncol (2017) 116(6):716–21. doi: 10.1002/jso.24699
13. Escobar C, Munker R, Thomas JO, Li BD, Burton GV. Update on Desmoid Tumors. Ann Oncol (2012) 23(3):562–9. doi: 10.1093/annonc/mdr386
14. Chilov D, Sinjushina N, Rita H, Taketo MM, Mäkelä TP, Partanen J. Phosphorylated β-Catenin Localizes to Centrosomes of Neuronal Progenitors and is Required for Cell Polarity and Neurogenesis in Developing Midbrain. Dev Biol (2011) 357(1):259–68. doi: 10.1016/j.ydbio.2011.06.029
15. Colombo C, Bolshakov S, Hajibashi S, Lopez-Terrada L, Wang W-L, Rao P, et al. ‘Difficult to Diagnose’ Desmoid Tumours: A Potential Role for CTNNB1 Mutational Analysis. Histopathology (2011) 59(2):336–40. doi: 10.1111/j.1365-2559.2011.03932.x
16. Dômont J, Salas S, Lacroix L, Brouste V, Saulnier P, Terrier P, et al. High Frequency of β-Catenin Heterozygous Mutations in Extra-Abdominal Fibromatosis: A Potential Molecular Tool for Disease Management. Br J Cancer (2010) 102(6):1032–6. doi: 10.1038/sj.bjc.6605557
17. Lazar AJF, Tuvin D, Hajibashi S, Habeeb S, Bolshakov S, Mayordomo-Aranda E, et al. Specific Mutations in the β-Catenin Gene (CTNNB1) Correlate With Local Recurrence in Sporadic Desmoid Tumors. Am J Pathol (2008) 173(5):1518–27. doi: 10.2353/ajpath.2008.080475
18. Braggio D, Zewdu A, Londhe P, Yu P, Lopez G, Batte K, et al. β-Catenin S45F Mutation Results in Apoptotic Resistance. Oncogene (2020) 39(34):5589–600. doi: 10.1038/s41388-020-1382-5
19. Lecarpentier Y, Schussler O, Hébert J-L, Vallée A. Multiple Targets of the Canonical WNT/β-Catenin Signaling in Cancers. Front Oncol (2019) 9:1–17. doi: 10.3389/fonc.2019.01248
20. Liu Y, Patel L, Mills GB, Lu KH, Sood AK, Ding L, et al. Clinical Significance of CTNNB1 Mutation and Wnt Pathway Activation in Endometrioid Endometrial Carcinoma. JNCI J Natl Cancer Inst (2014) 106(9):1–8. doi: 10.1093/jnci/dju245
21. Garcia-Rostan G, Camp RL, Herrero A, Carcangiu ML, Rimm DL, Tallini G. β-Catenin Dysregulation in Thyroid Neoplasms. Am J Pathol (2001) 158(3):987–96. doi: 10.1016/S0002-9440(10)64045-X
22. Pantel K, Alix-Panabières C. Cell Lines From Circulating Tumor Cells. Oncoscience [Internet] (2015) 202(10):815–6. doi: 10.18632/oncoscience.195
23. Alix-Panabières C, Pantel K. Circulating Tumor Cells: Liquid Biopsy of Cancer. Clin Chem (2013) 59(1):110–8. doi: 10.1373/clinchem.2012.194258
24. Pantel K, Brakenhoff RH. Dissecting the Metastatic Cascade. Nat Rev Cancer (2004) 4(6):448–56. doi: 10.1038/nrc1370
25. Massagué J, Obenauf AC. Metastatic Colonization by Circulating Tumour Cells. Nature (2016) 529(7586):298–306. doi: 10.1038/nature17038
26. Paterlini-Brechot P, Benali NL. Circulating Tumor Cells (CTC) Detection: Clinical Impact and Future Directions. Cancer Lett (2007) 253(2):180–204. doi: 10.1016/j.canlet.2006.12.014
27. Kim M-Y, Oskarsson T, Acharyya S, Nguyen DX, Zhang XH-F, Norton L, et al. Tumor Self-Seeding by Circulating Cancer Cells. Cell (2009) 139(7):1315–26. doi: 10.1016/j.cell.2009.11.025
28. Chang L, Asatrian G, Dry SM, James AW. Circulating Tumor Cells in Sarcomas: A Brief Review. Med Oncol (2015) 32(1):430. doi: 10.1007/s12032-014-0430-9
29. Chinen L, Mello C, Abdallah E, Ocea L, Buim M, Mingues N, et al. Isolation, Detection, and Immunomorphological Characterization of Circulating Tumor Cells (CTCs) From Patients With Different Types of Sarcoma Using Isolation by Size of Tumor Cells: A Window on Sarcoma-Cell Invasion. Onco Targets Ther (2014) 7:1609–17. doi: 10.2147/OTT.S62349
30. Laget S, Broncy L, Hormigos K, Dhingra DM, BenMohamed F, Capiod T, et al. Technical Insights Into Highly Sensitive Isolation and Molecular Characterization of Fixed and Live Circulating Tumor Cells for Early Detection of Tumor Invasion. PloS One (2017) 12(1):e0169427. doi: 10.1371/journal.pone.0169427
31. Krebs MG, Hou J-M, Sloane R, Lancashire L, Priest L, Nonaka D, et al. Analysis of Circulating Tumor Cells in Patients With Non-Small Cell Lung Cancer Using Epithelial Marker-Dependent and -Independent Approaches. J Thorac Oncol (2012) 7(2):306–15. doi: 10.1097/JTO.0b013e31823c5c16
32. Chinen LTD, de Carvalho FM, Rocha BMM, Aguiar CM, Abdallah EA, Campanha D, et al. Cytokeratin-Based CTC Counting Unrelated to Clinical Follow Up. J Thorac Dis (2013) 5(5):593–9. doi: 10.3978/j.issn.2072-1439.2013.09.18
33. Abdallah EA, Fanelli MF, Buim MEC, Machado Netto MC, Gasparini Junior JL, Souza e Silva V, et al. Thymidylate Synthase Expression in Circulating Tumor Cells: A New Tool to Predict 5-Fluorouracil Resistance in Metastatic Colorectal Cancer Patients. Int J Cancer (2015) 137(6):1397–405. doi: 10.1002/ijc.29495
34. Buim ME, Fanelli MF, Souza VS, Romero J, Abdallah EA, Mello C AL, et al. Detection of KRAS Mutations in Circulating Tumor Cells From Patients With Metastatic Colorectal Cancer. Cancer Biol Ther (2015) 16(9):1289–95. doi: 10.1080/15384047.2015.1070991
35. Abdallah EA, Fanelli MF, Souza e Silva V, Machado Netto MC, Gasparini Junior JL, Araújo DV, et al. MRP1 Expression in CTCs Confers Resistance to Irinotecan-Based Chemotherapy in Metastatic Colorectal Cancer. Int J Cancer (2016) 139(4):890–8. doi: 10.1002/ijc.30082
36. Corassa M, Fanelli MF, Oliveira TB, Mello CL, Machado Netto MC, Nicolau UR, et al. Circulating Tumor Microemboli as a Prognostic Factor for Locally Advanced or Metastatic Solid Tumors. J Clin Oncol (2016) 34(15_suppl):e23067–7. doi: 10.1200/JCO.2016.34.15_suppl.e23067
37. Fanelli MF, Oliveira TB, Braun AC, Corassa M, Abdallah EA, Nicolau UR, et al. Evaluation of Incidence, Significance, and Prognostic Role of Circulating Tumor Microemboli and Transforming Growth Factor-β Receptor I in Head and Neck Cancer. Head Neck (2017) 39(11):2283–92. doi: 10.1002/hed.24899
38. Abdallah EA, Braun AC, Flores BCTCP, Senda L, Urvanegia AC, Calsavara V, et al. The Potential Clinical Implications of Circulating Tumor Cells and Circulating Tumor Microemboli in Gastric Cancer. Oncologist (2019) 24(9):1–10. doi: 10.1634/theoncologist.2018-0741
39. Troncarelli Flores BC, Souza e Silva V, Ali Abdallah E, Mello CAL, Gobo Silva ML, Gomes Mendes G, et al. Molecular and Kinetic Analyses of Circulating Tumor Cells as Predictive Markers of Treatment Response in Locally Advanced Rectal Cancer Patients. Cells (2019) 8(7):641. doi: 10.3390/cells8070641
40. Abdallah EA, Souza e Silva V, Braun AC, Gasparini VA, Kupper BEC, Tariki MS, et al. A Higher Platelet-To-Lymphocyte Ratio is Prevalent in the Presence of Circulating Tumor Microemboli and is a Potential Prognostic Factor for Non-Metastatic Colon Cancer. Transl Oncol (2021) 14(1):100932. doi: 10.1016/j.tranon.2020.100932
41. Braun AC, de Mello CAL, Corassa M, Abdallah EA, Urvanegia AC, Alves VS, et al. EGFR Expression in Circulating Tumor Cells From High-Grade Metastatic Soft Tissue Sarcomas. Cancer Biol Ther (2018) 19(6):454–60. doi: 10.1080/15384047.2018.1433498
42. Sakorafas GH, Nissotakis C, Peros G. Abdominal Desmoid Tumors. Surg Oncol (2007) 16(2):131–42. doi: 10.1016/j.suronc.2007.07.009
43. Nieuwenhuis MH, Casparie M, Mathus-Vliegen LMH, Dekkers OM, Hogendoorn PCW, Vasen HFA. A Nation-Wide Study Comparing Sporadic and Familial Adenomatous Polyposis-Related Desmoid-Type Fibromatoses. Int J Cancer (2011) 129(1):256–61. doi: 10.1002/ijc.25664
44. Amintas S, Bedel A, Moreau-Gaudry F, Boutin J, Buscail L, Merlio J-P, et al. Circulating Tumor Cell Clusters: United We Stand Divided We Fall. Int J Mol Sci (2020) 21(7):2653. doi: 10.3390/ijms21072653
45. Aceto N, Bardia A, Miyamoto DT, Donaldson MC, Wittner BS, Spencer JA, et al. Circulating Tumor Cell Clusters Are Oligoclonal Precursors of Breast Cancer Metastasis. Cell (2014) 158(5):1110–22. doi: 10.1016/j.cell.2014.07.013
46. Hou J-M, Krebs MG, Lancashire L, Sloane R, Backen A, Swain RK, et al. Clinical Significance and Molecular Characteristics of Circulating Tumor Cells and Circulating Tumor Microemboli in Patients With Small-Cell Lung Cancer. J Clin Oncol (2012) 30(5):525–32. doi: 10.1200/JCO.2010.33.3716
47. Zhang D, Zhao L, Zhou P, Ma H, Huang F, Jin M, et al. Circulating Tumor Microemboli (CTM) and Vimentin+ Circulating Tumor Cells (CTCs) Detected by a Size-Based Platform Predict Worse Prognosis in Advanced Colorectal Cancer Patients During Chemotherapy. Cancer Cell Int (2017) 17(1):6. doi: 10.1186/s12935-016-0373-7
48. Wu G, Zhu R, Li Y, Zhao Y, Dai M. Prognostic Significance of Circulating Tumor Microemboli in Patients With Pancreatic Ductal Adenocarcinoma. Oncol Lett (2018) 5:7376–82. doi: 10.3892/ol.2018.8264
49. Cieply B, Zeng G, Proverbs-Singh T, Geller DA, Monga SPS. Unique Phenotype of Hepatocellular Cancers With Exon-3 Mutations in Beta-Catenin Gene. Hepatology (2009) 49(3):821–31. doi: 10.1002/hep.22695
50. Micalizzi DS, Farabaugh SM, Ford HL. Epithelial-Mesenchymal Transition in Cancer: Parallels Between Normal Development and Tumor Progression. J Mammary Gland Biol Neoplasia (2010) 15(2):117–34. doi: 10.1007/s10911-010-9178-9
51. Neuzillet C, Tijeras-Raballand A, Cohen R, Cros J, Faivre S, Raymond E, et al. Targeting the Tgfβ Pathway for Cancer Therapy. Pharmacol Ther (2015) 147:22–31. doi: 10.1016/j.pharmthera.2014.11.001
52. Sakurai T, Kudo M. Signaling Pathways Governing Tumor Angiogenesis. Oncology (2011) 81(s1):24–9. doi: 10.1159/000333256
53. Smith WL, Urade Y, Jakobsson P-J. Enzymes of the Cyclooxygenase Pathways of Prostanoid Biosynthesis. Chem Rev (2011) 111(10):5821–65. doi: 10.1021/cr2002992
54. Marnett LJ. The COXIB Experience: A Look in the Rearview Mirror. Annu Rev Pharmacol Toxicol (2009) 49(1):265–90. doi: 10.1146/annurev.pharmtox.011008.145638
55. Wang D, DuBois RN. The Role of Anti-Inflammatory Drugs in Colorectal Cancer. Annu Rev Med (2013) 64(1):131–44. doi: 10.1146/annurev-med-112211-154330
Keywords: circulating tumor cells, desmoid tumor, beta catenin expression, vimentin expression, TGF-βRI expression
Citation: Braun AC, Campos FAB, Abdallah EA, Ruano APC, Medina TS, Tariki MS, Pinto FFE, de Mello CAL and Chinen LTD (2021) Circulating Tumor Cells in Desmoid Tumors: New Perspectives. Front. Oncol. 11:622626. doi: 10.3389/fonc.2021.622626
Received: 28 October 2020; Accepted: 13 August 2021;
Published: 14 September 2021.
Edited by:
George Calin, University of Texas MD Anderson Cancer Center, United StatesReviewed by:
James Reuben, University of Texas MD Anderson Cancer Center, United StatesCopyright © 2021 Braun, Campos, Abdallah, Ruano, Medina, Tariki, Pinto, de Mello and Chinen. This is an open-access article distributed under the terms of the Creative Commons Attribution License (CC BY). The use, distribution or reproduction in other forums is permitted, provided the original author(s) and the copyright owner(s) are credited and that the original publication in this journal is cited, in accordance with accepted academic practice. No use, distribution or reproduction is permitted which does not comply with these terms.
*Correspondence: Alexcia C. Braun, YWxleGNpYWJyYXVuQGdtYWlsLmNvbQ==; Ludmilla T. D. Chinen, bHRkY2hpbmVuQGdtYWlsLmNvbQ==
Disclaimer: All claims expressed in this article are solely those of the authors and do not necessarily represent those of their affiliated organizations, or those of the publisher, the editors and the reviewers. Any product that may be evaluated in this article or claim that may be made by its manufacturer is not guaranteed or endorsed by the publisher.
Research integrity at Frontiers
Learn more about the work of our research integrity team to safeguard the quality of each article we publish.