- Department of Pathology and Molecular Pathology, University of Zurich and University Hospital Zurich, Zurich, Switzerland
Clear cell renal cell carcinoma (ccRCC) displays a highly varying clinical progression, from slow growing localized tumors to very aggressive metastatic disease (mRCC). Almost a third of all patients with ccRCC show metastatic dissemination at presentation while another third develop metastasis during the course of the disease. Survival rates of mRCC patients remain low despite the development of novel targeted treatment regimens. Biomarkers indicating disease progression could help to define its aggressive potential and thus guide patient management. However, molecular markers that can reliably assess metastatic dissemination and disease recurrence in ccRCC have not been recommended for clinical practice to date. Liquid biopsies could provide an attractive and non-invasive method to determine the risk of recurrence or metastatic dissemination during follow-up and thus assist the search for surveillance biomarkers in ccRCC tumors. A wide spectrum of circulating molecules have already shown considerable potential for ccRCC diagnosis and prognostication. In this review, we outline state of the art of the key circulating analytes such as cfDNA, cfRNA, proteins, and exosomes that may serve as biomarkers for the longitudinal monitoring of ccRCC progression to metastasis. Moreover, we address some of the prevailing limitations in the past approaches and present promising adoptable technologies that could help to pursue the implementation of liquid biopsies as a prognostic tool for mRCC.
Introduction
Kidney cancer is the seventh most frequent cancer worldwide and is responsible for nearly 100,000 deaths each year. Clear cell renal cell carcinoma (ccRCC), the most common subtype of RCC, accounts for almost 75% of detected cases and is therefore far more frequently studied than the rarer histologies (1). One of the landmark events in its tumorigenesis is loss of the short arm of chromosome 3p on which the VHL tumor suppressor is encoded. This is often concurrent with a gain of chromosome 5q resulting in the generation of a small population of tumor-initiating cells (2). Consequently, the inactivation of the second copy of the VHL gene heralds the development of clinically aggressive ccRCC (2). Genetically, ccRCC is characterized by high intra-tumor heterogeneity (3, 4). Recurrent somatic mutations found in ccRCCs occur in the epigenetic regulators PBRM1, SETD2 and BAP1, all of which are also located on chromosome 3p and are therefore prone to inactivation similar to VHL (5). These specific genetic changes are reflected at the RNA and protein levels, for instance, by activation of the HIF-pathway and a corresponding increase in expression of angiogenesis-related mRNA signatures and hypoxic signaling, which are direct consequences of VHL inactivation (6). Extensive metabolic reprogramming is another result of the genetic changes that occur during ccRCC initiation and progression and this is increasingly recognized to correlate with aggressive disease (7). This is exemplified by the inactivation of the pyruvate dehydrogenase complex (PDC) which in turn impairs the Krebs cycle and oxidative phosphorylation resulting in a metabolic shift toward glycolysis (8). Importantly, metabolic rewiring in ccRCC has been shown to induce HIF-signaling independent of VHL through signaling pathways that involve for example mTOR and MET. This metabolic distortion could influence epigenetic changes and chromatin dysregulation, contributing to the aggressiveness of the tumor (9).
In contrast to primary ccRCCs, which often show a high number of subclonal drivers, metastatically progressed disease sites have a more homogenous molecular landscape. They contain fewer somatic mutations indicating the excerption of only those clones that are metastatically competent. Conserved trajectories have been identified to lead to metastasis, with PBRM1 mutations often predicating dissemination (10). Other hallmark genomic alterations that lead to metastasis are the loss of chromosome 9p and 14q. Interestingly, microRNA (miRNA) signatures are also disparate between the primary and metastatic sites, with several miRNAs associating with worse patient outcomes (11). A prominent example is miR-30c, which showed decreased expression in metastatic disease corresponding to lower progression-free survival (PFS). This finding is in line with its observed function in cell adhesion and invasion (12).
Importantly, the clinical diagnosis of ccRCC is most often incidental. Almost 30% of ccRCC patients already present with metastatic disease while another 30% develop metastasis later during the course of the disease (1). The prognosis for metastatic RCC (mRCC) is still relatively dismal with a variable spectrum of overall survival (OS) times ranging from less than 6 months to more than 5 years (13). It is therefore clear that accurate prognostic and risk identification strategies that enable the early prediction of recurrences could impact ccRCC clinical management (Figure 1). In fact, the likelihood of a favorable response to treatment is superior with limited metastatic burden (14). However, no specific molecular marker has been recommended for this clinical use to date (15). Liquid biopsies are emerging as a minimally invasive, rapid, and cost-effective tool to determine cancer markers in biological liquids such as blood or urine (Figure 1) (16, 17). The source for these potential biomarkers is the “circulome”, which refers to the molecules released into circulation from all tissue, including the tumor tissue. Therefore, liquid biopsies may contain tumor-specific information in the form of circulating tumor cells (CTCs), circulating tumor DNA (ctDNA), circulating tumor RNA (ctRNA), secreted proteins, extracellular vesicles, metabolites, and tumor-educated platelets. Currently, a small number of non-invasive blood tests that detect ctDNA are used as companion diagnostic tool for cancers such as non-small cell lung carcinoma (NSCLC), prostate and colorectal carcinoma. These tests are mainly used as rationales for treatment decisions, for example to detect activating mutations in the Epidermal Growth Factor Receptor (EGFR) that can be treated by administering Osimertinib in patient with NSCLC (18). Alongside, several liquid biopsy tests are under investigation in clinical trials as reviewed by Heidrich et al. (19). Among the putative markers with prognostic relevance in mRCC, ctDNA, ctRNA, proteins and exosomes are currently under heavy examination. In this review, we will provide a brief overview of the recent developments in the identification of circulating biomarkers that are indicative of a metastatic lesion and which allow the identification of disease recurrence in ccRCC patients. Moreover, we present several novel and promising technologies that could overcome some of the current limitations of liquid biopsy analysis that have been roadblocks to implementing them as a prognostic tool with clinical utility (Figure 2).
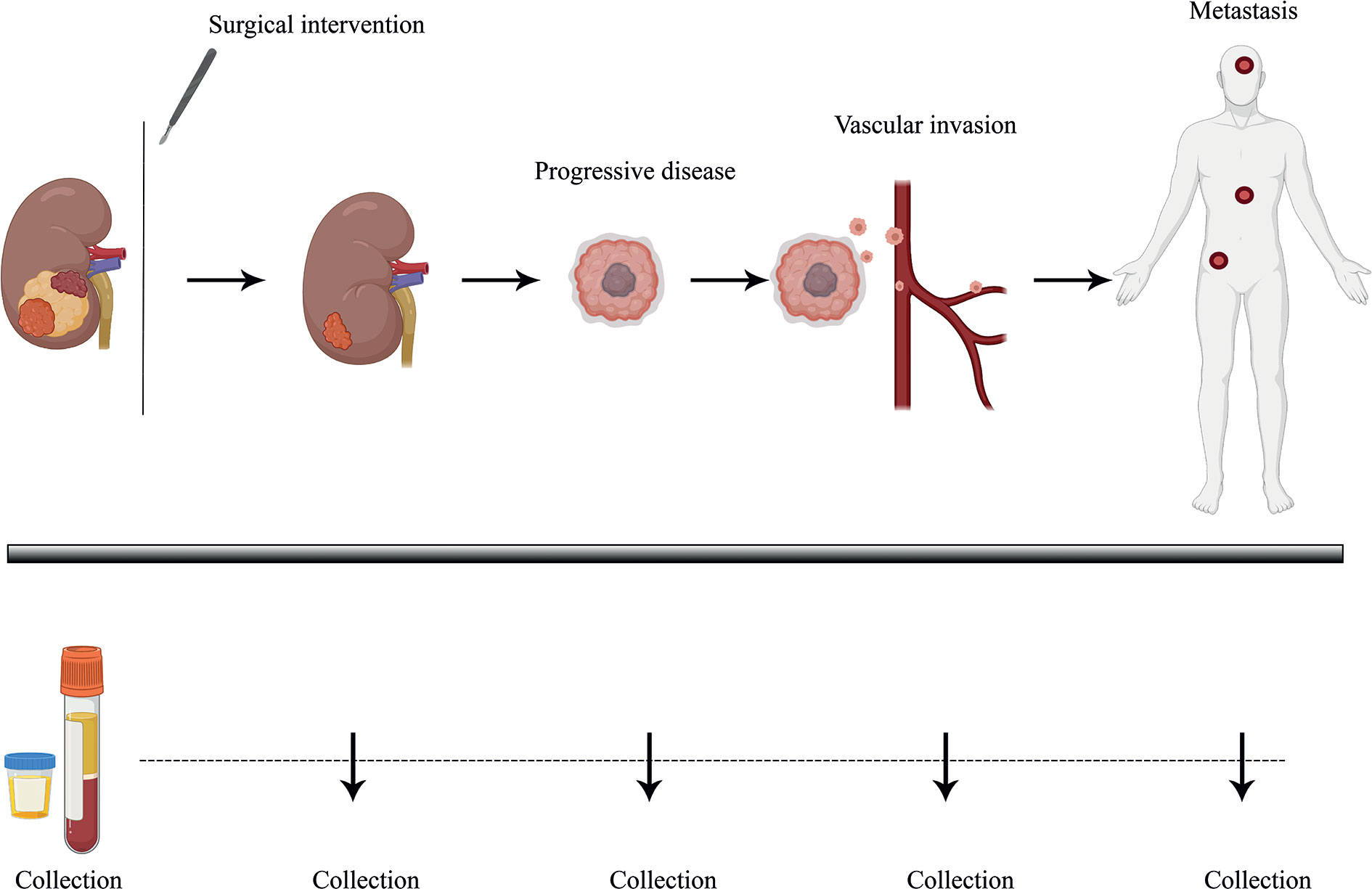
Figure 1 Longitudinal monitoring of disease progression via liquid biopsy. Liquid biopsy presents as a minimally invasive prognostic technique, allowing the surveying of disease burden and progression in patients through biological liquid samples such as blood and urine. ccRCC patients, who tend to show high variability in disease progression, could benefit from better therapeutic response and PFS (Progression-free survival) with continuous follow-up of tumor molecular profiling through analyzing tumor-specific circulating biomarkers over time. Plasma and urine samples could be collected over several time points and profiled via ultra-sensitive analytical techniques, helping guide clinical management strategies.
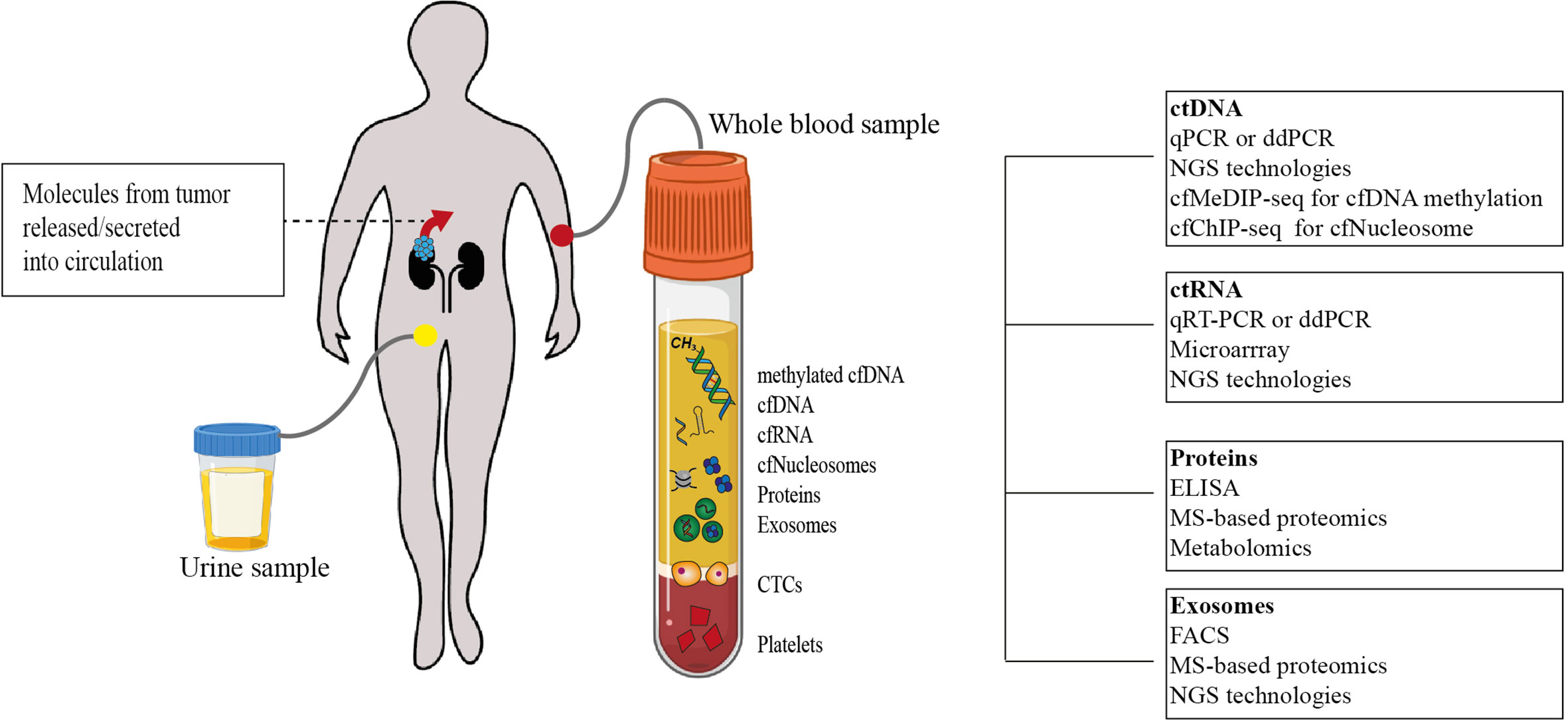
Figure 2 Tumor-specific circulome and technologies used for their analysis. Tumor-specific circulating biomarkers can include several molecules involved in tumorigenesis and tumor progression. Analytes that have been studied as potential biomarkers so far are depicted. Using a variety of techniques, their quantification at a single time point may allow disease staging and prognostication (cfDNA. cell-free DNA; cfRNA. cell-free RNA; cfNucleosomes. cell-free nucleosomes; CTCs, circulating tumor cells; ctDNA, circulating tumor DNA; qPCR, quantitative polymerase chain reaction; ddPCR, droplet digital polymerase chain reaction; NGS, next-generation sequencing; cfMeDIP-seq, cell-free methylated DNA immunoprecipitation sequencing; cfChIP-seq, cell-free chromatin immunoprecipitation sequencing; ctRNA, circulating tumor RNA; ELISA, enzyme-linked immunosorbent assay; MS, Mass spectrometry; FACS, fluorescence-activated cell sorting).
Circulating Tumor DNA
The highly aggressive and vascularized nature of ccRCC prompted the intuitive expectation that tumor material, such as DNA, could be shed into circulation constituting a powerful tool to profile the tumor genome bypassing the need for a tissue biopsy. We have identified a number of studies, using the search terms “Renal cell carcinoma” and “Circulating tumor DNA” from public databases, that have investigated such possibilities (Table 1). Early reports paved the way by demonstrating the feasibility of genetic analysis from liquid biopsies, initially proposing the use of cell-free DNA (cfDNA) concentration and fragmentation as a guide for predicting and following the progression toward mRCC. Repeatedly, cfDNA concentrations were shown to be significantly higher in patients with advanced or metastatic disease compared to healthy individuals and patients with localized tumors (Table 1). Interestingly, analysis of the housekeeping gene ACTB as a surrogate measure of cfDNA concentration showed a consistent and significant elevation in RCC patients compared to healthy controls (21). Additionally, Wan et al. reported that the average plasma cfDNA level was significantly higher in metastatic tumors than in localized disease indicating that they could even be reflective of ccRCC progression (22). Even though these observations are noteworthy, both studies reported only moderate sensitivity and specificity for the alterations and thus further validation is required to clarify the clinical benefit of cfDNA concentrations as a circulating biomarker.
Fragmentation of cfDNA has also been studied as a diagnostic and prognostic marker in RCC patients (28, 30). Several groups performed these analyses using marker DNA fragments from genes like ACTB, GAPDH and APP as well as Alu short interspersed nucleotide elements and the mitochondrial DNA fragments Mito-1 and Mito-2 (20, 21, 24). Lu et al. could correlate shorter cfDNA fragments of the gene amyloid beta (A4) precursor protein (APP) with prognostic factors for recurrence-free and OS in patients with ccRCC and the cfDNA integrity index calculated based on the ratio of these fragment concentrations showed a decreased trend from controls to mRCC patients (24). Similarly, mitochondrial and Alu elements showed increased fragmentation and lower cfDNA integrity in RCC patients. However, when analyzing DNA integrity using ACTB and GAPDH as markers, cfDNA fragmentation was increased in RCC compared to controls (20, 21). While it has been shown that cfDNA fragmentation could be a valuable biomarker, further work needs to clarify which genetic elements have to be selected to ensure a complete visualization of the cfDNA fragment landscape and its diagnostic and prognostic potential (24). ctDNA has been identified in renal cancer patients of all stages but the probability of detection increased with the tumor size indicating that advanced disease stages may be better reflected in liquid biopsies (29). However, a number of studies reported ctDNA to be much less abundant in liquid biopsy samples from RCC patients compared with those from other cancers and several groups showed that ccRCC-specific ctDNA could be detected in only about 30%–50% patients (23, 25, 26, 29, 31). In a recent study, Bacon et al. used the Roche SeqCap EZ Human Oncology Panel to analyze the coding regions of 981 cancer-related genes in plasma cfDNA of 55 mRCC patients (31). Even this comprehensive analysis could only detect evidence for RCC-derived ctDNA, such as a somatic mutation in more than one established RCC genes, in a third of the patients. It is noteworthy that this was the first study that accounted for non-RCC specific somatic clones in liquid biopsies which can stem from clonal hematopoiesis of indeterminate potential (CHIP) by analysis of patient-matched leukocyte DNA. This could have decreased the sensitivity toward cfDNA mutations while increasing the sensitivity to RCC-derived ctDNA mutations. In addition, this study reveals that the blood-borne ctDNA fraction was as low as 3.9%, which is considerably less than in metastatic breast or lung cancer (32, 33).
Two other recent studies have reported higher numbers of ctDNA-positive patients. One of the largest studies by Pal et al., which included 220 mRCC patients, promisingly reported RCC-specific alterations in genes such as VHL, TP53, EGFR, NF1, and ARID1A in almost 80% of the patients when using a driver gene deep sequencing approach, with great sensitivity to mutant cfDNA fragments (<1%) (27). This apparent discrepancy in detecting tumor-specific mutations in the aforementioned study could be due to two factors: Although Pal et al. analyzed a larger cohort, only 56% of the samples were histologically characterized, of which 70% were classified as ccRCC. In comparison, the cohort used by Bacon et al. was completely histologically classified and contained 85% ccRCC patients. Moreover, Pal et al. did not account for non-RCC specific somatic clones which may be a rather large contributor to the genetic alterations observed in RCC liquid biopsies.
A second recent and sophisticated pipeline to detect cell-free tumor DNA in RCC patients’ plasma and urine samples was introduced by Smith et al. and was termed as a “personalized method” of ctDNA sequencing. Similar to other studies, sequencing of plasma cfDNA alone could identify ctDNA in a third of the RCC patients, including those with mRCC. However, when the personalized method that was based on prior sequencing of the primary tumor tissue and subsequent assessment of the known mutations in corresponding liquid biopsies was applied ctDNA detection rates improved to ~50% (29). In addition, in a small cohort of patients, the authors could show the prognostic value of ctDNA analysis by revealing that longitudinal plasma sampling could track disease progression. Another important finding from this study is that plasma ctDNA represented 90% of the disparate mutations found in multiple biopsy regions from individual tumors of two well characterized ccRCC patients and thus indicated that ctDNA could be used to circumvent tumor sampling bias that might be present in conventional tissue biopsies. This is an attractive approach to overcome the well-established tumor heterogeneity present in ccRCCs (34).
Taken together, a number of studies indicate RCC as a ctDNA-low malignancy by showing that only 30%–50% of patients benefitted from characterization of ccRCC-specific ctDNA using the currently available profiling technologies (23, 25, 26, 29, 31). Despite these drawbacks, ctDNA was detected more frequently in plasma amongst patients with larger tumors and hence longitudinal sampling could be used to monitor the course of the disease at least in a subset of advanced patients. Even though cfDNA analysis does not seem to enable straightforward surveillance at the moment, novel technologies could significantly improve this situation in the future.
Proteins and Oncometabolites
Liquid biopsies could help to further investigate the proteomic landscape reflecting the changes triggered, for example, by extravasation of the ccRCC into circulation. Blood, abundant with proteins, is an inviting medium for exploring disease-related markers but it is technically challenging to mine tumor-specific signatures amidst highly abundant plasma proteins and other soluble factors. Studies identified with a keyword search for “renal cell carcinoma”, “liquid biopsy or plasma”, “protein or proteome” were further selected based on their diagnostic and prognostic value and are discussed in this section. A number of these studied utilized different experimental approaches in order to identify proteins with differential abundance in RCC plasma or serum relative to controls (Table 2; Please refer to Clark and Zhang Clin Proteom 2020 (52) for a comprehensive review). Historically, one of the most extensively studied proteins in this context is the Kidney Injury Molecule 1 (KIM1). KIM1 levels were found to be significantly increased in RCC patients (36). In fact, high grade ccRCCs showed an almost 7-fold increase in KIM1 abundance and mRCC patients displayed particularly high KIM1 levels in their plasma (47, 49). Despite the rather widespread expression of KIM1 in several renal diseases (53), circulating KIM1 showed 83% specificity in detecting early stage tumors with an increase to 97% specificity in later stages (47). While this makes it a promising biomarker, further studies need to validate the clinical utility of KIM1 as a ccRCC-specific circulating protein. Acknowledging the involvement of VHL mutations in ccRCC tumorigenesis, proteins downstream of the hypoxia-pathway represent a class of interesting soluble markers. For instance HIG2, a hypoxia inducible protein, was elevated approximately 3-fold in the plasma of RCC patients in an ELISA-based study and its abundance decreased drastically after nephrectomy (38). CAIX, one of the most prominent targets of the VHL-HIF-pathway, also emerged as a potential biomarker showing increased protein concentrations and activity in the plasma of ccRCC patients compared controls (45). Similarly, high IMP3 levels have been observed in RCC patients and correlated with the development of distant metastasis (48). Additionally, high levels of soluble CD27 were detected in sera of ccRCC patients and in vitro analyses suggested that this was triggered by the high expression of the HIF-target gene CD70 (43). These examples illustrate how the knowledge of ccRCC-specific cellular aberrations can be leveraged in the search for candidate biomarkers. Nevertheless, looking beyond the VHL-HIF-pathway, the TNF-related apoptosis-inducing ligand (TRAIL) was identified as a potential biomarker showing a 2-fold decrease in RCC patient sera and being highly predictive of venous invasion and metastasis (41).
Despite their initial promise, none of these circulating protein markers were clinically approved. Subsequently, large-scale proteomic technologies were utilized in order to provide a deeper characterization of ccRCC-specific protein assisting the search for candidate liquid biomarkers (Figure 2). An earlier study could distinguish RCC patients from non-RCC and healthy controls by using SELDI-TOF and applying pattern analysis based on five proteins with masses in the range of 3,900–5,900 Da (35). Similar studies have identified other peaks at 4,151 and 8,968 m/z that significantly differed between RCC and healthy controls and had an overall specificity of 80% (37). These even provided evidence for the utility of individual proteins including factor XIIIB, complement C3, misato homolog 1, hemopexin, alpha-1-B-glycoprotein (39) and HSC71 (44) as RCC-specific soluble biomarkers. Moreover, using MALDI-TOF, RNA-binding protein 6 (RBP6), tubulin beta chain (TUBB), and zinc finger protein 3 (ZFP3) were found to reduce following surgical intervention (40). Taken together, these findings underscore the opportunity to use the plasma proteome for longitudinal disease monitoring.
Much like it has been utilized with ctDNA, linking liquid biopsy protein profiles to those of the primary RCC could provide important complimentary data for the search of candidate biomarkers. In an interesting discovery study, White et al. used LC-MS/MS analysis to identify differentially expressed proteins in ccRCC compared to normal kidney tissue (42). From this analysis, heat shock protein beta-1 (HSPB1/Hsp27) emerged as a promising candidate and consequently the utility of Hsp27 as a useful non-invasive marker was confirmed in patient sera. Besides being elevated in serum and urine of ccRCC patients, Hsp27 was also associated with high grade (Grade 3–4) tumors.
Finally, it makes intuitive sense that the assessment of soluble immune-checkpoint proteins could have the added benefit of predicting immunotherapy responses besides their diagnostic or prognostic potential alone. Soluble factors such as sLAG3, sPD-L2, sBTLA, and sTIM3 were observed in higher concentrations in ccRCC patients and were significantly correlated with survival, death-risk and recurrence (50). Moreover, these proteins have already been developed as biomarkers for immune therapy prediction in several other cancer types (54). Apart from the proteome, other oncometabolites originating from metabolic processes such as amino acid metabolism, hormone synthesis and lipid transport including leucine, N-lactoyl-leucine, N-acetly-phenylalanine, hydroxylprolyl-valine, cortolone, and testosterone have been nominated as potential liquid biomarkers in RCC patients (46, 51). A panel consisting of the metabolites cortolone, testosterone and l-2-aminoadipate adenylate was able to distinguish RCC patients from benign renal tumors with 100% specificity at 75% sensitivity, indicating an increased effectiveness in discrimination when combining groups of biomarkers that are involved in disturbed metabolic pathways (51).
Taken together, several studies have already investigated RCC-specific metabolites and in particular proteins but a large proportion of these have only examined patient samples in comparison to healthy controls in order to delineate aberrant expression patterns specific for RCC diagnosis. Even though interesting markers have been nominated, information on the prognostic value of many of these candidates is still lacking. In addition, large-scale deep proteomic characterization has revealed various potential biomarkers but due to the lack of validation in clinical cohorts, very little can be used conclusively to define a panel of markers for ccRCC monitoring. Novel mass spectrometry and multi-marker based approaches are awaited to provide more comprehensive insights and a deeper understanding of potential secretory protein markers and their prognostic value in ccRCC.
Circulating RNA and Exosomes
Cell-free RNA either enters the blood through active release from cells in extracellular vesicles like exosomes or conjugated to proteins (55–57). Coding RNA such as messenger RNA (mRNA) as well as small non-coding RNAs like miRNA and lncRNA have presented themselves as potential liquid biopsy biomarkers (Figure 2). Search terms “renal cell carcinoma”, “Circulating RNA or mRNA or miRNA or lncRNA” yielded studies that were pruned to select a smaller collection with relevant clinical value for ccRCC prognosis. So far, miRNAs remain the most frequently studied class of RNA molecules probably owing to the shorter half-life of mRNA and the relative novelty of lncRNA (Table 3). Several miRNAs which have previously been studied in the context of cancer progression and development, have also been proposed as liquid biomarkers in ccRCC. One of the most interesting examples is miR-210, which is known to be regulated by the VHL/HIF-pathway (58) and has emerged as a novel indicator for ccRCC tumor burden. Elevated levels of circulating miR-210 have been reported in patient sera and following nephrectomy they were observed to decrease in the urine of disease-free patients during follow-up (61, 62). Several other miRNAs still remain to be explored as biomarkers in circulation. Studies in primary ccRCC tissue have identified 65 miRNAs, including miR-215, which were significantly different between patients with mRCC and localized ccRCCs (72). Whether these miRNAs can indicate metastatic dissemination of the primary tumor in liquid biopsies has not been not investigated to date. Circulating miRNAs that have already been implicated in predicting mRCC include miR-122-5p, miR-206 (63), and miR-221. Importantly, out of these miR-221 has also been shown to significantly correlate with lower survival (59). In addition, the combination of serum miR-508-3p and miR-885-5p could differentiate ccRCC patients, and these miRNAs have been implicated in the positive regulation of metabolic processes such as inositol phosphate metabolism and in the Hippo and Wnt signaling pathways that have been implicated in ccRCC tumorigenesis (64). Studying these miRNAs in metastatic patients and establishing their regulatory roles in ccRCC would likely improve their value as a circulating biomarker.
Messenger RNA is a crucial intermediate in relaying genetic changes to the protein level and may therefore reflect mutational and regulatory changes in the tumor. However, technical difficulties in detecting tumor-specific mRNA in patients’ blood has limited its development as a biomarker for disease monitoring. Recently, novel sequencing technologies have provided impetus to further investigate the potential of circulating mRNA and consequently CDK18 and CCND1 messengers were shown to be downregulated in blood of ccRCC patients (65) (Table 2). Similarly, an increase in lysyl oxidase (LOX) expression marked metastatic samples from the same cohort (65). In addition, circulating lncRNA (73), one of the newer players in the field of small non-coding RNAs, also showed promise as a RCC-specific biomarker. A signature of 5 lncRNAs (lncRNA-LET, PVT1, PANDAR, PTENP1, and linc00963) could distinguish RCC samples from controls with a specificity of 91% at 67% sensitivity in a training set independent of stage classification. An increase to 76% sensitivity was observed when the training set was limited to controls and stage I ccRCC patients, indicating good discrimination even for less advanced patients (60).
Exosomes are nanoscale secreted membrane-bound vesicles that play a role in cellular communication by transferring signaling molecules as packaged cargo. One of the most frequent cargo is miRNA, while several other molecules including DNA, proteins and other classes of RNAs are transported in exosomes as well (74). Studied far more in urine than in blood, these vesicles are observed to contain molecules that are capable of differentiating and identifying ccRCC and mRCC. Comparable to circulating miR-210, exosomal miR-210 was elevated in ccRCC patient sera and could distinguish ccRCC patients from healthy individuals, albeit only with a specificity of 62% (71). Considering that circulating levels of miR-210 have been described as relevant biomarkers in the context of cell-free analytes and as exosomal cargo, this is nevertheless one of the more promising molecules warranting further studies to assess its diagnostic and prognostic potential as a liquid biomarker for the clinical routine. Several other exosomal miRNAs or their combinations such as enumerated in Table 2 were also able to differentiate RCC patients from healthy controls and supported the proposed use of exosomal cargo as potential biomarkers in ccRCC (67, 69). Additionally the non-coding transcript lncARSR (activated in RCC with Sunitinib Resistance) that is transmitted via exosomes, showed increased levels in the serum of RCC patients, decreased after tumor resection and subsequently increased again during tumor relapse making it an interesting candidate for non-invasive disease monitoring (68). A small number of studies have investigated the potential of exosomal protein markers (66, 70) giving first insights into their differential abundance between healthy and tumor patients. Interestingly, comprehensive protein cargo analysis by LC/MS revealed that azurocidin (AZU1) was significantly enriched in tumor‐derived exosomes and these may even play a functional role in driving metastatic dissemination (70).
Despite the initial reports, neither lncRNA nor exosomal miRNAs or proteins have been thoroughly investigated as potential biomarkers for the metastatic disease yet. Large sample volumes and complicated and expensive processing set-ups appear as major roadblocks in this search. Therefore, technical advances are needed to improve the current approaches and pave the way to further investigate and translate RNA- or exosome-based cancer detection in the clinical setting.
Future Avenues in Liquid Biopsy
Evidently, the field of liquid biopsy analysis is quickly evolving and has shown considerable promise for anticipating cancer progression, for example in lung, breast and colorectal cancer (75–77). However, currently there is still insufficient evidence for the clinical utility of majority of the circulating molecules in many advanced cancers as well as in ccRCC (78). Several approaches are under heavy investigation and their successful implementation could provide further rationales for using liquid biopsies as a tool for ccRCC patient management (Figure 2). However, one of the most important aspects for consideration in any further developments is the need to validate the emergence of potential liquid biomarkers in larger patient cohorts in order to consider them as specific and sensitive non-invasive markers of clinical utility. Currently, many interesting studies have sought to assess differential features between samples from healthy and diseased individuals. Significantly more work will be required to obtain a deeper understanding of the prognostic potential of the candidate markers as they should not only be prioritized based on their discriminatory benefit but also for their value in disease monitoring, e.g., to indicate metastatic dissemination.
One of the rather unexpected obstacles in developing a clinically applicable liquid biopsy analysis platform was the low ctDNA abundance that has so far hindered ctDNA from becoming a simple alternative to tissue biopsy in diagnosing and tracking mRCC (23, 25, 26, 29, 31, 79). Nevertheless, mutant fragments derived from RCC cells have been identified in patients’ plasma and the mutations closely mirrored the known landscape of the primary tumors (31). Several studies indicated that there are opportunities for liquid biopsies in the longitudinal follow-up of ccRCC patients provided additional improvements will be made in isolation and detection approaches. Among the main challenges is the concomitant presence of mutant fragments from non-RCC somatic clones stemming, for example, from CHIP which was identified as a main cause for the discordance between plasma and tissue RCC samples (31, 79). Incorporating appropriate controls such as white blood cell DNA could prove to be essential to eliminate variants arising from such unrelated somatic expansions. Combining personalized mutations identified from archival tumor tissue is another attractive strategy that was proposed to improve the sensitivity of ctDNA detection in mRCC (25, 29, 79). Conversely, most recently, a novel approach that is based on genome-wide mutational signal integration has challenged the paradigm of increasing the sequencing depth of a limited set of target genes for reliable ctDNA detection (80). By placing the emphasis on broadening the mutational landscape, this genome-wide single-nucleotide variant (SNV) detection platform showed evidence for allowing ultra-sensitive detection even at low-sequencing depths as well as enabling quantitative dynamic monitoring of disease burden. Thus, this approach could prove to be an attractive alternative to overcome the low ccRCC-specific ctDNA abundance and significantly increase detection sensitivity in the future.
Epigenetic regulation presents a wide avenue to explore ccRCC specific patterns, particularly since chromatin remodelers are among the most frequently altered factors. Based on the principle that tumor cells acquire aberrant DNA methylation, cell-free methylated DNA immunoprecipitation sequencing (cfMeDIP Seq) was able to markedly improve sensitivity for detecting patients with mRCC. The assessment of top differentially methylated regions of the cell-free methylome could also distinguish ccRCC and control samples (81). Since blood cfDNA is derived from fragmented chromatin, it often remains associated with histones that may contain evidence of the epigenetic landscape of the cells they originate from (82, 83). Thus, circulating cell-free nucleosomes could become interesting targets for observing mRCC-specific changes in expression programs that are often imposed by alteration in SETD2, PBRM1, or BAP1. Chromatin immunoprecipitation sequencing of cell-free nucleosomes (cfChIP-seq) has emerged capable of identifying cell-of-origin expression marks as well as changes in gene activity and transcription in gastrointestinal cancers (82). Extrapolating this to ccRCC could open a new window to provide detailed information about the state of the disease from liquid biopsy analysis.
Novel proteomic technologies will also bolster the development of circulating biomarkers. To aid the acquisition of ccRCC-specific peptides, pre-fractionation with the aim to either remove high-abundance proteins or enrich certain proteins could be employed. In addition, novel MS-based proteomic approaches such as Microflow LC-MS/MS (84) and Trapped Ion Mobility Spectroscopy (TIMS) (85) constitute valuable technologies for biomarker discovery. These, combined with higher throughput, will likely help to identify mRCC-specific proteins in liquid biopsies. A promising impetus for further studies in this domain was provided with the identification of several proteins differing in abundance with the infiltration of ccRCC into the renal vasculature using nano-scale liquid chromatographic tandem electrospray ionization mass spectrometry (nLC-ESI-MS/MS) for the proteomic analysis of urine and plasma (86).
Circulating RNAs (circRNA) represent a newly discovered class of small non-coding RNAs that have recently come into light as potential biomarkers for kidney diseases. Studies leveraging primary tissue collections have identified several combinations of these circRNA that are capable of identifying ccRCC (87, 88) and also correlated with tumor grade (80). Importantly, circRNAs have already been discovered as exosomal cargo in urine and plasma from patients with Idiopathic Membranous Nephropathy (89), raising the interesting possibility that these molecules could also be studied as indicators of renal cancer. Exosomes show high potential as a useful vehicle for tracking disease progression and dissemination by virtue of their aiding intercellular communication. Due to their small size and low density, recovery from plasma or urine remains the limiting step towards straightforward isolation, detection, and quantification (90). A novel chemical affinity-based capture method has been developed for extracellular vesicle isolation (EVtrap) from plasma, which showed a 7-fold increase in capture compared to ultracentrifugation and can potentially ameliorate this problem. In a promising proof-of-concept study, phosphoproteomic analysis of RCC plasma samples revealed several proteins capable of distinguishing five RCC patients from five healthy controls (91) indicating that EVtrap could be exploited to develop additional markers for disease monitoring.
It is also becoming increasingly apparent that the best definition of tumor status and prognosis may arise from the simultaneous study of various complimentary constituents of the circulome and thus, a multi-marker based approach may prove to be useful toward developing reliable biomarkers for disease surveillance (92). Harnessing the indicative potential of several of the molecules described in this review together may in fact be key to achieving prognostic utility in ccRCC liquid biopsy profiling.
Conclusion
Liquid biopsy analysis offers a range of complementary information through the circulome and has the potential to cause a major breakthrough in clinical oncology. In contrast to conventional tissue biopsy, it may even be able to capture a larger amount of the molecular heterogeneity described for ccRCCs and inform about aggressive clones that have disseminated toward the metastatic niche. Before this potential can be realized, a number of hurdles remain but given the rapid pace of technological development there is an air of optimism regarding its utility, especially for monitoring the metastatic progression in ccRCC patients.
Author Contributions
HL and HB contributed to the conception of the review article. HL, HB, and DR drafted the manuscript. PS and HM critically revised the manuscript. All authors contributed to the article and approved the submitted version.
Funding
This work was supported by the Swiss National Science Foundation (SNSF grant number 310030_166391).
Conflict of Interest
The authors declare that the research was conducted in the absence of any commercial or financial relationships that could be construed as a potential conflict of interest.
Acknowledgments
Figures in the review were created with www.Biorender.com.
References
1. Hsieh JJ, Purdue MP, Signoretti S, Swanton C, Albiges L, Schmidinger M, et al. Renal cell carcinoma. Nat Rev Dis Primers (2017) 3:17009. doi: 10.1038/nrdp.2017.9
2. Mitchell TJ, Turajlic S, Rowan A, Nicol D, Farmery JHR, O’Brien T, et al. Timing the Landmark Events in the Evolution of Clear Cell Renal Cell Cancer: TRACERx Renal. Cell (2018) 173(3):611–623.e17. doi: 10.1016/j.cell.2018.02.020
3. Gerlinger M, Rowan AJ, Horswell S, Math M, Larkin J, Endesfelder D, et al. Intratumor Heterogeneity and Branched Evolution Revealed by Multiregion Sequencing. N Engl J Med (2012) 366(10):883–92. doi: 10.1056/NEJMoa1113205
4. Bolck HA, Corrò C, Kahraman A, von Teichman A, Toussaint NC, Kuipers J, et al. Tracing Clonal Dynamics Reveals that Two- and Three-dimensional Patient-derived Cell Models Capture Tumor Heterogeneity of Clear Cell Renal Cell Carcinoma. Eur Urol Focus (2019) 11. doi: 10.1016/j.euf.2019.06.009
5. Bihr S, Ohashi R, Moore AL, Rüschoff JH, Beisel C, Hermanns T, et al. Expression and Mutation Patterns of PBRM1, BAP1 and SETD2 Mirror Specific Evolutionary Subtypes in Clear Cell Renal Cell Carcinoma. Neoplasia (2019) 21(2):247–56. doi: 10.1016/j.neo.2018.12.006
6. Beuselinck B, Verbiest A, Couchy G, Job S, de Reynies A, Meiller C, et al. Pro-angiogenic gene expression is associated with better outcome on sunitinib in metastatic clear-cell renal cell carcinoma. Acta Oncol (2018) 57(4):498–508. doi: 10.1080/0284186X.2017.1388927
7. The Cancer Genome Atlas Research Network. Comprehensive molecular characterization of clear cell renal cell carcinoma. Nature (2013) 499(7456):43–9. doi: 10.1038/nature12222
8. Ricketts CJ, Cubas AAD, Fan H, Smith CC, Lang M, Reznik E, et al. The Cancer Genome Atlas Comprehensive Molecular Characterization of Renal Cell Carcinoma. Cell Rep (2018) 23(1):313–326.e5. doi: 10.1016/j.celrep.2018.03.075
9. Jones PA, Baylin SB. The epigenomics of cancer. Cell (2007) 128(4):683–92. doi: 10.1016/j.cell.2007.01.029
10. Turajlic S, Xu H, Litchfield K, Rowan A, Chambers T, Lopez JI, et al. Tracking Cancer Evolution Reveals Constrained Routes to Metastases: TRACERx Renal. Cell (2018) 173(3):581–594.e12. doi: 10.1016/j.cell.2018.03.057
11. Lukamowicz-Rajska M, Mittmann C, Prummer M, Zhong Q, Bedke J, Hennenlotter J, et al. MiR-99b-5p expression and response to tyrosine kinase inhibitor treatment in clear cell renal cell carcinoma patients. Oncotarget (2016) 7(48):78433–47. doi: 10.18632/oncotarget.12618
12. Heinzelmann J, Unrein A, Wickmann U, Baumgart S, Stapf M, Szendroi A, et al. MicroRNAs with Prognostic Potential for Metastasis in Clear Cell Renal Cell Carcinoma: A Comparison of Primary Tumors and Distant Metastases. Ann Surg Oncol (2014) 21(3):1046–54. doi: 10.1245/s10434-013-3361-3
13. Assi HI, Patenaude F, Toumishey E, Ross L, Abdelsalam M, Reiman T. A simple prognostic model for overall survival in metastatic renal cell carcinoma. Can Urol Assoc J (2016) 10(3–4):113–9. doi: 10.5489/cuaj.3351
14. Antonelli A, Cozzoli A, Zani D, Zanotelli T, Nicolai M, Cunico SC, et al. The follow-up management of non-metastatic renal cell carcinoma: definition of a surveillance protocol. BJU Int (2007) 99(2):296–300. doi: 10.1111/j.1464-410X.2006.06616.x
15. Escudier B, Porta C, Schmidinger M, Rioux-Leclercq N, Bex A, Khoo V, et al. Renal cell carcinoma: ESMO Clinical Practice Guidelines for diagnosis, treatment and follow-up. Ann Oncol (2019) 30(5):706–20. doi: 10.1093/annonc/mdz056
16. Kwapisz D. The first liquid biopsy test approved. Is it a new era of mutation testing for non-small cell lung cancer? Ann Transl Med (2017) 5(3):46. doi: 10.21037/atm.2017.01.32
17. Mattox AK, Bettegowda C, Zhou S, Papadopoulos N, Kinzler KW, Vogelstein B. Applications of liquid biopsies for cancer. Sci Trans Med (2019) 11(507):3. doi: 10.1126/scitranslmed.aay1984
18. Rijavec E, Coco S, Genova C, Rossi G, Longo L, Grossi F. Liquid Biopsy in Non-Small Cell Lung Cancer: Highlights and Challenges. Cancers (Basel) (2019) 12(1):17. doi: 10.3390/cancers12010017
19. Heidrich I, Ačkar L, Mossahebi Mohammadi P, Pantel K. Liquid biopsies: Potential and challenges. Int J Cancer (2020). ijc.33217. doi: 10.1002/ijc.33217
20. Gang F, Guorong L, An Z, Anne G-P, Christian G, Jacques T. Prediction of Clear Cell Renal Cell Carcinoma by Integrity of Cell-free DNA in Serum. Urology (2010) 75(2):262–5. doi: 10.1016/j.urology.2009.06.048
21. Hauser S, Zahalka T, Ellinger J, Fechner G, Heukamp LC, Ruecker AV, et al. Cell-free Circulating DNA: Diagnostic Value in Patients with Renal Cell Cancer. Anticancer Res (2010) 5.
22. Wan J, Zhu L, Jiang Z, Cheng K. Monitoring of Plasma Cell-Free DNA in Predicting Postoperative Recurrence of Clear Cell Renal Cell Carcinoma. UIN (2013) 91(3):273–8. doi: 10.1159/000351409
23. Bettegowda C, Sausen M, Leary RJ, Kinde I, Wang Y, Agrawal N, et al. Detection of Circulating Tumor DNA in Early- and Late-Stage Human Malignancies. Sci Transl Med (2014) 6(224):224ra24. doi: 10.1158/1538-7445.AM2014-5606
24. Lu H, Busch J, Jung M, Rabenhorst S, Ralla B, Kilic E, et al. Diagnostic and prognostic potential of circulating cell-free genomic and mitochondrial DNA fragments in clear cell renal cell carcinoma patients. Clin Chim Acta (2016) 452:109–19. doi: 10.1016/j.cca.2015.11.009
25. Corrò C, Hejhal T, Poyet C, Sulser T, Hermanns T, Winder T, et al. Detecting circulating tumor DNA in renal cancer: An open challenge. Exp Mol Pathol (2017) 102(2):255–61. doi: 10.1016/j.yexmp.2017.02.009
26. Maia MC, Bergerot PG, Dizman N, Hsu J, Jones J, Lanman RB, et al. Association of Circulating Tumor DNA (ctDNA) Detection in Metastatic Renal Cell Carcinoma (mRCC) with Tumor Burden. Kidney Cancer (2017) 1(1):65–70. doi: 10.3233/KCA-170007
27. Pal SK, Sonpavde G, Agarwal N, Vogelzang NJ, Srinivas S, Haas NB, et al. Evolution of Circulating Tumor DNA Profile from First-line to Subsequent Therapy in Metastatic Renal Cell Carcinoma. Eur Urol (2017) 72(4):557–64. doi: 10.1016/j.eururo.2017.03.046
28. Yamamoto Y, Uemura M, Nakano K, Hayashi Y, Wang C, Ishizuya Y, et al. Increased level and fragmentation of plasma circulating cell-free DNA are diagnostic and prognostic markers for renal cell carcinoma. Oncotarget (2018) 9(29):20467–75. doi: 10.18632/oncotarget.24943
29. Smith CG, Moser T, Mouliere F, Field-Rayner J, Eldridge M, Riediger AL, et al. Comprehensive characterization of cell-free tumor DNA in plasma and urine of patients with renal tumors. Genome Med (2020) 12(1):23. doi: 10.1186/s13073-020-00723-8
30. Yamamoto Y, Uemura M, Fujita M, Maejima K, Koh Y, Matsushita M, et al. Clinical significance of the mutational landscape and fragmentation of circulating tumor DNA in renal cell carcinoma. Cancer Sci (2019) 110(2):617–28. doi: 10.1111/cas.13906
31. Bacon JVW, Annala M, Soleimani M, Lavoie J-M, So A, Gleave ME, et al. Plasma Circulating Tumor DNA and Clonal Hematopoiesis in Metastatic Renal Cell Carcinoma. Clin Genitourinary Cancer (2020) 18(4):322–331.e2. doi: 10.1016/j.clgc.2019.12.018
32. Hu Z-Y, Xie N, Tian C, Yang X, Liu L, Li J, et al. Identifying Circulating Tumor DNA Mutation Profiles in Metastatic Breast Cancer Patients with Multiline Resistance. EBioMedicine (2018) 32:111–8. doi: 10.1016/j.ebiom.2018.05.015
33. Reckamp KL, Melnikova VO, Karlovich C, Sequist LV, Camidge DR, Wakelee H, et al. A Highly Sensitive and Quantitative Test Platform for Detection of NSCLC EGFR Mutations in Urine and Plasma. J Thorac Oncol (2016) 11(10):1690–700. doi: 10.1016/j.jtho.2016.05.035
34. Turajlic S, Xu H, Litchfield K, Rowan A, Horswell S, Chambers T, et al. Deterministic Evolutionary Trajectories Influence Primary Tumor Growth: TRACERx Renal. Cell (2018) 173(3):595–610.e11. doi: 10.1016/j.cell.2018.03.043
35. Won Y, Song H-J, Kang TW, Kim J-J, Han B-D, Lee S. Pattern analysis of serum proteome distinguishes renal cell carcinoma from other urologic diseases and healthy persons. Proteomics (2003) 3(12):2310–6. doi: 10.1002/pmic.200300590
36. Han WK, Alinani A, Wu C-L, Michaelson D, Loda M, McGovern FJ, et al. Human Kidney Injury Molecule-1 Is a Tissue and Urinary Tumor Marker of Renal Cell Carcinoma. JASN (2005) 16(4):1126–34. doi: 10.1681/ASN.2004070530
37. Hara T, Honda K, Ono M, Naito K, Hirohashi S, Yamada T. Identification of 2 serum biomarkers of renal cell carcinoma by surface enhanced laser desorption/ionization mass spectrometry. J Urol (2005) 174(4 Part 1):1213–7. doi: 10.1097/01.ju.0000173915.83164.87
38. Togashi A, Katagiri T, Ashida S, Fujioka T, Maruyama O, Wakumoto Y, et al. Hypoxia-Inducible Protein 2 (HIG2), a Novel Diagnostic Marker for Renal Cell Carcinoma and Potential Target for Molecular Therapy. Cancer Res (2005) 65(11):4817–26. doi: 10.1158/0008-5472.CAN-05-0120
39. Xu G, Hou C-R, Jiang H-W, Xiang C-Q, Shi N, Yuan H-C, et al. Serum protein profiling to identify biomarkers for small renal cell carcinoma. Indian J Biochem Biophys (2010) 47(4):211–8.
40. Yang J, Yang J, Gao Y, Zhao L, Liu L, Qin Y, et al. Identification of Potential Serum Proteomic Biomarkers for Clear Cell Renal Cell Carcinoma. PloS One (2014) 9(11):e111364 doi: 10.1371/journal.pone.0111364
41. Toiyama D, Takaha N, Shinnoh M, Ueda T, Kimura Y, Nakamura T, et al. Significance of serum tumor necrosis factor-related apoptosis-inducing ligand as a prognostic biomarker for renal cell carcinoma. Mol Clin Oncol (2013) 1(1):69–74. doi: 10.3892/mco.2012.35
42. White NMA, Masui O, DeSouza LV, Krakovska-Yutz O, Metias S, Romaschin AD, et al. Quantitative proteomic analysis reveals potential diagnostic markers and pathways involved in pathogenesis of renal cell carcinoma. Oncotarget (2014) 5(2):506–18. doi: 10.18632/oncotarget.1529
43. Ruf M, Mittmann C, Nowicka AM, Hartmann A, Hermanns T, Poyet C, et al. pVHL/HIF-Regulated CD70 Expression Is Associated with Infiltration of CD27+ Lymphocytes and Increased Serum Levels of Soluble CD27 in Clear Cell Renal Cell Carcinoma. Clin Cancer Res (2015) 21(4):889–98. doi: 10.1158/1078-0432.CCR-14-1425
44. Zhang Y, Cai Y, Yu H, Li H. iTRAQ-Based Quantitative Proteomic Analysis Identified HSC71 as a Novel Serum Biomarker for Renal Cell Carcinoma. Bio Med Res Int (2015) 2015:210–9. doi: 10.1155/2015/802153
45. Lucarini L, Magnelli L, Schiavone N, Crisci A, Innocenti A, Puccetti L, et al. Plasmatic carbonic anhydrase IX as a diagnostic marker for clear cell renal cell carcinoma. J Enzyme Inhib Med Chem (2017) 33(1):234–40. doi: 10.1080/14756366.2017.1411350
46. Knott ME, Manzi M, Zabalegui N, Salazar MO, Puricelli LI, Monge ME. Metabolic Footprinting of a Clear Cell Renal Cell Carcinoma in Vitro Model for Human Kidney Cancer Detection. J Proteome Res (2018) 17(11):3877–88. doi: 10.1021/acs.jproteome.8b00538
47. Kushlinskii NE, Gershtein ES, Naberezhnov DS, Taipov MA, Bezhanova SD, Pushkar’ D, et al. Kidney Injury Molecule-1 (KIM-1) in Blood Plasma of Patients with Clear-Cell Carcinoma. Bull Exp Biol Med (2019) 167(3):388–92. doi: 10.1007/s10517-019-04533-w
48. Tschirdewahn S, Panic A, Püllen L, Harke NN, Hadaschik B, Riesz P, et al. Circulating and tissue IMP3 levels are correlated with poor survival in renal cell carcinoma. Int J Cancer (2019) 145(2):531–9. doi: 10.1002/ijc.32124
49. Scelo G, Muller DC, Riboli E, Johansson M, Cross AJ, Vineis P, et al. KIM-1 as a blood-based marker for early detection of kidney cancer: a prospective nested case-control study. Clin Cancer Res (2018) 24(22):5594–601. doi: 10.1158/1078-0432.CCR-18-1496
50. Wang Q, Zhang J, Tu H, Liang D, Chang DW, Ye Y, et al. Soluble immune checkpoint-related proteins as predictors of tumor recurrence, survival, and T cell phenotypes in clear cell renal cell carcinoma patients. J Immunother Cancer (2019) 7:9. doi: 10.1186/s40425-019-0810-y
51. Zhang M, Liu X, Liu X, Li H, Sun W, Zhang Y. A pilot investigation of a urinary metabolic biomarker discovery in renal cell carcinoma. Int Urol Nephrol (2020) 52(3):437–46. doi: 10.1007/s11255-019-02332-w
52. Clark DJ, Zhang H. Proteomic approaches for characterizing renal cell carcinoma. Clin Proteom (2020) 17(1):28. doi: 10.1186/s12014-020-09291-w
53. Song J, Yu J, Prayogo GW, Cao W, Wu Y, Jia Z, et al. Understanding kidney injury molecule 1: a novel immune factor in kidney pathophysiology. Am J Transl Res (2019) 11(3):1219–29.
54. Nixon AB, Schalper KA, Jacobs I, Potluri S, Wang I-M, Fleener C. Peripheral immune-based biomarkers in cancer immunotherapy: can we realize their predictive potential? J Immuno Ther Cancer (2019) 7(1):325. doi: 10.1186/s40425-019-0799-2
55. Arroyo JD, Chevillet JR, Kroh EM, Ruf IK, Pritchard CC, Gibson DF, et al. Argonaute2 complexes carry a population of circulating microRNAs independent of vesicles in human plasma. Proc Natl Acad Sci USA (2011) 108(12):5003–8. doi: 10.1073/pnas.1019055108
56. Vickers KC, Palmisano BT, Shoucri BM, Shamburek RD, Remaley AT. MicroRNAs are transported in plasma and delivered to recipient cells by high-density lipoproteins. Nat Cell Biol (2011) 13(4):423–33. doi: 10.1038/ncb2210
57. Wang K, Zhang S, Weber J, Baxter D, Galas DJ. Export of microRNAs and microRNA-protective protein by mammalian cells. Nucleic Acids Res (2010) 38(20):7248–59. doi: 10.1093/nar/gkq601
58. Zhao A, Li G, Péoc’h M, Genin C, Gigante M. Serum miR-210 as a novel biomarker for molecular diagnosis of clear cell renal cell carcinoma. Exp Mol Pathol (2013) 94(1):115–20. doi: 10.1016/j.yexmp.2012.10.005
59. Teixeira AL, Ferreira M, Silva J, Gomes M, Dias F, Santos JI, et al. Higher circulating expression levels of miR-221 associated with poor overall survival in renal cell carcinoma patients. Tumor Biol (2014) 35(5):4057–66. doi: 10.1007/s13277-013-1531-3
60. Wu Y, Wang Y-Q, Weng W-W, Zhang Q-Y, Yang X-Q, Gan H-L, et al. A serum-circulating long noncoding RNA signature can discriminate between patients with clear cell renal cell carcinoma and healthy controls. Oncogenesis (2016) 5(2):e192. doi: 10.1038/oncsis.2015.48
61. Li G, Zhao A, Péoch M, Cottier M, Mottet N. Detection of urinary cell-free miR-210 as a potential tool of liquid biopsy for clear cell renal cell carcinoma. Urol Oncol: Semin Orig Invest (2017) 35(5):294–9. doi: 10.1016/j.urolonc.2016.12.007
62. Petrozza V, Pastore AL, Palleschi G, Tito C, Porta N, Ricci S, et al. Secreted miR-210-3p as non-invasive biomarker in clear cell renal cell carcinoma. Oncotarget (2017) 8(41):69551–8. doi: 10.18632/oncotarget.18449
63. Heinemann FG, Tolkach Y, Deng M, Schmidt D, Perner S, Kristiansen G, et al. Serum miR-122-5p and miR-206 expression: non-invasive prognostic biomarkers for renal cell carcinoma. Clin Epigenet (2018) 10:11. doi: 10.1186/s13148-018-0444-9
64. Liu S, Deng X, Zhang J. Identification of dysregulated serum miR-508-3p and miR-885-5p as potential diagnostic biomarkers of clear cell renal carcinoma. Mol Med Rep (2019) 20(6):5075–83. doi: 10.3892/mmr.2019.10762
65. Simonovic S, Hinze C, Schmidt-Ott KM, Busch J, Jung M, Jung K, et al. Limited utility of qPCR-based detection of tumor-specific circulating mRNAs in whole blood from clear cell renal cell carcinoma patients. BMC Urol (2020) 20:7. doi: 10.1186/s12894-019-0542-9
66. Raimondo F, Morosi L, Corbetta S, Chinello C, Brambilla P, Mina PD, et al. Differential protein profiling of renal cell carcinoma urinary exosomes. Mol Biosyst (2013) 9(6):1220–33. doi: 10.1039/c3mb25582d
67. Butz H, Nofech-Mozes R, Ding Q, Khella HWZ, Szabó PM, Jewett M, et al. Exosomal MicroRNAs Are Diagnostic Biomarkers and Can Mediate Cell–Cell Communication in Renal Cell Carcinoma. Eur Urol Focus (2016) 2(2):210–8. doi: 10.1016/j.euf.2015.11.006
68. Qu L, Ding J, Chen C, Wu Z-J, Liu B, Gao Y, et al. Exosome-Transmitted lncARSR Promotes Sunitinib Resistance in Renal Cancer by Acting as a Competing Endogenous RNA. Cancer Cell (2016) 29(5):653–68. doi: 10.1016/j.ccell.2016.03.004
69. Du M, Giridhar KV, Tian Y, Tschannen MR, Zhu J, Huang C-C, et al. Plasma exosomal miRNAs-based prognosis in metastatic kidney cancer. Oncotarget (2017) 8(38):63703–14. doi: 10.18632/oncotarget.19476
70. Jingushi K, Uemura M, Ohnishi N, Nakata W, Fujita K, Naito T, et al. Extracellular vesicles isolated from human renal cell carcinoma tissues disrupt vascular endothelial cell morphology via azurocidin. Int J Cancer (2018) 142(3):607–17. doi: 10.1002/ijc.31080
71. Zhang W, Ni M, Su Y, Wang H, Zhu S, Zhao A, et al. MicroRNAs in Serum Exosomes as Potential Biomarkers in Clear-cell Renal Cell Carcinoma. Eur Urol Focus (2018) 4(3):412–9. doi: 10.1016/j.euf.2016.09.007
72. White NMA, Khella HWZ, Grigull J, Adzovic S, Youssef YM, Honey RJ, et al. miRNA profiling in metastatic renal cell carcinoma reveals a tumour-suppressor effect for miR-215. Br J Cancer (2011) 105(11):1741–9. doi: 10.1038/bjc.2011.401
73. Hsiao K-Y, Sun HS, Tsai S-J. Circular RNA - New member of noncoding RNA with novel functions. Exp Biol Med (Maywood) (2017) 242(11):1136–41. doi: 10.1177/1535370217708978
74. Miranda KC, Bond DT, Levin JZ, Adiconis X, Sivachenko A, Russ C, et al. Massively parallel sequencing of human urinary exosome/microvesicle RNA reveals a predominance of non-coding RNA. PloS One (2014) 9(5):e96094. doi: 10.1371/journal.pone.0096094
75. Alimirzaie S, Bagherzadeh M, Akbari MR. Liquid biopsy in breast cancer: A comprehensive review. Clin Genet (2019) 95(6):643–60. doi: 10.1111/cge.13514
76. Norcic G. Liquid Biopsy in Colorectal Cancer-Current Status and Potential Clinical Applications. Micromachines (Basel) (2018) 9(6):300. doi: 10.3390/mi9060300
77. Santarpia M, Liguori A, D’Aveni A, Karachaliou N, Gonzalez-Cao M, Daffinà MG, et al. Liquid biopsy for lung cancer early detection. J Thorac Dis (2018) 10(Suppl 7):S882–97. doi: 10.21037/jtd.2018.03.81
78. Merker JD, Oxnard GR, Compton C, Diehn M, Hurley P, Lazar AJ, et al. Circulating Tumor DNA Analysis in Patients With Cancer: American Society of Clinical Oncology and College of American Pathologists Joint Review. JCO (2018) 36(16):1631–41. doi: 10.1200/JCO.2017.76.8671
79. Lasseter K, Nassar AH, Hamieh L, Berchuck JE, Nuzzo PV, Korthauer K, et al. Plasma cell-free DNA variant analysis compared with methylated DNA analysis in renal cell carcinoma. Genet Med (2020) 22:1366–73. doi: 10.1038/s41436-020-0801-x
80. Zviran A, Schulman RC, Shah M, Hill STK, Deochand S, Khamnei CC, et al. Genome-wide cell-free DNA mutational integration enables ultra-sensitive cancer monitoring. Nat Med (2020) 26:1114–24. doi: 10.1038/s41591-020-0915-3
81. Nuzzo PV, Berchuck JE, Spisak S, Korthauer K, Nassar A, Abou Alaiwi S, et al. Sensitive detection of renal cell carcinoma using plasma and urine cell-free DNA methylomes. JCO (2020) 38(6_suppl):728–8. doi: 10.1200/JCO.2020.38.6_suppl.728
82. Sadeh R, Fialkoff G, Sharkia I, Rahat A, Nitzan M, Fox-Fisher I, et al. ChIP-seq of plasma cell-free nucleosomes identifies cell-of-origin gene expression programs. Mol Biol (2019) doi: 10.1101/638643
83. Snyder MW, Kircher M, Hill AJ, Daza RM, Shendure J. Cell-free DNA comprises an in vivo nucleosome footprint that informs its tissues-of-origin. Cell (2016) 164(0):57–68. doi: 10.1016/j.cell.2015.11.050
84. Bache N, Geyer PE, Bekker-Jensen DB, Hoerning O, Falkenby L, Treit PV, et al. A Novel LC System Embeds Analytes in Pre-formed Gradients for Rapid, Ultra-robust Proteomics. Mol Cell Proteom (2018) 17(11):2284–96. doi: 10.1074/mcp.TIR118.000853
85. Bruderer R, Muntel J, Müller S, Bernhardt OM, Gandhi T, Cominetti O, et al. Analysis of 1508 Plasma Samples by Capillary-Flow Data-Independent Acquisition Profiles Proteomics of Weight Loss and Maintenance. Mol Cell Proteom (2019) 18(6):1242–54. doi: 10.1074/mcp.RA118.001288
86. Chinello C, Stella M, Piga I, Smith AJ, Bovo G, Varallo M, et al. Proteomics of liquid biopsies: Depicting RCC infiltration into the renal vein by MS analysis of urine and plasma. J Proteom (2019) 191:29–37. doi: 10.1016/j.jprot.2018.04.029
87. Franz A, Ralla B, Weickmann S, Jung M, Rochow H, Stephan C, et al. Circular RNAs in Clear Cell Renal Cell Carcinoma: Their Microarray-Based Identification, Analytical Validation, and Potential Use in a Clinico-Genomic Model to Improve Prognostic Accuracy. Cancers (Basel) (2019) 11(10):1473. doi: 10.3390/cancers11101473
88. Wang K, Sun Y, Tao W, Fei X, Chang C. Androgen receptor (AR) promotes clear cell renal cell carcinoma (ccRCC) migration and invasion via altering the circHIAT1/miR-195-5p/29a-3p/29c-3p/CDC42 signals. Cancer Lett (2017) 394:1–12. doi: 10.1016/j.canlet.2017.03.039
89. Ma H, Xu Y, Zhang R, Guo B, Zhang S, Zhang X. Differential expression study of circular RNAs in exosomes from serum and urine in patients with idiopathic membranous nephropathy. Arch Med Sci (2019) 15(3):738–53. doi: 10.5114/aoms.2019.84690
90. Stranska R, Gysbrechts L, Wouters J, Vermeersch P, Bloch K, Dierickx D, et al. Comparison of membrane affinity-based method with size-exclusion chromatography for isolation of exosome-like vesicles from human plasma. J Transl Med (2018) 16:1–9. doi: 10.1186/s12967-017-1374-6
91. Iliuk A, Wu X, Li L, Sun J, Hadisurya M, Boris RS, et al. Plasma-derived EV Phosphoproteomics through Chemical Affinity Purification. J Proteome Res (2020) 19(7):2563–74. doi: 10.1021/acs.jproteome.0c00151
Keywords: liquid biopsy, prognostic markers, renal cell carcinoma (RCC), translational research, tumor biomarkers
Citation: Lakshminarayanan H, Rutishauser D, Schraml P, Moch H and Bolck HA (2020) Liquid Biopsies in Renal Cell Carcinoma—Recent Advances and Promising New Technologies for the Early Detection of Metastatic Disease. Front. Oncol. 10:582843. doi: 10.3389/fonc.2020.582843
Received: 13 July 2020; Accepted: 29 September 2020;
Published: 28 October 2020.
Edited by:
Francesco Fabbri, Romagnolo Scientific Institute for the Study and Treatment of Tumors (IRCCS), ItalyReviewed by:
Alfredo Berruti, University of Brescia, ItalyLasse Dahl Ejby Jensen, Linköping University, Sweden
Copyright © 2020 Lakshminarayanan, Rutishauser, Schraml, Moch and Bolck. This is an open-access article distributed under the terms of the Creative Commons Attribution License (CC BY). The use, distribution or reproduction in other forums is permitted, provided the original author(s) and the copyright owner(s) are credited and that the original publication in this journal is cited, in accordance with accepted academic practice. No use, distribution or reproduction is permitted which does not comply with these terms.
*Correspondence: Hella A. Bolck, SGVsbGEuQm9sY2tAdXN6LmNo; Holger Moch, SG9sZ2VyLk1vY2hAdXN6LmNo