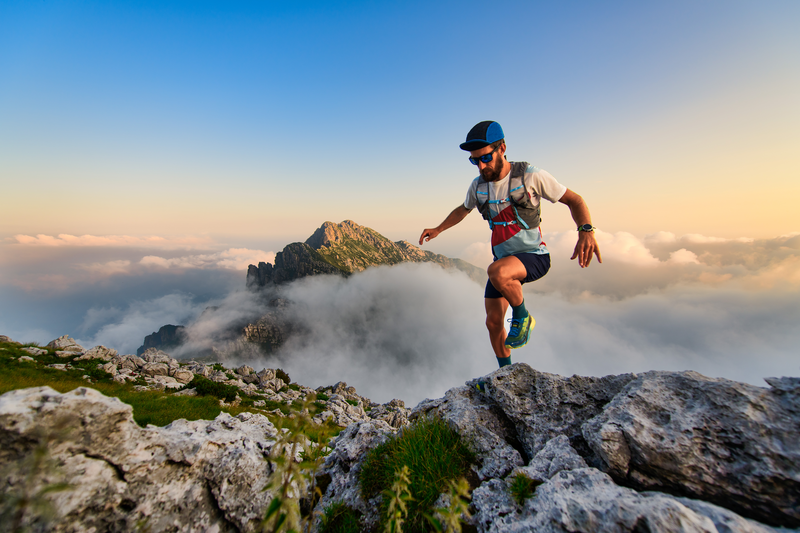
94% of researchers rate our articles as excellent or good
Learn more about the work of our research integrity team to safeguard the quality of each article we publish.
Find out more
ORIGINAL RESEARCH article
Front. Oncol. , 10 March 2021
Sec. Molecular and Cellular Oncology
Volume 11 - 2021 | https://doi.org/10.3389/fonc.2021.536346
This article is part of the Research Topic Targeting the Wnt/β-catenin Signaling Pathway in Cancer View all 10 articles
The molecular mechanisms by which uterine leiomyoma (UL) cells proliferate are unclear. Long noncoding RNA (lncRNA) is reported to participate in the occurrence and development of gynecological cancers. We investigated the molecular mechanisms that lncRNA uses in UL. We found that lncRNA Alu-mediated p21 transcriptional regulator (APTR) showed higher expression in UL tumor tissues compared with that in normal uterine tissues. APTR induced cell proliferation and colony formation both in vitro and in vivo. The JASPAR database showed that APTR was likely interacted with ERα, and these molecules were identified via laser scanning confocal microscopy and RNA immunoprecipitation analysis. To verify the correlation between APTR and ERα, we overexpressed and underexpressed APTR and simultaneously expressed ERα. The results showed that APTR function was suppressed. APTR increased the expressions of the proteins in the Wnt pathway, and inhibiting ERα eliminated these responses. In conclusion, our data suggest that APTR promoted leiomyoma cell proliferation through the Wnt pathway by targeting ERα, suggesting a new role of APTR in the Wnt signaling pathway in UL.
►APTR induced uterine leiomyoma cell proliferation and colony formation
► APTR directly interacted with ERα and they were co-localized in the nucleus
►APTR promoted leiomyoma cell proliferation through the Wnt pathway by targeting ERα
Uterine leiomyoma (UL) is the most common benign gynecological tumor, with an incidence rate of 30–40% in fertile women (1). UL may lead to asymptomatic complications such as uterine bleeding, severe dysmenorrhea, pelvic pain and infertility and is the leading indication for a hysterectomy (2). However, because the typical marriage age has increased, and many women have not completed childbearing, hysterectomies are often contraindicated (3). Therefore, nonsurgical treatments are urgently needed, and understanding the specific molecular mechanism of UL cell proliferation may improve patients’ quality of life.
In vitro and in vivo experiments have shown that UL is an estrogen-dependent tumor, and estrogen affects UL promotion and progression (4). Estrogen binds to its receptors and initiates transcription, then promotes UL cell proliferation (5). Estrogen receptors include estrogen receptors (ERs) α and β, and estrogenic action can be influenced by selective estrogen receptors (6). However, whether ERα or ERβ mediate estrogen function and how estrogen affects UL cell proliferation remain unclear.
Aberrant activation of Wnt/β-catenin has been detected in ER-positive breast cancer cells (7). β-Catenin is involved in the canonical Wnt signaling pathway, regulating diverse sets of cellular activities including cell proliferation (8). Enhanced nuclear β-catenin can accelerate interphase by shortening the cell cycle phase and can activate cyclin-D1 and c-Myc (9).
Long noncoding RNA (lncRNA) is reported to participate in gynecological cancers and is associated with the epithelial-mesenchymal transition (EMT) (10). H19 generates miR-675, an EMT-associated gene in prostate cancer (11). LncRNA is also reported to participate in endometrial carcinoma occurrence and development (12).
LncRNA Alu-mediated p21 transcriptional regulator (APTR) is the inhibitor that represses the p21 promoter in human glioblastomas (13). APTR is reported to play a crucial role in osteosarcoma (14), be a potential biomarker for liver cirrhosis (15) and have potential diagnostic value for papillary thyroid cancer (16). In this study, we examined whether APTR plays a pivotal role in UL progression and elucidated the possible mechanism.
Three patients were included in the study, and UL tumor tissue and adjacent normal uterine tissue specimens were acquired at the follicular phase. Inclusion criteria for this study were patients who (a) were nonmenopausal, (b) had undergone a hysterectomy because of UL between 12/2016 and 08/2017, (c) were without medical complications, and (d) had no history of hormone therapy. Paraffin-embedded tissues of 34 UL patients were included in the study, and all histopathologic evaluations were performed by experienced pathologists from the Shanghai First Maternity and Infant Hospital.
Ht-UtLM primary cells were collected from 30 patients who had undergone total hysterectomies at the follicular phase and were selected according to the above criteria. All were diagnosed by a pathologist. The detailed steps were previously described (17). Ht-UtLM-1 and the Ht-UtLM-2 came from the same patient’s primary cells. Because the primary cells cannot be subcultured, primary cells from different patients were used for different experiments and there were total 30 patients selected. It was investigated the cells culture was mycoplasma-free.
The Hospital’s Protection of Human Subjects Committee approved the study protocols. Samples were acquired with written informed consent from the Shanghai First Maternity and Infant Hospital affiliated with Tongji University School of Medicine.
Immunohistochemical staining was performed using the two-step plus Poly-HRP Anti-IgG Detection System (ZSGB-Bio, Beijing, China) per the manufacturer’s recommendations. Primary antibodies targeting ERα (C-311; cat. no. sc-787, Santa Cruz, CA, USA), ERβ (B-3; cat. no. 373853, Santa Cruz), Ki-67 (cat. no. 373853, Santa Cruz) and β-catenin (12F7; cat. no. sc-59737, Santa Cruz) were used.
Total RNA extraction and reverse transcription were performed per the manufacturer’s protocol. Semi-quantitative RT-PCR was performed using the standard protocol from the SYBR Green PCR kit (Toyobo, Osaka, Japan). GAPDH was used as the reference mRNA. DCt values were normalized to GAPDH levels. The 2–△△Ct method was used to measure the gene expression levels. The primer pairs used were as follows: human lncRNA APTR forward: 5′-AGTAGCAGGAGACAGCAT-3′, reverse: 5′-TGACAGCCTTCCACAATC-3′; α-SMA forward: 5′-CTTTCAAGCTGTTCCTGTC-3′, reverse: 5′-TGTGTTTCTCCTCTGTCC-3′; vimentin forward: 5′-GATGGTGTTTGGTCGCATA-3′, reverse: 5′- CGAATGCGCAGCACCAG-3′; KRT-19 forward: 5′-GGCGCCACCATTGAGAA CT-3′, reverse: 5′-GCCAGGCGGGCATTG-3′; and GAPDH forward: 5′-GGCTCCCTTGGGTATATGGT-3′, reverse: 5′-TTGATTTTGGAGGGATCTCG-3′.
Western blot analysis was performed to assess protein expression as previously described (18). Primary antibodies included anti-GAPDH (cat. no. G9545) purchased from Sigma, all others were purchased from Santa Cruz, including anti-ERα (sc-8002), anti-KRT-19 (sc-376126), anti-α-SMA (sc-53142), anti-vimentin (sc-6260), anti-actin (sc-8432), anti-c-Myc (sc-373712), anti-β-catenin (sc-7963) and anti-cyclin-D1 (sc-8396).
The full-length nucleotide sequence for APTR was obtained from the FLJ cDNA library. It was then inserted at the 3´ end of the APTR in the lentivirus vector (Invitrogen, V49810). The shRNA was constructed as previously described (19).
Cells were cultured in 10% Dulbecco’s modified Eagle’s medium containing 10% fetal bovine serum (Wisent, Canada) at 37°C with 5% CO2. For the colony-forming assay, cells (6 × 102) were transfected and incubated for 14 days. Colonies (>50 cells) were counted manually and plotted as previously described (18).
Cells were plated and grown for 96 h. The number of cells was determined using the 3-(4,5-dimethylthiazol-2yl)-2,5-diphenyltetrazolium bromide (MTT) assay as previously described (20).
RIP analysis to assess RNA-binding protein expression was performed as previously described (21). Primary antibodies included mouse monoclonal antibody against ERα (cat. no. sc-787, Santa Cruz) and mouse monoclonal antibody against actin (C-2; cat. no. sc-8432, Santa Cruz).
Cells were seeded at 1 × 105 cells/well in a 24-well cell plate one day prior to transfection with Superfect according to the manufacturer’s protocol (Tiangen Biotech co LTD, Beijing, China). Luciferase activity was normalized for transfection efficiency using the mutant promoter reporter plasmid, FOPflash (vs. TOPflash, the un-mutated plasmid), as an internal control (22).
Total RNA was extracted from cells using TRIzol™ Reagent (#15596026, Invitrogen, Carlsbad, CA, USA) per the manufacturer’s protocol, and ribosomal RNA was removed using the Ribo-Zero rRNA Removal Kit (Epicentre, Madison, WI, USA). Fragmented RNA (average length of ~200 bp) were subjected to first-strand and second-strand cDNA synthesis followed by adaptor ligation and enrichment with a low cycle per the instructions of the NEBNext® Ultra™ RNA Library Prep Kit for Illumina (NEB, USA). The purified library products were evaluated using the Agilent 2200 TapeStation and Qubit® 2.0 (Life Technologies, USA). The libraries were paired-end sequenced (PE150, sequencing reads were 150 bp) using the Illumina HiSeq Xten platform.
Fastp software (v0.17.0) was used to trim adaptor and remove low quality reads to get high quality clean reads. mRNA/LncRNA: hisat2 software (v2.04) was used to align the high quality clean reads to the human reference genome (UCSC hg19). Then, guided by the Ensembl GTF gene annotation file (v75), cuffdiff software (part of cufflinks, v2.2.1) was used to get the gene level FPKM as the expression profiles of mRNA, transcript level FPKM as the expression profiles of LncRNA, and fold change and p-value were calculated based on FPKM, differentially expressed mRNAs/LncRNAs were identified by cuffdiff software. Gene Ontology and KEGG pathway enrichment analysis were performed based on the differentially expressed mRNAs and LncRNA nearby genes. This part of the experiment was performed by Shanghai Yinxi Biomedical Technology Co., Ltd.
Ht-UtLM cells (5 × 106) with stably upregulated APTR were subcutaneously implanted into 4- to 6-week-old BALB/c nude mice purchased from Shanghai SLAC Laboratory Animal Company. Tumor growth was measured using a digital caliper every 5 days for 30 days. The mice were sacrificed on day 30 after cell implantation, and the tumor weights were measured.
The ERαcDNA and ERαRNAi plasmid were purchased from Origene (Maryland, USA) (23).
The cDNA encoding APTR was subcloned into the NheI and XhoI sites of pSL-MS2-12x vector (Addgene), named pSL-MS2-APTR, the sequence is as follow: ACTR-F-NheI 5’-TAGCTAGCAGTCCCGCTGACACCTT-3’, ACTR-R-XhoI 5’-ACCTCGAGAACCGTGAGTCCATTAAACCTC -3’. A digoxin (Roche, Mannheim, Germany)-labeled lncRNA-APTR complementary DNA probe was synthesized in vitro used for RNA fluorescence in situ hybridization (FISH). The following procedures were performed as previously described (24) without slight modification.
All experiments were repeated in triplicate. Data are expressed as the mean ± SD. Statistical significance between two groups was determined using Student’s t-test. P<0.05 was considered statistically significant.
UL tissues and adjacent normal uterine tissues from three patients were used to confirm the APTR expression levels. Illumina HiSeq was used to further verify the higher expression of APTR. APTR expressions in the tissues from the three patients were significantly increased compared with those of the normal tissues (Figures 1A, B).
Figure 1 LncRNA expression was high in leiomyoma tumors. (A, B) Differences in RNA transcription were detected between normal uterine tissues and leiomyoma tumors. High-level pathway sequencing showed that α-SMA expression was higher in leiomyoma tumors. (C) Gel electrophoresis, real-time PCR, and Western blot analyses confirmed higher α-SMA expression and lower vimentin and KRT-19 expression in leiomyoma tumors. (D) Gel electrophoresis and real-time PCR analyses confirmed higher APTR expression in leiomyoma tumors. (*P < 0.05, **P < 0.01).
Increased levels of α-SMA and decreased levels of vimentin and KRT-19 identified the leiomyoma tumor cells (Figure 1C). Next, real-time PCR analysis confirmed the higher APTR levels in the UL tissues (Figure 1D). Figures 1C, D showed one representative sample.
APTR was overexpressed in UL tumor cells. Thus, we investigated the role of APTR in regulating cell proliferation. We constructed APTR-overexpression and APTR-knockdown lentiviral vectors, infected Ht-UtLM cells, and selected stably infected cell clones for further study. The vectors were compared to a control (Figure 2A). MTT analysis showed that APTR-infected cells grew much faster than did the controls (Figures 2B, C). Colony-formation assays revealed that APTR-infected cells formed larger and more numerous colonies than did the controls (Figures 2D, E). Conversely, shRNA-infected cells had slower proliferation rates and formed smaller and fewer colonies than did the controls.
Figure 2 LncRNA APTR induced leiomyoma tumor cell proliferation. Ht-UtLM primary cells derived from leiomyoma tumors were used. (A) Stably infected Ht-UtLM cells were generated and identified. Real-time PCR analyses confirmed the efficiency. (B, C) Cell proliferation testing was conducted using MTT assays. APTR overexpression increased cell proliferation. Conversely, knocking down APTR decreased cell proliferation. (D, E) Colony-formation assays were performed on stably overexpressed or underexpressed APTR for 2 weeks. (F) Tumors from xenograft-transplanted nude mice 30 days after subcutaneously injecting APTR-overexpressing or control Ht-UtLM cells. (G) Xenograft volumes 30 days after cell injection. (H) Xenograft weights 30 days after cell injection.
The stably infected cells were subcutaneously transplanted into BALB/c nude mice. We suspended the cells at 5 × 106 cells/ml, and 100 μl were injected into the flanks of nude mice (n=5). We measured tumor sizes starting 5 days postinjection using the formula, W2 × L, every 5 days for 30 days. Mice were then euthanized, and the tumors were excised (Figure 2F). Tumor sizes (Figure 2G) and weights (Figure 2H) from APTR-overexpressing cells were increased compared with the controls.
According to the JASPAR database, lncRNA is likely interacted with ERα (Figure 3A). ENST00000447009 is lncRNA APTR, with an interaction strength of 10%. We next explored whether APTR interacts with ERα. The location of APTR was confirmed using FISH assay; APTR and ERα were co-localized in the nucleus (Figure 3B). We used RIP to verify the correlation between ERα and APTR, and the results showed that APTR is likely interacted with ERα (Figure 3C).
Figure 3 LncRNA APTR induced tumor growth by targeting ERα. LncRNA APTR was likely interacted with ERα. (A) LncRNA ENST00000447009 was lncRNA APTR. According to the JASPAR database, APTR was likely interacted with ERα. (B) Ht-UtLM cells with elevated APTR expression were seeded in 96-well plates. FISH analysis showed that ERα (red) co-localized with APTR (green) in the nuclei of Ht-UtLM cells. (C) RIP showed that APTR was in contact with ERα. (D) Ht-UtLM cells were transfected with either ERα cDNA or shRNA plasmids. ERα expression was analyzed by western blot. (E) Cell viability was assessed by MTT assay daily for 4 days. (F) Colony formation assays were performed on Ht-UtLM cells for 2 weeks. (G) Ht-UtLM cells that stably overexpressed or underexpressed APTR were screened via real-time PCR. (H, I) After overexpressing or underexpressing APTR or ERαRNAi, the cell viability was assessed using MTT assays daily for 4 days. (J, K) Colony-formation assays were performed on cells stably overexpressed or underexpressed for 2 weeks. (*P < 0.05).
In leiomyoma tumor cells, ERα promoted proliferation, as did APTR. Therefore, the correlation between ERα and APTR were measured. Western blot analysis was used to verify the overexpression and underexpression of ERα (Figure 3D). MTT analysis showed that ERα overexpression cells grew much faster than controls (Figure 3E). Colony formation assays also revealed that ERα overexpression cells formed larger and more colonies than controls (Figure 3F). Conversely, ERα α cells had slower proliferation rates and formed smaller and fewer colonies than controls.
APTR levels were overexpressed and underexpressed (Figure 3G). The effect of APTR on growth was abolished when simultaneously infected with ERαRNAi (Figures 3H, I). Colony-formation assays revealed the same results. Colonies formed by the APTR- and ERαRNAi-infected cells did not significantly differ from those formed by the control cells (Figures 3J, K).
By bioinformatic analysis, β-catenin promoter has four ERα binding sites (Figure 4A). β-catenin promoter luciferase reporter (TOPflash) was used to investigate the regulation of β-catenin by APTR. The Ht-UtLM cells were coinfected with APTR or shRNA and TOPflash, and the activity was examined. The results showed that TOPflash activity was increased by cotransfection with APTR and decreased by shRNA (Figures 4B, C). When the cells were cotransfected with both APTR and ERαRNAi or shRNA and ERαRNAi, both cell groups lost their responses (Figure 4D).
Figure 4 APTR activated the canonical Wnt pathway in Ht-UtLM cells by targeting ERα. (A) β-Catenin promoter has four ERα binding sites. (B) Cells were infected with or without APTR together with the TCF-luciferase reporter (TOPflash) or mutant TCF-luciferase reporter (FOPflash) for 24 h. Upregulated APTR increased TOPflash activation in Ht-UtLM cells. (C) Downregulated APTR repressed TOPflash activation in Ht-UtLM cells. (D) Infection with APTR and ERαRNAi did not affect the basal TOPflash activity. (E, F) Ht-UtLM cells were infected with APTR or shRNA alone or together with the ERαRNAi. Western blot analysis was performed with GAPDH as the loading control. (*P < 0.05, **P < 0.01).
Western blotting was used to further validate the effect of APTR. APTR upregulated β-catenin expression and simultaneously indirectly upregulated c-Myc and cyclin-D1, downstream proteins of the Wnt signaling pathway. Conversely, the β-catenin and downstream cyclin-D1 and c-Myc expression levels did not differ from those of the control groups. When the cells were coinfected with both APTR and ERαRNAi or shRNA and ERαRNAi, the changes in protein expressions between the two groups did not significantly differ (Figures 4E, F). These results indicated that APTR activated the Wnt pathway by targeting ERα.
In this study, we identified a new effect of lncRNA APTR, which targets the p21 promoter. We found high APTR expression levels in the leiomyoma tumor tissues using Illumina HiSeq and real-time PCR experiments to examine leiomyoma tumor tissues and adjacent normal cells. Bioinformatics analyses showed that APTR is likely interacted with ERα. Knocking down the ERα level abolished the effect of APTR on UL cell proliferation. This effect on APTR reveals a new role for ERα in the Wnt pathway and represents the first identification of the lncRNA and ERα pathway.
APTR represses the CDKN1A/P21 promoter, which has been correlated with cell proliferation in several cancers (13, 15). Downregulation of APTR has been correlated with tumorigenesis in papillary thyroid cancer and anaplastic thyroid cancer (16). In cirrhotic patients with portal hypertension, APTR was considered a prognostic marker, and higher APTR expression was associated with a poor prognosis (25).
Given the recently identified role of APTR, we first detected APTR expression in leiomyoma tissues and adjacent normal tissues. As expected, APTR had a higher expression in leiomyoma tissues. Further investigation of APTR’s function would be of interest since APTR overexpression promoted proliferation of the UL cell line, but underexpression of APTR had the opposite effect. Until now, the mechanism of APTR regulation as it relates to UL cell proliferation was unclear.
Long noncoding RNA (lncRNA) with more than 200 nucleotides is considered a transcript and is not translated into proteins (26). With little primary sequence conservation, many lncRNAs are localized to the nucleus (27), while some can encode small proteins (28). Bioinformatics analysis revealed that APTR was likely interacted with ERα, and we verified this using RIP. To further assess the function, we overexpressed APTR and underexpressed ERα simultaneously, which inhibited the effect of APTR. Underexpressing APTR and ERα simultaneously also inhibited the effect.
UL is reported to be estrogen-dependent (29), and estrogen that was uptaken or exposed bound to the estrogen receptor by acting with an estrogen-like effect (4). Estrogen can overexpress ERα and upregulate IGF-1 expression (30) and the VEGF pathways (31). The ERα signaling pathway can crosstalk with the TGF-β signaling pathway and mediate estrogen to promote UL cell proliferation (32).
Variant estrogen receptors have also been reported, such as ERα36, which is located in the mitochondria in Ht-UtLM cells. These receptors are associated with mitochondrial proteins and considered to be nongenomic signals with pivotal functions. Bisphenol A induced UL cell proliferation through ERα36 as a nongenomic signaling pathway (33).
ERβ was also found in UL. ERα expression was shown to be higher than that of ERβ (34). In our study, specimens were taken at the follicular phase, and ERβ had low expression in leiomyoma tumor tissues (Supplemental Figure 1). Furthermore, the effect on the UL cell proliferation was related to ERα.
Interestingly, in this study, the effect of APTR on UL cell proliferation was offset by ERα. Studies have reported that different APTR levels affect the cellular biological activity; for example, knocking down APTR inhibited TGF-β1-induced upregulation of α-SMA in hepatic stellate cells (15).
Aberrant activated Wnt/β-catenin was detected in this study. We also detected β-catenin activity in ER-positive UL patients, which verified the effect of APTR in the Wnt/β-catenin pathway. Knocking down ERα depleted this effect. We also found a new checkpoint for ERα. LncRNA APTR promotes UL cell proliferation by activating the Wnt/β-catenin pathway, and ERα was the target of the effect of APTR.
Current studies are done in primary cells from only one patient for one and the same experiment, to avoid patient-specific issues, results will be confirmed in more patients in the near future.
The original contributions presented in the study are publicly available. This data can be found here: [https://www.ncbi.nlm.nih.gov/geo/query/acc.cgi?acc=GSE168497/GSE168497].
The studies involving human participants were reviewed and approved by The Hospital’s Protection of Human Subjects Committee. The patients/participants provided their written informed consent to participate in this study. The animal study and study protocols were reviewed and approved by The Hospital’s Protection of Human Subjects Committee. Written informed consent was obtained from the individual(s) for the publication of any potentially identifiable images or data included in this article.
All authors contributed equally to this paper. All authors contributed to the article and approved the submitted version.
The authors declare that the research was conducted in the absence of any commercial or financial relationships that could be construed as a potential conflict of interest.
This study was supported by the National Natural Science Foundation of China (81871126), the Foundation Project of Shanghai Municipal Commission of Health and Family Planning (201640248), and the Shanghai Pudong new area health and family planning commission (PW2018D-13). We thank Traci Raley, MS, ELS, from Liwen Bianji, Edanz Editing China (www.liwenbianji.cn/ac) for editing a draft of this manuscript.
The Supplementary Material for this article can be found online at: https://www.frontiersin.org/articles/10.3389/fonc.2021.536346/full#supplementary-material
Supplementary Figure 1 | IHC staining shows ERα and ERβ expressions in uterine leiomyoma tumor compared to adjacent normal uterine tissues. The expressions of ERα and ERβ in uterine leiomyoma tumor and adjacent normal uterine tissues. The scale bar is 100 μm.
1. Salimi S, Khodamian M, Narooie-Nejad M, Hajizadeh A, Fazeli K, Namazi L, et al. Association of polymorphisms and haplotypes in the cytochrome P450 1B1 gene with uterine leiomyoma: A case control study. BioMed Rep (2015) 3:201–6. doi: 10.3892/br.2014.413
2. Falcone T, Walters MD. Hysterectomy for benign disease. Obstet Gynecol (2008) 111:753–67. doi: 10.1097/AOG.0b013e318165f18c
3. Sato S, Maekawa R, Yamagata Y, Tamural I, Lee L, Okada M, et al. Identification of uterine leiomyoma-specific marker genes based on DNA methylation and their clinical application. Sci Rep (2016) 6:30652. doi: 10.1038/srep30652
4. Ishikawa H, Ishi K, Sema VA, Kakazu R, Bulun SE, Kurita T. Progesterone is essential for maintenance and growth of uterine leiomyoma. Endocrinology (2010) 15:2433–42. doi: 10.1210/en.2009-1225
5. Chuffa LG, Seiva FR, Fávaro WJ, Amorim JP, Teixeira GR, Mendes LO, et al. Melatonin and ethanol intake exert opposite effects on circulating estradiol and progesterone and differentially regulate sex steroid receptors in the ovaries, oviducts, and uteri of adult rats. Reprod Toxicol (2013) 39:40–9. doi: 10.1016/j.reprotox.2013.04.001
6. Ellmanns S, Sticht H, Thiel F, Bechmann MW, Strick R, Strissel PL. Estrogen and progesterone receptors: from molecular structures to clinical targets. Cell Mol Life Sci (2009) 66:2405–26. doi: 10.1007/s00018-009-0017-3
7. Sakunrangsit N, Ketchart W. Plumbagin inhibits cancer stem-like cells, angiogenesis and suppresses cell proliferation and invasion by targeting Wnt/β-catenin pathway in endocrine resistant breast cancer. Pharmacol Res (2019) 3:104517. doi: 10.1016/j.phrs.2019.104517
8. Mohammed MK, Shao C, Wang J, Wei Q, Wang X, Collier Z, et al. Wnt/β-catenin signaling plays an ever-expanding role in stem cell self-renewal, tumorigenesis and cancer chemoresistance. Genes Dis (2016) 3:11–40. doi: 10.1016/j.gendis.2015.12.004
9. Farahani R, Rezaei-Lotfi S, Simonian M, Hunter N. Bi-modal reprogramming of cell cycle by MiRNA-4673 amplifies human neurogenic capacity. Cell Cycle (2019) 18:848–68. doi: 10.1080/15384101.2019.1595873
10. Lin X, Qiu J, Hua K. Long non-coding RNAs as emerging regulators of epithelial to mesenchymal transition in gynecologic cancers. Biosci Trends (2018) 12:342–53. doi: 10.5582/bst.2018.01181
11. Zhu M, Chen Q, Liu X, Sun Q, Zhao X, Deng R, et al. lncRNA H19/miR-675 axis represses prostate cancer metastasis by targeting TGFBI. FEBS J (2014) 281:3766–75. doi: 10.1111/febs.12902
12. Li Q, Shen F, Zhao L. The relationship between lncRNA PCGEM1 and STAT3 during the occurrence and development of endometrial carcinoma. BioMed Pharmacother (2018) 107:918–28. doi: 10.1016/j.biopha.2018.08.091
13. Negishi M, Wongpalee SP, Sarkar S, Park J, Lee KY, Shibata Y, et al. A new lncRNA, APTR, associates with and represses the CDKN1A/p21 promoter by recruiting polycomb proteins. PloS One (2014) 9:e95216. doi: 10.1371/journal.pone.0095216
14. Guan H, Shang G, Cui Y, Liu J, Sun X, Cao W, et al. Long noncoding RNA APTR contributes to osteosarcoma progression through repression of miR-132-3p and upregulation of yes -associatied protein 1. J Cell Physiol (2019) 234:8998–9007. doi: 10.1002/jcp.27572
15. Yu F, Zheng J, Mao Y, Dong P, Li G, Lu Z, et al. Long non-coding RNA APTR promotes the activation of hepatic stellate cells and the progression of liver fibrosis. Biochem Biophys Res Commun (2015) 463:679–85. doi: 10.1016/j.bbrc.2015.05.124
16. Zhang K, Li C, Liu J, Li Z, Ma C. Down-Regulation of APTR and it’s Diagnostic Value in Papillary and Anaplastic Thyroid Cancer. Pathol Oncol Res (2018) 26(1):559–65. doi: 10.1007/s12253-018-0561-y
17. Lee SM, Choi ES, Ha E, Ji KY, Shin SJ, Jung J. Gyejibongnyeong Hwan (Gui Zhi Fu Ling Wan) Ameliorates Human Uterine Myomas via Apoptosis. Front Pharmacol (2019) 10:1105. doi: 10.3389/fphar.2019.01105
18. Zhang Y, Bao W, Wang K, Lu W, Wang H, Tong H, et al. SOX17 is a tumor suppressor in endometrial cancer. Oncotarget (2016) 7:76036–46. doi: 10.18632/oncotarget.12582
19. Gui D, Peng W, Jiang W, Huang G, Liu G, Ye Z, et al. Ubiquitin-specific peptidase 46 (USP46) suppresses renal cell carcinoma tumorigenesis through AKT pathway inactivation. Biochem Biophys Res Commun (2019) 19:689–96. doi: 10.1016/j.bbrc.2019.09.036
20. Zhang Y, Jiang F, Bao W, Zhang H, He X, Wang H, et al. SOX17 increases the cisplatin sensitivity of an endometrial cancer cell line. Cancer Cell Int (2016) 16:29. doi: 10.1186/s12935-016-0304-7
21. Gagliardi M, Matarazzo MR. RIP: RNA Immunoprecipitation. Methods Mol Biol (2016) 1480:73–86. doi: 10.1007/978-1-4939-6380-5_7
22. Khan S, Kaur R, Shah BA, Malik F, Kumar A, Bhushan S, et al. A Novel cyano derivative of 11-Keto-beta-boswellic acid causes apoptotic death by disrupting PI3K/AKT/Hsp-90 cascade, mitochondrial integrity, and other cell survival signaling events in HL-60 cells. Mol Carcinog (2012) 51:679–95. doi: 10.1002/mc.20821
23. Xu N, Shen C, Luo Y, Xia L, Xue F, Xia Q, et al. Upregulated miR-130a increases drug resistance by regulating RUNX3 and Wnt signaling in cisplatin-treated HCC cell. Biochem Biophys Res Commun (2012) 425:468–72. doi: 10.1016/j.bbrc.2012.07.127
24. Xiangsong WU, Wang F, Li H, Hu Y, Jiang L, Zhang F, et al. LncRNA-PAGBC acts as a microRNA spong and promotes gallbladder tumorigenesis. EMBO Rep (2017) 10):1837–53. doi: 10.15252/embr.201744147
25. Yu S, Qi Y, Jiang J, Wang H, Zhou Q. APTR is a prognostic marker in cirrhotic patients with portal hypertension during TIPS procedure. Gene (2018) 645:30–3. doi: 10.1016/j.gene.2017.12.040
26. Kopp F, Mendell JT. Functional Classification and Experimental Dissection of Long Noncoding RNAs. Cell (2018) 172:393–407. doi: 10.1016/j.cell.2018.01.011
27. Li W, Notani D, Rosenfeld MG. Enhancers as non-coding RNA transcription units: recent insights and future perspectives. Nat Rev Genet (2016) 17:207–23. doi: 10.1038/nrg.2016.4
28. Matsumoto A, Pasut A, Matsumoto M, Yamashita R, Fung J, Monteleone E, et al. mTORC1 and muscle regeneration are regulated by the LINC00961-encodedSPAR polypeptide. Nature (2017) 541:228–32. doi: 10.1038/nature21034
29. Islam MS, Protic O, Giannubilo SR, Toti P, Tranguilli AL, Petraglia F, et al. Uterine leiomyoma: available medical treatments and new possible therapeutic options. J Clin Endocrinol Metab (2013) 98:921–34. doi: 10.1210/jc.2012-3237
30. Shen Y, Ren ML, Feng X, Gao YX, Xu Q, Cai YL. Does nonylphenol promote the growth of uterine fibroids? Eur J Obstet Gynecol Reprod Biol (2014) 178:134–7. doi: 10.1016/j.ejogrb.2014.04.038
31. Shen Y, Ren ML, Feng X, Cai YL, Gao YX, Xu Q. An evidence in vitro for the influence of bisphenol A on uterine leiomyoma. Eur J Obstet Gynecol Reprod Biol (2014) 178:80–3. doi: 10.1016/j.ejogrb.2014.03.052
32. Shen Y, Lu Q, Zhang P, Wu Y, Ren M. The effect of TGF-β signaling on regulating proliferation of uterine leiomyoma cell via ERα signaling activated by bisphenol A, octylphenol and nonylphenol in vitro. J Cancer Res Ther (2018) 14(Supplement):S276–81. doi: 10.4103/0973-1482.235342
33. Yu L, Das P, Vall AJ, Yan Y, Gao X, Sifre MI, et al. Bisphenol A induces human uterine leiomyoma cell proliferation through membrane-associated ERα36 via nongenomic signaling pathways. Mol Cell Endocrinol (2019) 484:59–68. doi: 10.1016/j.mce.2019.01.001
Keywords: long noncoding RNA (lncRNA) Alu-mediated p21 transcriptional regulator (APTR), uterine leiomyoma, proliferation, ERα, Wnt pathway
Citation: Zhou W, Wang G, Li B, Qu J and Zhang Y (2021) LncRNA APTR Promotes Uterine Leiomyoma Cell Proliferation by Targeting ERα to Activate the Wnt/β-Catenin Pathway. Front. Oncol. 11:536346. doi: 10.3389/fonc.2021.536346
Received: 12 March 2020; Accepted: 01 February 2021;
Published: 10 March 2021.
Edited by:
Zhe-Sheng Chen, St. John’s University, United StatesReviewed by:
Xiaozhuo Liu, University at Buffalo, United StatesCopyright © 2021 Zhou, Wang, Li, Qu and Zhang. This is an open-access article distributed under the terms of the Creative Commons Attribution License (CC BY). The use, distribution or reproduction in other forums is permitted, provided the original author(s) and the copyright owner(s) are credited and that the original publication in this journal is cited, in accordance with accepted academic practice. No use, distribution or reproduction is permitted which does not comply with these terms.
*Correspondence: Yongli Zhang, ZG9jdG9yemhhbmcyQDE2My5jb20=
Disclaimer: All claims expressed in this article are solely those of the authors and do not necessarily represent those of their affiliated organizations, or those of the publisher, the editors and the reviewers. Any product that may be evaluated in this article or claim that may be made by its manufacturer is not guaranteed or endorsed by the publisher.
Research integrity at Frontiers
Learn more about the work of our research integrity team to safeguard the quality of each article we publish.