- 1Department of Neurosurgery, Huashan Hospital, Shanghai Medical College, Fudan University, Shanghai, China
- 2Department of Neurosurgery, Medizinische Fakultät Carl Gustav Carus, Technische Universität Dresden, Dresden, Germany
- 3Department of Neurosurgery, Harvard Medical School, Massachusetts General Hospital, Boston, MA, United States
- 4Department of Critical Care Medicine, Huashan Hospital, Shanghai Medical College, Fudan University, Shanghai, China
Background: Studies have shown mitochondrial genome content (mtDNA content) varies in many malignancies. However, its distribution and prognostic values in high-grade meningioma remain largely unknown. In this retrospective study, we sought to assess a putative correlation between the mtDNA content and clinical characteristics.
Methods: Mitochondrial DNA was extracted from 87 World Health Organization grade III meningioma samples using a qPCR method. The distribution of mtDNA content in WHO grade III meningioma and its correlations with clinical variables were assessed. Furthermore, we prognostic values were also determined.
Results: Mean mtDNA content was 617.7 (range, 0.8–3000). There was no mtDNA distribution difference based on the histological subtypes (P = 0.07). Tumors with preoperative radiation were associated with lower mtDNA content (P = 0.041), whereas no correlations with other clinical variables were observed. A high mtDNA content was associated with significantly better PFS (P = 0.044) and OS (P = 0.019). However, in patients who received postoperative radiotherapy, low mtDNA content was associated with better PFS (P = 0.028), while no difference in OS was observed (P = 0.272). Low mtDNA content was also associated with better OS and PFS in subgroups of patients with ER negative status (PFS, P = 0.002; OS, P = 0.002).
Conclusions: Consistent with other tumors, high mtDNA content was associated with better outcome in WHO grade III meningioma in our cohort. However, for patients who received post-operative radiation therapy, low mtDNA content was associated with better PFS. These findings suggest that mtDNA content may further be explored as a potential biomarker for high-grade meningioma patients and for those who received postoperative radiation therapy.
Introduction
Tumors of the meninges constitute approximately a third of all primary intracranial neoplasms (1). Meningiomas are thought to arise from the arachnoid cap cells of the intracranial and spinal regions. According to the newest WHO 2016 Central Nervous System (CNS) tumor grading criterion, meningiomas are classified to three WHO grades and fifteen histological subtypes (2). Although the majority of meningiomas are benign and slowly growing tumors, there exist a subset of tumors manifesting aggressive and malignant biological behaviors, usually accompanied with higher tumor grade. The histological grading is strongly associated with tumor recurrence and clinical outcome, with five-year survival rates ranging only 28–61% from reported studies. Although grade III meningioma constitutes only a small proportion (1–2%) of all meningiomas, the malignancy and the clinical outcome remains dismal, with a median overall survival ranging from 2.6 to 5.8 years, compared with the other two lower grade meningioma (3–8). Grade III meningioma is defined by a mitotic index equal or higher than 20 mitoses per 10 high power fields and is further classified to three different subtypes based on the histological features, namely anaplastic, papillary, and rhabdoid (2). The anaplastic subtype is the most common in grade III meningioma, representing about 80% of all grade III meningiomas, while the papillary and rhabdoid constitute about 10%, respectively (9).
The mainstream treatment for grade III meningioma involves radical surgical resection followed by stereotactic radiotherapy or radiotherapy (10). Unfortunately, a considerable proportion of grade III meningioma patients still suffer from recurrence despite in time radiation therapy applied (11, 12). Great efforts have been made to investigate potential medical treatment and identify the predictors for radiation sensitivity. To the best of our knowledge, very few have been identified and none of them are applied for clinical use.
Although genetic and cellar studies have identified a number of genes, proteins, and molecules implicated in the tumor genesis, progression and malignant transformation of meningioma during the last several decades, most molecules studied are concerned with nuclear DNA alternations (13). The role of mitochondria in meningioma has not been well deciphered. Mitochondria contain their own genome, encode their own transitional machinery and 13 critical proteins for the oxidative phosphorylation system, which plays a vital role in reactive oxygen production, redox signaling, apoptosis, and many other biological processes (14). Each mitochondrion possesses multiple copies of a mitochondria genome. The functional component of mitochondrial DNA in a cell depends mainly on the content of mitochondrial genomes and the integrity of each mtDNA molecule. Broad ranges of mtDNA content in cells have been reported, from a few in embryonic to several thousands in cardiac myocytes (15). Studies have demonstrated that alterations of mtDNA content are involved in the development and progression of cancer, and the mtDNA content is often changed in tumors compared to non-neoplastic tissues (16–24). Although the content of mtDNA varies in a variety of tumors, it has never been reported in meningioma before, let alone in grade III meningioma. There is only one study investigating the mutational status of mtDNA and they concluded that mtDNA instabilities is relate to tumorogenesis of meningioma (25).
In this retrospective study, we investigated mtDNA content in a cohort of grade III meningioma patients and its association with clinical characteristics, treatment status and prognosis.
Patients and Methods
Studies and Patients
Samples from eighty-seven meningioma patients (44 males and 43 females) with WHO grade III meningioma who underwent surgical resection between 2003 and 2008 at the Neurosurgical Department of Huashan Hospital, Fudan University, were included in the current analysis (Table 1). Based on their histology, the meningiomas were assigned to one of three WHO groups: 63 anaplastic, 12 papillary, and 12 rhabdoid meningiomas. Among those, 59 (67.8%) were primary tumors and 28 (32.2%) were recurrent. The histopathological results were independently re-evaluated and confirmed by two experienced neuro-pathologists (Dr. Yin Wang and Dr. Hong Chen) according to the WHO 2016 meningioma grading criterion. All participants gave their written informed consent and ethical approval for the study was obtained from the Human Subjects Institutional Review Board at Huashan Hospital, Fudan University (KY-2012-17).
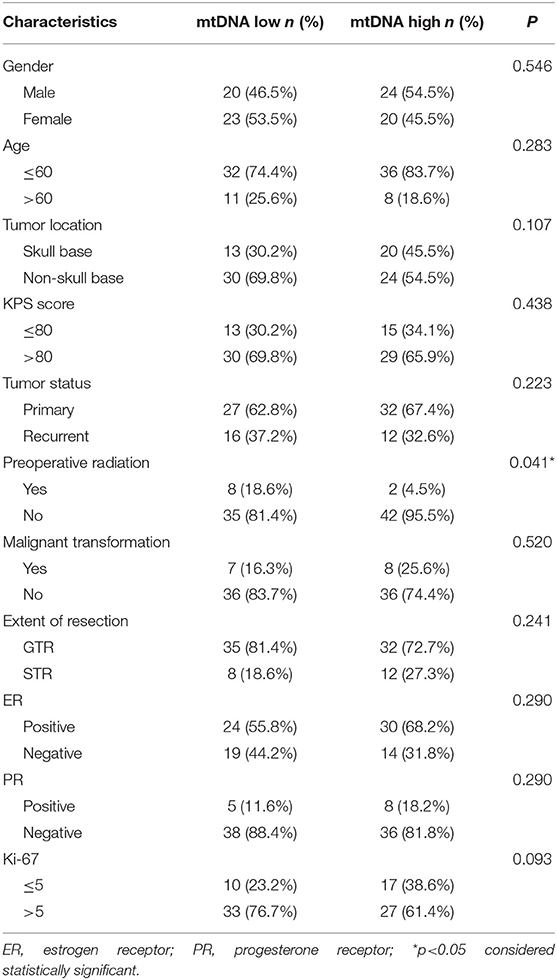
Table 1. Association between mtDNA content and clinicalpathological variables of Grade III meningioma patients.
DNA Extraction
All paraffin-embedded meningioma tissues used for DNA extraction were reviewed by Dr. Yin Wang to ensure that at least 50% of the cells were neoplastic. DNA was extracted using the GenElute™ FFPE DNA Purification Kit (Sigma-Aldrich, Kenilworth, USA) according to the manufacturer's instructions and reported other authors (26). Briefly, the tissues were treated with xylene first to remove paraffin, and then were subjected to digestion and DNA subsequently isolated from the digested tissues. The concentration and purity of the DNA were assessed using a Nanodrop 2000 spectrophotometer (Thermo Scientific, Wilmington, DE, USA) and all samples were diluted to a concentration of 0.2 ng/μL DNA and stored at −80°C before mtDNA content analysis.
mtDNA Content
mtDNA content was determined using a quantitative real-time PCR method. This assay measures relative mtDNA content by measuring the ratio of mitochondrial copy number to single copy nuclear gene. The mitochondrially encoded NADH dehydrogenase subunit 1 gene (MT-ND1) and nuclear single copy gene β-globin were used in our study. The specific sequences of primers and TaqMan probes used for the amplification were as follows: MT-ND1 forward (MT-ND1-F), 5′-CCCTAAAACCCGCCACATCT-3′; MT-ND1 reverse (MT-ND1-R), 5′-GAGCGATGGTGAGAGCTAAGGT-3′; β-globin forward (β-globin-1), 5′-GTGCACCTGACTCCTGAGGAGA-3′; β-globin reverse (β-globin−2), 5′-CCTTGATACCAACCTGCCCAG-3′. The TaqMan probes were labeled with 5′-FAM (6-carboxyfluorescein, fluorescent reporter) and 3′-TAMRA (6-carboxy-tetramethylrhodamine, fluorescent quencher). qPCR amplification for each sample was performed using TaqMan™ Universal Master Mix II on the Applied Biosystems (Foster City, CA) Quantstudio 6 real-time PCR system. All samples were run in triplicate for both mitochondrial and nuclear genes on a 384 well plate with a 7500 Fast Real-time PCR system (qRT-PCR; PE7500 real-time PCR instrument; Applied Biosystems, Foster City, CA, USA). Proper positive and negative controls, a calibrator DNA and a standard curve, were also included in each run to monitor the performance of PCR reactions. The thermal conditions for both primers were 95°C for 30 s, followed by 35 cycles of 94°C for 30 s, 58°C for 30 s, and 72°C for 50 s with signal acquisition. The results were analyzed with the 7500v2.0.4 software (Applied Biosystems). The ratio of MT-ND1 copy number to β-globin copy number was calculated for each sample from standard curves. Furthermore, the ratio of each sample was normalized to a calibrator DNA to standardize between different runs, and it was defined as relative mtDNA content.
Immunohistochemistry
Immuno-histochemical staining was carried out by using monoclonal antibodies, including Ki-67, ER and PR (Signal way [SAB], Shanghai, China; 1:200 dilution). Immuno-histological staining of these antigens was evaluated by two experienced neuro-pathologists as descried above. The tumor was considered ER or PR positive if > 10% of the tumor nuclei showed staining; weak positive when 1–9% of the tumor nuclei were stained; and negative for tumors with no nuclei staining (27, 28).
Statistical Analysis
All statistical computations used in this study were performed using Stata 13.3 for Windows. mtDNA content distribution was tested with the Shapiro-Wilk test. Fisher's exact test was used to analyze associations between the clinical variables and mtDNA content. The association between mtDNA content and Ki-67 labeling index was tested using the logistic regression model. Continuous variables with skewed distribution were compared with Mann-Whitney U test. Survival curves were drawn as Kaplan-Meier survival plots and analyzed with log-rank test. Cox proportional hazards regression model was applied for the univariate, multivariate and stratified prognostic analysis. A P value < 0.05 was considered to be statistically significant.
Results
mtDNA Content Distribution and Its Association With Clinical Variables
The mtDNA content was measured using a real-time PCR based method in a cohort of 87 WHO grade III meningiomas. The mean mtDNA quantity was 617.7 (range, 0.8–3000) mtDNA per cell. The mean mtDNA quantity was 955.8 (range, 0.8–3000) in anaplastic meningioma, 988.8 (range, 548.5–2336.8) in papillary subtype, and 612.7 (range, 460.2–1815.2) in rhabdoid subtype. No significant difference in the distribution of mtDNA was observed between the three histological subtypes (p = 0.07, Kruskal-Wallis test). Therefore, we combined these three histological subtypes together for further statistical analysis. Linear regression model was performed to see whether the mtDNA distribution is associated with tumor proliferation marker (Ki-67 labeling index). No correlation between these two parameters was observed (P = 0.056, r = 0.031). Since mtDNA content was not normally distributed (P < 0.0001, Shapiro-Wilk test), we classified the cohort into two groups based on the median of mtDNA content (median, 782.2), resulting in mtDNA low group (43 tumors) and high group (44 tumors).
To evaluate the clinical relevance of mtDNA, we then compared the mean mtDNA with various clinicopathological characteristics. As shown in Table 1, no significant differences were observed between mtDNA and gender, age, tumor location (skullbase vs. convexity), preoperative KPS score, tumor recurrent status, malignant transformation, extent of tumor resection, ER status, PR status, or Ki-67 labeling index (P > 0.05, Fisher's exact test). However, there was a significant difference with regard to preoperative radiation therapy; patients who received radiation therapy before surgery (N = 10) were more prevalent in the mtDNA low group (Figure 1, P = 0.041, Fisher's exact test).
Prognostic Analysis of mtDNA Content in Grade III Meningioma Patients
We next studied the association between mtDNA content and progression free survival (PFS) and overall survival (OS). As shown in Figure 2, patients with high mtDNA content showed a significantly longer PFS (P = 0.044, log-rank test) as well as OS (P = 0.019, log-rank test). The median PFS in mtDNA low and high group were 43.93 months (range, 1–136 months) and 68.14 months (range, 3–160 months, p = 0.049), respectively, while the median OS was 58.19 (range, 1–138 months) and 78.89 (range, 4–160 months, p = 0.023), respectively. Univariate Cox proportional hazards model revealed that patients with high mtDNA content had a significantly better outcome regarding extent of resection (GTR) and negative ER (Table 2). We next performed multiple Cox proportional hazards model by testing a number of prognostic factors including ER status (5, 29). Here, we found that along with ER, mtDNA was also an independent prognostic factor for both PFS (P = 0.002) and OS (P = 0.002) (Table 2).
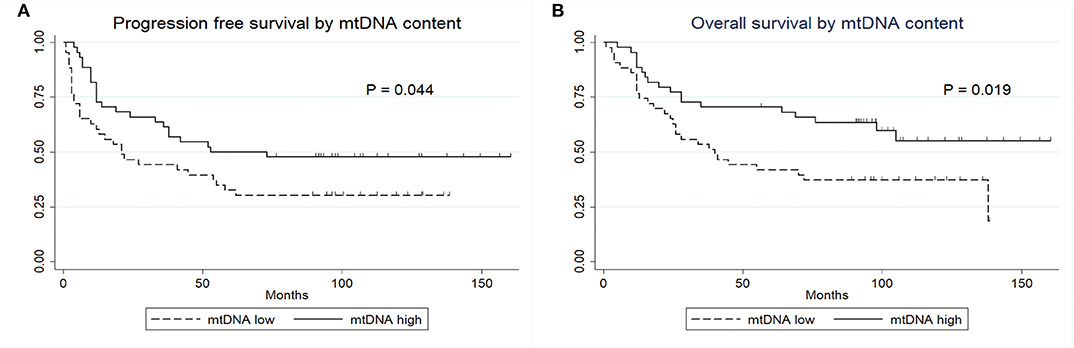
Figure 2. Kaplan-Meier survival curves. (A) PFS of patients by mtDNA content. (B) OS of patients by mtDNA content.
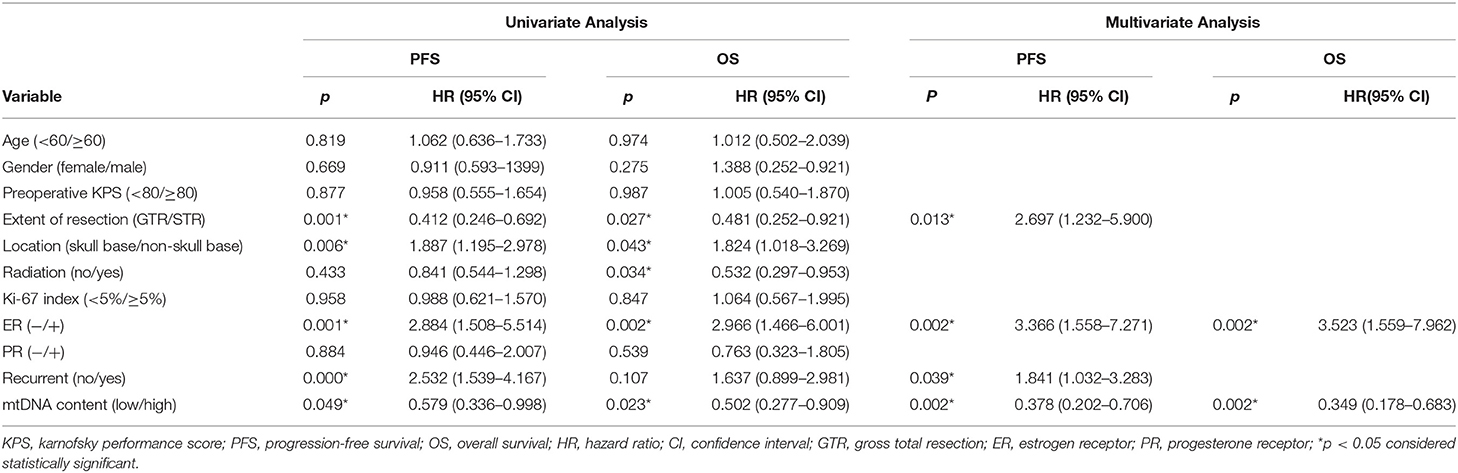
Table 2. Univariate and multivariate analysis of prognostic factors for WHO grade III meningioma patients.
Prognostic Analysis of mtDNA Content in Grade III Meningioma Patients Receiving Postoperative Radiation
Radiation therapy has been frequently associated with mitochondria damage (30). Therefore, we analyzed the prognostic factors in patients who received postoperative radiation therapy. A total of 47 patients in our cohort received either gamma knife or external beam radiation after tumor resection. The tumor mtDNA content in patients who underwent postoperative radiation therapy was not normally distributed (P < 0.0001, Shapiro-Wilk test). In this subgroup, we further classified patients into mtDNA low and high group based on the median mtDNA content in this group, which was 295.7 per cell. Three patients who previously harbored meningiomas with low mtDNA in the initial 87 patients cohort were reclassified as mtDNA high tumors in this 47 patients group.
No statistically significant differences were observed between the clinical variables and the mtDNA content, including preoperative radiation status (P > 0.05, Fisher's exact test, Table 3). Interestingly, the univariate Cox proportional survival analysis revealed that low mtDNA content in the irradiated tumor group was associated with better PFS (P = 0.035) (Table 4), which was further confirmed by Kaplan-Meier analysis (P = 0.028) (Figure 3). However, it was not associated with OS (P = 0.272) (Figure 3). Our multivariate analysis of patients with post-operative radiation did not identify mtDNA content as a significant independent factor for PFS or OS, while ER status was found to be a very significant prognostic factor (Table 4). Stratified analysis revealed that, for patients who received postoperative radiation therapy, low mtDNA content was associated with longer OS and PFS in patients with ER negative status (PFS, P = 0.002; OS, P = 0.002) (Figure 4).
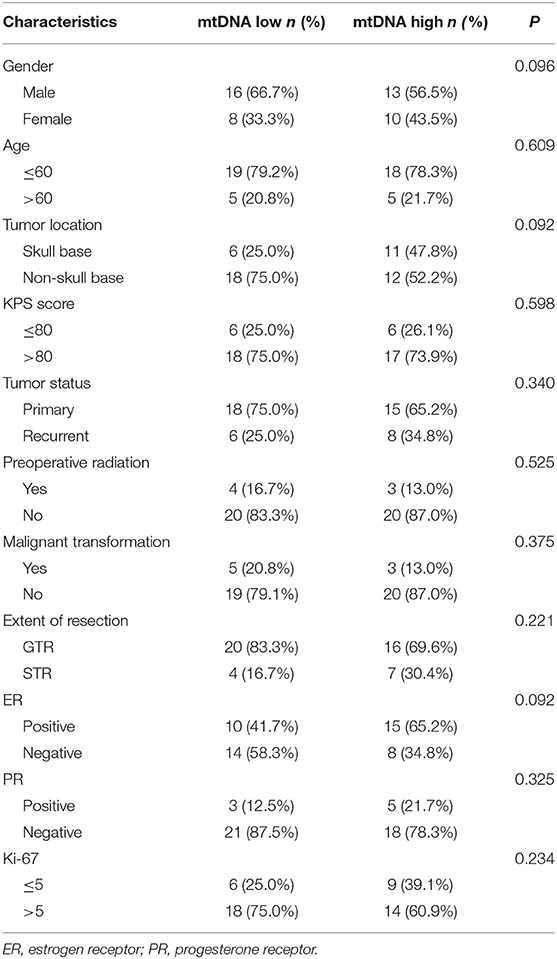
Table 3. Association between mtDNA content and clinical-pathological variables of Grade III meningioma patients who receive post-operative radiation therapy.
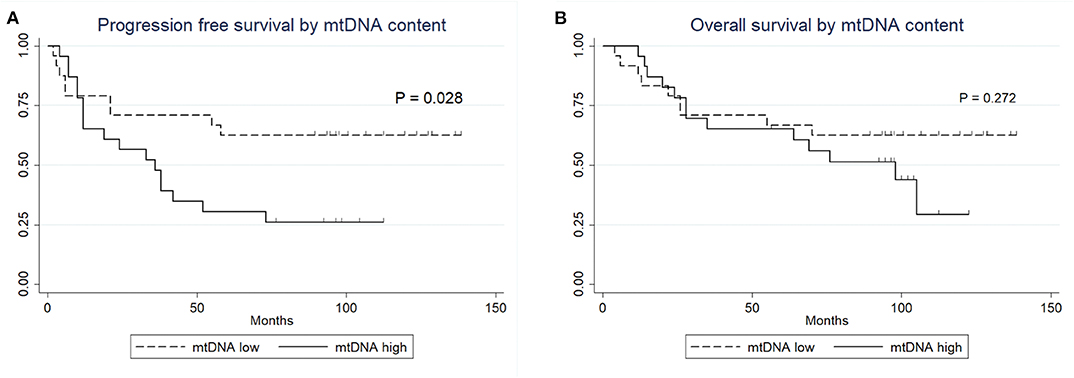
Figure 3. Kaplan-Meier survival curves. (A) PFS of patients with post-operative radiation therapy by mtDNA content. (B) OS of patients with post-operative radiation therapy by mtDNA content.
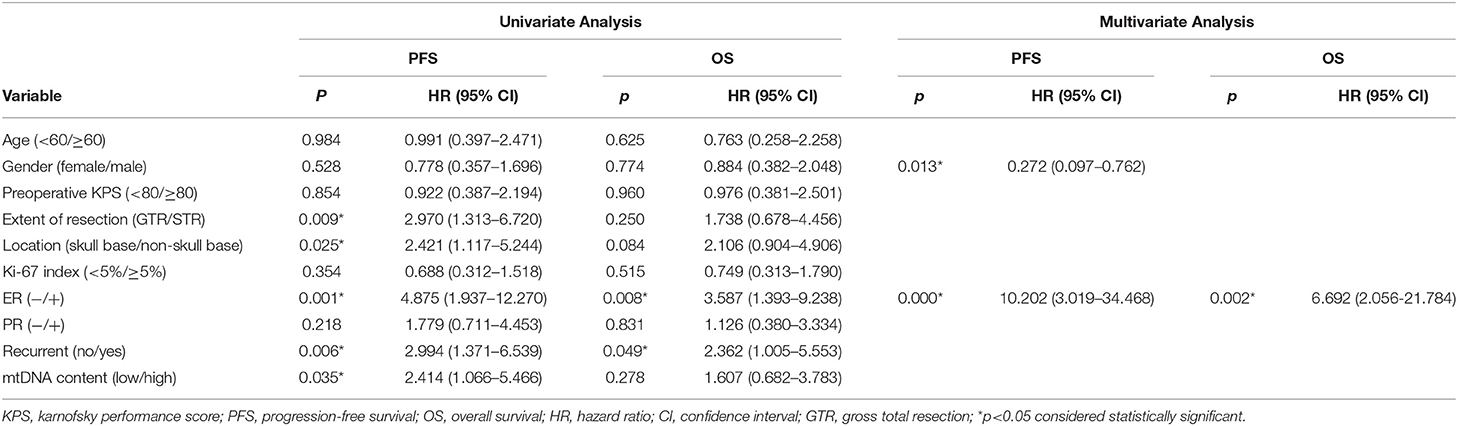
Table 4. Univariate and Multivariate Analysis of Prognostic Factors for WHO grade III meningioma patients receiving post-operative radiation therapy.
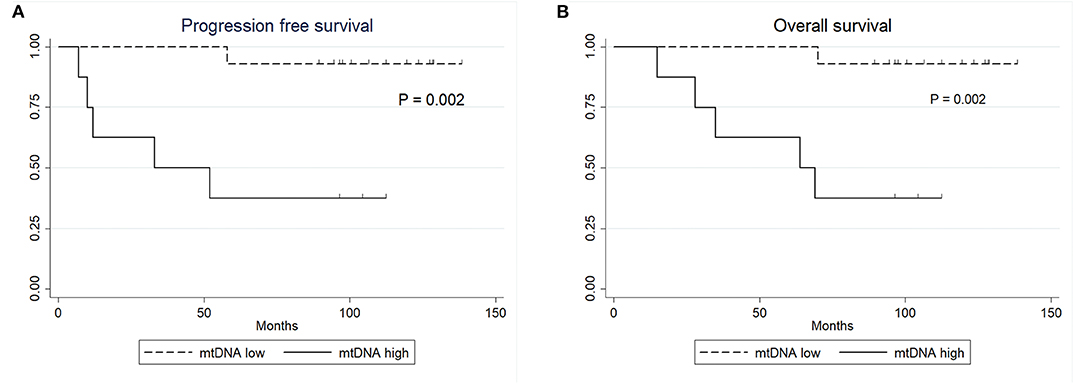
Figure 4. Kaplan-Meier survival curves. (A) PFS of patients with ER negative status by mtDNA content. (B) OS of patients with ER negative status by mtDNA content.
Discussions
WHO grade III meningioma are rare and represent 1–2% of all meningiomas. Initial standard-of-care therapy for malignant meningiomas is surgical resection followed by radiation therapy. Nevertheless, the majority patients with malignant meningiomas devlope recurrent tumors and have a dismal outcome (3, 5). Extensive efforts have been made to explore possible prognostic factors that can predict the outcome in malignant meningiomas (31–36). In addition, we previously reported that ER is an independent negative prognostic factor for WHO grade III meningioma (5). In this study, we explored the distribution of mtDNA content and its prognostic value in a total of 87 patients with WHO grade III meningioma. We demonstrated that patients with high intratumoral mtDNA content had a significantly better outcome regarding both OS and PFS. However, in the subgroup of patients who received postoperative radiation therapy, low mtDNA content was associated with better PFS, although it was not an independent prognostic factor in multivariate analysis.
mtDNA content variation has been reported to play an important role in the development of several malignancies. As early as 1996, Liang et al. demonstrated high mtDNA content is associated with more proliferative abilities (37). Since then, an increasing number of studies focused on mtDNA copy number variation and its correlations with tumors. In our cohort, mtDNA content distribution was not associated with the Ki-67 labeling index. This is in concordance with previous studies that demonstrated no correlation between the Ki-67 labeling index and outcomes in grade III meningioma (5, 38). In tumors, the mtDNA content has been shown to be altered compared to adjacent non-neoplastic tissues, including breast, esophageal and prostate cancers (16–18). In addition, a progressive decrease in mtDNA content has been observed during malignant transformation in glioma, breast, prostate, endometrial and head and neck cancers (17–19, 21, 22, 39). We analyzed distribution of mtDNA content between the three histological subtypes within the WHO grade III meningioma and no difference was observed, which was consistent with our previous study demonstrating no difference in the clinical features between the three distinct histological subtypes (5). We did not separately analyze the mtDNA content by the histological subtype was that there were two few papillary and habdoid meningioma patients in the cohort which would lead to statistical bias. Zhang et al. revealed that in their series of 151 glioma patients, mtDNA content was associated with recurrent status in glioma, which was confirmed by two recent studies (40, 41). In breast cancer, Marjolein reported low mtDNA content was more prevalent in ER positive breast cancers (20). In our cohort, however, we did not find any correlations between mtDNA content and major tumor clinical characteristics including tumor recurrence, malignant transformation, and ER status. Nevertheless, low mtDNA content was more prevalent in patients who received preoperative radiation therapy, which may be explained by the radiation-induced DNA damage in the mitochondrial genome combined by impaired enzyme synthesis.
Although most studies revealed that high mtDNA content was associated with better outcome in cancer, some studies showed contrary data (18, 42–51). Thus, the role of mtDNA in cancer prognosis remains controversial. Consistent with the results reported in breast, prostate, and esophageal cancer and glioma, we found a strong correlation between outcome and mtDNA content in our cohort. High mtDNA content was associated with both better PFS and OS of patients with grade III meningioma. In addition, along with ER status, mtDNA content was an independent prognostic factor for both PFS and OS, suggesting that alterations in mitochondria genome might be associated with progression in high grade meningioma.
Interestingly, our survival analysis demonstrated that in patients who received postoperative radiation (n = 47), low tumor mtDNA content was associated with better PFS, whereas no OS difference was observed. Consistently, studies in breast cancer demonstrated that low tumor mtDNA content is associated with better outcome after receiving chemotherapy due to induction of mitochondrial damage. Likewise, as radiation can induce mitochondrial damage (52), a suitable hypothesis is that tumors with low mtDNA content may be more susceptible to mitochondrial damage induced by radiation than those with high mtDNA content.
A recent work by Soon et al. reported that mitochondrial DNA is mutated in several glioma cells and could subsequently induce mitochondrial dysfunction and higher oxidative stress (53). Mitochondrion dysfunction has been theorized as a crucial player in many tumors by increasing the production of reactive oxygen species (ROS) through the activity of the mitochondrial electron transport chain (54). This altered cellular respiratory system may generate excessive reactive oxygen species, and further lead to a vicious cycle of DNA damages. This hypothesis was also proved in pediatric high-grade glioma in a recent study by Shen et al. (41). In their study, higher mtDNA content was associated with better outcome while reducing mtDNA content induced inhibited tumor growth. Reducing mtDNA content in combination with radiation demonstrated synergistic effect. Their study gave us a hint that in grade III meningioma, treatment strategies using pharmacological inhibition of mtDNA content in combination of radiation could also be effective. Pharmacological agents such as DCA can reduce tumor mtDNA content and inhibit meningioma growth, while reduced mtDNA content may render these tumors more sensitive to postoperative radiotherapy.
The main limitation of our study is the small patient number included, especially in the radiation subgroup, which may limit the statistical power of survival and stratified analyses. Moreover, meningioma patients with lower histological grade were excluded. Consequently, the distribution of mtDNA content in different grades of meningioma cannot be evlautaed.
Conclusions
In conclusion, our study highlights the prognostic association of mtDNA content in WHO grade III meningioma. High mtDNA content was an independent prognostic factor for better PFS and OS. However, for patients who received postoperative radiation therapy, low mtDNA content was associated with better PFS. Tumor mtDNA content may serve as a marker to predict the outcome of patients with WHO grade III meningioma. Further studies are warranted to explore the mtDNA distribution in lower WHO grade tumors and the possible role of the mitochondrial genome in the progression of meningioma and response to radiation therapy.
Data Availability Statement
The raw data supporting the conclusions of this manuscript will be made available by the authors, without undue reservation, to any qualified researcher.
Ethics Statement
The studies involving human participants were reviewed and approved by Huashan Hospital Fudan University. The patients/participants provided their written informed consent to participate in this study.
Author Contributions
LH, HZ, YG, and QX: study concepts. LH: study design and manuscript preparation, quality control of data and algorithms. LH, HZ, JD, and SS: data acquisition. LH, TJ, HW, and QX: data analysis and interpretation. LH, TJ, and HW: statistical analysis. TJ, HW, and QX: manuscript editing. HW, YG, and QX: manuscript review. All authors have read and agreed to the published version of the manuscript.
Funding
This study was supported by grants from the National Key R&D Program of China (2018YFC1312600 and 2018YFC1312604 to YG), National Natural Science Foundation of China (81772674 to YG) and the Science and Technology Commission of Shanghai Municipality (18140900200 to YG).
Conflict of Interest
The authors declare that the research was conducted in the absence of any commercial or financial relationships that could be construed as a potential conflict of interest.
Acknowledgments
We thank Dr. Yin Wang and Dr. Hong Chen for their help in re-confirming the histological grade of patients' samples based on 2016 WHO criterion.
Abbreviations
mtDNA, mitochondrial genome content; GTR, gross total resection; STR, subtotal resection; WHO, World Health Organization; ER, estrogen receptor; PR, progesterone receptor; PFS, progression free survival; OS, overall survival.
References
1. Ostrom QT, Gittleman H, Liao P, Vecchione-Koval T, Wolinsky Y, Kruchko C, et al. CBTRUS Statistical Report: Primary brain and other central nervous system tumors diagnosed in the United States in 2010-2014. Neuro Oncol. (2017) 19(suppl_5):v1–v88. doi: 10.1093/neuonc/nox158
2. Louis DN, Perry A, Reifenberger G, von Deimling A, Figarella-Branger D, Cavenee WK, et al. The 2016 World Health Organization Classification of Tumors of the Central Nervous System: a summary. Acta Neuropathol. (2016) 131:803–20. doi: 10.1007/s00401-016-1545-1
3. Champeaux C, Wilson E, Brandner S, Shieff C, Thorne L. World Health Organization grade III meningiomas. A retrospective study for outcome and prognostic factors assessment. Br J Neurosurg. (2015) 29:693–8. doi: 10.3109/02688697.2015.1054350
4. Balasubramanian SK, Sharma M, Silva D, Karivedu V, Schmitt P, Stevens GH, et al. Longitudinal experience with WHO. Grade III (anaplastic) meningiomas at a single institution. J Neurooncol. (2017) 131:555–563. doi: 10.1007/s11060-016-2321-8
5. Hua L, Zhu H, Li J, Tang H, Kuang D, Wang Y, et al. Prognostic value of estrogen receptor in WHO. Grade III meningioma: a long-term follow-up study from a single institution. J Neurosurg. (2017) 131:1–9. doi: 10.3171/2017.2.jns162566
6. Shan B, Zhang J, Song Y, Xu J. Prognostic factors for patients with World Health Organization grade III meningiomas treated at a single center. Medicine (Baltimore). (2017) 96:e7385. doi: 10.1097/md.0000000000007385
7. Champeaux C, Jecko V. World Health Organization grade III meningiomas. A retrospective study for outcome and prognostic factors assessment. Neurochirurgie. (2016) 62:203–8. doi: 10.1016/j.neuchi.2016.05.001
8. Zhang GJ, Zhang GB, Zhang YS, Li H, Li CB, Zhang LW, et al. World Health Organization Grade III (Nonanaplastic) meningioma: experience in a series of 23 cases. World Neurosurg. (2018). doi: 10.1016/j.wneu.2018.01.149
9. Wang DJ, Xie Q, Gong Y, Mao Y, Wang Y, Cheng HX, et al. Histopathological classification and location of consecutively operated meningiomas at a single institution in China from 2001 to 2010. Chin Med J (Engl). (2013) 126:488–93.
10. Goldbrunner R, Minniti G, Preusser M, Jenkinson MD, Sallabanda K, Houdart E, et al. EANO guidelines for the diagnosis and treatment of meningiomas. Lancet Oncol. (2016) 17:e383–91. doi: 10.1016/S1470-2045(16)30321-7
11. Hwang KL, Hwang WL, Bussiere MR, Shih AH. The role of radiotherapy in the management of high-grade meningiomas. Chin Clin Oncol. (2017) 6(Suppl 1):S5. doi: 10.21037/cco.2017.06.09
12. Orton A, Frandsen J, Jensen R, Shrieve DC, Suneja G. Anaplastic meningioma: an analysis of the National Cancer Database from 2004 to 2012. J N.eurosurg. (2017) 131:1–6. doi: 10.3171/2017.2.jns162282
13. Preusser M, Brastianos PK, Mawrin C. Advances in meningioma genetics: novel therapeutic opportunities. Nat Rev Neurol. (2018) 14:106–15. doi: 10.1038/nrneurol.2017.168
14. Chan DC. Mitochondria: dynamic organelles in disease, aging, and development. Cell. (2006) 125:1241–52. doi: 10.1016/j.cell.2006.06.010
15. Moraes CT. What regulates mitochondrial DNA copy number in animal cells? Trends Genet. (2001) 17:199–205. doi: 10.1016/s0168-9525(01)02238-7
16. Hsu CW, Yin PH, Lee HC, Chi CW, Tseng ML. Mitochondrial DNA content as a potential marker to predict response to anthracycline in breast cancer patients. Breast J. (2010) 16:264–70. doi: 10.1111/j.1524-4741.2010.00908.x
17. Rogounovitch TI, Saenko VA, Shimizu-Yoshida Y, Abrosimov AY, Lushnikov EF, Roumiantsev PO, et al. Large deletions in mitochondrial DNA in radiation-associated human thyroid tumors. Cancer Res. (2002) 62:7031–41.
18. Jiang WW, Masayesva B, Zahurak M, Carvalho AL, Rosenbaum E, Mambo E, et al. Increased mitochondrial DNA content in saliva associated with head and neck cancer. Clin Cancer Res. (2005) 11:2486–91. doi: 10.1158/1078-0432.ccr-04-2147
19. Kim MM, Clinger JD, Masayesva BG, Ha PK, Zahurak ML, Westra WH, et al. Mitochondrial DNA quantity increases with histopathologic grade in premalignant and malignant head and neck lesions. Clin Cancer Res. (2004) 10:8512–5. doi: 10.1158/1078-0432.ccr-04-0734
20. Weerts MJA, Hollestelle A, Sieuwerts AM, Foekens JA, Sleijfer S, Martens MJW. Low tumor mitochondrial DNA. Content is associated with better outcome in breast cancer patients receiving anthracycline-based chemotherapy. Clin Cancer Res. (2017) 23:4735–43. doi: 10.1158/1078-0432.ccr-17-0032
21. Yu M, Wan Y, Zou Q. Decreased copy number of mitochondrial DNA in Ewing's sarcoma. Clin Chim Acta. (2010) 411:679–83. doi: 10.1016/j.cca.2010.01.035
22. Riaz N, Sarungbam J, Tickoo SK, Al-Ahmadie HA, Lee W, Seshan VE, et al. High copy number of mitochondrial DNA (mtDNA) predicts good prognosis in glioma patients. Elife. (2015) 5:1207–16. doi: 10.7554/eLife.10769
23. Mambo E, Chatterjee A, Xing M, Tallini G, Haugen BR, Yeung SC, et al. Tumor-specific changes in mtDNA content in human cancer. Int J Cancer. (2005) 116:920–4. doi: 10.1002/ijc.21110
24. Bai RK, Chang J, Yeh KT, Lou MA, Lu JF, Tan DJ, et al. Mitochondrial DNA content varies with pathological characteristics of breast cancer. J. O.ncol. (2011) 2011:496189. doi: 10.1155/2011/496189
25. Vega A, Salas A, Gamborino E, Sobrido MJ, Macaulay V, Carracedo A. mtDNA mutations in tumors of the central nervous system reflect the neutral evolution of mtDNA in populations. Oncogene. (2004) 23:1314–20. doi: 10.1038/sj.onc.1207214
26. Robbe P, Popitsch N, Knight SJL, Antoniou P, Becq J, He M, et al. Clinical whole-genome sequencing from routine formalin-fixed, paraffin-embedded specimens: pilot study for the 100,000 Genomes Project. Genet Med. (2018) 20:1196–205. doi: 10.1038/gim.2017.241
27. Blankenstein MA, Berns PM, Blaauw G, Mulder E, Thijssen HJ. Search for estrogen receptors in human meningioma tissue sections with a monoclonal antibody against the human estrogen receptor. Cancer Res. (1986) 46 (8 Suppl):4268s−70s.
28. Hua L, Zhu H, Li J, Tang H, Kuang D, Wang Y, et al. Prognostic value of estrogen receptor in WHO. Grade III meningioma: a long-term follow-up study from a single institution. J Neurosurg. (2018) 128:1698–706.
29. Hua L, Zhu H, Xie Q, Gong Y. Is there any relationship between estrogen receptor/progesterone receptor status and recurrence of meningioma? Journal N Eurosurger. (2018) 128:1900–1.
30. Galluzzi L, Yamazaki T, Kroemer G. Linking cellular stress responses to systemic homeostasis. Nat Rev Mol Cell Biol. (2018) 19:731–45. doi: 10.1038/s41580-018-0068-0
31. Juratli TA, Brastianos PK, Cahill PD. TERT alterations in progressive treatment-resistant meningiomas. Neurosurgery. (2018) 65(CN_suppl_1):66–8. doi: 10.1093/neuros/nyy154
32. Juratli TA, McCabe D, Nayyar N, Williams EA, Silverman IM, Tummala SS, et al. DMD genomic deletions characterize a subset of progressive/higher-grade meningiomas with poor outcome. Acta Neuropathol. (2018) 136:779–92. doi: 10.1007/s00401-018-1899-7
33. Juratli TA, Thiede C, Koerner MVA, Tummala SS, Daubner D, Shankar GM, et al. Intratumoral heterogeneity and TERT promoter mutations in progressive/higher-grade meningiomas. Oncotarget. (2017) 8:109228–37. doi: 10.18632/oncotarget.22650
34. Sahm F, Schrimpf D, Stichel D, Jones DTW, Hielscher T, Schefzyk S, et al. von Deimling: DNA methylation-based classification and grading system for meningioma: a multicentre, retrospective analysis. Lancet Oncol. (2017) 18:682–94. doi: 10.1016/S1470-2045(17)30155-9
35. Goutagny S, Nault JC, Mallet M, Henin D, Rossi JZ, Kalamarides M. High incidence of activating TERT promoter mutations in meningiomas undergoing malignant progression. Brain Pathol. (2014) 24:184–9. doi: 10.1111/bpa.12110
36. Peyre M, Gauchotte G, Giry M, Froehlich S, Pallud J, Graillon T, et al. De novo and secondary anaplastic meningiomas: a study of clinical and histomolecular prognostic factors. Neuro Oncol. (2017) 20:1113–21. doi: 10.1093/neuonc/nox231
37. Liang BC, Hays L. Mitochondrial DNA copy number changes in human gliomas. Cancer Lett. (1996) 105:167–73.
38. Hua L, Luan S, Li H, Zhu H, Tang H, Liu H, et al. Angiomatous meningiomas have a very benign outcome despite frequent peritumoral edema at onset. World Neurosurg. (2017) 108:465–73. doi: 10.1016/j.wneu.2017.08.096
39. Fan AX, Radpour R, Haghighi MM, Kohler C, Xia P, Hahn S, et al. Mitochondrial DNA content in paired normal and cancerous breast tissue samples from patients with breast cancer. J Cancer Res Clin Oncol. (2009) 135:983–9. doi: 10.1007/s00432-008-0533-9
40. Dardaud LM, Bris C, Desquiret-Dumas V, Boisselier B, Tabouret E, Mokhtari K, et al. High mitochondrial DNA copy number is associated with longer survival in young patients with glioblastoma. Neuro Oncol. (2019) 135:983–9. doi: 10.1093/neuonc/noz072
41. Shen H, Yu M, Tsoli M, Chang C, Joshi S, Liu J, et al. Targeting reduced mitochondrial DNA quantity as a therapeutic approach in pediatric high-grade gliomas. Neuro Oncol. (2020) 22:139–51. doi: 10.1093/neuonc/noz140
42. Kalsbeek AMF, Chan EKF, Grogan J, Petersen DC, Jaratlerdsiri W, Gupta R, et al. Altered mitochondrial genome content signals worse pathology and prognosis in prostate cancer. Prostate. (2018) 78:25–31. doi: 10.1002/pros.23440
43. Zhang Y, Qu Y, Gao K, Yang Q, Shi B, Hou P, et al. High copy number of mitochondrial DNA (mtDNA) predicts good prognosis in glioma patients. Am J. Cancer Res. (2015) 5:1207–16.
44. Bao D, Ba Y, Zhou F, Zhao J, Yang Q, Ge N, et al. Alterations of telomere length and mtDNA copy number are associated with overall survival in hepatocellular carcinoma patients treated with transarterial chemoembolization. Cancer Chemother Pharmacol. (2016) 78:791–9. doi: 10.1007/s00280-016-3128-1
45. Jiang WW, Rosenbaum E, Mambo E, Zahurak M, Masayesva B, Carvalho AL, et al. Decreased mitochondrial DNA content in posttreatment salivary rinses from head and neck cancer patients. Clin Cancer Res. (2006) 12:1564–9. doi: 10.1158/1078-0432.ccr-05-1471
46. Dang S, Qu Y, Wei J, Shao Y, Yang Q, Ji M, et al. Low copy number of mitochondrial DNA (mtDNA) predicts worse prognosis in early-stage laryngeal cancer patients. Diagn Pathol. (2014) 9:28. doi: 10.1186/1746-1596-9-28
47. Zhang G, Qu Y, Dang S, Yang Q, Shi B, Hou P. Variable copy number of mitochondrial DNA (mtDNA) predicts worse prognosis in advanced gastric cancer patients. Diagn Pathol. (2013) 8:173. doi: 10.1186/1746-1596-8-173
48. Nishioka T, Soemantri A, Ishida T. mtDNA/nDNA ratio in 14484 LHON mitochondrial mutation carriers. J. H.um Genet. (2004) 49:701–5. doi: 10.1007/s10038-004-0209-5
49. Li H, Tian Z, Zhang Y, Yang Q, Shi B, Hou P, et al. Increased copy number of mitochondrial DNA predicts poor prognosis of esophageal squamous cell carcinoma. Oncol Lett. (2018) 15:1014–20. doi: 10.3892/ol.2017.7416
50. Koochekpour S, Marlowe T, Singh KK, Attwood K, Chandra D. Reduced mitochondrial DNA content associates with poor prognosis of prostate cancer in African American men. PLoS ONE. (2013) 8:e74688. doi: 10.1371/journal.pone.0074688
51. Wang Y, He S, Zhu X, Qiao W, Zhang J. High copy number of mitochondrial DNA predicts poor prognosis in patients with advanced stage colon cancer. Prostate. (2016) 31:e382–e388. doi: 10.5301/jbm.5000211
52. Wallace DC. Mitochondrial DNA variation in human radiation and disease. Cell. (2015) 163:33–8. doi: 10.1016/j.cell.2015.08.067
53. Soon BH, Abdul Murad NA, Then SM, Abu Bakar A, Fadzil F, Thanabalan J, et al. Mitochondrial DNA. Mutations in grade II and III glioma cell lines are associated with significant mitochondrial dysfunction and higher oxidative stress. Front Physiol. (2017) 8:231. doi: 10.3389/fphys.2017.00231
Keywords: meningioma, MtDNA content, grade III meningioma, prognosis, radiation, malignant meningioma
Citation: Hua L, Juratli TA, Zhu H, Deng J, Wang D, Sun S, Xie Q, Wakimoto H and Gong Y (2020) High Tumor Mitochondrial DNA Content Correlates With an Improved Patient's Outcome in WHO Grade III Meningioma. Front. Oncol. 10:542294. doi: 10.3389/fonc.2020.542294
Received: 12 March 2020; Accepted: 17 August 2020;
Published: 18 September 2020.
Edited by:
Allen Ho, Stanford University, United StatesCopyright © 2020 Hua, Juratli, Zhu, Deng, Wang, Sun, Xie, Wakimoto and Gong. This is an open-access article distributed under the terms of the Creative Commons Attribution License (CC BY). The use, distribution or reproduction in other forums is permitted, provided the original author(s) and the copyright owner(s) are credited and that the original publication in this journal is cited, in accordance with accepted academic practice. No use, distribution or reproduction is permitted which does not comply with these terms.
*Correspondence: Hiroaki Wakimoto, hwakimoto@mgh.harvard.edu; Ye Gong, drgongye@163.com
†These authors share first authorship