- 1Neurosurgery department, The First Affiliated Hospital of Wenzhou Medical University, Wenzhou, China
- 2Department of Translational Medicine, Genetron Health (Beijing) Technology, Co. Ltd., Beijing, China
- 3Holistic Integrative Pharmacy Institutes and Comprehensive Cancer Diagnosis and Treatment Center, College of Medicine, Hangzhou Normal University, Hangzhou, China
- 4Thoracic surgery department, The First Affiliated Hospital of Wenzhou Medical University, Wenzhou, China
- 5Pathology department, The First Affiliated Hospital of Wenzhou Medical University, Wenzhou, China
- 6Genetron Health (Beijing) Technology, Co. Ltd., Beijing, China
Background: Brain metastasis mainly originates from lung cancer. Napsin A and TTF-1 factors have frequently been detected in lung adenocarcinoma cases. Brain metastasis tumors with napsin A and TTF-1 positive are easily classified as lung adenocarcinoma origin. However, some thyroid cancers also exhibit these clinical features. Besides, lung is the most common metastasis of undifferential thyroid cancer. Therefore, it requires development of novel diagnostic tools to aid in distinguishing between pulmonary and thyroid origin.
Patient Findings: We reported a case that was initially diagnosed as brain metastatic lung cancer based on immunohistochemistry results. Analysis of next-generation sequencing (NGS) data from the brain lesion revealed that the cancer may have originated from the thyroid. We detected combo mutations in TERT promoter mutation, RET fusion and TP53, which are common in undifferential thyroid cancer (UTC), but rare for lung cancer. These results, coupled with identification of PAX8, indicated that this patient had UTC. Additionally, her three sons, despite being asymptomatic, were all diagnosed with papillary thyroid carcinoma.
Summary: The patient received anlotinib treatment and showed good clinical outcomes. One month after anlotinib treatment, the pulmonary nodules were found to be controlled, and the thyroid tumor drastically reduced, and tracheal compression relieved. She continued anlotinib treatment for the following two months, but died one month later because the treatment stopped owing to financial reasons. All her sons underwent total thyroidectomy with lymph node dissection.
Conclusions: Although NGS has been reported to assist in diagnosis of the origin of some tumors, this is the first evidence of NGS for the determination of the origin of thyroid tumors. To our knowledge, this is the first time that a combination of multiple mutations has been used to help determine the origin of a tumor, compared with the previous single mutant gene. Moreover, this is the first evidence on the use of anlotinib for treatment of UTC with distant metastasis. Besides, all three sons of the patient had thyroid carcinoma in subsequent examinations, indicating high-risk for familial non-medullary thyroid cancer in UTC patients and necessity for performing thyroid ultrasound testing in other family members.
Introduction
Brain metastasis results from various types of cancer, key among them being lung cancer (1). Clinical diagnosis of lung adenocarcinoma results from positive expression of napsin A aspartic peptidase (napsin A), and transcription termination factor-1 (TTF-1) markers (2, 3). In fact, brain metastasis tumors that positively express napsin A and TTF-1 are always classified as lung adenocarcinoma origin.
Undifferential thyroid cancer (UTC) is the least common type accounting for 1-2% of thyroid cancer cases. In fact, advanced distant metastatic disease is the most challenging condition among patients (4, 5). Intrathoracic, neck lymph nodes and lungs are the most common metastatic sites of UTC (6, 7). UTC often expresses PAX8 and loses thyroid cancer-specific marker-thyroglobulin (TG), and some of UTC tumors show positive napsin A and TTF-1 expression (3, 8). Immunophenotyping of the metastases for some UTC tumors is, therefore, similar to that for primary lung adenocarcinoma. Next-generation sequencing (NGS) has been successfully applied in clinical diagnosis, enabling identification of genetic features. Oncogenetic mutations in the MAPK (BRAF, RAS), PI3K and mismatch repair pathways, RAC1, TP53, and TERT promoters, as well as CCDC6-RET fusion have often been identified in UTC. However, mutations in the TERT promoter and RET fusion have been rarely detected in lung cancer (9, 10). Despite identification of several oncogenic mutations, only one mutation-driven targeted therapy has so far been approved for BRAF-mutant UTC (10).
Anlotinib is an oral novel multi-target tyrosine kinase inhibitor that targets RET, vascular endothelial growth factor receptor (VEGFR), fibroblast growth factor receptor (FGFR), platelet-derived growth factor receptors (PGFR) and c-kit (11, 12). When compared to sunitinib, anlotinib exhibits significantly lower side effects. Consequently, anlotinib has currently become an effective compound for inhibition of multi-targeting receptor tyrosine kinases, owing to its efficacy against multiple cancers, including lung cancer (13–16), advanced medullary thyroid cancer (17), soft tissue sarcoma and metastatic renal cell carcinoma (12). However, its potential to treat UTC has not been reported.
In the current study, we studied a case that was initially considered brain metastatic lung cancer based on the immunohistochemistry (IHC) results, but found to be cancer originating from thyroid after NGS of the brain lesions. Specifically, we identified combo mutations in, TERT promoter, RET fusion and TP53, which are common for UTC but rare for lung cancer. We administered anlotinib treatment to the patient and achieved good clinical outcomes. This is a first study reporting the use of anlotinib for UTC with lung metastasis.
Case description
A 63-year-old female patient was presented to hospital, in March 2019, with repeated dizziness and visual impairment. A computerized tomography/magnetic resonance imaging (CT/MRI) revealed a mass lesion in the left occipital lobe. In addition to the brain mass, positron emission tomography (PET)-CT also indicated a hyper-metabolic mass in the left thyroid as well as some opaque mottled shadows and pulmonary nodules in the lung (Figure 1). Further ultrasound revealed multiple thyroid nodules, the largest calcified one located in the left thyroid and measuring 41*30*44 mm. The patient was subjected to brain tumor resection. IHC studies of the resected brain tumor positively identified cytokeratin 7 (CK7), napsin A and TTF-1, but TG was negative (Figures 2A–C; Details on IHC methods were showed in Supplementary Data). Fine-needle aspiration (FNA) of the thyroid lesion identified clusters of undifferentiated tumor cells. Based on these results, the pathologist hypothesized that the lesions in brain and thyroid had migrated from undifferentiated lung adenocarcinoma. However, the NGS results from FSZ-Thyroid NGS Panel V1 (Genetronhealth) revealed TERT promoter mutation, RET fusion and TP53 mutation in the brain lesion (Table 1 and Supplementary Figure 1). The details of NGS were shown in the Supplementary Data. These mutations combo are indicative of undifferential thyroid cancer (9), and rarely occur in lung cancers. Since the patient did not consent to further surgery, we did not obtain any operation samples on the thyroid or lung for further analysis. In addition, we positively detected PAX8 through IHC (Figure 2D). This factor is negative in lung adenocarcinoma, but always positive in thyroid cancer (18). Based on these results (positive PAX8, negative TG, cytopathology of thyroid FNA and the special genomic features), we concluded that the patient had undifferential thyroid cancer, with lung and brain metastasis. Due to the patient with brain metastasis and without BRAF mutations, RET-targeted BLU-667 clinical trial and BRAF inhibitor were not suitable for this patient. Therefore, indication therapy or off-label treatment were needed for this patient. After comprehensive consideration, we administered anlotinib, a novel multi-kinase inhibitor that has also been found to block RET (11). One month after treatment, the pulmonary nodules were found to be controlled, thyroid tumor drastically reduced and tracheal compression relieved (Figure 3). Unfortunately, the patient stopped anlotinib treatment, after three months, owing to financial constraints. Only two weeks after discontinuation of anlotinib, the patient went back to the hospital exhibiting breathlessness and cough. A CT scan revealed a large mass outbreak in her lungs, and she eventually died of respiratory failure.
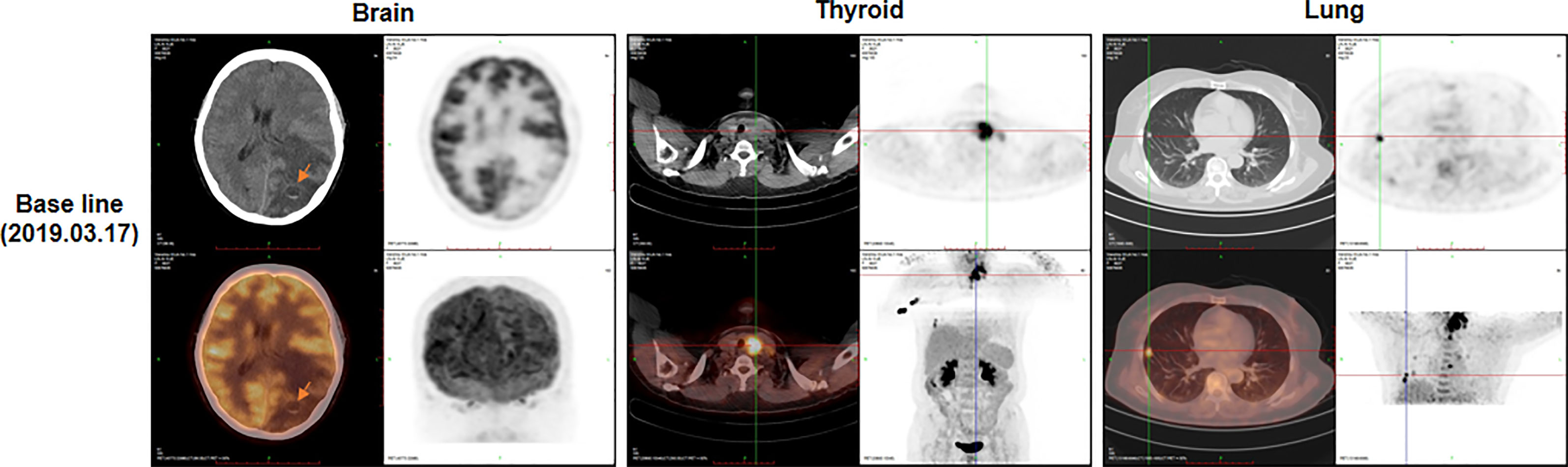
Figure 1 The positron emission tomography-computed tomography (PET-CT) at baseline (before surgery). PET-CT showed masses in brain (arrow), thyroid and lung.
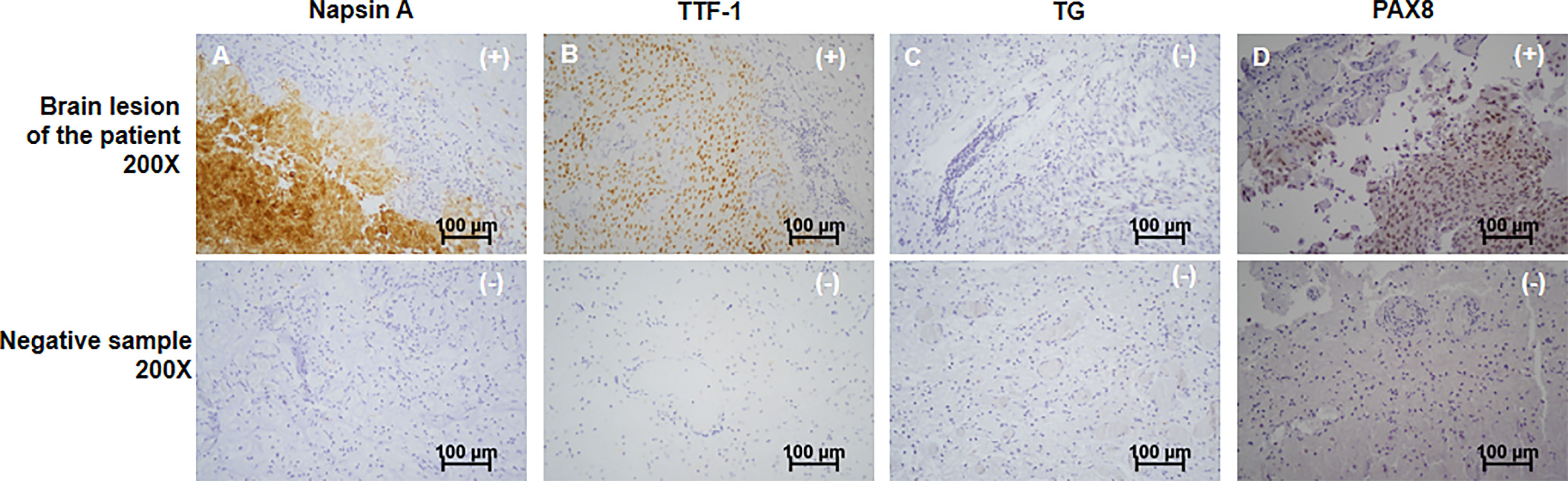
Figure 2 Immunohistochemistry of the patient’s brain cancer. From top to bottom, the immunochemistry results of the patient’s brain tumor and negative control sample were shown. The patient’s tumor showed positive of Napsin A (A), TTF-1 (B) and PAX8 (D), showing as “(+)” in top right corner of the graphs. Negative of TG (C) was identified, presenting as “(-)” in top right corner of the graphs. Corresponding negative results from negative control sample were also shown. Scale bar was in the lower right corner of each image. All images were magnified by 200 times. Scale bars (100μm) were provided.
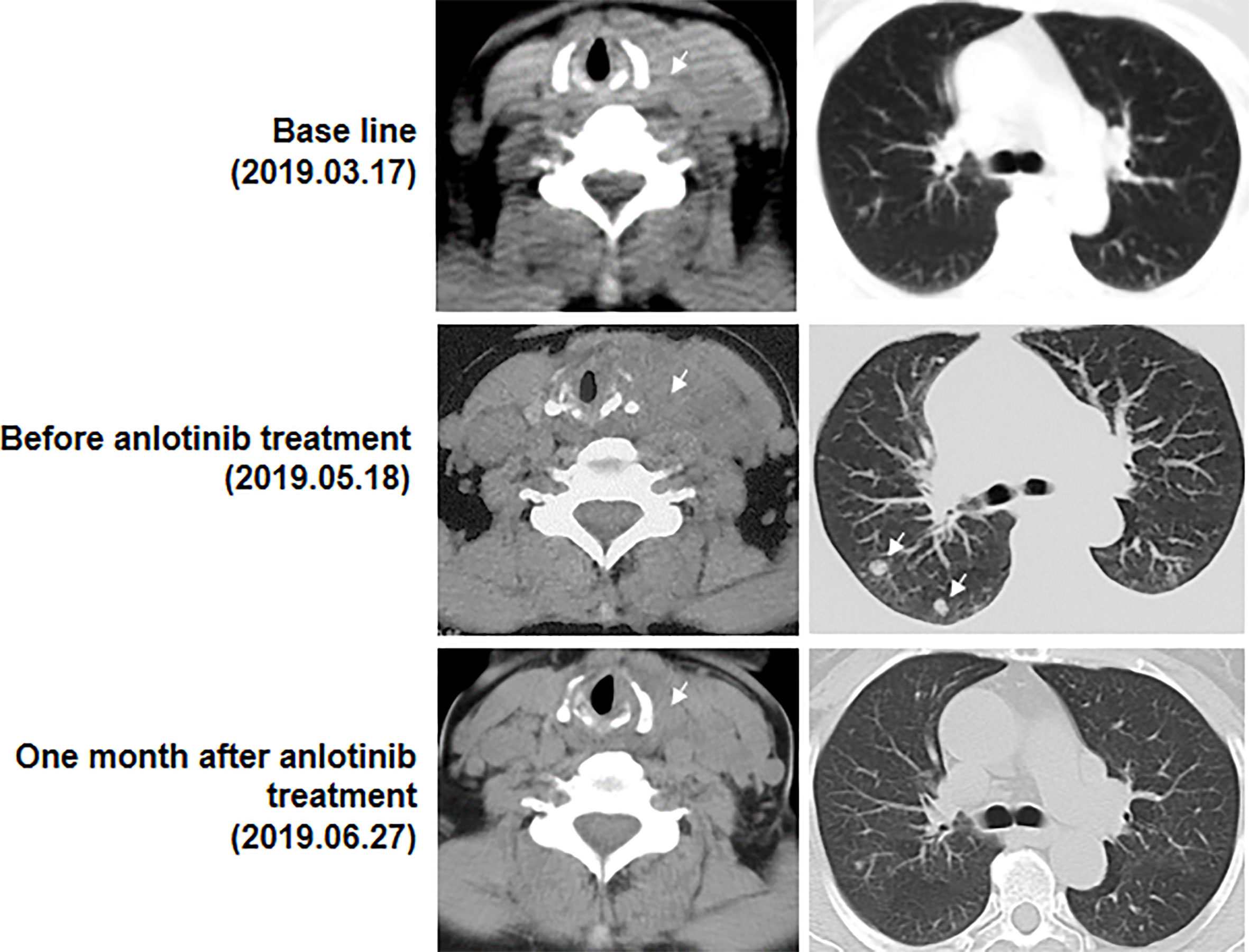
Figure 3 Computed tomography images (CT) of the thyroid and lung before and after anlotinib treatment. Arrows represent the masses. At base line, in addition to brain lesions, there were a mass in the left thyroid and some opaque mottled shadows and pulmonary nodules in the lung. Then the patient experienced brain surgery. Before anlotinib treatment, a huge mass in the left thyroid and two obvious pulmonary nodules was found. One month after anlotinib treatment, the pulmonary nodules were found to be controlled, thyroid tumor drastically reduced and tracheal compression relieved.
Given the patient’s critical condition, three sons of the proband, who were asymptomatic, were presented to the local hospital and subjected to thyroid ultrasound examinations. They were all found with thyroid lesions. Total thyroidectomy, with lymph node dissection, was performed on all of them. Results revealed a thyroid papillary carcinoma (1.2cm), in the right thyroid lobe and isthmus (without lymph node metastasis) of the 36 years old youngest son. Pathologic diagnosis in the elder son (40 years old) revealed a follicular adenoma in the left lobe as well as a thyroid papillary microcarcinoma in the right lobe and isthmus. Lymph node metastasis were found in Level VI of the neck. Unfortunately, a more extensive lymph node metastasis was further detected at Level II, III, IV and VI of the neck in the eldest son (42 years old). All tumor tissues from the three sons were positive of TTF-1, TG and PAX8, negative of Napsin A (Supplementary Figure 2). Targeted sequencing by using FSZ-Thyroid NGS Panel V1 (Genetronhealth) was performed on the tumor tissues of the three sons, the results of which showed all the tissues had BRAF V600E mutations (Table 1 and Supplementary Figure 3).
Discussion
We present a case of UTC, with lung and brain metastasis, in which genetic features including CCDC6-RET fusion, TERT promoter and TP53 mutations were identified. Based on these features, a multi-targeted reagent, anlotinib, was used to treat the patient and resulted in good clinical outcomes. This is the first study reporting the use of mutation combo and IHC for the confirmation of UTC and the use of anlotinib for the treatment of UTC. The most noteworthy point in the present case is that traditional IHC markers combined with genetic characteristic of tumor are more effective in accurately determining the source of metastatic tumor. Generally, IHC is a major diagnostic tool for determining the origin of metastatic tumors (19, 20). However, this diagnosis is not enough for some special cases. A combined detection of napsin A and TTF-1 through IHC has been considered a reliable procedure for diagnosing adenocarcinoma originating from the lungs (2, 3). However, several studies have recently shown that both of Napsin A and TTF-1 are positive in approximately 15% of UTC cases (8). TG, a specific thyroid cancer marker due to its especial expression in tissues of thyroid origin has been reported (3), but its expression is often lost following dedifferentiation of UTC (8). Therefore, a positive napsin A and TTF-1 detection, as well as negative TG, is considered a misdiagnosis for lung adenocarcinoma. Studies have reported that some genetic alterations can be used to aid in determining the source of metastatic carcinoma, with higher specificity than traditional IHC markers (16). For example, IDH has been found to be mutated in low-grade gliomas, chondrosarcomas, hematologic malignancies and bile duct cancer (21, 22). BRCA1 and BRCA2 are prone to mutations in breast and ovarian cancers, with evidence of BRCA gene mutations indicating the genetic origin of these cancers (23). In addition, TERT promoter mutations are frequently identified in melanoma, thyroid cancer, bladder cancer and glioblastoma, while they are rare (2.57%) in lung cancer. On the contrary, EGFR L858R mutation is only found in lung cancer (24). Changes in RET expression tend to frequently occur in thyroid, and invasive breast cancers, as well as pancreatic ductal adenocarcinomas, but are rare in lung cancer. Moreover, the most common RET fusions are CCDC6-RET in thyroid cancer and KIF5B-RET in lung cancer (25). A combination of mutations including CCDC6-RET fusion, TERT-promoter and TP53 often occurs in dedifferentiated thyroid cancer (9). However, there is a probability of only 0.005% of this happening in lung adenocarcinoma (24, 25). Combo mutations from NGS in this case clearly confirmed the origin of the thyroid tumors; thus, this is the first case that the determination of the tumor origin by the assist of combo mutations, compared with previous single mutant gene. Moreover, IHC analysis positively detected PAX8, which has been strongly associated with thyroid origin (8), suggesting that this factor is an additional tool for discriminating thyroid from lung cancer in the setting of a double positive of napsin A and TTF-1.
Our results showed that anlotinib is effective in treating UTC, a rare thyroid cancer with a high mortality rate. Conventional treatments, such as surgery, radiotherapy and chemotherapy, have not effectively managed. In fact, the only approved targeted therapy, dabrafenib with trametinib, only works in BRAFV600E-positive anaplastic thyroid carcinoma patients. Several novel biological agents, as well as immune checkpoint and aurora kinase inhibitors, are currently under testing for treatment of UTC (26). Case in the current study, a patient with RET fusion, who was excluded from RET-targeted BLU-667 clinical trial because of brain metastasis, and the patient without BRAF mutation, also excluded from BRAF inhibitor, finally benefited from anlotinib. Anlotinib recently showed durable antitumor activity in lung cancers (13–16), medullary thyroid carcinoma (MTC) (17) and some other cancers (12). To the best of our knowledge, this was the first time that anlotinib has been used to manage UTC. The treatment resulted in good control of primary and lung metastasis lesions. Manageable adverse events were demonstrated in anlotinib-treated lung cancer (15) and MTC (17), here also no serious side effects occurred. These clinical results indicate that anlotinib may develop a new avenue for UTC therapy.
In addition, the patient’s sons all diagnosed with thyroid carcinoma, indicating obvious characteristics of familial non-medullary thyroid cancer (FNMTC). Previous studies have implicated FNMTC as an independent risk factor for increased aggressiveness of thyroid cancers (27), which may explain the critical and serious condition of the proband. To the best of our knowledge, no reports have studied FNMTC in UTC patients. It is, therefore, imperative to perform thyroid ultrasound testing on family members of patients diagnosed with UTC.
Overall, this report describes the genetic characteristics of a UTC patient, with distant metastases, and the obvious benefits of targeted therapy using anlotinib. We illustrate that a combination of driver mutations, detected by NGS, could directly guide understanding of the origin of tumors and the corresponding targeted therapy. Taken together, our findings indicate that this treatment could be an acceptable option for the urgent management of UTC.
Data Availability Statement
The raw data supporting the conclusions of this article will be made available by the authors, without undue reservation.
Ethics Statement
Written informed consent was obtained from the patient’s relative for the publication of any potentially identifiable images or data included in this article.
Author Contributions
XL, QL, XZ, DC, LC, ZD, and JH collected this case. SZ and JL provided pathology information of this case. HZ and DW provided bioinformatics analysis of NGS data. QL and XZ wrote the manuscript. JF, YF, SW, TM, and XL modified this paper. All authors contributed to the article and approved the submitted version.
Funding
This work was supported by grants from the Wenzhou Municipal Science and Technology Bureau of China (Y2020063 and Y20180175).
Conflict of Interest
Authors XZ, JH, YF, HZ, DW, SW, and TM were employed by the company Genetron Health (Beijing) Technology, Co. Ltd., Beijing, China.
The remaining authors declare that the research was conducted in the absence of any commercial or financial relationships that could be construed as a potential conflict of interest.
Supplementary Material
The Supplementary Material for this article can be found online at: https://www.frontiersin.org/articles/10.3389/fonc.2021.569429/full#supplementary-material
Supplementary Figure 1 | The mutation IGV results of the patient. (A–C) IGV results of TERT upstream promoter mutation (c.C228T (Chr5: 1295228)), CCDC6-RET fusion (CCDC6{NM_005436.4}:r.1_303+1_RET{NM_020975.4}:r.2137_5617) and TP53 mutation (c.842A>T (Chr17: 7577096)) of the patient’s tumor sample and their corresponding IGV results of negative control sample.
Supplementary Figure 2 | Immunochemistry results of each son’s tumor tissue. From top to bottom, the immunochemistry results of the eldest son, second son and youngest son’s tumor were shown. The three sons’ tumors showed negative of Napsin A, showing as “(-)”in top right corner of the graphs. Positive of TTF-1, PAX8 and TG were identified in all of the three sons’ tumors, presenting as “(+)”in top right corner of the graphs. Negative results from negative control samples were also shown. The immunohistochemical tests of the patient and her three sons were repeated simultaneously, so the same negative controls were used. Scale bar was in the lower right corner of each image. All images were magnified by 200 times.
Supplementary Figure 3 | IGV results of BRAF mutation (c.1799T>A (Chr7: 140453136)) of the tumor samples of the patient’s three sons. Corresponding IGV results of negative control sample were also provided.
References
1. Cagney DN, Martin AM, Catalano PJ, Redig AJ, Lin NU, Lee EQ, et al. Incidence and prognosis of patients with brain metastases at diagnosis of systemic malignancy: a population-based study. Neuro Oncol (2017) 19:1511–21. doi: 10.1093/neuonc/nox077
2. Travis W, Burke E, Burke AP, Marx A, Nicholson AG. WHO classification of tumours of the lung, pleura, thymus and heart fourth edition. Geneva: IARC press (2015).
3. Wu J, Zhang Y, Ding T, Cheng R, Gong W, Guo Y, et al. Napsin A Expression in Subtypes of Thyroid Tumors: Comparison with Lung Adenocarcinomas. Endocr Pathol (2019) 31(1):39–45. doi: 10.1007/s12022-019-09600-6
4. Cabanillas ME, McFadden DG, Durante C. Thyroid cancer. Lancet (2016) 388:2783–95. doi: 10.1016/S0140-6736(16)30172-6
5. Molinaro E, Romei C, Biagini A, Sabini E, Agate L, Mazzeo S, et al. Anaplastic thyroid carcinoma: from clinicopathology to genetics and advanced therapies. Nat Rev Endocrinol (2017) 13:644–60. doi: 10.1038/nrendo.2017.76
6. Pomorski L, Bartos M. Metastasis as the first sign of thyroid cancer. Neoplasma (1999) 46:309–12.
7. Rühli FJ, Hilfiker PR. Metastasis of thyroid cancer to the heart. AJR Am J Roentgenol (2001) 177:474. doi: 10.2214/ajr.177.2.1770474
8. Chernock RD, El-Mofty SK, Becker N, Lewis JS, Napsin A. Expression in Anaplastic, Poorly Differentiated, and Micropapillary Pattern Thyroid Carcinomas. Am J Surg Pathology (2013) 37:1215–22. doi: 10.1097/PAS.0b013e318283b7b2
9. Landa I, Ibrahimpasic T, Boucai L, Sinha R, Knauf JA, Shah RH, et al. Genomic and transcriptomic hallmarks of poorly differentiated and anaplastic thyroid cancers. J Clin Invest (2016) 126:1052–66. doi: 10.1172/JCI85271
10. Pozdeyev N, Rose MM, Bowles DW, Schweppe RE. Molecular Therapeutics for Anaplastic Thyroid Cancer. Semin Cancer Biol (2020) 61:23–9. doi: 10.1016/j.semcancer.2020.01.005
11. Sun Y, Niu W, Du F, Du C, Li S, Wang J, et al. Safety, pharmacokinetics, and antitumor properties of anlotinib, an oral multi-target tyrosine kinase inhibitor, in patients with advanced refractory solid tumors. J Hematol Oncol (2016) 9:105. doi: 10.1186/s13045-016-0332-8
12. Shen G, Zheng F, Ren D, Du F, Dong Q, Wang Z, et al. Anlotinib: a novel multi-targeting tyrosine kinase inhibitor in clinical development. J Hematol Oncol (2018) 11:120. doi: 10.1186/s13045-018-0664-7
13. Cheng Y, Wang Q, Li K, Shi J, Wu L, Han B, et al. OA13.03 Anlotinib as Third-Line or Further-Line Treatment in Relapsed SCLC: A Multicentre, Randomized, Double-Blind Phase 2 Trial. J Thoracic Oncol (2018) 13:S351–2. doi: 10.1016/j.jtho.2018.08.308
14. Han B, Li K, Zhao Y, Li B, Cheng Y, Zhou J, et al. Anlotinib as a third-line therapy in patients with refractory advanced non-small-cell lung cancer: a multicentre, randomised phase II trial (ALTER0302). Br J Cancer (2018) 118:654–61. doi: 10.1038/bjc.2017.478
15. Si X, Zhang L, Wang H, Zhang X, Wang M, Han B, et al. Management of anlotinib-related adverse events in patients with advanced non-small cell lung cancer: Experiences in ALTER-0303. Thorac Cancer (2019) 10:551–6. doi: 10.1111/1759-7714.12977
16. Han B, Li K, Wang Q, Zhang L, Shi J, Wang Z, et al. Effect of Anlotinib as a Third-Line or Further Treatment on Overall Survival of Patients With Advanced Non-Small Cell Lung Cancer: The ALTER 0303 Phase 3 Randomized Clinical Trial. JAMA Oncol (2018) 4:1569–75. doi: 10.1001/jamaoncol.2018.3039
17. Sun Y, Du F, Gao M, Ji Q, Li Z, Zhang Y, et al. Anlotinib for the Treatment of Patients with Locally Advanced or Metastatic Medullary Thyroid Cancer. Thyroid (2018) 28:1455–61. doi: 10.1089/thy.2018.0022
18. Chernock RD. Immunohistochemistry of thyroid gland carcinomas: clinical utility and diagnostic pitfalls. Diagn Histopathol (2016) 22:184–90. doi: 10.1016/j.mpdhp.2016.04.008
19. Conner JR, Hornick JL. Metastatic carcinoma of unknown primary: diagnostic approach using immunohistochemistry. Adv Anat Pathol (2015) 22:149–67. doi: 10.1097/PAP.0000000000000069
20. Stelow EB, Yaziji H. Immunohistochemistry, carcinomas of unknown primary, and incidence rates. Semin Diagn Pathol (2018) 35:143–52. doi: 10.1053/j.semdp.2017.11.012
21. Mondesir J, Willekens C, Touat M, de Botton S. IDH1 and IDH2 mutations as novel therapeutic targets: current perspectives. J Blood Med (2016) 7:171–80. doi: 10.2147/JBM.S70716
22. Kamath SD, Lin X, Kalyan A. A Case of Metastatic Biliary Tract Cancer Diagnosed Through Identification of an IDH1 Mutation. Oncol (2019) 24:151–6. doi: 10.1634/theoncologist.2018-0210
23. Kuchenbaecker KB, Hopper JL, Barnes DR, Phillips K-A, Mooij TM, Roos-Blom M-J, et al. Risks of Breast, Ovarian, and Contralateral Breast Cancer for BRCA1 and BRCA2 Mutation Carriers. JAMA (2017) 317:2402. doi: 10.1001/jama.2017.7112
24. Ma X, Gong R, Wang R, Pan Y, Cai D, Pan B, et al. Recurrent TERT promoter mutations in non-small cell lung cancers. Lung Cancer (2014) 86:369–73. doi: 10.1016/j.lungcan.2014.10.009
25. Li AY, McCusker MG, Russo A, Scilla KA, Gittens A, Arensmeyer K, et al. RET fusions in solid tumors. Cancer Treat Rev (2019) 81:101911. doi: 10.1016/j.ctrv.2019.101911
26. Ranganath R, Shah MA, Shah AR. Anaplastic thyroid cancer. Curr Opin Endocrinol Diabetes Obes (2015) 22:387–91. doi: 10.1097/MED.0000000000000189
27. Tavarelli M, Russo M, Terranova R, Scollo C, Spadaro A, Sapuppo G, et al. Familial Non-Medullary Thyroid Cancer Represents an Independent Risk Factor for Increased Cancer Aggressiveness: A Retrospective Analysis of 74 Families. Front Endocrinol (Lausanne) (2015) 6:117. doi: 10.3389/fendo.2015.00117
Keywords: next-generation sequencing, undifferential thyroid cancer, TERT promoter mutation, RET fusion, anlotinib, tumor origin
Citation: Li Q, Zhang X, Feng J, Cheng D, Cai L, Dai Z, Zhao S, Li J, Huang J, Fang Y, Zhu H, Wang D, Wang S, Ma T and Lu X (2021) Case Report: Next-Generation Sequencing Reveals Tumor Origin in a Female Patient With Brain Metastases. Front. Oncol. 11:569429. doi: 10.3389/fonc.2021.569429
Received: 04 June 2020; Accepted: 18 March 2021;
Published: 12 April 2021.
Edited by:
Qinghua Xu, Canhelp Genomics, ChinaReviewed by:
Hamid Morjani, Université de Reims Champagne-Ardenne, FranceVandna Kukshal, Washington University School of Medicine in St. Louis, United States
Copyright © 2021 Li, Zhang, Feng, Cheng, Cai, Dai, Zhao, Li, Huang, Fang, Zhu, Wang, Wang, Ma and Lu. This is an open-access article distributed under the terms of the Creative Commons Attribution License (CC BY). The use, distribution or reproduction in other forums is permitted, provided the original author(s) and the copyright owner(s) are credited and that the original publication in this journal is cited, in accordance with accepted academic practice. No use, distribution or reproduction is permitted which does not comply with these terms.
*Correspondence: Xianghe Lu, ZHJfbHV4aEBzaW5hLmNvbQ==; Tonghui Ma, dG9uZ2h1aW1hMDgxOEBzaW5hLmNvbQ==