- 1Department of Infectious Diseases and Medical Immunology, Beijing Youan Hospital, Capital Medical University, Beijing, China
- 2Beijing Key Laboratory for HIV/AIDS Research, Beijing, China
- 3Department of Hepatology, The Sixth People's Hospital of Qingdao, Qingdao, China
- 4Center of Transfusion-Transmitted Infectious Diseases, Institute of Blood Transfusion, Chinese Academy of Medical Sciences (CAMS), Chengdu, China
Hepatocellular carcinoma (HCC) is one of the most common cancers worldwide and has high mortality. Biomarkers related to HCC, such as alpha-fetoprotein, and imaging technology, such as ultrasound and computed tomography, have been used to screen and monitor HCC, but HCC is still difficult to diagnose effectively in the early stage due to the low sensitivity of the above mentioned traditional methods. There is an urgent need for noninvasive biomarkers to facilitate the screening and early diagnosis of HCC. With the advancement of next-generation sequencing, genetic biomarkers are becoming the core of cancer diagnosis. Genetic biomarkers such as peripheral blood circulating tumor DNA, microRNAs, long noncoding RNAs, circular RNAs, and exosomes have become the focus of early HCC diagnostics. HCC genetic biomarkers have been implemented in clinical practice. In this review, we describe the available literature on peripheral blood genetic biomarkers in the diagnosis of early HCC.
Introduction
Liver cancer is the fifth most common cancer worldwide and has become the third leading cause of cancer-related deaths, based on reports from the World Health Organization. Hepatocellular carcinoma (HCC) accounts for ~90% of primary liver cancer, and chronic hepatitis B virus (HBV) and hepatitis C virus (HCV) infection is the main cause of HCC, accounting for 80% of HCC cases worldwide (1–3). Approximately 15% of HCC cases are attributed to nonalcoholic fatty liver disease, alcoholic cirrhosis, and other causes (4, 5). The annual incidence of HCC in patients with liver cirrhosis (LC) by any cause is 2–4% (5, 6). Early diagnosis of HCC is difficult because most patients with cirrhosis are asymptomatic. Therefore, over the past few decades, deaths related to HCC have increased significantly. Most HCC patients are found to be in an advanced stage and face 1-year survival rates of 15–39% with limited treatment options (7, 8). The overall 5-year survival rate for HCC patients is 10–20% (9). However, early diagnosis can significantly improve HCC prognosis, and emerging evidence has shown that surgical resection and liver transplantation in patients with early detection may be the best chance for the treatment of HCC (10). The 5-year survival rate of patients with early HCC treated by hepatectomy or liver transplantation increased to 60–70% (11–14). Moreover, a meta-analysis of 47 studies involving 15,158 patients showed that overall survival improved by diagnosing HCC at a very early stage, meaning that patients were eligible for potentially curative therapies such as surgical resection and liver transplantation (15). Clinical practice guidelines for HCC recommend the use of imaging tests such as computed tomography (CT), magnetic resonance imaging (MRI), ultrasound (US), or a combination with serum alpha-fetoprotein (AFP) assay for the diagnosis of HCC at an early stage (16). However, imaging has limitations in diagnostic accuracy and sensitivity, while common serum markers show poor diagnostic performance (5). Histological biopsy is the gold standard for the diagnosis of HCC, and the risks of biopsy and the difficulty to take a biopsy sample at the early tumor stage have limited its wide applicability. Therefore, it is urgent to find new biomarkers with high sensitivity and specificity that are useful in the early diagnosis of HCC. With the advent of high-throughput sequencing technology, genetic biomarkers such as cell-free DNA (cfDNA), epigenetic changes, and circulating RNA [microRNAs (miRNAs), long noncoding RNAs (lncRNA), and circular RNAs (circRNA)] from peripheral blood have become the focus of the early diagnosis of HCC. HCC genetic biomarkers have been widely developed and integrated in clinical practice, as shown in Figure 1. In this review, we aimed to describe current studies on the detection of miRNAs, lncRNAs, circRNAs, exosomes, and circulating tumor DNA (ctDNA) in peripheral blood as candidate genetic markers for the early diagnosis of HCC. Eventually, early diagnosis will provide more treatment options and improve the survival rate of HCC patients.
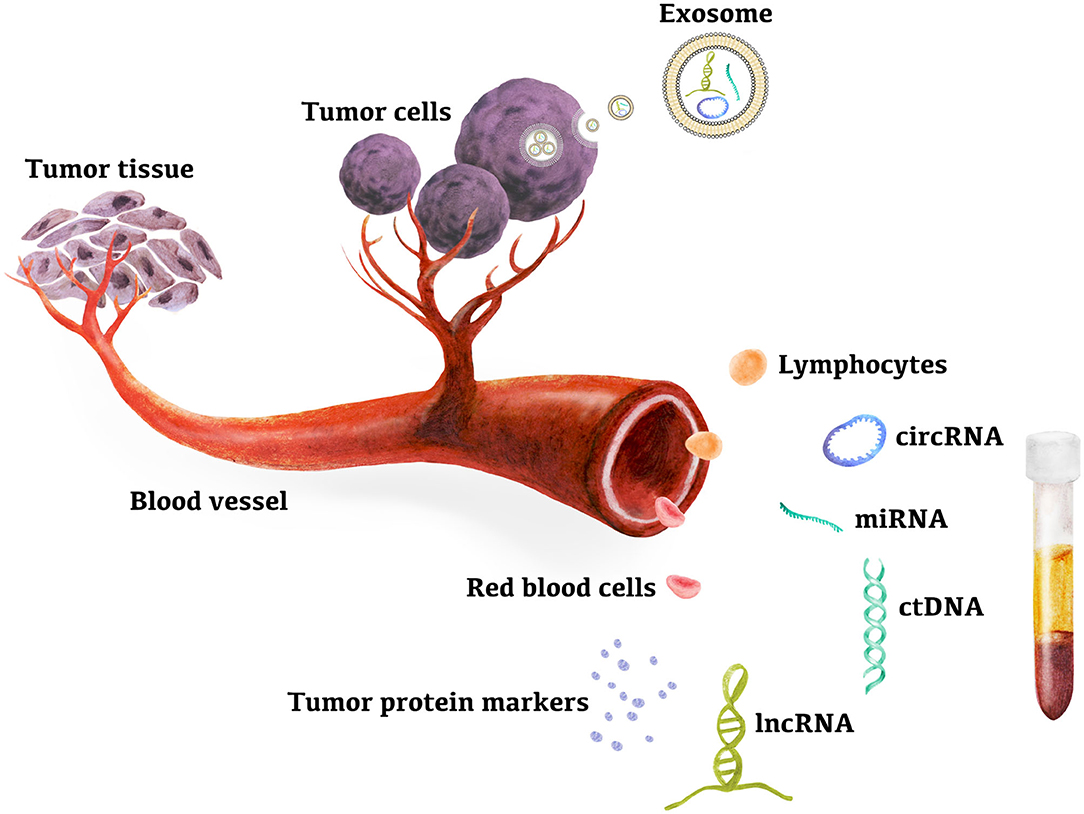
Figure 1. Peripheral blood genetic biomarkers for HCC early diagnosis. Evidences suggested that ctDNAs in plasma and miRNAs, lncRNAs, circRNAs in exsome, plasma, and PBMC are new candidates for high sensitive biomarkers for early diagnosis of hepatocellular carcinoma. miRNA, microRNA; lncRNA, long noncoding RNA; circRNA, circular RNA; ctDNA, circulating tumor DNA.
MicroRNAs
MiRNA is a short single-stranded noncoding endogenous RNA molecule with a length of ~22 nucleotides. It regulates gene expression by targeting messenger RNA (mRNA), leading to degradation or inhibition of translation (17, 18). MiRNAs play an important role in cell development, proliferation, and apoptosis, and they may regulate cell death resistance and avoid immune damage, tissue invasion/metastasis, angiogenesis, and gene mutation, contributing to tumorigenesis and cancer progression (17). MiRNAs in serum and plasma have been identified as tumor biomarker targets. There is a correlation between abnormal circulating miRNA expression levels and the clinicopathological characteristics of certain malignancies (such as breast cancer, prostate cancer, lung cancer, and HCC) (19–22). Circulating miRNAs are stable, readily available and not affected by RNase activity in the pathogenesis of HCC; thus, circulating miRNAs are the promising early-stage HCC biomarkers (22, 23). Numerous studies have been conducted on HCC biomarkers of circulating miRNAs (as shown in Table 1).
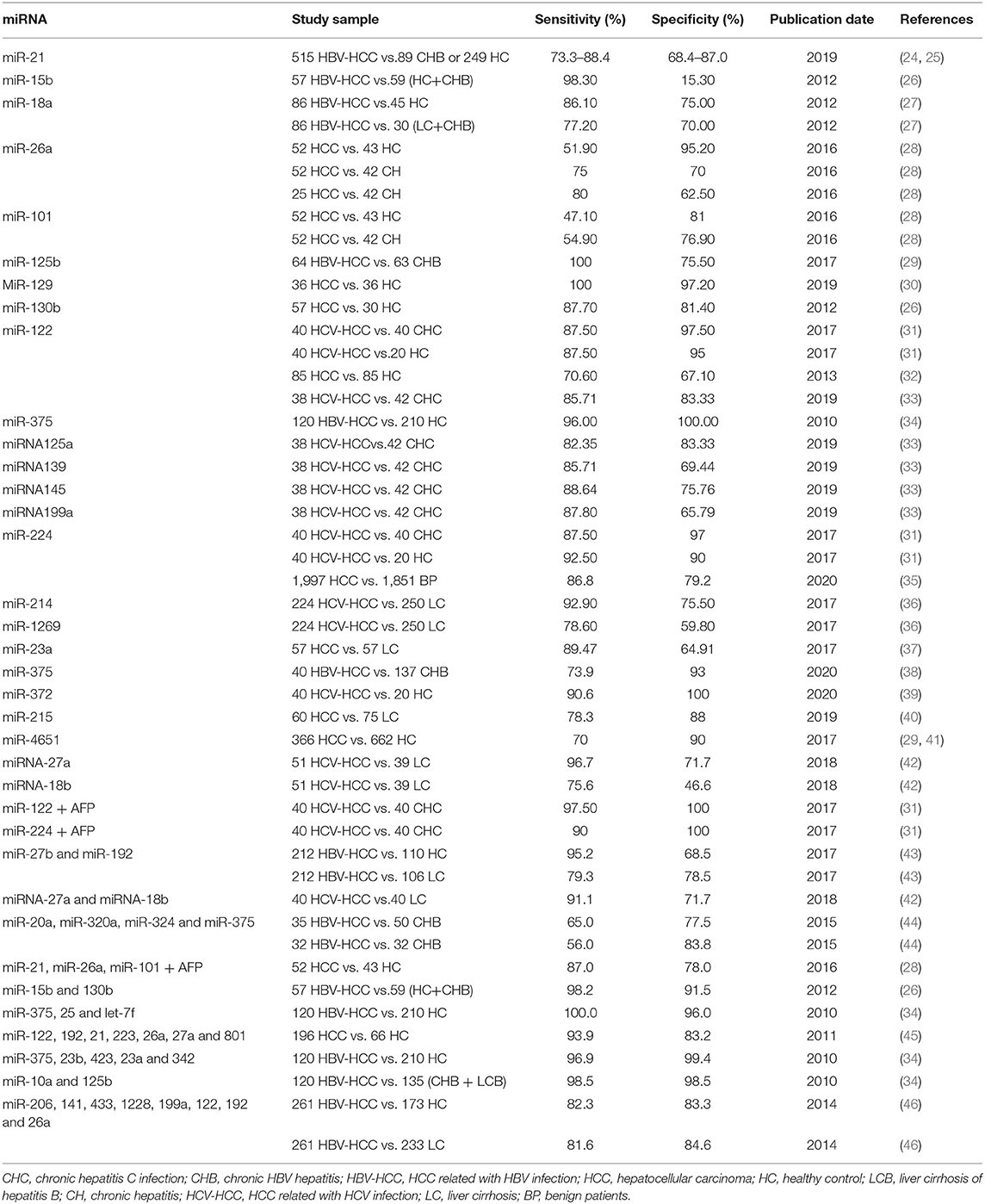
Table 1. Sensitivity and specificity of selected studies and blood biomarkers for detecting hepatocellular carcinoma.
A meta-analysis evaluated microRNA-21 as a biomarker for the early diagnosis of HCC. A total of 515 HCC patients and 338 healthy controls (HCs) or patients with chronic hepatitis (CH) were included in six published studies from different laboratories. The pooled sensitivity and specificity of miR-21 for HCC diagnosis were 85.2% (73.3–88.4%) and 79.2% (68.4–87.0%), respectively. The area under the curve-receiver operating characteristic curve (AUC-ROC) was 0.89, and it was concluded that miR-21 is a useful biomarker for the early diagnosis of HCC (24). However, further large-scale prospective studies are needed to validate the clinical application of miR-21 for the early diagnosis of HCC. Previous studies reported that the level of serum miR-21 in HCC patients was significantly higher than that in patients with chronic hepatitis (CH) and healthy controls (HCs). MiR-21 belongs to oncogenic miRNA and has oncogenic effects. MiR-21 targets tumor suppressor genes such as programmed cell death 4, phosphatase, and tensin homolog and promotes HCC cell proliferation, migration and invasion (47, 48). A study evaluated circulating miRNAs as biomarkers for the early diagnosis of HCC in Egypt and the results showed that miR-214 and miR-1269 could be used as early biomarkers for tracking the progression of LC to HCC with AUC values of 0.842 (sensitivity 92.9% and specificity 75.5%) and 0.691 (sensitivity 78.6% and specificity 59.8%), respectively. Further research found that the AUC value of the miRNA panel (miR-214, miR-125b, miR-1269, and miR-375) detected HCC in patients with liver cirrhosis (LC) increased to 0.99 with specificity 98.3% and sensitivity 98.7%. The miRNA panel represents an accurate and sensitive biomarker for the early diagnosis of HCC (36). Another study evaluated circulating miRNAs as biomarkers for the early screening of HCC in patients from China. In the exploration phase, high expression of miR-15b and miR-130b in HCC tumor tissues was identified. These miRNAs are also detectable in serum samples of HCC patients and are sharply reduced after tumor surgical resection, showing that miR-15b and miR-130b are from tumor-derived sources. In the validation phase, the sensitivity and specificity of miR-130b for detecting HCC from chronic hepatitis B (CHB) and HC were 87.7% and 81.4%, respectively. MiR-15b had the highest sensitivity (98.3%) but low specificity (15.3%) in detecting HCC. The sensitivity and specificity of combined miR-15b and miR-130b in detecting HCC improved to 98.2 and 91.5%, respectively, and the accuracy rate of combined miR-15b and miR-130b in the diagnosis of early-stage HCC was 97.8%. The results show that miR-15b and miR-130b may be used as biomarkers for early HCC detection (26). Tan et al. proposed and validated the serum miRNA panel (8 differentially expressed miRNAs: miR-206, miR-141, miR-433, miR-1228, miR-199a, miR-122, miR-192, and miR-26a) as biomarkers to detect HCC in training and validation cohorts. The sensitivity of the miRNA panel in detecting HCC in patients with LC was higher than that of AFP (cut-off value 20 ng/mL) (81.6 vs. 57.3%), while the specificity of the miRNA panel was similar to that of AFP (84.6 vs. 79.5%). The diagnostic performance of the miRNA panel in HCC was better than that of AFP (46). Other studies have shown that the AUC value of the miRNA panel (seven differentially expressed miRNAs: miR-29a, miR-29c, miR-133a, miR-143, miR-145, miR-192, and miR-505) is higher than AFP to distinguish patients with HCC from controls. The miRNA panel had a higher AUC than AFP in identifying small HCC (0.833 vs. 0.727) and early-stage HCC (0.824 vs. 0.754) and can also detect AFP-negative HCC with an AUC value of 0.825 (49). Previous studies reported that the levels of serum miR-192, miRNA-125b and miR-23a in HCC patients could serve as biomarkers for predicting microvascular invasion and survival time (48, 50–52). Recently, Amr et al. assessed the diagnostic potential of miR-122 and miR-224 as biomarkers for early HCC. The sensitivity of miR-122 and miR-224 distinguishing early HCC from CH is 87.5%, and the specificity of miR-122 and miR-224 is 97.5 and 97%, respectively. The diagnostic accuracy of miR-122 and miR-224 in distinguishing early HCC from CH is 0.98 and 0.93, respectively (31). Other studies from different labs have also evaluated the sensitivity and specificity of miR-122 to distinguish early HCC from the control group (32, 33). These studies indicate that the sensitivity to distinguish HCC from HC and CHC is 70.6 and 85.71%, and the specificity is 67.1 and 83.33%, respectively. Therefore, miR-122 and miR-224 can be used as markers for early diagnosis of HCC. It is worth noting that miR-26a can distinguish early-stage HCC from CH, with an AUC value of 0.753 (80% sensitivity and 62.5% specificity) (28). These results show that miRNAs may be a suitable target for the development of the next generation of biomarkers for early diagnostic HCC, and miRNA panels or combined miRNAs and proteins such as AFP may provide a high diagnostic accuracy of HCC as compared with single miRNA. However, these studies had some limitations, since the enrolled patients mainly originated from Asia, Europe and America, and fewer African populations were enrolled. Therefore, the expression of miRNAs in HCC patients was not explored in different ethnicities. At present, there is no consensus on which miRNAs or combinations of miRNAs are more suitable for early HCC diagnosis. The profiles of miRNA expression in different etiologies of HCC (HBV-HCC, HCV-HCC, and nonalcoholic fatty liver disease-associated HCC) are also different, and further research is needed. The upregulation of some miRNAs was detected in patients with chronic liver inflammation. Thus, these miRNAs must be selected for combination with other markers for HCC diagnosis.
The miRNA-based targeted therapy of HCC may be a potential strategy for anticancer immunotherapies. Recently, miRNAs such as miR-16, miR-21, miR-155, and miR-34 have been introduced as therapeutic targets in clinical trials for cancer treatment, including multiple solid tumors and hematological malignances, and have improved the overall survival rate of cancer patients (53, 54). A miRNA-based strategy may be suitable for the development of the next generation of HCC treatments.
Long Noncoding RNAs
MiRNA is not the only nucleic acid used as a biomarker for HCC, but the long noncoding RNA (lncRNA) is also a current research hotspot as a potential biomarker (55). The lncRNAs are a subgroup of noncoding RNA transcripts over 200 nucleotides in length and play a key role in the onset and development of cancers. They have been recognized as emerging stars in tumor research due to their important functions in cancer biology (56–58). Growing evidence has confirmed that many lncRNAs are frequently dysregulated in human cancers and participate in various biological processes, such as cell proliferation, apoptosis, differentiation, angiogenesis, invasion, and metastasis (59–65). The expression and dysregulation of many lncRNAs exhibit cell type specificity and cancer type specificity (66–68). Recently, evidence has shown that abnormal lncRNA expression is related to the initiation and progression of HCC (67, 69–71). Therefore, lncRNAs may be ideal HCC biomarkers and have potential use in the diagnosis of early HCC. LncRNAs were evaluated as candidate biomarkers for HCC diagnosis, as summarized in Table 2.
Li et al. (91) identified several candidate lncRNAs that are abnormally expressed in HCC tumor tissues on the basis of the published literature. Serum samples from HCC cohorts and HCs were then used to evaluate the utility of candidate lncRNAs as HCC biomarkers. The results of this study identified two lncRNAs (HULC and Linc00152) as potential HCC biomarkers. Both HULC and Linc00152 were upregulated in the plasma of patients with HCC, and the expression of HULC and Linc00152 in plasma was positively correlated with the expression in tumor tissue. The AUCs of HULC and Linc00152 to distinguish HCC from HC were 0.78 and 0.85, respectively. The AUC of the combination of HULC and Linc00152 to distinguish HCC from the healthy control group was 0.87, and the addition of AFP improved the AUC to 0.89. Thus, circulating HULC and Linc00152 were shown to be potential noninvasive biomarkers for predicting the diagnosis of early HCC. A study also reported that the diagnostic performance of circulating HULC and Linc00152 was superior to these lncRNAs from HCC tissue (92). Furthermore, as an oncogenic lncRNA, HULC promotes the growth of HCC cells by downregulating the expression of the tumor suppressor gene p18, and enhances epithelial-mesenchymal transition (EMT) to promote the hepatocarcinogenesis and metastasis of HCC by sponging miR-200a-3p and upregulating the expression of Zinc finger E-box-binding homeobox 1. HULC can also promote HCC progression by activating acyl-CoA synthetase long chain family member 1 and inhibiting miR-372 and miR-9 expression (93–95). The level of circulating HULC was significantly associated with tumor size, recurrence and Edmondson grades (96). LINC00152 regulated HCC development by sponging miR-215 and miR-193a/b-3p (97, 98). The level of circulating Linc00152 was significantly associated with tumor size and differentiation grade (96). Thus, HULC and Linc00152 can be used as indicators of HCC prognosis. A recently study reported that HULC triggers autophagy by stabilizing silent information regulator 1 protein and attenuated the chemosensitivity of HCC cells. Thus, HULC-targeted therapy enhances the chemosensitivity of HCC cells, and HULC can be used as a target to develop a sensitizing strategy for HCC chemotherapy (99). Different laboratories assessed serum lncRNA–urothelial carcinoma associated-1 (lncRNA-UCA1) expression as a novel biomarker for the diagnosis of HCC (75, 82), and the expression of lncRNA-UCA1 was significantly upregulated in HCC tissues compared to adjacent nontumor tissues and upregulated in the plasma of patients with HCC as compared with controls. There was a strong positive association between the expression of lncRNA-UCA1 in plasma and in HCC tumor tissue. Study reported that the sensitivity of lncRNA-UCA1 to distinguish HCC from HCs was between 91.4 and 92.7%, and the specificity was between 82.1 and 88.6%. In these two studies, the diagnostic performance of lncRNA-UCA1 was better than that of AFP; and the sensitivity and specificity of AFP combined with lncRNA-UCA1 in detecting HCC were 100 and 74.2%, respectively. The sensitivity of AFP combined with lncRNA-UCA1 was increased, and the specificity was decreased. In clinical practice, sensitivity may be more important than specificity in the monitoring of patients with HCC, as delayed diagnosis of HCC may lead to early progression to an advanced stage. The aberrant expression of lncRNA-UCA1 promotes HCC cell proliferation, EMT, and invasion and inhibits cell apoptosis by sponging miR-203 and miR-216b and facilitating the G1/S transition through cyclic-dependent kinase 2, which contributes to the occurrence and progression of HCC (95, 100). Elevated circulating lncRNA-UCA1 levels in HCC patients were related to large tumor sizes, vascular invasion, high tumor grades, recurrence, and Edmondson grades. Moreover, the 5-year overall survival of HCC patients with low circulating lncRNA-UCA1 levels was better than that of patients with high circulating lncRNA-UCA1 levels. The results showed that lncRNA-UCA1 may be a prognostic marker for HCC prognosis (82, 100). It was also reported that the sensitivity and specificity of lncRNA-UCA1 combined with c-JUN in the detection of HCC were 97.1 and 80% in the HC and CH groups, respectively. For early HCC detection, the combination of lncRNA-UCA1 and c-JUN reached 100% sensitivity and 80% specificity, and serum levels of lncRNA-UCA1 and c-JUN may be promising biomarkers in the diagnosis of early HCC (75). Although serum lncRNA-UCA1 and c-JUN were detected in patients with CH, high expression levels of lncRNA-UCA1 and C-JUN in serum samples were present in patients with HCC. The optimal cutoff values for lncRNA-UCA1 or C-JUN could discriminate HCC from CHC groups (75, 82). Studies have shown that the sensitivity and specificity of combined AX800134 and uc001ncr in the early diagnosis of HCC from healthy controls and CHB are 95.7 and 88.1%, respectively (88). The above results emphasize the practicability of early diagnosis of HCC based on lncRNAs. The levels of RP11–160H22.5, XLOC_014172, and LOC149086 were upregulated in the plasma of patients with HCC, and the AUCs of the combination of RP11–160H22.5, XLOC_014172, and LOC149086 to distinguish HCC from the healthy control group were 0.999 and 0.896 in the training set and validation set, respectively (89). Circulating RP11–160H22.5, XLOC_014172, and LOC149086 can be used as potential noninvasive biomarkers for the diagnosis of early HCC. Most of the HCC patients presented markedly decreased levels of these lncRNAs for a month after liver resection; however, some HCC patients showed an increased level after liver resection, which was highly associated with metastasis and poor outcomes. These initial studies are encouraging enough to consider lncRNAs as potential biomarkers for HCC, especially given the lack of efficient serum biomarkers for early HCCs, as lncRNAs were shown to be useful outcome predictors in HCC patients. However, most studies on the diagnosis of HCC based on lncRNAs have included subjects with only one or two etiologies. LncRNAs should also be investigated as markers in the diagnosis of HCC associated with nonalcoholic fatty liver disease (NAFLD), alcohol consumption, HBV infection, or HCV infection in large trials with different groups of patients.
Current studies suggest that many lncRNAs are differentially expressed in HCC and that the aberrant expression of lncRNAs is correlated with HCC progression and may serve as markers for the efficacy of treatment or as therapeutic targets of HCC (95, 101, 102). However, highly sensitive and reliable detection approaches, such as next-generation sequencing and digital PCR, are required for assaying clinical samples due to the low expression levels of lncRNAs in peripheral blood.
Circular RNAs
CircRNA is a new class of noncoding RNA produced by the “reverse splicing” of protein-coding mRNA or linear noncoding RNA, which is connected by upstream 3′ splicing sites and downstream 5′ splicing sites to form covalently closed continuous circRNA (67). Because of the rapid advances in the development of combined high-throughput sequencing and bioinformatics analysis, many circRNAs have recently been discovered. They are highly stable and conservative, widespread, and diverse in eukaryotic cells, and participate in a variety of physiological and pathological processes. Studies have shown that abnormal circRNA expression has been observed in a variety of tumors, such as colorectal cancer, breast cancer, and HCC. An increasing number of studies have shown that circRNAs regulate the occurrence and development of HCC by targeting different miRNA- and protein-coding genes, such as tumor cell proliferation, epithelial-mesenchymal transformation, apoptosis, autophagy, angiogenesis, and metastasis. Many circRNAs are highly expressed in the blood and are resistant to ribonuclease, with high tissue specificity and developmental stage specificity of HCC, thus circRNAs may represent the promising potential biomarkers for HCC diagnosis (103–109).
Several studies have reported abnormal expression of circ_000244, circ_104075, circ_000520, cSMARCA5, and circ_001565 in the serum/plasma of HCC and clarified the diagnostic performance of these circRNAs in HCC (110–115). Zhang et al. revealed that HCC tissues and serum circ_104075 were highly expressed as compared with the control group. The high expression of serum circ_104075 is specific for HCC, and HCC stage is positively correlated with serum circ_104075 level. The AUC value of circ_104075 that distinguishes HCC from HC is 0.973 (sensitivity: 0.960; specificity: 0.983). Circ_104075 showed better diagnostic performance compared with AFP (AUC value: 0.726), des-gamma-carboxyprothrombin (DCP) (AUC value: 0.771), Lens culinaris agglutinin-reactive AFP (AUC value: 0.766), lncRNA, and miRNA biomarkers such as DANCR (AUC: 0.851), miR-223 (AUC: 0.818), miR-21 (AUC: 0.782), and UCA1 (AUC: 0.735); and study showed that the high serum expression of circ_104075 was specific to HCC, so that the circulating circ_104075 can be used as a promising diagnostic biomarker for the early diagnosis of HCC (114). Result suggests that the level of serum circ_104075 in HCC patients has been found to decrease significantly after curative treatment. Circ_104075 promoted HCC tumorigenesis and development by sponging miR-582, which is an inhibitor of yes-associated protein (YAP), a major downstream effector that contributes to liver tumorigenesis. High serum circ_104075 levels in HCC patients were significantly related to advanced cancer stage (114, 116). Other researchers have identified novel and potential serum circRNA panel HCC biomarkers that reliably detect early HCC patients. Circ_000244, circ_000520, and circ_001565 are highly related to HCC with a high-ranking score. The expression levels of circ_000520 and circ_001565 in HCC patients were significantly lower than those in the HC and chronic hepatitis C (CHC) groups, and the level of circ_000244 was significantly higher than that of the healthy control group and CHC group. The AUC values of circ_000244, circ_000520, and circ_001565 discriminate between HCC and healthy groups were 0.974, 0.943, and 0.839, respectively, which are higher than that of AFP (AUC: 0.726). When combined, the circRNA panel showed remarkably high sensitivity (100%) and specificity (83.3%), indicating that the circRNA panel is also a potential diagnostic early HCC biomarker. A study also showed that serum circ_000520 was an independent prognostic factor of recurrence-free survival (RFS) in HCC patients who underwent operative procedures, chemotherapy, and radiotherapy (113). Recent studies reported (111) that plasma hsa_circ_0001445 levels in HCC patients were significantly lower than those in HCs, patients with LC, and patients with CHB. Patients with LC and CHB have lower plasma hsa_circ_0001445 levels than patients with HC; however, no significant differences were found in plasma hsa_circ_0001445 levels between patients with LC and patients with CHB. Therefore, plasma hsa_circ_0001445 can be used to distinguish patients with HCC from patients with HC, patients with LC or patients with CHB. In addition, the combined diagnostic value of plasma hsa_circ_0001445 and serum AFP was also analyzed. Compared with the use of serum AFP level or plasma hsa_circ_0001445 level alone, the efficacy of combined diagnosis of HCC is higher. No significant correlation was found between circ_0001445 and aminotransferase levels, such as alanine aminotransferase and aspartate aminotransferase. These results indicate that plasma hsa_circ_0001445 can be used as a new diagnostic and monitoring biomarker for HCC. Circ_0001445 can inhibit the proliferation, migration and invasion of HCC cells and induce the apoptosis of HCC cells by sponging miR-17 and miR-181b, which can affect HCC progression (108, 112, 115). Circ_0001445 was significantly related to overall survival and RFS in HCC patients who underwent liver resection. Thus, circ_0001445 may serve as a potential predictive biomarker for HCC (109, 114). In addition to serum circRNA as a diagnostic marker for HCC, the abnormal expression of circRNA in PBMCs of HCC patients was also studied (117), and research showed that the expression of circ_0000798 in PBMCs of HCC patients was significantly upregulated. The AUC value that distinguishes HCC from the HC group is 0.703, and the expression of circRNA is positively correlated with tumor size, indicating the potential role of circ_0000798 as a biomarker for early diagnosis of HCC (117). Previous studies have also shown that the diagnostic performance of plasma hsa_circ_0027089 is comparable to that of AFP which distinguishes HBV-related HCC from HBV-related cirrhosis and healthy participants, plasma hsa_circ_0027089 can serve as a biomarker for diagnosis of HBV-related HCC (118). However, there are not enough preclinical data to demonstrate that circRNAs can serve as targets or therapeutic vectors for cancer treatment. Currently, many studies focus on the diagnosis of HCC; however, circRNAs for the early diagnosis of HCC are mainly related to the use of tissue samples from HCC patients, and studies have shown that circRNAs from HCC tissue samples can be used as effective biomarkers for the early diagnosis of HCC (108, 115, 119, 120). There are few studies on using peripheral blood circRNAs as an early diagnosis tool for HCC, but an increasing number of circRNAs are found to involve in tumor pathophysiology. In future research, the expression of circRNA in noninvasive peripheral blood samples should be detected. These circRNAs show great potential in the diagnosis of HCC (108, 115, 121).
Exosomes
Exosomes are extracellular vehicles (EVs) with size in 30–200 nm diameter that are secreted by almost all types of cells. They can be found in most body fluids (like serum, plasma, lymph, and saliva, etc.) and have variable components such as encapsulate lipids and multiple types of proteins as well as nucleic acids including mRNA, miRNA, lncRNAs, and DNA detected inside exosomes, which can reflect the status of host cells (122). In the liver, exosomes are mainly released from three types of cells: hepatocytes, immune cells (such as Kupffer cells, natural killer cells, and lymphocytes) and nonparenchymal liver cells (such as hepatic stellate cells). Exosomes are involved in all aspects of intrahepatic cell interactions, and they involve many physiological and pathological processes (123). Accumulating evidence shows that mRNA, microRNA, lncRNA, protein, and circRNA transmitted by exosomes play an important role in the occurrence and progression of HCC (123–125). Tumor cells derive exosomes to communicate with neighboring or distant cells to further promote tumor growth, progression and metastasis (126). Tumor-derived exosomes can enter the circulatory system and be easily detected in the blood (127–132). Recently, an increasing number of studies have suggested that nucleic acids in exosomes are a group of molecules that can serve as HCC biomarkers (132–134). Exosomal circRNAs can distinguish colon cancer patients from HC patients (135), demonstrating their significance as potential noninvasive biomarkers for the diagnosis of tumors. Recently, studies have shown that circRNAs are abundant and stable in HCC cell exosomes (136), and in vivo and in vitro studies have shown that exosomal circRNAs, such as circPTGR1, Cdr1as, and circDB, were significantly elevated in the serum of HCC patients and promoted HCC cell proliferation and metastasis (124, 136–138). Studies have shown that the expression of exosomal circPTGR1 in the serum of HCC patients is significantly increased and positively correlated with tumor-node-metastasis (TNM) stage as compared with HCs (136). Therefore, exosomal circRNA may be a promising diagnostic biomarker for HCC, but this requires further investigations.
Several studies have shown that exosomal mRNA, microRNA, and lncRNA can be used as biomarkers for HCC screening (23, 128, 133, 139–144). Xu et al. have shown that the exosomal hnRNPH1 mRNA level in HCC patients is significantly higher than those in the LC, CHB, and HC groups, and its expression level is positively correlated with lymph node metastasis and TNM stage. Gender, age, status of HBsAg, and cirrhosis were found to be unrelated to the expression of exosomal hnRNPH1 mRNA. Exosomal hnRNPH1 mRNA showed a sensitivity and specificity of 85.2 and 76.5%, respectively, for distinguishing HCC from CHB, with an AUC of 0.865. The combination of hnRNPH1 mRNA with AFP significantly increased the AUC value. We searched the exosomal hnRNPH1 mRNA in the exoRBase database established by Li et al., and we found that the expression rank of exosomal hnRNPH1 mRNA in HCC was 0–10% (145). Exosomal hnRNPH1 mRNA may be used as an independent marker for early HCC diagnosis. The results also suggested that exosomal hnRNPH1 mRNA is a prognostic indicator for patients with HCC. Elevated levels of exosomal hnRNPH1 mRNA were related to worse overall survival in patients with HCC (139). Another study evaluated exosomal miR-21-5p and miR-92a-3p as biomarkers for the early screening of HCC in patients from Romania (146). This study included 48 patients diagnosed with HCC during screening, who underwent surgical resection or liver transplantation, 38 patients with LC, and 20 HCs. The analysis of the expression profile indicated that miR-21-5p was upregulated and miR-92a-3p was downregulated in plasma-derived exosomes from HCC subjects compared with LC subjects. AUC for HCC diagnosis based on AFP was 0.72. The combination of AFP and exosomal miR-21-5p and miR-92a-3p in a logistic regression equation for early screening of HCC, and the AUC for the combination of AFP and exosomal miR-21-5p and miR-92a-3p was 0.85, significantly better than serum AFP alone. Circulating exosomal miR-21-5p and miR-92a-3p could be served as potential biomarkers for HCC diagnosis in LC patients subjected to screening and surveillance.
The expression levels of lncRNA ENSG00000258332.1 and LINC000635 in serum exosomes of HCC patients were significantly higher than those of patients with CHB. The AUCs of exosomal lncRNA ENSG00000258332.1 and LINC00635 in discriminating HCC from CHB were 0.719 (71.6% sensitivity and 83.4% specificity) and 0.750 (with 76.2% sensitivity and 77.7% specificity), respectively. The AUC for the combination of lncRNAs (ENSG00000258332.1 and LINC00635) and AFP was 0.894 (83.6% sensitivity and 87.7% specificity), and exosomal ENSG00000258332.1 and LINC000635 were positively correlated with lymph node metastasis, TNM stage and overall survival in HCC patients. These data suggest that these lncRNAs could be potential biomarkers for early HCC diagnosis and the prognosis of HCC (141).
Studies have shown that the serum levels of exosomal miR-122 and miR-148a were significantly higher in early HCC than in LC. The AUCs of exosomal miR-122 and miR-148a in discriminating early HCC from LC were 0.816 and 0.891, respectively. The AUC for the combinations of exosomal miR-122, miR-148a, and AFP in discriminating early HCC from LC was 0.947 (87.0% sensitivity and 90.0% specificity). Exosomal miR-122 had the best diagnostic performance, with an AUC of 0.990 (100% sensitivity and 92.0% specificity), discriminating early HCC from CH. However, exosomal miR-122 and miR-148a were not different between the HCC and CH groups; therefore, the combination of these miRNAs and other markers of HCC diagnosis, such as exosomal lncRNAs, could discriminate early HCC from CH (144). A study also showed that the serum exosomal miR-122 expression level was significantly reduced in transarterial chemoembolization (TACE)-treated HCC patients, and the ratio of miR-122 expression before TACE/after TACE was related to disease specific survival (147). Recently, exosome-based HCC therapeutics have been explored in preclinical studies but not in clinical trials. For example, miR-122 was transfected into adipose tissue-derived mesenchymal stem cells (AMSCs), and the AMSCs delivered miR-122 to HCC cells by AMSC-derived exosomes based on the HCC tropism of AMSCs. Exosomal miR-122 enhanced the chemosensitivity of HCC cells to chemotherapeutic agents such as sorafenib by inducing G0/G1 arrest and apoptosis (124). Exosomal miR-125b levels predicted the recurrence of HCC patients who had undergone curative resection with an AUC of 0.739 (83.0% sensitivity and 67.9% specificity), and the exosomal miR-125b levels were significantly associated with tumor number, TNM stage and overall survival (148). Exosomal miRNA signatures or their combination with traditional biomarkers may be used as a suitable peripheral screening tool for HCC. Therefore, exosomes may be effective markers of early HCC and potential therapeutic targets of HCC, which deserve further study.
Circulating Tumor DNA
In 1948, Mandel et al. (149) first discovered cfDNA in peripheral blood but released cfDNA into the peripheral blood by normal and malignant cells. In 1977, Leon et al. (150) found for the first time that the concentration of cfDNA in the serum and plasma of cancer patients was significantly higher than that of healthy controls. Extensive studies have demonstrated that cfDNAs in cancer patients harbor tumor-specific genetic alterations. Several subsequent studies have shown that cfDNA in cancer patients possesses cancer-associated molecular characteristics and further confirmed that tumor cells can release DNA into peripheral blood (151–155). ctDNA is a DNA fragment containing tumor-specific genetic alterations released directly from living or dead tumor cells in primary or metastatic tumor tissue into the blood (156, 157). ctDNA represents a small fraction of the total cfDNA in cancer patients (158, 159). Therefore, liquid biopsy can analyze blood or other body fluid ctDNA to obtain genetic or epigenetic information, which can be used for tumor screening, diagnosis, prognosis, treatment monitoring or recurrence (159, 160). Liquid biopsy has a deeper understanding of genetic and epigenetic changes in the peripheral blood of HCC patients. Genetic or epigenetic alterations in cfDNA in association with HCC are detectable by liquid biopsy and are reliable indicators of changes occurring in tumor tissues (140, 159). The liquid biopsy detection method can continuously monitor tumor genes and early diagnosis HCC in a noninvasive and precise way. Evidence shows that circulating free DNA molecules in body fluids such as blood and saliva may be used as early biomarkers of HCC (161–165).
Studies have shown that peripheral blood cfDNA levels in HCC patients are correlated with HCC, and total blood cfDNA and ctDNA concentrations in HCC patients are significantly higher than in patients with HC (166, 167). Nonspecific increases in cfDNA and ctDNA concentrations are associated with HCC tumor size, and cfDNA and ctDNA levels may have potential application value in screening HCC (159, 166, 168–172). One study enrolled 72 patients with HCC, 37 patients with CH or LC, and 41 patients with HC. cfDNA levels in patients with HCC were significantly higher than those in patients with HC or benign patients. The cfDNA levels were positively associated with tumor size. The AUC value for distinguishing HCC from the HC group and benign patients was 0.949 and 0.874, respectively. Combined cfDNA and serum AFP revealed an elevated AUC of 0.974 with 95.1% sensitivity and 94.4% specificity in discriminating HCC from controls (166). One study analyzed the levels of cfDNA and AFP in 24 patients with HCC and 62 patients with CHB, and the results suggested that the cfDNA levels were significantly higher in HCC patients than in CHB patients. cfDNA levels were positively associated with AFP. Yan et al. established a mathematical model including serum AFP level, cfDNA level, and age, collectively referred to as the HCC index. The HCC index diagnoses HCC from patients with CHB with an AUC of 0.98, a sensitivity of 87%, and a specificity of 100%. The performance of the HCC index in the diagnosis of HCC is better than that of cfDNA alone or the AFP alone (165). The combination of liquid biopsy-based cfDNA with other proteins or genetic biomarkers is expected to be a clinical tool for the early diagnosis of HCC.
Cancer-specific genetic and epigenetic aberrations in ctDNA are detectable by liquid biopsy as biomarkers for the diagnosis and monitoring of HCC. In general, ctDNA aberrations include single nucleotide mutations (173, 174), DNA copy number variations (CNVs), and changes in methylation status (175, 176). DNA methylation is a well-studied epigenetic modification; and it is an epigenetic regulator of gene expression and regulates gene expression by affecting changes in chromatin structure, DNA stability, and DNA-protein interactions (177). Increased methylation of tumor suppressor genes is an early event in many tumors; therefore, it can usually be detected in the early stage of cancer or precancerous state (161, 178). ctDNA-bearing cancer-specific methylation patterns have been investigated as feasible biomarkers in cancers (161). A meta-analysis showed that the DNA methylation status of certain genes was related to the occurrence and development of HCC, including p15 and p16, APC, SPINT2, SFRP1, TFP12, GSTP1, and RASSF1A (179). Multiple studies have shown that the methylation status of several tumor suppressor genes in HCC plasma and tumor tissue is highly consistent (159). Therefore, different methylation status may become a tool for HCC diagnosis. Research by Wong et al. showed that 92% of HCC patients have plasma/serum methylation of p15 and p16, and the combination of these epigenetic markers may prove valuable for noninvasive HCC diagnosis and monitoring (175). Zhang et al. studied the methylation status of p16, p15, and RASSF1A in the serum of 50 patients with HCC and 50 HCs, and the results showed that the overall accuracy of predicting HCC was 89%, and the sensitivity and specificity were 84 and 94%, respectively. Changes in DNA methylation status may be a valuable biomarker for early detection and monitoring of the clinical course of HCC (180). Huang et al. conducted a comprehensive analysis of the methylation status of 4 genes, including APC, GSTP1, RASSF1A, and SFRP1. The results showed that the methylation status of 4 genes from the HC group identified an AUC of HCC of 0.933, while the AUC of a single gene was 0.800–0.881. The results showed that the AUC value for the methylation status of 4 genes in discriminating HCC from HCs was 0.933, while the AUC of a single gene was 0.800–0.881 (181). Using cfDNA samples from 1,098 HCC patients and 835 HC patients, Xu et al. identified an HCC-specific methylation marker panel (BMPR1A, PSD, ARHGAP25, KLF3, PLAC8, ATXN1, Chr 6:170, Chr 6:3, ATAD2, Chr 8:20). Since the methylation profiles of HCC tumor DNA and matched plasma ctDNA were highly correlated; they constructed a diagnostic prediction model with these methylation profiles. Their study demonstrated that this diagnostic prediction model showed better diagnostic performance in differentiating HCC from HCs (161). These studies have shown that methylation characteristics of HCC can be reliably identified in peripheral blood samples, which may be used as biomarkers for the diagnosis and prognosis of HCC.
In addition to the changes in methylation status, tumor-specific single nucleotide mutations of various genes have been found in the peripheral blood of HCC patients, including TP53, HCK and CTNNB1 (182–185). Six genes (TP53, CTNNB1, AXIN1, JAK1, EPS15, and CACNA2D4) are considered to harbor the most important mutations in HCC. Studies have shown that regardless of AFP status, the AUC of these six selected mutations genes to diagnose HCC is 0.92 (186). In another study, 34 long-term follow-up HCC patients with peripheral blood mononucleoside mutations, such as TP53, CTNNB1, PLCB1, PCLO, ROBO1, BAZ2B, and TTN, and HCC clinical processes were analyzed. Studies have shown that plasma samples have a high degree of consistency with single nucleotide mutations in corresponding tumor tissues. Dynamic changes in single nucleotide mutations were well correlated with the patient's tumor burden, ctDNA mutation profiles accurately assessed the patient's tumor burden with high consistency as compared with imaging results, and ctDNA mutation profiles could discover tumor occurrence early in advance of imaging for an average of 4.6 months and showed superior performance as compared with serum biomarkers AFP and DCP (187). Therefore, tumor-specific single nucleotide mutation profiles may be used as biomarkers for the early detection of HCC (188, 189). Studies have also shown that the TP53 p.R249S mutation is more common in HBV- and aflatoxin-associated HCC than in HCC associated with other etiologies in peripheral blood, and the detection of the TP53 p.R249S mutation shows promise but may only be highly specific for HCC in certain regions and races. In China, the TP53 p.R249S mutation detected in HCC cases was higher than that detected in Thailand and Africa (159, 190, 191). Ethnicity and the significant heterogeneity of HCC genetics in association with different etiologies (for instance, alcohol-related liver disease vs. HBV vs. HCV vs. NAFLD) have posed a major challenge to the development of a universal biomarker panel for detecting HCC. Studies have shown that the clinical significance of plasma DNA for copy number aberrations (CNAs) in high-risk populations warrants further investigation (192). For high-risk populations such as LC without any DNA for CNA in plasma, none of them developed HCC during the follow-up period. Plasma DNA for CNA might have future value in screening HCC in high-risk populations. However, CNAs are present in almost all types of cancer (193), which is also a challenge for us to find HCC-specific CNA patterns to diagnose early HCC.
Conclusions
Although the above described serum genetic markers have better relative sensitivity and specificity, there are still many limitations to be overcome for the diagnosis of early HCC. The main limitation of most studies using nucleic acid molecules as biomarkers of HCC is the limited size of the cohort, and the heterogeneity of HCC should be also considered. A larger patient cohort including patients with different liver disease conditions, such as alcohol-induced HCC, HBV/HCV-associated HCC and HCCs caused by other etiologies, is necessary to achieve a comprehensive analysis, and also the different races can be the basis for the evaluation of the clinical usefulness of the suggested gene biomarkers. Ideal biomarkers not only have sufficient sensitivity and specificity but perhaps more importantly, they must be widely used for monitoring and cost-effectiveness. The cost of separation, processing and amplification of these molecules, as well as quantitative detection and sequencing, might be so high that it would be difficult to apply on a large scale. These small numbers of cohorts can lead to promising results in research, but verification in large cohorts and the establishment of standard cut-off values for screening and diagnostic purposes are still needed. In addition, the establishment of a standardized test is essential for effective screening and diagnosis. Finally, due to the high heterogeneity of HCC, a panel containing multiple genetic and epigenetic alterations as well as protein and metabolite biomarkers could achieve the best diagnostic sensitivity and specificity to distinguish HCC from liver fibrosis or cirrhosis. CancerSEEK analysis detects eight tumor-associated protein biomarkers and mutations in 1933 distinct genomic positions with 98% sensitivity and 99% specificity for HCC detection with an estimated cost of $500 to perform a test (194).
Author Contributions
TS, LL, and BS conceived of the presented idea. TS, SW, and BS wrote the article, and BS supervised the manuscript. All authors listed have made a substantial, direct and intellectual contribution to the work, and approved it for publication. All authors read and approved the final manuscript.
Funding
This work was supported by the National Natural Science Foundation of China (NSFC, 81772165 and 81974303 to BS), the National 13th Five-Year Grand Program on Key Infectious Disease Control (2017ZX10202102-005-003 to BS, 2017ZX10202101-004-001 to TZ, 2018ZX10715-005-002-002 to CG, and 2017ZX10202101-001-004 to WW), the Beijing Municipal of Science and Technology Major Project (D161100000416003 to HW, Z161100000516148 to SW, and D161100000416005 to CG), the Sichuan Science and Technology Program (2019YFS0376 to SW), and the Beijing Key Laboratory for HIV/AIDS Research (BZ0089). The funders had no role in study design, data collection and analysis, decision to publish, or preparation of the manuscript.
Conflict of Interest
The authors declare that the research was conducted in the absence of any commercial or financial relationships that could be construed as a potential conflict of interest.
Abbreviations
HCC, Hepatocellular carcinoma; AFP, Alpha-fetoprotein; CT, Computed tomography; HBV, Hepatitis B virus; HCV, Hepatitis C virus; LC, Liver cirrhosis; MRI, Magnetic resonance imaging; US, Ultrasound; cfDNA, Cell-free DNA; ctDNA, Circulating tumor DNA; miRNA, MicroRNA; mRNA, Messenger RNA; HCs, Healthy controls; CH, Chronic hepatitis; AUC-ROC, Area under the curve-receiver operating characteristic curve; CHB, Chronic hepatitis B; lncRNA, long noncoding RNA; circRNA, Circular RNA; CNVs, copy number variations; CNAs, copy number aberrations.
References
1. Heimbach JK, Kulik LM, Finn RS, Sirlin CB, Abecassis MM, Roberts LR, et al. AASLD guidelines for the treatment of hepatocellular carcinoma. Hepatology. (2018) 67:358–80. doi: 10.1002/hep.29086
2. El-Serag HB. Epidemiology of viral hepatitis and hepatocellular carcinoma. Gastroenterology. (2012) 142:1264–73.e1261. doi: 10.1053/j.gastro.2011.12.061
3. Yang JD, Roberts LR. Hepatocellular carcinoma: a global view. Nat Rev Gastroenterol Hepatol. (2010) 7:448–58. doi: 10.1038/nrgastro.2010.100
4. EASL. 2017 Clinical Practice Guidelines on the management of hepatitis B virus infection. J Hepatol. (2017) 67:370–98. doi: 10.1016/j.jhep.2017.03.021
5. Yang JD, Hainaut P, Gores GJ, Amadou A, Plymoth A, Roberts LR. A global view of hepatocellular carcinoma: trends, risk, prevention and management. Nat Rev Gastroenterol Hepatol. (2019) 16:589–604. doi: 10.1038/s41575-019-0186-y
6. Villanueva A. Hepatocellular carcinoma. N Engl J Med. (2019) 380:1450–62. doi: 10.1056/NEJMra1713263
7. A new prognostic system for hepatocellular carcinoma: a retrospective study of 435 patients: the Cancer of the Liver Italian Program (CLIP) investigators. Hepatology. (1998) 28:751–5. doi: 10.1002/hep.510280322
8. Altekruse SF, McGlynn KA, Reichman ME. Hepatocellular carcinoma incidence, mortality, and survival trends in the United States from 1975 to (2005). J Clin Oncol. (2009) 27:1485–91. doi: 10.1200/JCO.2008.20.7753
9. Yu R, Tan Z, Xiang X, Dan Y, Deng G. Effectiveness of PIVKA-II in the detection of hepatocellular carcinoma based on real-world clinical data. BMC Cancer. (2017) 17:608. doi: 10.1186/s12885-017-3609-6
10. Llovet JM, Di Bisceglie AM, Bruix J, Kramer BS, Lencioni R, Zhu AX, et al. Design and endpoints of clinical trials in hepatocellular carcinoma. J Natl Cancer Inst. (2008) 100:698–711. doi: 10.1093/jnci/djn134
11. Kotewall CN, Cheung TT. Optimizing hepatectomy for hepatocellular carcinoma in Asia-patient selection and special considerations. Transl Gastroenterol Hepatol. (2018) 3:75. doi: 10.21037/tgh.2018.09.09
12. Kumari R, Sahu MK, Tripathy A, Uthansingh K, Behera M. Hepatocellular carcinoma treatment: hurdles, advances and prospects. Hepat Oncol. (2018) 5:HEP08. doi: 10.2217/hep-2018-0002
13. Llovet JM, Burroughs A, Bruix J. Hepatocellular carcinoma. Lancet. (2003) 362:1907–17. doi: 10.1016/S0140-6736(03)14964-1
14. Kim TH, Yoon JH, Lee JM. Emerging role of hepatobiliary magnetic resonance contrast media and contrast-enhanced ultrasound for noninvasive diagnosis of hepatocellular carcinoma: emphasis on recent updates in major guidelines. Korean J Radiol. (2019) 20:863–79. doi: 10.3348/kjr.2018.0450
15. Singal AG, Pillai A, Tiro J. Early detection, curative treatment, and survival rates for hepatocellular carcinoma surveillance in patients with cirrhosis: a meta-analysis. PLoS Med. (2014) 11:e1001624. doi: 10.1371/journal.pmed.1001624
16. Yu SJ. A concise review of updated guidelines regarding the management of hepatocellular carcinoma around the world: 2010-2016. Clin Mol Hepatol. (2016) 22:7–17. doi: 10.3350/cmh.2016.22.1.7
17. Shen S, Lin Y, Yuan X, Shen L, Chen J, Chen L, et al. Biomarker microRNAs for diagnosis, prognosis and treatment of hepatocellular carcinoma: a functional survey and comparison. Sci Rep. (2016) 6:38311. doi: 10.1038/srep38311
18. Macfarlane LA, Murphy PR. MicroRNA: biogenesis, function and role in cancer. Curr Genomics. (2010) 11:537–61. doi: 10.2174/138920210793175895
19. Bertoli G, Cava C, Castiglioni I. MicroRNAs: new biomarkers for diagnosis, prognosis, therapy prediction and therapeutic tools for breast cancer. Theranostics. (2015) 5:1122–43. doi: 10.7150/thno.11543
20. Casanova-Salas I, Rubio-Briones J, Calatrava A, Mancarella C, Masia E, Casanova J, et al. Identification of miR-187 and miR-182 as biomarkers of early diagnosis and prognosis in patients with prostate cancer treated with radical prostatectomy. J Urol. (2014) 192:252–9. doi: 10.1016/j.juro.2014.01.107
21. Li J, Yang H, Li Y, Liu Y, Chen S, Qi C, et al. microRNA-146 up-regulation predicts the prognosis of non-small cell lung cancer by miRNA in situ hybridization. Exp Mol Pathol. (2014) 96:195–9. doi: 10.1016/j.yexmp.2013.11.004
22. Xu J, An P, Winkler CA, Yu Y. Dysregulated microRNAs in hepatitis B virus-related hepatocellular carcinoma: potential as biomarkers and therapeutic targets. Front Oncol. (2020) 10:1271. doi: 10.3389/fonc.2020.01271
23. Chen X, Ba Y, Ma L, Cai X, Yin Y, Wang K, et al. Characterization of microRNAs in serum: a novel class of biomarkers for diagnosis of cancer and other diseases. Cell Res. (2008) 18:997–1006. doi: 10.1038/cr.2008.282
24. Qu J, Yang J, Chen M, Cui L, Wang T, Gao W, et al. MicroRNA-21 as a diagnostic marker for hepatocellular carcinoma: a systematic review and meta-analysis. Pak J Med Sci. (2019) 35:1466–71. doi: 10.12669/pjms.35.5.685
25. Li J, Jin B, Wang T, Li W, Wang Z, Zhang H, et al. Serum microRNA expression profiling identifies serum biomarkers for HCV-related hepatocellular carcinoma. Cancer Biomark. (2019) 26:501–12. doi: 10.3233/CBM-181970
26. Liu AM, Yao TJ, Wang W, Wong KF, Lee NP, Fan ST, et al. Circulating miR-15b and miR-130b in serum as potential markers for detecting hepatocellular carcinoma: a retrospective cohort study. BMJ Open. (2012) 2:e000825. doi: 10.1136/bmjopen-2012-000825
27. Li L, Guo Z, Wang J, Mao Y, Gao Q. Serum miR-18a: a potential marker for hepatitis B virus-related hepatocellular carcinoma screening. Dig Dis Sci. (2012) 57:2910–6. doi: 10.1007/s10620-012-2317-y
28. Zhuang C, Jiang W, Huang D, Xu L, Yang Q, Zheng L, et al. Serum miR-21, miR-26a and miR-101 as potential biomarkers of hepatocellular carcinoma. Clin Res Hepatol Gastroenterol. (2016) 40:386–96. doi: 10.1016/j.clinre.2015.11.002
29. Chen S, Chen H, Gao S, Qiu S, Zhou H, Yu M, et al. Differential expression of plasma microRNA-125b in hepatitis B virus-related liver diseases and diagnostic potential for hepatitis B virus-induced hepatocellular carcinoma. Hepatol Res. (2017) 47:312–20. doi: 10.1111/hepr.12739
30. Shaker OG, Abdelwahed MY, Ahmed NA, Hassan EA, Ahmed TI, Abousarie MA, et al. Evaluation of serum long noncoding RNA NEAT and MiR-129-5p in hepatocellular carcinoma. IUBMB Life. (2019) 71:1571–8. doi: 10.1002/iub.2096
31. Amr KS, Elmawgoud Atia HA, Elazeem Elbnhawy RA, Ezzat WM. Early diagnostic evaluation of miR-122 and miR-224 as biomarkers for hepatocellular carcinoma. Genes Dis. (2017) 4:215–21. doi: 10.1016/j.gendis.2017.10.003
32. Luo J, Chen M, Huang H, Yuan T, Zhang M, Zhang K, et al. Circulating microRNA-122a as a diagnostic marker for hepatocellular carcinoma. Onco Targets Ther. (2013) 6:577–83. doi: 10.2147/OTT.S44215
33. El-Ahwany EGE, Mourad L, Zoheiry MMK, Abu-Taleb H, Hassan M, Atta R, et al. MicroRNA-122a as a non-invasive biomarker for HCV genotype 4-related hepatocellular carcinoma in Egyptian patients. Arch Med Sci. (2019) 15:1454–61. doi: 10.5114/aoms.2019.86621
34. Li LM, Hu ZB, Zhou ZX, Chen X, Liu FY, Zhang JF, et al. Serum microRNA profiles serve as novel biomarkers for HBV infection and diagnosis of HBV-positive hepatocarcinoma. Cancer Res. (2010) 70:9798–807. doi: 10.1158/0008-5472.CAN-10-1001
35. Yang L, Wei C, Li Y, He X, He M. miR-224 is an early-stage biomarker of hepatocellular carcinoma with miR-224 and miR-125b as prognostic biomarkers. Biomark Med. (2020) 14:1485–500. doi: 10.2217/bmm-2020-0099
36. Elemeery MN, Badr AN, Mohamed MA, Ghareeb DA. Validation of a serum microRNA panel as biomarkers for early diagnosis of hepatocellular carcinoma post-hepatitis C infection in Egyptian patients. World J Gastroenterol. (2017) 23:3864–75. doi: 10.3748/wjg.v23.i21.3864
37. Mohamed AA, Ali-Eldin ZA, Elbedewy TA, El-Serafy M, Ali-Eldin FA, AbdelAziz H. MicroRNAs and clinical implications in hepatocellular carcinoma. World J Hepatol. (2017) 9:1001–7. doi: 10.4254/wjh.v9.i23.1001
38. Zhang W, Fu T, Guo Z, Zhang Y, Zhang L, Su H, et al. Serum miR-375 levels are closely related to disease progression from HBV infection to HBV-related hepatocellular carcinoma. Biomed Res Int. (2020) 2020:5819385. doi: 10.1155/2020/5819385
39. Shaker O, Mahfouz H, Salama A, Medhat E. Long Non-Coding HULC and miRNA-372 as diagnostic biomarkers in hepatocellular carcinoma. Rep Biochem Mol Biol. (2020) 9:230–40. doi: 10.29252/rbmb.9.2.230
40. El Mahdy HA, Abdelhamid IA, Amen AI, Abdelsameea E, Hassouna MM. MicroRNA-215 as a diagnostic marker in Egyptian patients with hepatocellular carcinoma. Asian Pac J Cancer Prev. (2019) 20:2723–31. doi: 10.31557/APJCP.2019.20.9.2723
41. Wu XM, Xi ZF, Liao P, Huang HD, Huang XY, Wang C, et al. Diagnostic and prognostic potential of serum microRNA-4651 for patients with hepatocellular carcinoma related to aflatoxin B1. Oncotarget. (2017) 8:81235–49. doi: 10.18632/oncotarget.16027
42. Rashad NM, El-Shal AS, Shalaby SM, Mohamed SY. Serum miRNA-27a and miRNA-18b as potential predictive biomarkers of hepatitis C virus-associated hepatocellular carcinoma. Mol Cell Biochem. (2018) 447:125–36. doi: 10.1007/s11010-018-3298-8
43. Zhu HT, Liu RB, Liang YY, Hasan AME, Wang HY, Shao Q, et al. Serum microRNA profiles as diagnostic biomarkers for HBV-positive hepatocellular carcinoma. Liver Int. (2017) 37:888–96. doi: 10.1111/liv.13356
44. Wen Y, Han J, Chen J, Dong J, Xia Y, Liu J, et al. Plasma miRNAs as early biomarkers for detecting hepatocellular carcinoma. Int J Cancer. (2015) 137:1679–90. doi: 10.1002/ijc.29544
45. Zhou J, Yu L, Gao X, Hu J, Wang J, Dai Z, et al. Plasma microRNA panel to diagnose hepatitis B virus-related hepatocellular carcinoma. J Clin Oncol. (2011) 29:4781–8. doi: 10.1200/JCO.2011.38.2697
46. Tan Y, Ge G, Pan T, Wen D, Chen L, Yu X, et al. A serum microRNA panel as potential biomarkers for hepatocellular carcinoma related with hepatitis B virus. PLoS One. (2014) 9:e107986. doi: 10.1371/journal.pone.0107986
47. Tomimaru Y, Eguchi H, Nagano H, Wada H, Kobayashi S, Marubashi S, et al. Circulating microRNA-21 as a novel biomarker for hepatocellular carcinoma. J Hepatol. (2012) 56:167–75. doi: 10.1016/j.jhep.2011.04.026
48. Balaceanu LA. Biomarkers vs imaging in the early detection of hepatocellular carcinoma and prognosis. World J Clin Cases. (2019) 7:1367–82. doi: 10.12998/wjcc.v7.i12.1367
49. Lin XJ, Chong Y, Guo ZW, Xie C, Yang XJ, Zhang Q, et al. A serum microRNA classifier for early detection of hepatocellular carcinoma: a multicentre, retrospective, longitudinal biomarker identification study with a nested case-control study. Lancet Oncol. (2015) 16:804–15. doi: 10.1016/S1470-2045(15)00048-0
50. Liu M, Wang L, Zhu H, Rong W, Wu F, Liang S, et al. A preoperative measurement of serum microRNA-125b may predict the presence of microvascular invasion in hepatocellular carcinomas patients. Transl Oncol. (2016) 9:167–72. doi: 10.1016/j.tranon.2016.03.002
51. Iorio MV, Croce CM. MicroRNA dysregulation in cancer: diagnostics, monitoring and therapeutics. A comprehensive review. EMBO Mol Med. (2012) 4:143–59. doi: 10.1002/emmm.201100209
52. Cho HJ, Kim SS, Nam JS, Kim JK, Lee JH, Kim B, et al. Low levels of circulating microRNA-26a/29a as poor prognostic markers in patients with hepatocellular carcinoma who underwent curative treatment. Clin Res Hepatol Gastroenterol. (2017) 41:181–9. doi: 10.1016/j.clinre.2016.09.011
53. Rupaimoole R, Slack FJ. MicroRNA therapeutics: towards a new era for the management of cancer and other diseases. Nat Rev Drug Discov. (2017) 16:203–22. doi: 10.1038/nrd.2016.246
54. Mollaei H, Safaralizadeh R, Rostami Z. MicroRNA replacement therapy in cancer. J Cell Physiol. (2019) 234:12369–84. doi: 10.1002/jcp.28058
55. Peng L, Yuan XQ, Zhang CY, Peng JY, Zhang YQ, Pan X, et al. The emergence of long non-coding RNAs in hepatocellular carcinoma: an update. J Cancer. (2018) 9:2549–58. doi: 10.7150/jca.24560
56. Prensner JR, Chinnaiyan AM. The emergence of lncRNAs in cancer biology. Cancer Discov. (2011) 1:391–407. doi: 10.1158/2159-8290.CD-11-0209
57. Du Z, Fei T, Verhaak RG, Su Z, Zhang Y, Brown M, et al. Integrative genomic analyses reveal clinically relevant long noncoding RNAs in human cancer. Nat Struct Mol Biol. (2013) 20:908–13. doi: 10.1038/nsmb.2591
58. Li J, Han L, Roebuck P, Diao L, Liu L, Yuan Y, et al. TANRIC: an interactive open platform to explore the function of lncRNAs in cancer. Cancer Res. (2015) 75:3728–37. doi: 10.1158/0008-5472.CAN-15-0273
59. Sun L, Luo H, Liao Q, Bu D, Zhao G, Liu C, et al. Systematic study of human long intergenic non-coding RNAs and their impact on cancer. Sci China Life Sci. (2013) 56:324–34. doi: 10.1007/s11427-013-4460-x
60. Mercer TR, Dinger ME, Mattick JS. Long non-coding RNAs: insights into functions. Nat Rev Genet. (2009) 10:155–9. doi: 10.1038/nrg2521
61. Castellanos-Rubio A, Fernandez-Jimenez N, Kratchmarov R, Luo X, Bhagat G, Green PH, et al. A long noncoding RNA associated with susceptibility to celiac disease. Science. (2016) 352:91–5. doi: 10.1126/science.aad0467
62. Wang P, Xue Y, Han Y, Lin L, Wu C, Xu S, et al. The STAT3-binding long noncoding RNA lnc-DC controls human dendritic cell differentiation. Science. (2014) 344:310–3. doi: 10.1126/science.1251456
63. Lee S, Kopp F, Chang TC, Sataluri A, Chen B, Sivakumar S, et al. Noncoding RNA NORAD regulates genomic stability by sequestering PUMILIO proteins. Cell. (2016) 164:69–80. doi: 10.1016/j.cell.2015.12.017
64. Shuai Y, Ma Z, Lu J, Feng J. LncRNA SNHG15: a new budding star in human cancers. Cell Prolif. (2019) 53:e12716. doi: 10.1111/cpr.12716
65. Shi H, Xu Y, Yi X, Fang D, Hou X. Current Research Progress on Long Noncoding RNAs Associated with Hepatocellular Carcinoma. Anal Cell Pathol (Amst). (2019) 2019:1534607. doi: 10.1155/2019/1534607
66. Yan X, Hu Z, Feng Y, Hu X, Yuan J, Zhao SD, et al. Comprehensive genomic characterization of long non-coding RNAs across human cancers. Cancer Cell. (2015) 28:529–40. doi: 10.1016/j.ccell.2015.09.006
67. Wong CM, Tsang FH, Ng IO. Non-coding RNAs in hepatocellular carcinoma: molecular functions and pathological implications. Nat Rev Gastroenterol Hepatol. (2018) 15:137–51. doi: 10.1038/nrgastro.2017.169
68. Cabili MN, Trapnell C, Goff L, Koziol M, Tazon-Vega B, Regev A, et al. Integrative annotation of human large intergenic noncoding RNAs reveals global properties and specific subclasses. Genes Dev. (2011) 25:1915–27. doi: 10.1101/gad.17446611
69. Niu ZS, Niu XJ, Wang WH. Long non-coding RNAs in hepatocellular carcinoma: Potential roles and clinical implications. World J Gastroenterol. (2017) 23:5860–74. doi: 10.3748/wjg.v23.i32.5860
70. Lin YH, Wu MH, Yeh CT, Lin KH. Long non-coding RNAs as mediators of tumor microenvironment and liver cancer cell communication. Int J Mol Sci. (2018) 19:3742. doi: 10.3390/ijms19123742
71. Bao H, Su H. Long Noncoding RNAs act as novel biomarkers for hepatocellular carcinoma: progress and prospects. Biomed Res Int. (2017) 2017:6049480. doi: 10.1155/2017/6049480
72. Ma X, Wang X, Yang C, Wang Z, Han B, Wu L, et al. DANCR acts as a diagnostic biomarker and promotes tumor growth and metastasis in hepatocellular carcinoma. Anticancer Res. (2016) 36:6389–98. doi: 10.21873/anticanres.11236
73. Konishi H, Ichikawa D, Yamamoto Y, Arita T, Shoda K, Hiramoto H, et al. Plasma level of metastasis-associated lung adenocarcinoma transcript 1 is associated with liver damage and predicts development of hepatocellular carcinoma. Cancer Sci. (2016) 107:149–54. doi: 10.1111/cas.12854
74. Ma W, Wang H, Jing W, Zhou F, Chang L, Hong Z, et al. Downregulation of long non-coding RNAs JPX and XIST is associated with the prognosis of hepatocellular carcinoma. Clin Res Hepatol Gastroenterol. (2017) 41:163–70. doi: 10.1016/j.clinre.2016.09.002
75. El-Tawdi AH, Matboli M, El-Nakeep S, Azazy AE, Abdel-Rahman O. Association of long noncoding RNA and c-JUN expression in hepatocellular carcinoma. Expert Rev Gastroenterol Hepatol. (2016) 10:869–77. doi: 10.1080/17474124.2016.1193003
76. Gao S, Xu X, Wang Y, Zhang W, Wang X. Diagnostic utility of plasma lncRNA small nucleolar RNA host gene 1 in patients with hepatocellular carcinoma. Mol Med Rep. (2018) 18:3305–13. doi: 10.3892/mmr.2018.9336
77. Wang ZF, Hu R, Pang JM, Zhang GZ, Yan W, Li ZN. Serum long noncoding RNA LRB1 as a potential biomarker for predicting the diagnosis and prognosis of human hepatocellular carcinoma. Oncol Lett. (2018) 16:1593–601. doi: 10.3892/ol.2018.8825
78. Jing W, Gao S, Zhu M, Luo P, Jing X, Chai H, et al. Potential diagnostic value of lncRNA SPRY4-IT1 in hepatocellular carcinoma. Oncol Rep. (2016) 36:1085–92. doi: 10.3892/or.2016.4859
79. Luo P, Liang C, Zhang X, Liu X, Wang Y, Wu M, et al. Identification of long non-coding RNA ZFAS1 as a novel biomarker for diagnosis of HCC. Biosci Rep. (2018) 38:1–10. doi: 10.1042/BSR20171359
80. Tang J, Zhuo H, Zhang X, Jiang R, Ji J, Deng L, et al. A novel biomarker Linc00974 interacting with KRT19 promotes proliferation and metastasis in hepatocellular carcinoma. Cell Death Dis. (2014) 5:e1549. doi: 10.1038/cddis.2014.518
81. Wang X, Zhang W, Tang J, Huang R, Li J, Xu D, et al. LINC01225 promotes occurrence and metastasis of hepatocellular carcinoma in an epidermal growth factor receptor-dependent pathway. Cell Death Dis. (2016) 7:e2130. doi: 10.1038/cddis.2016.26
82. Kamel MM, Matboli M, Sallam M, Montasser IF, Saad AS, El-Tawdi AHF. Investigation of long noncoding RNAs expression profile as potential serum biomarkers in patients with hepatocellular carcinoma. Transl Res. (2016) 168:134–45. doi: 10.1016/j.trsl.2015.10.002
83. Chao Y, Zhou D. lncRNA-D16366 Is a potential biomarker for diagnosis and prognosis of hepatocellular carcinoma. Med Sci Monit. (2019) 25:6581–6. doi: 10.12659/MSM.915100
84. Sun L, Su Y, Liu X, Xu M, Chen X, Zhu Y, et al. Serum and exosome long non coding RNAs as potential biomarkers for hepatocellular carcinoma. J Cancer. (2018) 9:2631–9. doi: 10.7150/jca.24978
85. Dai M, Chen S, Wei X, Zhu X, Lan F, Dai S, et al. Diagnosis, prognosis and bioinformatics analysis of lncRNAs in hepatocellular carcinoma. Oncotarget. (2017) 8:95799–809. doi: 10.18632/oncotarget.21329
86. Huang J, Zheng Y, Xiao X, Liu C, Lin J, Zheng S, et al. A circulating long noncoding RNA panel serves as a diagnostic marker for hepatocellular carcinoma. Dis Markers. (2020) 2020:5417598. doi: 10.1155/2020/5417598
87. Yu J, Han J, Zhang J, Li G, Liu H, Cui X, et al. The long noncoding RNAs PVT1 and uc002mbe.2 in sera provide a new supplementary method for hepatocellular carcinoma diagnosis. Medicine (Baltimore). (2016) 95:e4436. doi: 10.1097/MD.0000000000004436
88. Wang K, Guo WX, Li N, Gao CF, Shi J, Tang YF, et al. Serum LncRNAs profiles serve as novel potential biomarkers for the diagnosis of HBV-positive hepatocellular carcinoma. PLoS One. (2015) 10:e0144934. doi: 10.1371/journal.pone.0144934
89. Tang J, Jiang R, Deng L, Zhang X, Wang K, Sun B. Circulation long non-coding RNAs act as biomarkers for predicting tumorigenesis and metastasis in hepatocellular carcinoma. Oncotarget. (2015) 6:4505–15. doi: 10.18632/oncotarget.2934
90. El-Tawdi AH, Matboli M, Shehata HH, Tash F, El-Khazragy N, Azazy Ael S, et al. Evaluation of circulatory RNA-based biomarker panel in hepatocellular carcinoma. Mol Diagn Ther. (2016) 20:265–77. doi: 10.1007/s40291-016-0200-9
91. Li J, Wang X, Tang J, Jiang R, Zhang W, Ji J, et al. HULC and Linc00152 act as novel biomarkers in predicting diagnosis of hepatocellular carcinoma. Cell Physiol Biochem. (2015) 37:687–96. doi: 10.1159/000430387
92. Chen S, Zhang Y, Wu X, Zhang C, Li G. Diagnostic value of lncRNAs as biomarker in hepatocellular carcinoma: an updated meta-analysis. Can J Gastroenterol Hepatol. (2018) 2018:8410195. doi: 10.1155/2018/8410195
93. Zhang H, Liao Z, Liu F, Su C, Zhu H, Li Y, et al. Long noncoding RNA HULC promotes hepatocellular carcinoma progression. Aging (Albany NY). (2019) 11:9111–27. doi: 10.18632/aging.102378
94. Cui M, Xiao Z, Wang Y, Zheng M, Song T, Cai X, et al. Long noncoding RNA HULC modulates abnormal lipid metabolism in hepatoma cells through an miR-9-mediated RXRA signaling pathway. Cancer Res. (2015) 75:846–57. doi: 10.1158/0008-5472.CAN-14-1192
95. Qiu L, Tang Q, Li G, Chen K. Long non-coding RNAs as biomarkers and therapeutic targets: Recent insights into hepatocellular carcinoma. Life Sci. (2017) 191:273–82. doi: 10.1016/j.lfs.2017.10.007
96. Xie H, Ma H, Zhou D. Plasma HULC as a promising novel biomarker for the detection of hepatocellular carcinoma. Biomed Res Int. (2013) 2013:136106. doi: 10.1155/2013/136106
97. Ma P, Wang H, Sun J, Liu H, Zheng C, Zhou X, et al. LINC00152 promotes cell cycle progression in hepatocellular carcinoma via miR-193a/b-3p/CCND1 axis. Cell Cycle. (2018) 17:974–84. doi: 10.1080/15384101.2018.1464834
98. Wang J, Zhang Y, Lu L, Lu Y, Tang Q, Pu J. Insight into the molecular mechanism of LINC00152/miR-215/CDK13 axis in hepatocellular carcinoma progression. J Cell Biochem. (2019) 120:18816–25. doi: 10.1002/jcb.29197
99. Xiong H, Ni Z, He J, Jiang S, Li X, Gong W, et al. LncRNA HULC triggers autophagy via stabilizing Sirt1 and attenuates the chemosensitivity of HCC cells. Oncogene. (2017) 36:3528–40. doi: 10.1038/onc.2016.521
100. Lanzafame M, Bianco G, Terracciano LM, Ng CKY, Piscuoglio S. The role of long non-coding RNAs in hepatocarcinogenesis. Int J Mol Sci. (2018) 19:682. doi: 10.3390/ijms19030682
101. Bhan A, Soleimani M, Mandal SS. Long noncoding RNA and cancer: a new paradigm. Cancer Res. (2017) 77:3965–81. doi: 10.1158/0008-5472.CAN-16-2634
102. Beermann J, Piccoli MT, Viereck J, Thum T. Non-coding RNAs in development and disease: background, mechanisms, and therapeutic approaches. Physiol Rev. (2016) 96:1297–325. doi: 10.1152/physrev.00041.2015
103. Zhang J, Liu H, Hou L, Wang G, Zhang R, Huang Y, et al. Circular RNA_LARP4 inhibits cell proliferation and invasion of gastric cancer by sponging miR-424-5p and regulating LATS1 expression. Mol Cancer. (2017) 16:151. doi: 10.1186/s12943-017-0719-3
104. Du WW, Yang W, Liu E, Yang Z, Dhaliwal P, Yang BB. Foxo3 circular RNA retards cell cycle progression via forming ternary complexes with p21 and CDK2. Nucleic Acids Res. (2016) 44:2846–58. doi: 10.1093/nar/gkw027
105. Yang W, Du WW, Li X, Yee AJ, Yang BB. Foxo3 activity promoted by non-coding effects of circular RNA and Foxo3 pseudogene in the inhibition of tumor growth and angiogenesis. Oncogene. (2016) 35:3919–31. doi: 10.1038/onc.2015.460
106. Yao Z, Luo J, Hu K, Lin J, Huang H, Wang Q, et al. ZKSCAN1 gene and its related circular RNA (circZKSCAN1) both inhibit hepatocellular carcinoma cell growth, migration, and invasion but through different signaling pathways. Mol Oncol. (2017) 11:422–37. doi: 10.1002/1878-0261.12045
107. Chen G, Shi Y, Liu M, Sun J. circHIPK3 regulates cell proliferation and migration by sponging miR-124 and regulating AQP3 expression in hepatocellular carcinoma. Cell Death Dis. (2018) 9:175. doi: 10.1038/s41419-017-0204-3
108. Wang M, Yu F, Li P. Circular RNAs: characteristics, function and clinical significance in hepatocellular carcinoma. Cancers (Basel). (2018) 10:258. doi: 10.3390/cancers10080258
109. Li Z, Zhou Y, Yang G, He S, Qiu X, Zhang L, et al. Using circular RNA SMARCA5 as a potential novel biomarker for hepatocellular carcinoma. Clin Chim Acta. (2019) 492:37–44. doi: 10.1016/j.cca.2019.02.001
110. Hu J, Wang E, Liu L, Wang Q, Xia D, Bai W, et al. Sorafenib may enhance antitumour efficacy in hepatocellular carcinoma patients by modulating the proportions and functions of natural killer cells. Invest New Drugs. (2019) 38:1247–56. doi: 10.1007/s10637-019-00885-2
111. Zhang X, Zhou H, Jing W, Luo P, Qiu S, Liu X, et al. The circular RNA hsa_circ_0001445 regulates the proliferation and migration of hepatocellular carcinoma and may serve as a diagnostic biomarker. Dis Markers. (2018) 2018:3073467. doi: 10.1155/2018/3073467
112. Yu J, Xu QG, Wang ZG, Yang Y, Zhang L, Ma JZ, et al. Circular RNA cSMARCA5 inhibits growth and metastasis in hepatocellular carcinoma. J Hepatol. (2018) 68:1214–27. doi: 10.1016/j.jhep.2018.01.012
113. Matboli M, Shafei AE, Ali MA, Ashry AM, Kamal KM, Agag MA, et al. circRNAs (hsa_circ_00156, hsa_circ _000224, and hsa_circ _000520) are novel potential biomarkers in hepatocellular carcinoma. J Cell Biochem. (2018) 120. doi: 10.1002/jcb.28045
114. Zhang X, Xu Y, Qian Z, Zheng W, Wu Q, Chen Y, et al. circRNA_104075 stimulates YAP-dependent tumorigenesis through the regulation of HNF4a and may serve as a diagnostic marker in hepatocellular carcinoma. Cell Death Dis. (2018) 9:1091. doi: 10.1038/s41419-018-1132-6
115. Qiu L, Xu H, Ji M, Shang D, Lu Z, Wu Y, et al. Circular RNAs in hepatocellular carcinoma: Biomarkers, functions and mechanisms. Life Sci. (2019) 231:116660. doi: 10.1016/j.lfs.2019.116660
116. Chen R, Zhu S, Fan XG, Wang H, Lotze MT, Zeh HJ, et al. High mobility group protein B1 controls liver cancer initiation through yes-associated protein-dependent aerobic glycolysis. Hepatology. (2018) 67:1823–41. doi: 10.1002/hep.29663
117. Lei B, Zhou J, Xuan X, Tian Z, Zhang M, Gao W, et al. Circular RNA expression profiles of peripheral blood mononuclear cells in hepatocellular carcinoma patients by sequence analysis. Cancer Med. (2019) 8:1423–33. doi: 10.1002/cam4.2010
118. Zhu K, Zhan H, Peng Y, Yang L, Gao Q, Jia H, et al. Plasma hsa_circ_0027089 is a diagnostic biomarker for hepatitis B virus-related hepatocellular carcinoma. Carcinogenesis. (2020) 41:296–302. doi: 10.1093/carcin/bgz154
119. Yao R, Zou H, Liao W. Prospect of circular RNA in hepatocellular carcinoma: a novel potential biomarker and therapeutic target. Front Oncol. (2018) 8:332. doi: 10.3389/fonc.2018.00332
120. Hao Q, Han Y, Xia W, Wang Q, Qian H. Systematic review and meta-analysis of the utility of circular RNAs as biomarkers of hepatocellular carcinoma. Can J Gastroenterol Hepatol. (2019) 2019:1684039. doi: 10.1155/2019/1684039
121. Jiang YL, Shang MM, Dong SZ, Chang YC. Abnormally expressed circular RNAs as novel non-invasive biomarkers for hepatocellular carcinoma: a meta-analysis. World J Gastrointest Oncol. (2019) 11:909–24. doi: 10.4251/wjgo.v11.i10.909
122. Aheget H, Mazini L, Martin F, Belqat B, Marchal JA, Benabdellah K. Exosomes: their role in pathogenesis, diagnosis and treatment of diseases. Cancers (Basel). (2020) 13:84. doi: 10.3390/cancers13010084
123. Wu Q, Zhou L, Lv D, Zhu X, Tang H. Exosome-mediated communication in the tumor microenvironment contributes to hepatocellular carcinoma development and progression. J Hematol Oncol. (2019) 12:53. doi: 10.1186/s13045-019-0739-0
124. Wang H, Lu Z, Zhao X. Tumorigenesis, diagnosis, and therapeutic potential of exosomes in liver cancer. J Hematol Oncol. (2019) 12:133. doi: 10.1186/s13045-019-0806-6
125. Abudoureyimu M, Zhou H, Zhi Y, Wang T, Feng B, Wang R, et al. Recent progress in the emerging role of exosome in hepatocellular carcinoma. Cell Prolif. (2019) 52:e12541. doi: 10.1111/cpr.12541
126. Liu H, Li B. The functional role of exosome in hepatocellular carcinoma. J Cancer Res Clin Oncol. (2018) 144:2085–95. doi: 10.1007/s00432-018-2712-7
127. Zhu LP, He YJ, Hou JC, Chen X, Zhou SY, Yang SJ, et al. The role of circRNAs in cancers. Biosci Rep. (2017) 37:BSR20170750. doi: 10.1042/BSR20170750
128. Chen R, Xu X, Tao Y, Qian Z, Yu Y. Exosomes in hepatocellular carcinoma: a new horizon. Cell Commun Signal. (2019) 17:1. doi: 10.1186/s12964-018-0315-1
129. Vella LJ, Sharples RA, Lawson VA, Masters CL, Cappai R, Hill AF. Packaging of prions into exosomes is associated with a novel pathway of PrP processing. J Pathol. (2007) 211:582–90. doi: 10.1002/path.2145
130. Caby MP, Lankar D, Vincendeau-Scherrer C, Raposo G, Bonnerot C. Exosomal-like vesicles are present in human blood plasma. Int Immunol. (2005) 17:879–87. doi: 10.1093/intimm/dxh267
131. Zhou R, Chen KK, Zhang J, Xiao B, Huang Z, Ju C, et al. The decade of exosomal long RNA species: an emerging cancer antagonist. Mol Cancer. (2018) 17:75. doi: 10.1186/s12943-018-0823-z
132. Li LM, Liu ZX, Cheng QY. Exosome plays an important role in the development of hepatocellular carcinoma. Pathol Res Pract. (2019) 215:152468. doi: 10.1016/j.prp.2019.152468
133. Cui Y, Xu HF, Liu MY, Xu YJ, He JC, Zhou Y, et al. Mechanism of exosomal microRNA-224 in development of hepatocellular carcinoma and its diagnostic and prognostic value. World J Gastroenterol. (2019) 25:1890–8. doi: 10.3748/wjg.v25.i15.1890
134. Fang T, Lv H, Lv G, Li T, Wang C, Han Q, et al. Tumor-derived exosomal miR-1247-3p induces cancer-associated fibroblast activation to foster lung metastasis of liver cancer. Nat Commun. (2018) 9:191. doi: 10.1038/s41467-017-02583-0
135. Li Y, Zheng Q, Bao C, Li S, Guo W, Zhao J, et al. Circular RNA is enriched and stable in exosomes: a promising biomarker for cancer diagnosis. Cell Res. (2015) 25:981–4. doi: 10.1038/cr.2015.82
136. Wang G, Liu W, Zou Y, Deng Y, Luo J, Zhang Y, et al. Three isoforms of exosomal circPTGR1 promote hepatocellular carcinoma metastasis via the miR449a-MET pathway. EBioMedicine. (2019) 40:432–45. doi: 10.1016/j.ebiom.2018.12.062
137. Su Y, Lv X, Yin W, Zhou L, Hu Y, Zhou A, et al. CircRNA Cdr1as functions as a competitive endogenous RNA to promote hepatocellular carcinoma progression. Aging (Albany NY). (2019) 11:8182–203. doi: 10.18632/aging.102312
138. Zhang H, Deng T, Ge S, Liu Y, Bai M, Zhu K, et al. Exosome circRNA secreted from adipocytes promotes the growth of hepatocellular carcinoma by targeting deubiquitination-related USP7. Oncogene. (2019) 38:2844–59. doi: 10.1038/s41388-018-0619-z
139. Xu H, Dong X, Chen Y, Wang X. Serum exosomal hnRNPH1 mRNA as a novel marker for hepatocellular carcinoma. Clin Chem Lab Med. (2018) 56:479–84. doi: 10.1515/cclm-2017-0327
140. Liu XN, Cui DN, Li YF, Liu YH, Liu G, Liu L. Multiple “Omics” data-based biomarker screening for hepatocellular carcinoma diagnosis. World J Gastroenterol. (2019) 25:4199–212. doi: 10.3748/wjg.v25.i29.4199
141. Xu H, Chen Y, Dong X, Wang X. Serum exosomal long noncoding RNAs ENSG00000258332.1 and LINC00635 for the diagnosis and prognosis of hepatocellular carcinoma. Cancer Epidemiol Biomarkers Prev. (2018) 27:710–6. doi: 10.1158/1055-9965.EPI-17-0770
142. Wang F, Li L, Piontek K, Sakaguchi M, Selaru FM. Exosome miR-335 as a novel therapeutic strategy in hepatocellular carcinoma. Hepatology. (2018) 67:940–54. doi: 10.1002/hep.29586
143. Sohn W, Kim J, Kang SH, Yang SR, Cho JY, Cho HC, et al. Serum exosomal microRNAs as novel biomarkers for hepatocellular carcinoma. Exp Mol Med. (2015) 47:e184. doi: 10.1038/emm.2015.68
144. Wang Y, Zhang C, Zhang P, Guo G, Jiang T, Zhao X, et al. Serum exosomal microRNAs combined with alpha-fetoprotein as diagnostic markers of hepatocellular carcinoma. Cancer Med. (2018) 7:1670–9. doi: 10.1002/cam4.1390
145. Li S, Li Y, Chen B, Zhao J, Yu S, Tang Y, et al. exoRBase: a database of circRNA, lncRNA and mRNA in human blood exosomes. Nucleic Acids Res. (2018) 46:D106–12. doi: 10.1093/nar/gkx891
146. Sorop A, Iacob R, Iacob S, Constantinescu D, Chitoiu L, Fertig TE, et al. Plasma small extracellular vesicles derived miR-21-5p and miR-92a-3p as potential biomarkers for hepatocellular carcinoma screening. Front Genet. (2020) 11:712. doi: 10.3389/fgene.2020.00712
147. Suehiro T, Miyaaki H, Kanda Y, Shibata H, Honda T, Ozawa E, et al. Serum exosomal microRNA-122 and microRNA-21 as predictive biomarkers in transarterial chemoembolization-treated hepatocellular carcinoma patients. Oncol Lett. (2018) 16:3267–73. doi: 10.3892/ol.2018.8991
148. Liu W, Hu J, Zhou K, Chen F, Wang Z, Liao B, et al. Serum exosomal miR-125b is a novel prognostic marker for hepatocellular carcinoma. Onco Targets Ther. (2017) 10:3843–51. doi: 10.2147/OTT.S140062
149. Mandel P, Metais P. [Nuclear Acids In Human Blood Plasma]. C R Seances Soc Biol Fil. (1948) 142:241–3.
150. Leon SA, Shapiro B, Sklaroff DM, Yaros MJ. Free DNA in the serum of cancer patients and the effect of therapy. Cancer Res. (1977) 37:646–50.
151. Vasioukhin V, Anker P, Maurice P, Lyautey J, Lederrey C, Stroun M. Point mutations of the N-ras gene in the blood plasma DNA of patients with myelodysplastic syndrome or acute myelogenous leukaemia. Br J Haematol. (1994) 86:774–9. doi: 10.1111/j.1365-2141.1994.tb04828.x
152. Cachia PG, Taylor C, Thompson PW, Tennant GB, Masters G, Pettersson T, et al. Non-dysplastic myelodysplasia? Leukemia. (1994) 8:677–81.
153. Sorenson GD, Pribish DM, Valone FH, Memoli VA, Bzik DJ, Yao SL. Soluble normal and mutated DNA sequences from single-copy genes in human blood. Cancer Epidemiol Biomarkers Prev. (1994) 3:67–71.
154. Thierry AR, Mouliere F, El Messaoudi S, Mollevi C, Lopez-Crapez E, Rolet F, et al. Clinical validation of the detection of KRAS and BRAF mutations from circulating tumor DNA. Nat Med. (2014) 20:430–5. doi: 10.1038/nm.3511
155. Murtaza M, Dawson SJ, Tsui DW, Gale D, Forshew T, Piskorz AM, et al. Non-invasive analysis of acquired resistance to cancer therapy by sequencing of plasma DNA. Nature. (2013) 497:108–12. doi: 10.1038/nature12065
156. Ocker M. Biomarkers for hepatocellular carcinoma: what's new on the horizon? World J Gastroenterol. (2018) 24:3974–9. doi: 10.3748/wjg.v24.i35.3974
157. Alix-Panabieres C, Pantel K. Clinical applications of circulating tumor cells and circulating tumor DNA as liquid biopsy. Cancer Discov. (2016) 6:479–91. doi: 10.1158/2159-8290.CD-15-1483
158. Ye Q, Ling S, Zheng S, Xu X. Liquid biopsy in hepatocellular carcinoma: circulating tumor cells and circulating tumor DNA. Mol Cancer. (2019) 18:114. doi: 10.1186/s12943-019-1043-x
159. Banini BA, Sanyal AJ. The use of cell free DNA in the diagnosis of HCC. Hepatoma Res. (2019) 5:1–10. doi: 10.20517/2394-5079.2019.30
160. Crowley E, Di Nicolantonio F, Loupakis F, Bardelli A. Liquid biopsy: monitoring cancer-genetics in the blood. Nat Rev Clin Oncol. (2013) 10:472–84. doi: 10.1038/nrclinonc.2013.110
161. Xu RH, Wei W, Krawczyk M, Wang W, Luo H, Flagg K, et al. Circulating tumour DNA methylation markers for diagnosis and prognosis of hepatocellular carcinoma. Nat Mater. (2017) 16:1155–61. doi: 10.1038/nmat4997
162. Oussalah A, Rischer S, Bensenane M, Conroy G, Filhine-Tresarrieu P, Debard R, et al. Plasma mSEPT9: a novel circulating cell-free DNA-based epigenetic biomarker to diagnose hepatocellular carcinoma. EBioMedicine. (2018) 30:138–47. doi: 10.1016/j.ebiom.2018.03.029
163. Howell J, Atkinson SR, Pinato DJ, Knapp S, Ward C, Minisini R, et al. Identification of mutations in circulating cell-free tumour DNA as a biomarker in hepatocellular carcinoma. Eur J Cancer. (2019) 116:56–66. doi: 10.1016/j.ejca.2019.04.014
164. Pezzuto F, Buonaguro L, Buonaguro FM, Tornesello ML. The role of circulating free DNA and MicroRNA in non-invasive diagnosis of HBV- and HCV-related hepatocellular carcinoma. Int J Mol Sci. (2018) 19:1007. doi: 10.3390/ijms19041007
165. Yan L, Chen Y, Zhou J, Zhao H, Zhang H, Wang G. Diagnostic value of circulating cell-free DNA levels for hepatocellular carcinoma. Int J Infect Dis. (2018) 67:92–7. doi: 10.1016/j.ijid.2017.12.002
166. Huang Z, Hua D, Hu Y, Cheng Z, Zhou X, Xie Q, et al. Quantitation of plasma circulating DNA using quantitative PCR for the detection of hepatocellular carcinoma. Pathol Oncol Res. (2012) 18:271–6. doi: 10.1007/s12253-011-9438-z
167. Mezzalira S, De Mattia E, Guardascione M, Dalle Fratte C, Cecchin E, Toffoli G. Circulating-free DNA analysis in hepatocellular carcinoma: a promising strategy to improve patients' management and therapy outcomes. Int J Mol Sci. (2019) 20:5498. doi: 10.3390/ijms20215498
168. Piciocchi M, Cardin R, Vitale A, Vanin V, Giacomin A, Pozzan C, et al. Circulating free DNA in the progression of liver damage to hepatocellular carcinoma. Hepatol Int. (2013) 7:1050–7. doi: 10.1007/s12072-013-9481-9
169. An Y, Guan Y, Xu Y, Han Y, Wu C, Bao C, et al. The diagnostic and prognostic usage of circulating tumor DNA in operable hepatocellular carcinoma. Am J Transl Res. (2019) 11:6462–74.
170. Tokuhisa Y, Iizuka N, Sakaida I, Moribe T, Fujita N, Miura T, et al. Circulating cell-free DNA as a predictive marker for distant metastasis of hepatitis C virus-related hepatocellular carcinoma. Br J Cancer. (2007) 97:1399–403. doi: 10.1038/sj.bjc.6604034
171. Iizuka N, Sakaida I, Moribe T, Fujita N, Miura T, Stark M, et al. Elevated levels of circulating cell-free DNA in the blood of patients with hepatitis C virus-associated hepatocellular carcinoma. Anticancer Res. (2006) 26:4713–9. doi: 10.1016/S0166-6851(00)00331-5
172. Ng CKY, Di Costanzo GG, Tosti N, Paradiso V, Coto-Llerena M, Roscigno G, et al. Genetic profiling using plasma-derived cell-free DNA in therapy-naive hepatocellular carcinoma patients: a pilot study. Ann Oncol. (2018) 29:1286–91. doi: 10.1093/annonc/mdy083
173. Chan KC, Jiang P, Zheng YW, Liao GJ, Sun H, Wong J, et al. Cancer genome scanning in plasma: detection of tumor-associated copy number aberrations, single-nucleotide variants, and tumoral heterogeneity by massively parallel sequencing. Clin Chem. (2013) 59:211–24. doi: 10.1373/clinchem.2012.196014
174. Szymanska K, Chen JG, Cui Y, Gong YY, Turner PC, Villar S, et al. TP53 R249S mutations, exposure to aflatoxin, and occurrence of hepatocellular carcinoma in a cohort of chronic hepatitis B virus carriers from Qidong, China. Cancer Epidemiol Biomarkers Prev. (2009) 18:1638–43. doi: 10.1158/1055-9965.EPI-08-1102
175. Wong IH, Lo YM, Yeo W, Lau WY, Johnson PJ. Frequent p15 promoter methylation in tumor and peripheral blood from hepatocellular carcinoma patients. Clin Cancer Res. (2000) 6:3516–21. doi: 10.1159/000007311
176. Tang JC, Feng YL, Guo T, Xie AY, Cai XJ. Circulating tumor DNA in hepatocellular carcinoma: trends and challenges. Cell Biosci. (2016) 6:32. doi: 10.1186/s13578-016-0100-z
178. Baylin SB, Jones PA. Epigenetic determinants of cancer. Cold Spring Harb Perspect Biol. (2016) 8:1–35. doi: 10.1101/cshperspect.a019505
179. Zhang C, Huang C, Sui X, Zhong X, Yang W, Hu X, et al. Association between gene methylation and HBV infection in hepatocellular carcinoma: a meta-analysis. J Cancer. (2019) 10:6457–65. doi: 10.7150/jca.33005
180. Zhang YJ, Wu HC, Shen J, Ahsan H, Tsai WY, Yang HI, et al. Predicting hepatocellular carcinoma by detection of aberrant promoter methylation in serum DNA. Clin Cancer Res. (2007) 13:2378–84. doi: 10.1158/1078-0432.CCR-06-1900
181. Huang ZH, Hu Y, Hua D, Wu YY, Song MX, Cheng ZH. Quantitative analysis of multiple methylated genes in plasma for the diagnosis and prognosis of hepatocellular carcinoma. Exp Mol Pathol. (2011) 91:702–7. doi: 10.1016/j.yexmp.2011.08.004
182. Guichard C, Amaddeo G, Imbeaud S, Ladeiro Y, Pelletier L, Maad IB, et al. Integrated analysis of somatic mutations and focal copy-number changes identifies key genes and pathways in hepatocellular carcinoma. Nat Genet. (2012) 44:694–8. doi: 10.1038/ng.2256
183. Yu L, Liu X, Han C, Lu S, Zhu G, Su H, et al. XRCC1 rs25487 genetic variant and TP53 mutation at codon 249 predict clinical outcomes of hepatitis B virus-related hepatocellular carcinoma after hepatectomy: a cohort study for 10 years' follow up. Hepatol Res. (2016) 46:765–74. doi: 10.1111/hepr.12611
184. Cai ZX, Chen G, Zeng YY, Dong XQ, Lin MJ, Huang XH, et al. Circulating tumor DNA profiling reveals clonal evolution and real-time disease progression in advanced hepatocellular carcinoma. Int J Cancer. (2017) 141:977–85. doi: 10.1002/ijc.30798
185. Kan Z, Zheng H, Liu X, Li S, Barber TD, Gong Z, et al. Whole-genome sequencing identifies recurrent mutations in hepatocellular carcinoma. Genome Res. (2013) 23:1422–33. doi: 10.1101/gr.154492.113
186. Xiong Y, Xie CR, Zhang S, Chen J, Yin ZY. Detection of a novel panel of somatic mutations in plasma cell-free DNA and its diagnostic value in hepatocellular carcinoma. Cancer Manag Res. (2019) 11:5745–56. doi: 10.2147/CMAR.S197455
187. Cai Z, Chen G, Zeng Y, Dong X, Li Z, Huang Y, et al. Comprehensive liquid profiling of circulating tumor DNA and protein biomarkers in long-term follow-up patients with hepatocellular carcinoma. Clin Cancer Res. (2019) 25:5284–94. doi: 10.1158/1078-0432.CCR-18-3477
188. Qu C, Wang Y, Wang P, Chen K, Wang M, Zeng H, et al. Detection of early-stage hepatocellular carcinoma in asymptomatic HBsAg-seropositive individuals by liquid biopsy. Proc Natl Acad Sci U S A. (2019) 116:6308–12. doi: 10.1073/pnas.1819799116
189. Duan J, Wu Y, Liu J, Zhang J, Fu Z, Feng T, et al. Genetic biomarkers for hepatocellular carcinoma in the era of precision medicine. J Hepatocell Carcinoma. (2019) 6:151–66. doi: 10.2147/JHC.S224849
190. Kimbi GC, Kew MC, Yu MC, Arakawa K, Hodkinson J. 249ser p53 mutation in the serum of black southern African patients with hepatocellular carcinoma. J Gastroenterol Hepatol. (2005) 20:1185–90. doi: 10.1111/j.1440-1746.2005.03951.x
191. Kuang SY, Lekawanvijit S, Maneekarn N, Thongsawat S, Brodovicz K, Nelson K, et al. Hepatitis B 1762T/1764A mutations, hepatitis C infection, and codon 249 p53 mutations in hepatocellular carcinomas from Thailand. Cancer Epidemiol Biomarkers Prev. (2005) 14:380–4. doi: 10.1158/1055-9965.EPI-04-0380
192. Jiang P, Chan CW, Chan KC, Cheng SH, Wong J, Wong VW, et al. Lengthening and shortening of plasma DNA in hepatocellular carcinoma patients. Proc Natl Acad Sci U S A. (2015) 112:E1317–25. doi: 10.1073/pnas.1500076112
193. Beroukhim R, Mermel CH, Porter D, Wei G, Raychaudhuri S, Donovan J, et al. The landscape of somatic copy-number alteration across human cancers. Nature. (2010) 463:899–905. doi: 10.1038/nature08822
Keywords: hepatocellular carcinoma, biomarkers, microRNA, exosome, nucleic acid
Citation: Song T, Li L, Wu S, Liu Y, Guo C, Wang W, Dai L, Zhang T, Wu H and Su B (2021) Peripheral Blood Genetic Biomarkers for the Early Diagnosis of Hepatocellular Carcinoma. Front. Oncol. 11:583714. doi: 10.3389/fonc.2021.583714
Received: 12 October 2020; Accepted: 18 February 2021;
Published: 11 March 2021.
Edited by:
Rengyun Liu, The First Affiliated Hospital of Sun Yat-Sen University, ChinaReviewed by:
Shengli Li, Shanghai Jiao Tong University, ChinaJean-Luc Raoul, Institut de Cancérologie de l'Ouest (ICO), France
Copyright © 2021 Song, Li, Wu, Liu, Guo, Wang, Dai, Zhang, Wu and Su. This is an open-access article distributed under the terms of the Creative Commons Attribution License (CC BY). The use, distribution or reproduction in other forums is permitted, provided the original author(s) and the copyright owner(s) are credited and that the original publication in this journal is cited, in accordance with accepted academic practice. No use, distribution or reproduction is permitted which does not comply with these terms.
*Correspondence: Hao Wu, whdoc@ccmu.edu.cn; Bin Su, binsu@ccmu.edu.cn
†These authors have contributed equally to this work