- Department of Radiation Oncology, Medical College of Wisconsin, Milwaukee, WI, United States
Summary: Skin cancer patients may be treated definitively using radiation therapy (RT) with electrons, kilovoltage, or megavoltage photons depending on tumor stage and invasiveness. This study modeled tumor control probability (TCP) based on the pooled clinical outcome data of RT for primary basal and cutaneous squamous cell carcinomas (BCC and cSCC, respectively). Four TCP models were developed and found to be potentially useful in developing optimal treatment schemes based on recommended ASTRO 2020 Skin Consensus Guidelines for primary, keratinocyte carcinomas (i.e. BCC and cSCC).
Background: Radiotherapy (RT) with electrons or photon beams is an excellent primary treatment option for keratinocyte carcinoma (KC), particularly for non-surgical candidates. Our objective is to model tumor control probability (TCP) based on the pooled clinical data of primary basal and cutaneous squamous cell carcinomas (BCC and cSCC, respectively) in order to optimize treatment schemes.
Methods: Published reports citing crude estimates of tumor control for primary KCs of the head by tumor size (diameter: ≤2 cm and >2 cm) were considered in our study. A TCP model based on a sigmoidal function of biological effective dose (BED) was proposed. Three-parameter TCP models were generated for BCCs ≤2 cm, BCCs >2cm, cSCCs ≤2 cm, and cSCCs >2 cm. Equivalent fractionation schemes were estimated based on the TCP model and appropriate parameters.
Results: TCP model parameters for both BCC and cSCC for tumor sizes ≤2 cm and >2cm were obtained. For BCC, the model parameters were found to be TD50 = 56.62 ± 6.18 × 10-3 Gy, k = 0.14 ± 2.31 × 10−2 Gy−1 and L = 0.97 ± 4.99 × 10−3 and TD50 = 55.78 ± 0.19 Gy, k = 1.53 ± 0.20 Gy−1 and L = 0.94 ± 3.72 × 10−3 for tumor sizes of ≤2 cm and >2 cm, respectively. For SCC the model parameters were found to be TD50 = 56.81 ± 19.40 × 104 Gy, k = 0.13 ± 7.92 × 104 Gy−1 and L = 0.96 ± 1.31 × 10-2 and TD50 = 58.44 ± 0.30 Gy, k = 2.30 ± 0.43 Gy−1 and L = 0.91± 1.22 × 10−2 for tumors ≤2cm and >2 cm, respectively. The TCP model with the derived parameters predicts that radiation regimens with higher doses, such as increasing the number of fractions and/or dose per fraction, lead to higher TCP, especially for KCs >2 cm in size.
Conclusion: Four TCP models for primary KCs were developed based on pooled clinical data that may be used to further test the recommended kV and MV x-ray and electron RT regimens from the 2020 ASTRO guidelines. Increasing both number of fractions and dose per fraction may have clinically significant effects on tumor control for tumors >2 cm in size for both BCC and cSCC.
Introduction
Skin cancer is the most common form of cancer in the United States with an annual incidence of five million cases (1). Keratinocyte carcinoma (KC) consists of basal and cutaneous squamous cell carcinomas (BCCs and cSCCs, respectively) are the most common forms of skin cancers [more commonly known as non-melanoma skin cancers (NMSC)] (2) and account for over 95% of skin cancer diagnoses (3). Definitive radiotherapy (RT) using kilovoltage (kV) x-rays, megavoltage electrons (MeV), and megavoltage (MV) x-rays, especially for deeply invasive KC, is an excellent treatment option for skin cancer, particularly as an alternative to surgery in non-operative candidates, when there are cosmetic concerns with surgery or when patients refuse surgery. Reported tumor control rates using definitive RT for BCCs and cSCCs are upwards of 90−95% and are comparable to surgical resection (4–6). However, the benefit of RT may depend on tumor size: control rates for smaller tumors (≤2 cm) greatly exceed 90% while control rates for large (>2 cm) may be as low as 60−70% (4–6). A variety of dose and fractionation schemes for definitive RT of BCCs and cSCCs are reported in the literature. The RT techniques used in these studies mostly pre-date the era of image guided radiation and thus employed large treatment margins. The ASTRO 2020 Skin Consensus Guidelines was published in early 2020 and recommended various treatment scenarios for NMSC that include RT delivery technique and fractionation considerations for primary RT and post-operative scenarios, etc. (3) The purpose of this work is to add to this recent report by modeling tumor control probability (TCP) based on available clinical outcome data of definitive RT kV photons, MV photons and MeV electrons for BCC and cSCC in order to optimize treatment schedules based on tumor size using modern conformal RT techniques.
Materials and Methods
A literature review was performed using the Preferred Reporting Items for Systematic Reviews and Meta-Analyses (PRISMA) procedure described by Moher et al. (7) (Figure 1) using the search criteria and the references within the recent ASTRO guidelines (3). A second search was subsequently performed using a PubMed search with search string “radiotherapy AND basal cell carcinoma AND squamous cell carcinoma,” and a date range from 1/1980 to 6/2020. Next, an additional search of articles referencing those articles found in the recent ASTRO guidelines and the PubMed search was subsequently performed. Reports citing crude estimates of primary tumor site recurrence rate (RR, i.e. number recurred in the field divided by the total number of BCC or cSCC patients) for primary BCCs and cSCCs of the head, head and neck region, or extremities by tumor size (i.e. tumor diameter) were considered for use in developing our TCP model. The modality considered in our analysis consisted of using kV photons available on superficial and orthovoltage machines, as well as MV photons and electrons typically available on linear accelerators. The TCP was subsequently calculated as TCPliterature = 1 – RR. Our model was based on the following phenomenological sigmoidal equation (8):
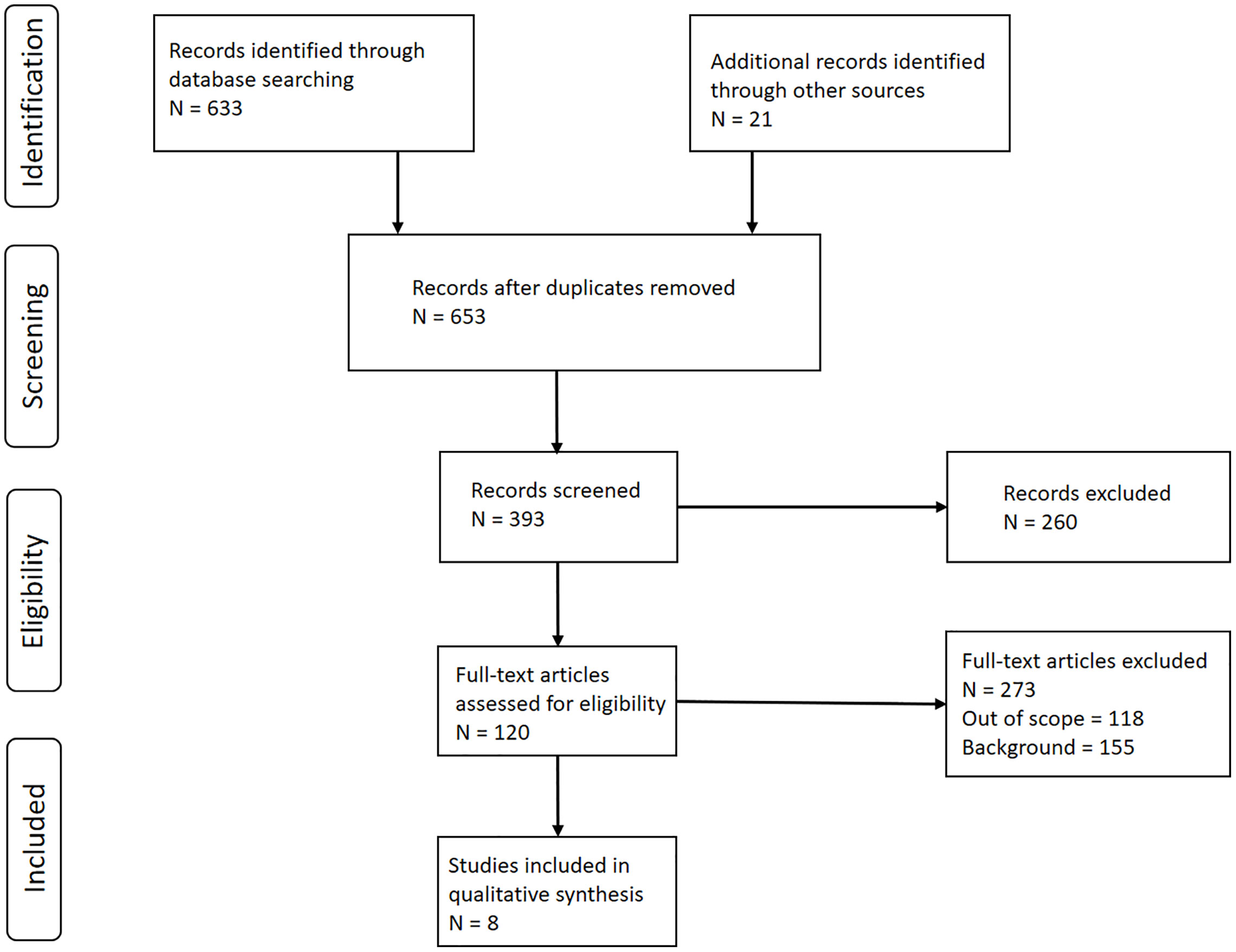
Figure 1 Preferred Reporting Items for Systematic Reviews and Meta-Analysis (PRISMA) Diagram. Schematic depicting the literature review process for the development of basal and cutaneous squamous cell carcinoma (BCC and cSCC, respectively) tumor control probability (TCP) models. Created based on Moher D, A Liberati, J Tetzlaff and DC Altman, 2009 [ref (7)].
where D is the total prescribed dose, d is the dose per fraction (Gy/fx), α and β are linear quadratic (LQ) model radiobiological parameters (we use α/β = 10 Gy, in line with the ASTRO guidelines), TD50 is the dose required to achieve 50% tumor control, k is a fitting constant related to the slope of the dose response curve at TD50, and L is a logistic function maximum value or level. The values for D and d used were the mean values reported in the manuscript for the patient cohort of each tumor type and size (e.g. BCC ≤2 cm in diameter). Our analysis considered TD50, k and L as fitting parameters and were estimated using the chi-squared fitting method by minimization of the following equation:
where M = number of data points, and are the TCP of the model and report, respectively, for the ith data point, while is the statistical error in the ith data point. The statistical error was calculated using the following (9):
where Ni is the number of responders that did not recur for the ith data point (or alternatively the number of responders controlled at the time the data point was obtained), σRR,i. is the uncertainty of the response rate of the ith data point, RRliterature is the response rate of the ith data point, dTCPliterature/dRR is the derivative of the tumor control probability with respect to the recurrence rate (i.e. this quantity evaluates to −1). Optimization was performed and standard errors in parameter estimates were obtained using Matlab (MathWorks, Natick, MA). Additional analysis was performed by calculating the TCP for each of the four models using suggested dose-fractionation schemes from the recently published ASTRO Clinical Practice Guidelines using various treatment modalities including MeV electrons, kV photons and MV photons.
Results
Literature search yielded a total of eight reports useful for the development of a TCP model for BCC and cSCC for which tumor control could be specified based on tumor size ≤2 cm and >2 cm (Table 1) (4, 5, 10–15). Seven of these reports used orthovoltage and megavoltage photons/electrons for treatment (3, 4, 6–8), while van Hezewijk et al. exclusively used electrons (13). All of these reports provided response data for tumor sizes ≤2 cm and seven reports provided data for tumors >2 cm. The majority of the 120 reports were not included in the final analysis because they didn’t specify the radiation treatment schedule for their patient cohort by tumor size (i.e. tumor stage). If it was specified, that schedule was stated for the entire cohort and not further broken down by tumor size. The last major reason for rejection was that the report only specified treatment outcomes and radiation schedule for the entire patient population nor was it possible to discern the characteristics of the patient outcome and radiation schedule by tumor size.
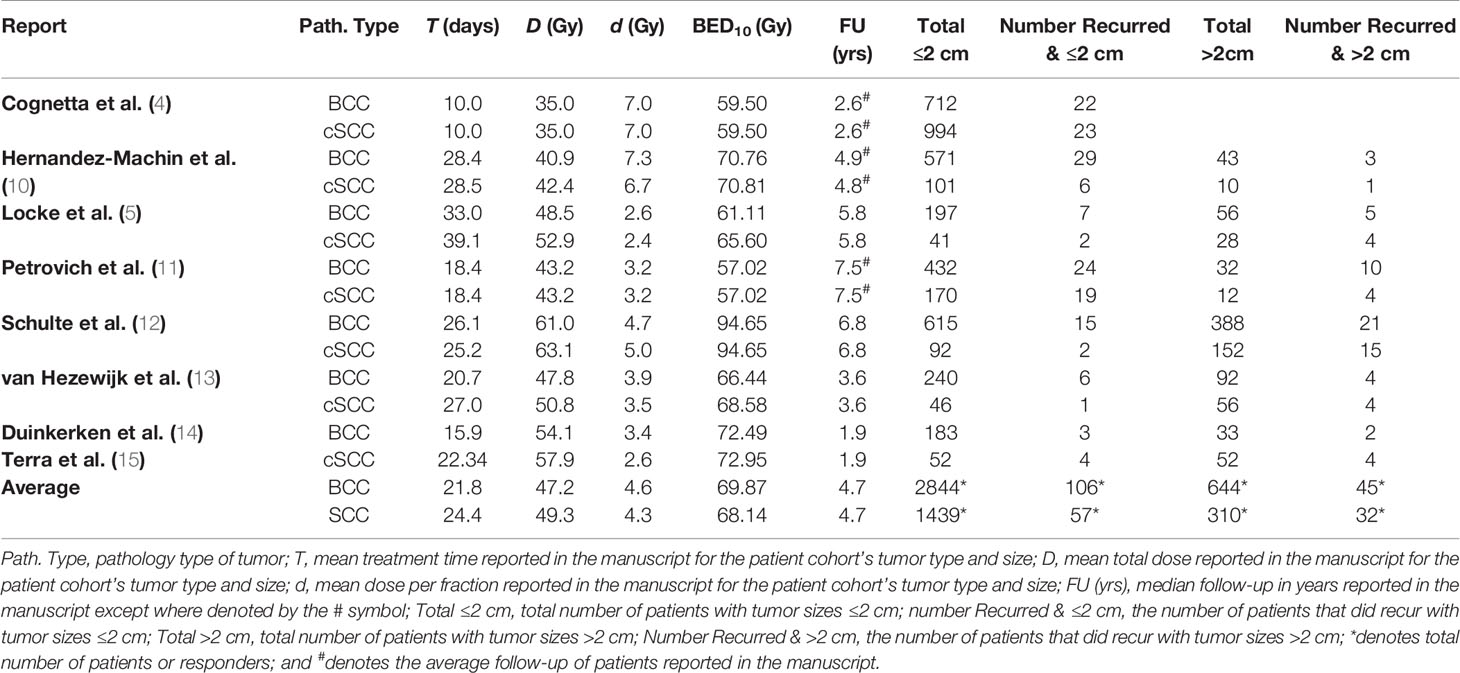
Table 1 Description of studies used in the development of a tumor control probability (TCP) model for basal and cutaneous squamous cell carcinomas (BCC and cSCC, respectively) by tumor size.
The numbers of recurrences were found to be 125 of 3,594 for BCC tumors ≤2 cm and 45 of 644 for BCC tumors >2 cm recurred. For the treatment of BCCs, the reported mean total radiation dose was 47.2 Gy (range: 35–61 Gy), the mean dose per fraction was 4.6 Gy/fx (range: 2.6–7.3 Gy/fx), [biological effective dose (BED) using LQ model with α/β = 10 Gy (BED10)] BED10 = 69.9 Gy, (range: 57.0–94.7 Gy)], and the mean total treatment time was 21.8 days (range: 10.0–39.1 days). The average follow-up time was 4.7 years (range: 1.9–7.5 years).
The number of recurrences was found to be 57 of 1,806 for cSCC tumors ≤2 cm, and 36 of 284 for cSCC tumors >2 cm recurred. For the treatment of cSCCs, the reported mean total dose was 49.3 Gy (range: 35–63.1 Gy), the mean dose per fraction was 4.3 Gy/fx (range: 2.4–7.0 Gy/fx), [BED10 = 68.1 Gy, (range: 59.5–94.7 Gy)], and mean total treatment time was 23.5 days (range: 6.0–39.2 days). The average follow-up time was 4.7 years (range: 1.9–7.5 years).
Four sets of model parameters for the TCP model (Eq. 1) were generated for (BCC and cSCC stratified by tumor size using a 2 cm cutoff (BCC ≤2cm, BCC >2cm, cSCC ≤2 cm, and cSCC >2 cm) (Table 2) assuming an α/β = 10 Gy. For BCC, the model parameters were found to be TD50 = 56.62 ± 6.18 × 10−3 Gy, k = 0.14 ± 2.31 × 10−2 Gy−1 and L = 0.97 ± 4.99 × 10−3 and TD50 = 55.78 ± 0.19 Gy, k = 1.53 ± 0.20 Gy−1 and L = 0.94 ± 3.72 × 10−3 for tumor sizes of ≤2 cm and >2 cm, respectively. For SCC the model parameters were found to be TD50 = 56.81 ± 19.4 × 104 Gy, k = 0.13 ± 7.92 × 104 Gy−1 and L = 0.96 ± 1.31 × 10−2 and TD50 = 58.44 ± 0.30 Gy, k = 2.30 ± 0.43 Gy−1 and L = 0.91 ± 1.22 × 10−2 for tumors ≤2 cm and >2 cm, respectively. The TCP response curves for BCC and cSCC tumors are depicted in Figure 2.
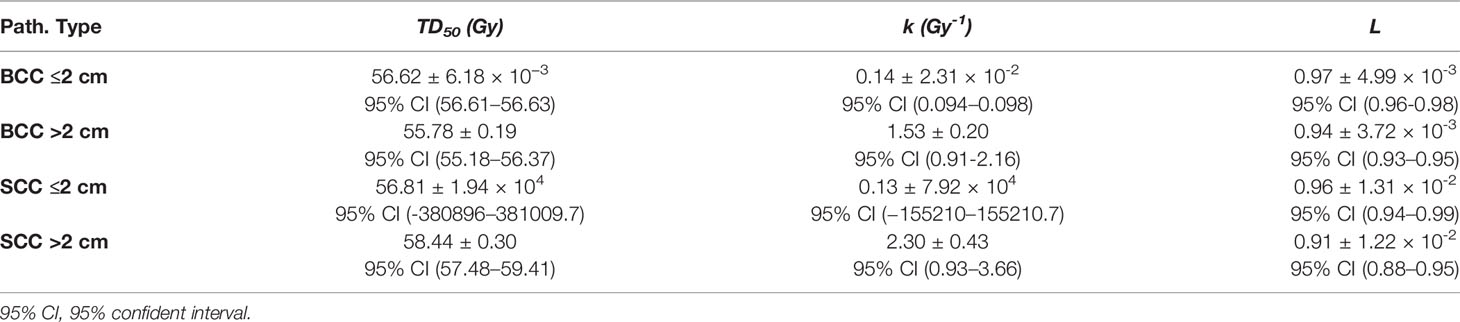
Table 2 Tumor control probability (TCP) model parameters for basal and cutaneous squamous cell carcinomas (BCC and cSCC, respectively) tumors of size ≤2 and >2 cm.
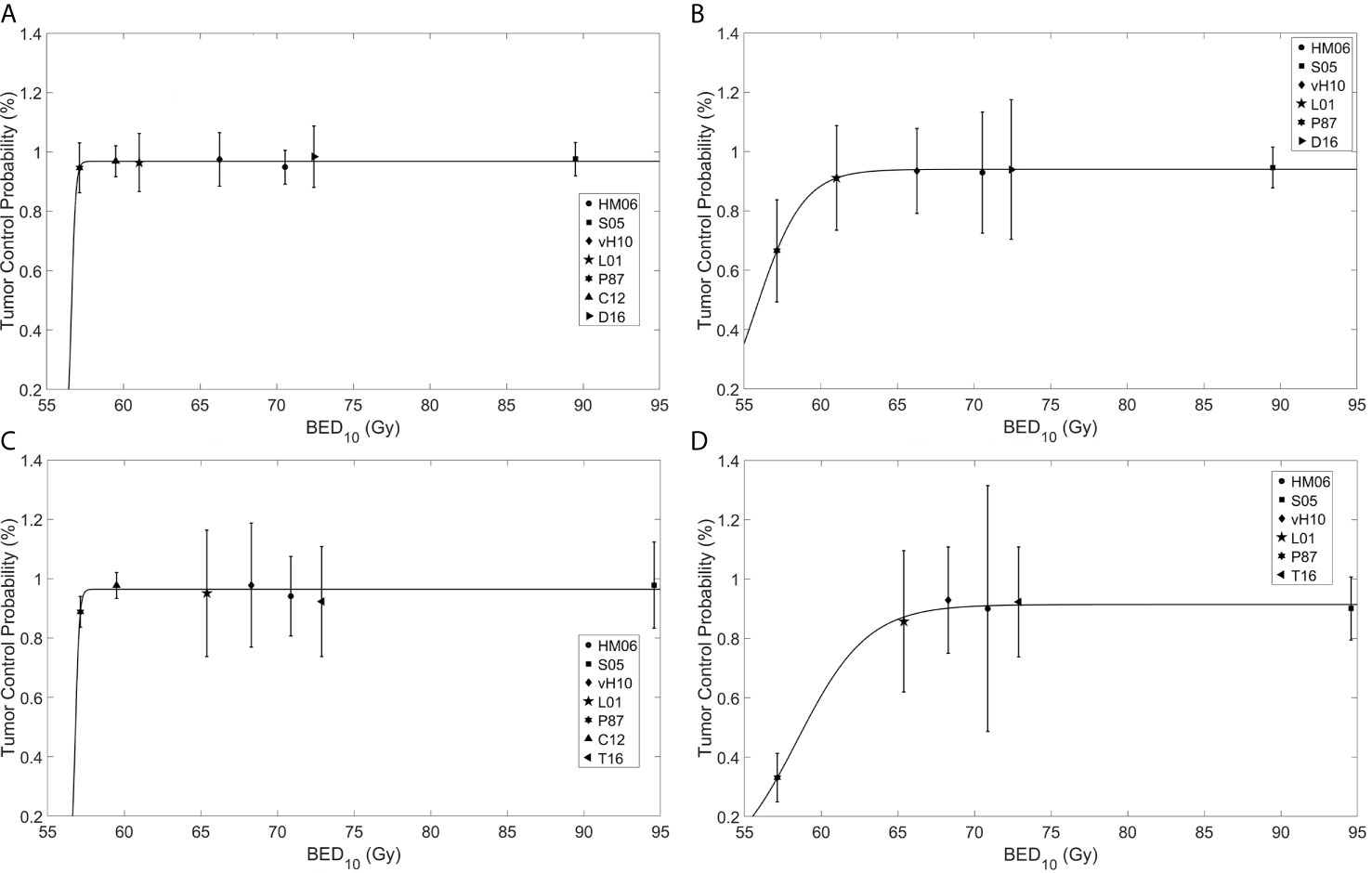
Figure 2 Fitting of a tumor control probability (TCP) model to reported clinical data for basal cell carcinoma (BCC) of size (A) ≤2 cm and (B) >2 cm, and cutaneous squamous cell carcinoma (cSCC) of size (C) ≤2 and (D) >2 cm. The abscissa is the biological effective dose calculated using the linear quadratic model with α/β = 10 Gy (BED10), while the ordinate is the TCP. HM06, Hernández-Machin et al., 2006 (10) (circle); S05, Schulte et al., 2005 (12) (square); VH10, van Hezewijk et al., 2010 (13) (diamond); L01, Locke et al., 2001 (5) (pentagram); P87, Petrovich et al., 1987 (11) (hexagram); Co12, Cognetta et al., 2012 (4) (upward pointing triangle); D16, Duinkerken et al., 2016 (14) (right pointing triangle); T16, Terra et al., 2016 (15) (left pointing triangle).
The TCP model was used to calculate the BED10 of different fractionation schemes (Table 3, reported in Appendix KQ4 of Likhacheva et al.). For example, a TCP of 95% or more for smaller sized tumors (i.e. ≤2 cm) could be achieved using a fractionation scheme of 15 treatments of 3 Gy per fraction (Gy/fx), regardless of tumor type. In contrast, larger sized tumors show lower tumor control for the same BED10 using the recommended fractionation schemes in Likhacheva et al. (e.g. 20 treatments of 2.35 Gy/fx). In contrast to smaller tumors, for tumors >2 cm, TCP varied by histological type; BCC tumors have higher TCP values compared to cSCC tumors of the same size and fractionation schedule (e.g. TCPBCC>2 = 0.765 and TCPSCC>2= 0.417 for 20 treatments of 2.35 Gy/fx).
Discussion
The projected increase in KC (formerly known as NMSC) incidence necessitates the development of outcome-based models to help design optimal schemes for RT treatment planning and clinical trials to further investigate the dose response relationship with tumor stage (1, 2). The TCP model along with appropriate parameters is the first to our knowledge to be developed for BCCs and cSCCs stratified by tumor size. This model was developed using crude estimates of tumor control from a literature review of patient populations treated with primary definitive RT for BCCs and cSCCs. Therefore, the model is not applicable in designing treatment schedules for patients treated for recurrent disease or in the post-operative setting. Additionally, the model would not be applicable for treatment sites other than the head and neck region since recurrent BCC and cSCC risk factors were developed using reports in the anatomical region (3). Nor would the model be applicable in situations where the appropriate margins were applied in creating the target volume [i.e. planning target volume (PTV)] for treatment (3, 16). However, our model would be useful in designing future prospective and randomized trials of KC in order to characterize the role of definitive RT in the management of this disease following the guidelines set forth in Likhacheva et al. (3).
Our model utilizes a phenomenological-based function for TCP with a small number of parameters that’s consistent with the KC clinical data, which could be viewed as a weakness. However, this is in contrast to a Poisson-based model, which would have more parameters leading to an overfitting of the data and have less predictive power given the small number of reports published over a large time period (17). Additionally, our models used the LQ model-derived BED to compare different RT fractionation regimes rather than physical dose and assumed an α/β of 10 Gy, which is consistent with the dose-fractionation analysis in the recent ASTRO guidelines (3). This seemed reasonable considering that Trott et al., Dale & Thomas et al. reported 95% confidence intervals in their estimates of KC α/β that included or were within 0.5 Gy of 10 Gy (18–20). We did not consider time in our model since Fitzpatrick et al. suggest that a reduction in total BED over the course of 1–2 months is insignificant for BCC and cSCC tumors (6). The reported models in our report may be useful in designing more comprehensive clinical trials to better illuminate dose–response by tumor size and/or stage, as well as develop more predictive TCP models of KC.
There are sources of uncertainty and other limitations to our modeling approach. The basis of much of the literature review was based on the ASTRO 2020 skin cancer guidelines, which used a time period of May 1988 to June 2018 on non-metastatic BCC and cSCC patients treated to curative intent (3). Our use of a wider time frame with basic keywords, as well as reviewing papers citing or cited by the references included in the guidelines, was to catch any manuscripts that may not have been included in the ASTRO consensus guidelines, which are literature search found two not included (11, 15). The patient population included KC patients treated definitively with kV photons, MeV electrons, and MV photons and excluded patients treated with high dose rate (HDR) brachytherapy despite the latter having similar local control and cosmetic outcomes as the former (3). Our rationale is that our model would be used as the basis of a clinical trial investigating the dose response of KCs by tumor stage, and kV and MV photons and MeV electrons are suggested for treating all tumor stages and commonly available on linear accelerators (MV photons and MeV electrons) and orthovoltage superficial machine (kV photons) that are used in radiation oncology and dermatological clinics (3). However, brachytherapy isn’t suggested for high T stage (hence large sized) tumors nor is it as commonly used as linear accelerators (3). Though with skin cancer rates projected to rise in the future, Cognetta et al. showed the kV photons could be useful tool in the dermatology clinic’s management of skin cancer in the increasing elderly and frail patient population (4). The wide range of follow-up times (i.e. 1.9–7.5 years) is a source of uncertainty in our analysis. The patient cohort in studies with shorter follow-up times may not have had sufficient time to reach stable outcome, particularly those with higher stage tumors (21). The use of the prescribed dose in our analysis represents another source of uncertainty given the reported associations of the minimum target dose and tumor control (22). Increasing tumor control by way increasing D and d should be done without exceeding normal tissue tolerances. Disregarding of normal tissue doses in the quest for improved tumor control could cause unacceptable toxicity as has been found in Hodgkin’s Lymphoma (23).
Conclusion
TCP models along with appropriate model parameters for primary BCC and cSCC tumors of size ≤2 cm and >2 cm were derived based on the best available clinical data, which may be used to develop alternative treatment schedules accounting for tumor size and utilizing modern RT technologies. The TCP calculations suggest that the recommended fractionation schedules in the ASTRO guidelines may be optimized to improve local control for tumor sizes larger than 2 cm. Based on the present work, larger sized BCC and cSCC tumors may be treated with higher fractionated doses. For example, for BCCs >2 cm, 16 (or more) treatments of 3 Gy/fx (or higher) in order to achieve a TCP of ~90% or higher. Similarly, cSCC tumors >2 cm, 20 or more treatments of 3 Gy in order to achieve a TCP of 90% or higher.
Data Availability Statement
The raw data supporting the conclusions of this article will be made available by the authors, without undue reservation.
Author Contributions
MA and JW provided clinical expertise on non-melanoma skin cancer. PP and XL developed clinically relevant TCP model in consultation with MA and JW. All authors contributed to the article and approved the submitted version.
Conflict of Interest
The authors declare that the research was conducted in the absence of any commercial or financial relationships that could be construed as a potential conflict of interest.
Acknowledgments
This work is supported partially by MCW Cancer Center Imaging Research Program Seed Grant and Meinerz Foundation.
This work was presented at the 2013 ASTRO annual conference as an oral presentation and the 2020 ASTRO annual conference as a poster presentation.
References
1. Medical Expenditure Panel Survey. Rockville, MD: Agency for Healthcare Research and Quality. Available at: https://meps.ahrq.gov/mepsweb/data_stats/download_data_files.jsp (Accessed February 12, 2020).
2. Nehal KS, Bichakjian CK. Update on Keratinocyte Carcinomas. N Engl J Med (2018) 379(4):363–74. doi: 10.1056/NEJMra1708701
3. Likhacheva A, Awan M, Barker CA, Bhatnager A, Bradfield L, Brady MS, et al. Definitive and Postoperative Radiation Therapy for Basal and Squamous Cell Cancers of the Skin: Executive Summary of an American Society for Radiation Oncology Clinical Practice Guideline. Pract Radiat Oncol (2020). doi: 10.1016/j.prro.2019.10.014
4. Cognetta AB, Howard BM, Heaton HP, Stoddard ER, Hong HG, Green WH. Superficial X-Ray in the Treatment of Basal and Squamous Cell Carcinomas: A Viable Option in Select Patients. J Am Acad Dermatol (2012) 67(6):1235–41. doi: 10.1016/j.jaad.2012.06.001
5. Locke J, Karimpour S, Young G, Lockett MA, Perez CA. Radiotherapy for Epithelial Skin Cancer. Int J Radiat Oncol Biol Phys (2001) 51(3):748–55. doi: 10.1016/s0360-3016(01)01656-x
6. Fitzpatrick PJ. Skin Cancer of the Head–Treatment by Radiotherapy. J Otolaryngol (1984) 13(4):261–6.
7. Moher D, Liberati A, Tetzlaff J, Altman DG. Preferred Reporting Items for Systematic Reviews and Meta-Analyses: The PRISMA Statement. BMJ (2009) 339:b2535. doi: 10.1136/bmj.b2535
8. Scheenstra AEH, Rossi MMG, Belderbos JSA, Damen EMF, Lebesque JV, Sonke J-J. Local Dose-Effect Relations for Lung Perfusion Post Stereotactic Body Radiotherapy. Radiother Oncol J Eur Soc Ther Radiol Oncol (2013) 107(3):398–402. doi: 10.1016/j.radonc.2013.04.003
9. Mathew A, Pandey M, Murthy NS. Survival Analysis: Caveats and Pitfalls. Eur J Surg Oncol J Eur Soc Surg Oncol Br Assoc Surg Oncol (1999) 25(3):321–9. doi: 10.1053/ejso.1998.0650
10. Hernández-Machin B, Borrego L, Gil-García M, Hernández BH. Office-Based Radiation Therapy for Cutaneous Carcinoma: Evaluation of 710 Treatments. Int J Dermatol (2007) 46(5):453–9. doi: 10.1111/j.1365-4632.2006.03108.x
11. Petrovich Z, Kuisk H, Langholz B, Astrahan M, Luxton G, Chak L, et al. Treatment Results and Patterns of Failure in 646 Patients With Carcinoma of the Eyelids, Pinna, and Nose. Am J Surg (1987) 154(4):447–50. doi: 10.1016/0002-9610(89)90022-6
12. Schulte K-W, Lippold A, Auras C, Bramkamp G, Breitkopf C, Elsmann HJ, et al. Soft X-Ray Therapy for Cutaneous Basal Cell and Squamous Cell Carcinomas. J Am Acad Dermatol (2005) 53(6):993–1001. doi: 10.1016/j.jaad.2005.07.045
13. van Hezewijk M, Creutzberg CL, Putter H, Chin A, Schneider I, Hoogeveen M, et al. Efficacy of a Hypofractionated Schedule in Electron Beam Radiotherapy for Epithelial Skin Cancer: Analysis of 434 Cases. Radiother Oncol J Eur Soc Ther Radiol Oncol (2010) 95(2):245–9. doi: 10.1016/j.radonc.2010.02.024
14. Duinkerken CW, Lohuis PJFM, Heemsbergen WD, Heemsbergen WD, Zupan-Kajcovski B, Navran A, et al. Orthovoltage for Basal Cell Carcinoma of the Head and Neck: Excellent Local Control and Low Toxicity Profile. Laryngoscope (2016) 126(8):1796–802. doi: 10.1002/lary.25865
15. Terra JB, Gaster MB, Halmos GB, Roodenburg JL, van der Vegt B, Romeijn RT, et al. Local Control of 151 Head and Neck Cutaneous Squamous Cell Carcinoma After Radiotherapy: A Retrospective Study on Efficacy and Prognostic Factors. Clin Otolaryngol Off J ENT-UK; Off J Netherlands Soc Oto-Rhino-Laryngology Cerv-fac Surg (2017) 42(4):851–5. doi: 10.1111/coa.12707
16. Jones D. Icru Report 50—Prescribing, Recording and Reporting Photon Beam Therapy. Med Phys (1994) 21(6):833–4. doi: 10.1118/1.597396
17. van der Schaaf A, Langendijk JA, Fiorino C, Rancati T. Embracing Phenomenological Approaches to Normal Tissue Complication Probability Modeling: A Question of Method. Int J Radiat Oncol (2015) 91(3):468–71. doi: 10.1016/j.ijrobp.2014.10.017
18. Trott KR, Maciejewski B, Preuss-Bayer G, Skolyszewski J. Dose-Response Curve and Split-Dose Recovery in Human Skin Cancer. Radiother Oncol J Eur Soc Ther Radiol Oncol (1984) 2(2):123–9. doi: 10.1016/s0167-8140(84)80048-1
19. Dale RG. The Application of the Linear-Quadratic Dose-Effect Equation to Fractionated and Protracted Radiotherapy. Br J Radiol (1985) 58(690):515–28. doi: 10.1259/0007-1285-58-690-515
20. Thames HD, Bentzen SM, Turesson I, Overgaard M, Van den Bogaert W. Time-Dose Factors in Radiotherapy: A Review of the Human Data. Radiother Oncol (1990) 19(3):219–35. doi: 10.1016/0167-8140(90)90149-Q
21. King CR, Freeman D, Kaplan I, Fuller D, Bolzicco G, Collins S, et al. Stereotactic Body Radiotherapy for Localized Prostate Cancer: Pooled Analysis From a Multi-Institutional Consortium of Prospective Phase II Trials. Radiother Oncol (2013) 109(2):217–21. doi: 10.1016/j.radonc.2013.08.030
22. Santiago A, Barczyk S, Jelen U, Engenhart-Cabillic R, Wittig A. Challenges in Radiobiological Modeling: can We Decide Between LQ and LQ-L Models Based on Reviewed Clinical NSCLC Treatment Outcome Data? Radiat Oncol (2016) 11:67. doi: 10.1186/s13014-016-0643-5
Keywords: tumor control probability (TCP), basal cell/carcinoma, squamous cell carcinama, biological effective dose (BED), dose response modeling
Citation: Prior P, Awan MJ, Wilson JF and Li XA (2021) Tumor Control Probability Modeling for Radiation Therapy of Keratinocyte Carcinoma. Front. Oncol. 11:621641. doi: 10.3389/fonc.2021.621641
Received: 26 October 2020; Accepted: 23 March 2021;
Published: 17 May 2021.
Edited by:
John Austin Vargo, University of Pittsburgh Medical Center, United StatesReviewed by:
Panayiotis Mavroidis, University of North Carolina at Chapel Hill, United StatesDavid McDermott, West Virginia University, United States
Copyright © 2021 Prior, Awan, Wilson and Li. This is an open-access article distributed under the terms of the Creative Commons Attribution License (CC BY). The use, distribution or reproduction in other forums is permitted, provided the original author(s) and the copyright owner(s) are credited and that the original publication in this journal is cited, in accordance with accepted academic practice. No use, distribution or reproduction is permitted which does not comply with these terms.
*Correspondence: Phillip Prior, pprior@mcw.edu