- 1Department of Anesthesiology, The First Hospital of Jilin University, Changchun, China
- 2Department of Radiology, The Second Part of The First Hospital of Jilin University, Changchun, China
- 3Clinical Laboratory, The First Hospital of Jilin University, Changchun, China
As a chemotherapeutic agent, bortezomib (BTZ) is used for the treatment of multiple myeloma with adverse effect of painful peripheral neuropathy. Our current study was to determine the inhibitory effects of blocking microRNA-155 (miR-155) signal on BTZ-induced neuropathic pain and the underlying mechanisms. We employed real time RT-PCR and western blot analysis to examine the miR-155 and expression of pro−inflammatory tumor necrosis factor-α receptor (TNFR1) in the dorsal horn of the spinal cord. Its downstream signals p38-MAPK and JNK and transient receptor potential ankyrin 1 (TRPA1) were also determined. Mechanical pain and cold sensitivity were assessed by behavioral test. In result, inhibition of miR-155 significantly attenuated mechanical allodynia and thermal hyperalgesia in BTZ rats, which was accompanied with decreasing expression of TNFR1, p38-MAPK, JNK, and TRPA1. In contrast, miRNA-155 mimics amplified TNFR1-TRPA1 pathway and augmented mechanical pain and cold sensitivity. In addition, mechanical and thermal hypersensitivity induced by miRNA-155 mimics were attenuated after blocking TNFR1, p38-MAPK, JNK, and TRPA1. Overall, we show the key role of miR-155 in modifying BTZ-induced neuropathic pain through TNFR1-TRPA1 pathway, suggesting that miR-155 is a potential target in preventing neuropathic pain development during intervention of BTZ.
Introduction
Bortezomib (BTZ) is an inhibitor of the proteasome complex and is primarily used to treat multiple myeloma (1, 2). Nonetheless, one of its adverse effects is dose-limiting peripheral neuropathy (3). Because of a poor understanding of the mechanisms leading to BTZ-induced pain, treatment options have been limited. Therefore, it is noteworthy to determine molecular mediators of BTZ-induced neuropathy in order to provide a base for application of drugs and further to make therapeutic strategies with chemotherapeutic in patients with multiple myeloma.
MicroRNAs (miRNAs) are small noncoding endogenous RNA molecules, repressing their target mRNA through complementary binding in the message 3′-UTR (4). They have important effects in processes of multiple physiological responses including cell death and survival, cellular response to stress, stem cell division, and pluripotency (5). MiRNAs also contribute to disease processes such as cancer, cardiovascular disease and neurodegenerative diseases (6–8). As a result of their small size, relative ease of delivery, and sequence specificity in recognizing their targets, miRNAs are reflected as therapeutic targets of drug development (9). Notably, miRNA-155 (miR-155) plays a role in various physiological and pathological processes among various miRNAs (10–13). For instance, MiR-155 is involved in chronic immune activity through T cells by the downregulation of lymphocyte-associated antigens (14). In autoimmune diseases, miR-155 is found in patients' tissues and synovial fibroblasts (12). In multiple sclerosis, miR-155 is upregulated in resident myeloid cells of the nervous systems, blood monocytes and activated microglia (15).
The inflammatory process is involved in neuropathic pain (16, 17). Proinflammatory cytokines (PICs), including interleukin-1β (IL-1β), interleukin-6 (IL-6) and tumor necrosis factor-α (TNF-α), are elevated in the nervous system after nerve injury and/or inflammation, responsible for mechanical and thermal hypersensitization (18). In particular, TNF-α has a role in regulating neuropathic pain (16, 17). In pain models, TNF-α in sensory nerves is upregulated following peripheral nerve injury (19). TNF-α evokes hyperalgesia and allodynia in naive rats (20). Chemotherapeutic drugs paclitaxel or vincristine upregulate TNF-α (20). A blockade of TNF-α with its inhibitor or genetic impairment attenuates mechanical hyperalgesia and allodynia (19). BTZ treatment increases TNF-α in dorsal root ganglion (DRG) and spinal dorsal horn (21, 22) and TNF-α antibody inhibits allodynia by BTZ (23).
Transient receptor potential ankyrin 1 (TRPA1) has a functional role in regulating pain and neurogenic inflammation due to channel activation to various compounds (24–28). TRPA1 is presented in sensory nerves (27) and is involved in mechanical and cold hypersensitivity (29, 30). Further studies indicate that TRPA1 mediates mechanical and cold hypersensitivity by chemotherapeutics (31, 32). A recent study showed that a blockade of TNF-α signal attenuates intracellular p38-MAPK and JNK in the DRG and this alleviates mechanical hyperalgesia and cold hypersensitivity by BTZ via decreasing TRPA1 expression (33).
Nonetheless, it remains unrevealed for the role of miRNA-155 in control of TNF-α signal in neuropathy by BTZ. Accordingly, we examined the role played by miR-155 in modulating neuropathic pain after BTZ therapy. We hypothesized that inhibition of miR-155 alleviates mechanical hyperalgesia and cold hypersensitivity by BTZ. We also hypothesized that inhibition of miRNA-155 attenuates upregulation of TNF-α receptor (TNFR1), intracellular p38-MAPK and JNK signal and TRPA1 in the dorsal horn. We further hypothesized that blocking TNFR1-TRPA1 signal attenuates pain hypersensitivity induced by miRNA-155 mimics.
Materials and Methods
Animals
We performed all animal protocols in accordance with the guidelines of the International Association for the Study of Pain, approved by the Research Committee of our institution. Wistar rats (200–250 g) had free access to food and water and they were housed in individual cages in a temperature-controlled room on a 12/12 h light/dark cycle.
Development of Neuropathic Pain and Intrathecal Administration
On the basis of our previous report (34), “BTZ (0.4 mg/kg body weight; dissolved in saline; Haoran BioTech Co., Shanghai, China) was given intraperitoneally (i.p.) once daily for five consecutive days. Control animals were given with an equivalent volume of vehicle (saline).”
Three days prior to each experiment, sodium pentobarbital (60 mg/kg, i.p.) was used to anesthetize the rats to implant intrathecal catheter for administration of drugs. In brief, one end of polyethylene-10 tubing was inserted intrathecally through an incision in the cisternal membrane and advanced 7–9 cm caudal until the tip of the catheter was positioned at the lumbar spinal level (L5 to L6). The other end of the intrathecal tubing was sutured to the musculature and skin at the incision site and externalized to the back of the rat.
After the end of BTZ administration, the following intrathecal injection was performed each day for three consecutive days: miR-155 inhibitor (sequence: 5′AAU UAC GAU UAG CAC UAU CCC CA-3′; 3 μg, Biomics Biotech, Nantong, China), miR-155 mimics (sequence: 5′-UUA AUG CUA AUC GUG AUA GGG GU-3′; 3 μg, Biomics Biotech, Nantong, China) and their corresponding scramble for negative controls (3 μg, Biomics Biotech, Nantong, China). MiR-155 inhibitor, miR-155 mimics and their respective scramble were dissolved in artificial cerebrospinal fluid (aCSF) before they were used; and their dosages used in this study were based on the published work (35). TNF-α synthesis inhibitor pentoxifylline (PTX, 10 μg), TRPA1 antagonist HC030031 (5 μg), p38-MAPK inhibitor SB203580 (5 μg), and JNK inhibitor SP600125 (5 μg) were also given by individual intrathecal injection each day for three consecutive days. Those drugs were obtained from Sigma Co. (St. Louis, MO, US) and all drugs were dissolved in aCSF before they were used. In each experiment, a Hamilton microsyringe (250 μL) was connected to the intrathecal tubing to make 100 μl of delivery. A schematic diagram of experimental protocols and the schedule giving drugs was shown in Figure 1A.
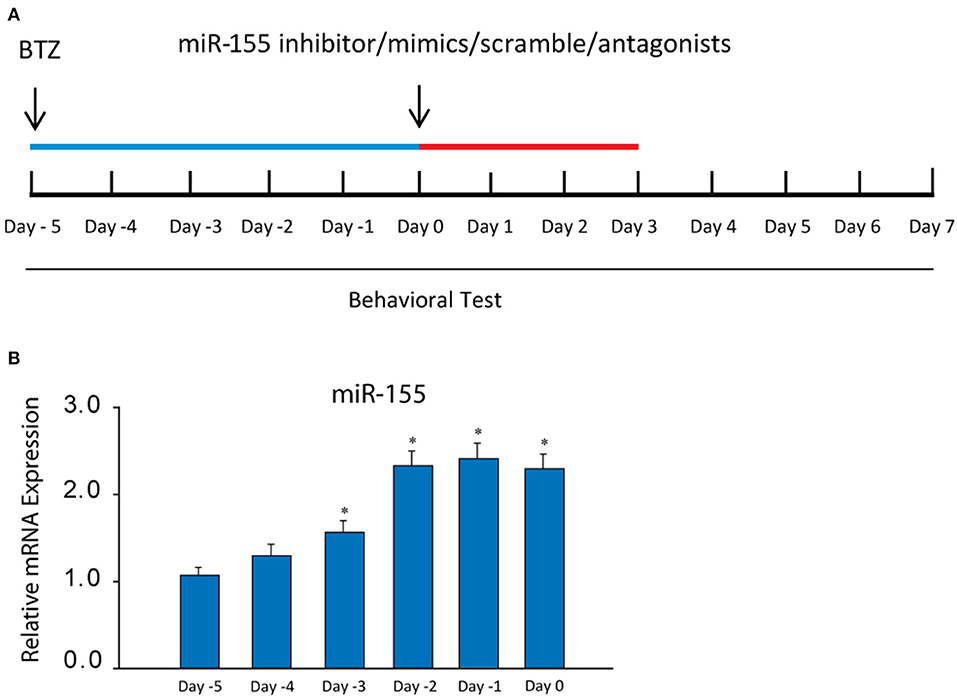
Figure 1. (A) A schematic diagram showing the schedule giving miR-155 inhibitor and mimics, their scrambles and receptor blockers as well as experimental protocols. BTZ was injected [intraperitoneally (i.p.), daily, 0.4 mg/kg body weight] over 5 days (indicated by blue bar). The starting day is expressed as “day −5.” An equivalent volume of vehicle was given in control animals. Then, miR-155 inhibitor/mimics, their scrambles, PTX, HC030031, SB203580, and SP600125 were administered (indicated as red bar) at the end of BTZ injection (day 0), which was also marked as day 0 in the Figures for results examining neuropathic pain. (B) showing that the levels of miR-155 mRNA were increased in the dorsal horn of the spinal cord after administration of BTZ and remained at a high level. *P < 0.05 vs. its level at days −5 and −4. The number of rats = 8–10 in each group.
Real-Time PCR
As described in our previous publication (35), “the tissues of the L5-L6 spinal cord dorsal horn were processed for the extraction of total RNA (RNeasy Mini Kit; Qiagen). RT-PCR was performed using the TaqmanW Universal PCR Master Mix and 18 s rRNA (TaqmanW PDAR) was used as an endogenous control to correct for variations in the samples. RT-PCR was performed in duplicate in 96-well plates containing 2 μL of cDNA. The thermal conditions of the cycles were 50°C/2 min, 60°C/30 min, and 95°C/5 min; and this was followed by 40 cycles at 94°C for 20 s and 62°C for 60 s. The ABI PRISM SDS 7000 thermal cycler was used to obtain the data. Using the 2-ΔΔCt comparative method, relative quantification of target gene was implemented and the threshold cycle value was defined by the point at which there was a statistically significant detectable increase in fluorescence.”
Western Blot Analysis
As described in our previous publication (34), “the samples containing total protein of the L5-L6 dorsal horn tissues were extracted, centrifuged and the supernatants were collected to assess protein concentrations. Then the supernatant (containing 20 μg of protein) were loaded onto gels and electrically transferred to the membrane. The membrane was incubated overnight with primary rabbit antibodies (1:500), namely anti-TNFR1 (Abcam #ab90463), anti-TRPA1 (Novus Bio, NB100-91319), anti-p-p38-MAPK (USBio, USB#403230) and anti-p-JNK1 (Abcam #ab47337). The primary antibodies were purchased from Abcam Co and/or Antibodies-online Inc. After being washed, the membranes were incubated with anti-rabbit secondary antibody (1:1000, Sigma Co). The bands recognized by immunoreactive proteins were visualized by exposure of the membrane onto an x-ray film. The Scion Image software was employed to determine the optical density of immunoreactive proteins bands.”
Behavioral Test
As described previously in our publications (34, 35), “we examined mechanical paw withdrawal threshold (PWT) of rat hindpaw in response to the stimulation of von Frey filaments. In brief, the filaments were bent for 5–10 s in our protocols. If a response was seen, we applied the filament of next lower force. If a response was not seen, we applied the filament of next greater force. To avoid injury during tests, the cutoff strength of the von Frey filament was 18 g. The tactile stimulus having a 50% likelihood of withdrawal was determined by the “up-down” method (36). We repeated each trial 2 times at ~2 min intervals. The mean value was used as the force producing a withdrawal response.”
We used Thermal Place Preference System to execute the thermal place preference test to assess a cold avoidance behavior as described in our previous study (34, 35). “We kept the first plate at neutral temperature (25°C) and the second plate at cold temperature (12°C). The test was executed in darkness and lasted 3 min and we left the rats free to explore both plates. The time spent on the cold plate was recorded using an infrared camera connected to a computer. To avoid learning or any place preference unrelated to cold, we inverted the temperature of the plates between two consecutive sessions. The animal studies were performed in a blind manner. Cold sensitivity was expressed as % time spent on the cold plate over 3 min [time on cold plate (seconds) /180 s × %].”
Statistical Analysis
We used SPSS for Windows version 13.0 to perform all statistical analyses; and all data were analyzed using a two-way repeated-measures analysis of variance with Tukey's post hoc tests. We presented values as means ± standard error of mean. For all analyses of this study, differences were considered significant at P < 0.05.
Results
MiR-155 After BTZ Intervention
First, we determined the changes of miR-155 evoked by BTZ. An increase of miR-155 was seen 3 days after the beginning of BTZ intervention (Figure 1B). According to this result, in the rest of experiments the time point (day 0) was selected for injection miR-155 inhibitor and mimics, and receptor antagonists.
Effects of MiR-155 TNFR1-TRPA1 Signal
In addition, our data presented in Figure 2A show that TNFR1, TRPA1 and p-p38-MAPK/p-JNK were increased in BTZ rats (P < 0.05, BTZ vs. control). Application of miR-155 inhibitor had attenuating effects on TNFR1-TRPA1 signal expression at the protein levels (P < 0.05, BTZ with inhibitor vs. BTZ and BTZ with scramble). In this experiment, protein expression of TNFR1, TRPA1 and p-p38-MAPK/p-JNK were not observed to be changed after application of miR-155 inhibitor scramble (P > 0.05, BTZ vs. BTZ with scramble).
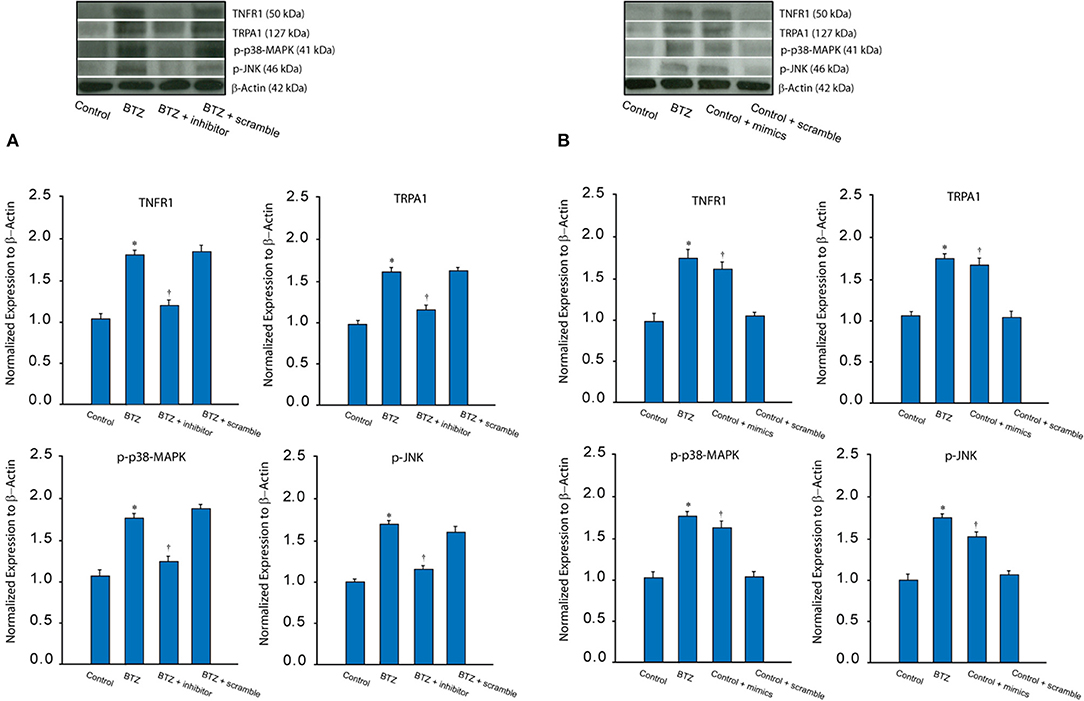
Figure 2. (A) Typical bands and averaged data show that BTZ increased the protein levels of TNFR1 (a subtype TNF-α receptor) and TRPA1 as well as intracellular signal p-p38-MAPK and p-JNK in the dorsal horn of the spinal cord as compared with controls. Furthermore, miR-155 inhibitor attenuated increases of these receptor and signal pathways in BTZ animals. Note that miR-155 inhibitor scramble did not alter upregulation of TNFR1, TRPA1 p-p38-MAPK/p-JNK induced by BTZ. *P < 0.05 vs. control rats and BTZ rats with inhibitor. †P < 0.05, BTZ rats with inhibitor vs. BTZ rats with scramble. n = 8–12 in each group. (B) Typical bands and averaged data show that TNFR1, TRPA1 and p-p38-MAPK/p-JNK were increased in the dorsal horn of control rats after intrathecal injection of miR-155 mimics; whereas those expressions were not altered after intrathecal injection of scramble. *P < 0.05 vs. control rats. †P < 0.05, control rats with mimics vs. control rats and control rats with scramble. n = 8–12 in each group.
Furthermore, we examined the effect of miR-155 mimics on TNFR1-TRPA1 signal pathways in control rats. Figure 2B shows that miR-155 mimics, but not its scramble, amplified TNFR1, TRPA1 and p-p38-MAPK/p-JNK (P < 0.05, control with mimics vs. control and control with scramble). Insignificant difference was seen in TNFR1-TRPA1 between control rats and control rats with miR-155 mimics scramble (P > 0.05).
Effects of MiR-155 on Pain Response
Less PWT was seen in BTZ rats compared with control rats (Figure 3A). PWT was amplified 1 day after miR-155 inhibitor in BTZ rats and this effect was observed to last for 7 days (P < 0.05, BTZ vs. BTZ with inhibitor). In comparison with miR-155 inhibitor, its scramble failed to alter PWT in BTZ rats (P > 0.05, BTZ vs. BTZ with scramble). Figure 3B shows that BTZ decreased % time spent on the cold plate. MiR-155 inhibitor increased % time spent on the cold plate in BTZ rats (P < 0.05, BTZ vs. BTZ with inhibitor). Similarly, miR-155 inhibitor scramble had no effects in BTZ rats (P > 0.05, BTZ vs. BTZ with scramble).
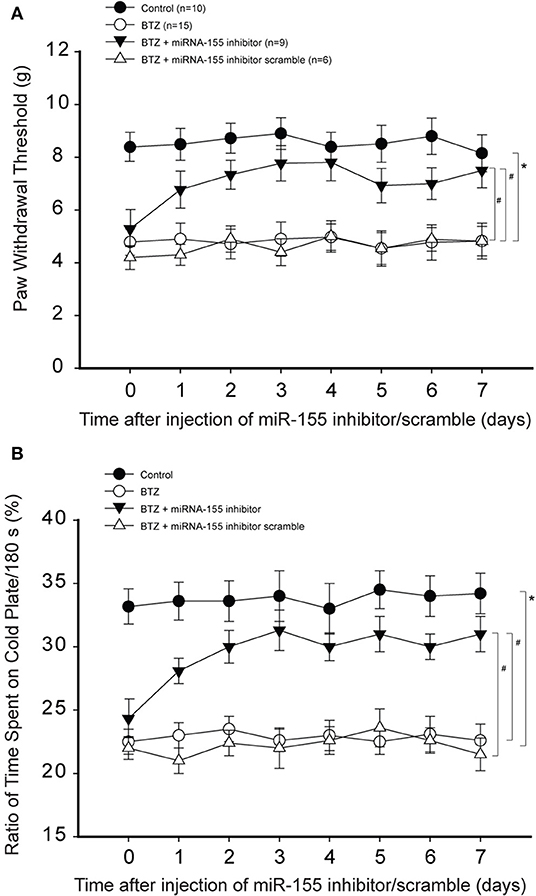
Figure 3. Effects of miR-155 inhibitor on mechanical and cold sensitivity. Paw withdrawal threshold (PWT) and cold sensitivity expressed as time spent on the cold plate (%) were examined in control rats and BTZ rats. (A) PWT was smaller in BTZ than in control rats at different time courses. Intrathecal injection of miR-155 inhibitor (3 μg each day over three consecutive days), but not its scramble, increased PWT in BTZ rats 1 day after its injection. (B) showing that time spent on the cold plate was less in BTZ rats than in control rats. MiR-155 inhibitor elevated % time spent on the cold plates in BTZ rats 1 day after its injection. Mir-155 inhibitor scramble had no effects on % time spent on the cold plates in BTZ rats. *P < 0.05, BTZ rats vs. control rats. #P < 0.05, BTZ rats with inhibitor vs. BTZ rats and BTZ rats with scramble. The number of rats in each group was shown on the figure.
Next, we determined the effects of miR-155 mimics on PWT and % time spent on the cold plate in control rats. Figure 4A demonstrates that miR-155 mimics decreased PWT in control rats (P < 0.05, control vs. control with mimics), but miR-155 mimics scramble did not have significant effects on PWT. Similarly, Figure 4B shows that miR-155 mimics, but not its scramble, decreased % time spent on the cold plate in control rats (P < 0.05, control with mimics vs. control and control with scramble).
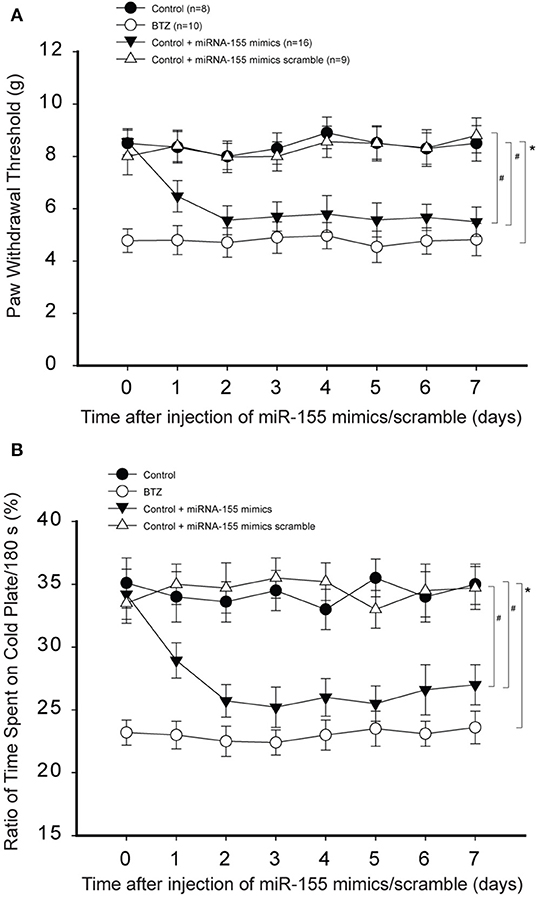
Figure 4. Effects of miR-155 mimics on mechanical and cold sensitivity. Paw withdrawal threshold (PWT) and cold sensitivity expressed as time spent on the cold plate (%) were examined in control rats and BTZ rats. (A) Intrathecal injection of miR-155 mimics (3 μg each day over three consecutive days) decreased PWT in control rats 1 day after its injection as compared with scramble. (B) showing that time spent on the cold plate was less in BTZ rats than in control rats. In control rats, miR-155 mimics decreased % time spent on the cold plates one day after its injection, but miR-155 mimics scramble did not change % time spent on the cold plates. *P < 0.05, BTZ rats vs. control rats. #P < 0.05, control rats with mimics vs. control rats and control rats with scramble. The number of rats in each group was shown on the figure.
Effects of Inhibiting TNFR1-TRPA1 Pathway
We were to determine if the effects of miR-155 were via TNFR1-TRPA1 signals. Thus, we examined if blocking those signal pathways can attenuate hypersensitivity of mechanical and cold stimulation induced by miR-155 mimics in control rats. Figures 5A,B show that miR-155 mimics decreased PWT and decreased % time spent on the cold plate in control rats. As PTX or HC030031 was given by intrathecal injection, decreases in PWT and % time spent on the cold plate were mostly improved in rats with miR-155 mimics (P < 0.05, rats with mimics vs. rats with mimics plus PTX or HC030031).
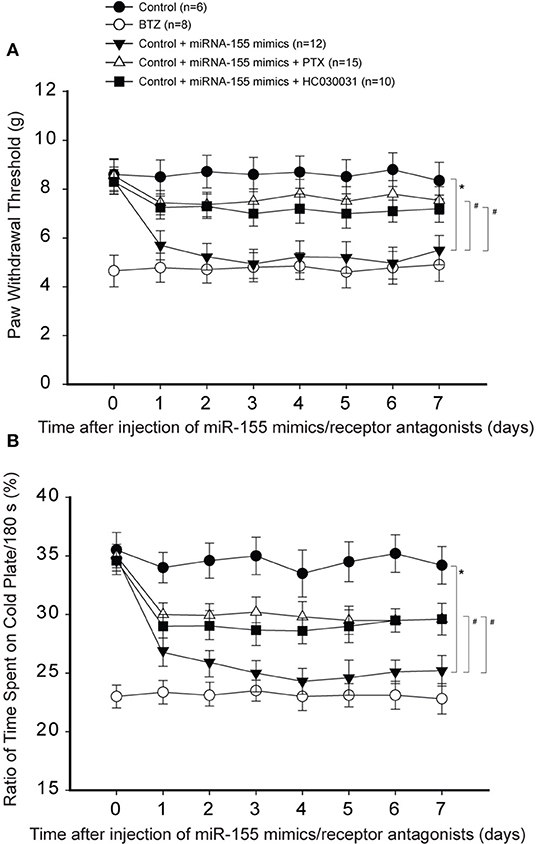
Figure 5. Effects of blocking TNFR1 and TRPA1 on mechanical and cold sensitivity. TNF-α signal was inhibited by pentoxifylline (PTX; 10 μg each day over three consecutive days). TRPA1 was blocked by administration of HC030031 (5 μg each day over three consecutive days). (A) PWT was smaller in BTZ than that in control rats for all time courses. As compared with controls, intrathecal injection of miR-155 mimics (3 μg each day over three consecutive days) significantly decreased PWT, and the effects of miR-155 mimics were attenuated by injection of PTX and HC030031. (B) showing that % time spent on the cold plate was less in BTZ rats and in rats with injection of miR-155 mimics. PTX and HC030031 attenuated the effects of miR-155 mimics. *P < 0.05, control rats vs. control rats with mimics; #P < 0.05, rats with mimics vs. rats with mimics plus PTX or mimics plus HC030031. The number of rats in each group was shown on the figure.
Likewise, Figures 6A,B demonstrate that inhibiting p38-MAPK and JNK using respective SB203580 and SP600125 also attenuated reductions in PWT and % time spent on the cold plate in animals with miR-155 mimics (P < 0.05, rats with mimics vs. rats with mimics plus SB203580 or SP600125).
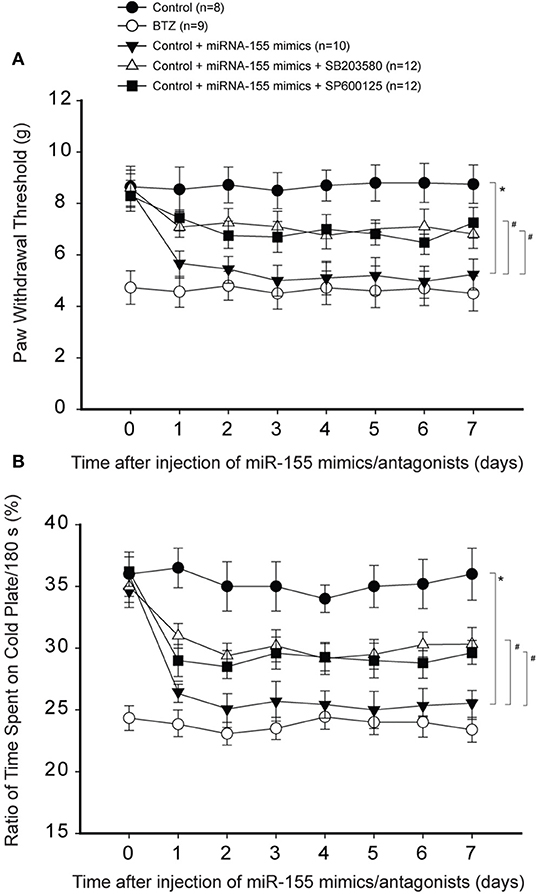
Figure 6. Effects of blocking p38-MAPK and JNK signal on mechanical and cold sensitivity. Intrathecal injection of SB203580 (5 μg each day over three consecutive days) and SP600125 (5 μg each day over three consecutive days) were performed to inhibit p38-MAPK and JNK signal pathway. (A,B) As compared with controls, intrathecal injection of miR-155 mimics (3 μg each day over three consecutive days) significantly decreased PWT and % time spent on the cold plate. With respective SB203580 and SP600125, decreased PWT and % time spent on the cold plate by miR-155 mimics were largely restored. *P < 0.05, control rats vs. control rats with mimics; #P < 0.05, rats with mimics vs. rats with mimics plus SB203580 or mimics plus SP600125. The number of rats in each group was shown on the figure.
Discussion
With progression of cancer, pain is one of the most common and distressing symptoms suffered by patients (37). Cancer pain can be due to a tumor compressing; nerve and other tissue changes caused by a hormone and immune responses; and/or treatments and diagnostic procedures (37, 38). Notably, pain caused by chemotherapy can continue even after the end of treatment (37, 39, 40). Therefore, cancer pain during chemotherapy is considered a significant issue in clinics.
BTZ is frequently applied for treatment of multiple myeloma (1–3), but painful neuropathy and heightened cold sensitivity are main complications during application of BTZ (3). Of note, BTZ induces neuropathic pain in rats after initiation of its chemotherapy (21–23). Indeed, the abnormal sensation was ablated many days after BTZ (21–23). Using this rat model, a recent study showed that blocking TNF-α and TRPA1 signal in the DRG attenuated mechanical hyperalgesia and cold hypersensitivity following BTZ, and the effects were via engagement of intracellular signals p38-MAPK and p-JNK (33).
The sensory nerves (neurons) and dorsal horn of the spinal cord paly a primary role in conducting signals of pain responses (16, 17). The prior reports suggest that TNF-α is increased in DRG and spinal dorsal horn after administration of BTZ (21, 22). We also observed that BTZ increased TNFR1-TRPA1 in the dorsal horn of rats. Interestingly, intrathecal application of miR-155 inhibitor attenuated TNFR1-TRPA1 and p38-MAPK and p-JNK in the dorsal horn of BTZ rats. This also attenuated mechanical hyperalgesia and cold hypersensitivity by BTZ. In contrast, miR-155 mimics injected into the dorsal horn of control rats amplified TNFR1-TRPA1 and led to mechanical hyperalgesia and cold hypersensitivity. In addition, blocking TNFR1-TRPA1 and p38-MAPK/p-JNK attenuated amplified pain by intrathecal injection of miR-155 mimics. Furthermore, we showed that miR-155 inhibitor decreased the protein levels of TRPA1 in BTZ rats. Likewise, miR-155 mimics increased the protein levels of TRPA1 in control rats. These results suggest that there is an interaction between miR-155 signal and activation of TRPA1 pathway. Overall, our data suggest the role of miR-155 in modifying mechanical hyperalgesia and cold hypersensitivity during BTZ therapy.
In general, miR-155 is involved with disease processes related to inflammation. For instance, deficiency of miR-155 inhibits IL-17 and decreases renal damage in nephropathy (41). The lack of miR-155 can decrease production of B and T cells in autoimmune arthritis (42). A high level of miR-155 is observed in patients with gouty arthritis compared with healthy controls (43). It was also reported that deficiency in miR-155 alleviates inflammatory bowel disease through downregulation of the Th1/Th17 (44). We demonstrated that BTZ amplifies miR-155 in the dorsal horn and miR-155 inhibitor can attenuate BTZ-induced TNF-α signal pathway leading to decreases of neuropathic pain. Moreover, miR-155 mimics amplifies pain response to mechanical and thermal stimulation.
Anti-inflammatory protein suppressor of cytokine signaling 1 (SOCS1) is a target gene of miR-155 (45). SOCS1 is a key regulator of inflammatory signals, negatively regulating the inflammation feedback (46). Deficiency of SOCS1 leads to amplified responsiveness to inflammatory stimuli in various cells or in animals (47, 48). In a prior study, the role of miR-155 and SOCS1 in neuropathic pain was identified (49). This prior study showed that miR-155 was upregulated in the dorsal horn after chronic constriction injury. MiR-155 inhibitor alleviated neuroinflammation and neuropathic pain. Also, miR-155 inhibitor suppressed NF-κB and p38-MAPK activation via SOCS1. Consistent with this previous finding, our current results showed that miR-155 mRNA is elevated in the dorsal horn after administration of BTZ and this increase remains for several days. This is accompanied with upregulation of protein expression of p38-MAPK as well as JNK. MiR-155 inhibitor attenuates p38-MAPK signal and attenuates BTZ-induced mechanical and thermal hypersensitivity. Thus, miR-155 is likely a potential target for the therapeutic intervention of neuropathic pain during BTZ therapy.
A variety of miRNAs has the role in pain processing in experimental and clinical pain (50). For instance, miR-203 is involved in neuropathic pain through Rap1a and its downstream signal MEK/ERK (51). Inhibition of miR-21 attenuates neuropathic pain in rats (52). Activation of miR-195 by peripheral nerve injury aggravates neuropathic pain via autophagy and this also leads to increases of IL-1β, and TNF-α (53). Thus, further studies are needed to determine the networks of miRNAs in engagement of neuropathic pain.
In general, cytotoxic drugs used for chemotherapy activate oxidative stress signals and thereby induce peripheral neuropathy (35, 54, 55). The previous study has demonstrated that TRPA1 is activated in the process of BTZ-induced pain and oxidative stress is involved in activation of the signal pathways (55). In this previous study (55), BTZ evoked mechanical, cold, and selective chemical hypersensitivity in mice and the effects of BTZ are reverted by treatment with the TRPA1 antagonist HC-030031 and by the oxidative stress scavenger α-lipoic acid. Thus, it is speculated that activation of oxidative stress signal is likely involved in the role of miR-155 in BTZ-induced neuropathic pain in our current study.
Neuropathic pain can last ~30 days after the end of BTZ administration. We need to acknowledge a study limitation of the current report that we did not examine pain response >7 days after BTZ in this report. We examined the effects of miR-155 inhibitor and miR-155 mimics on neuropathic pain 1–7 days after BTZ. Our design was based on the reasons: (1) significant pain was developed after a few days after BTZ; and (2) we performed intrathecal delivery of inhibitor/mimics for three consecutive days. The main focus of this study was to determine if miR-155 inhibitor can attenuate neuropathic pain induced by BTZ. If we can show the effectiveness of inhibitor 7 days after BTZ, this would indicate that its effects can last longer with a longer usage of inhibitor (i.e., 30 days after BTZ). Nonetheless, we have shown that miR-155 inhibitor alleviated pain response 7 days after BTZ in our current report.
In conclusion, the protein expression levels of TNFR1 and TRPA1 and intracellular p38-MAPK/JNK in the dorsal horn are upregulated by BTZ. Inhibition of miR-155 decreased those signal pathway expression and attenuated mechanical allodynia and thermal hyperalgesia in BTZ rats. In addition, miRNA-155 mimics increased mechanical pain and cold sensitivity without injection of BTZ. Mechanical and thermal hypersensitivity induced by miRNA-155 mimics were attenuated after blocking TNFR1, TRPA, p38-MAPK, and JNK. We provided evidence for the role of miR-155 in regulating BTZ-induced neuropathic pain through TNFR1-TRPA1 pathway, having clinical implications that miR-155 is a potential target in preventing neuropathic pain during BTZ chemotherapeutics.
Data Availability Statement
The datasets generated for this study are available on request to the corresponding author.
Ethics Statement
The animal study was reviewed and approved by Institutional Animal Care and Use Committee of Jilin University.
Author Contributions
ZD and JZ contributed to the data collection and analysis and drafted the paper. JL and XP contributed to the data analysis. HW contributed to the experimental designs of this study and oversaw all data collection and analysis and reviewed manuscript.
Conflict of Interest
The authors declare that the research was conducted in the absence of any commercial or financial relationships that could be construed as a potential conflict of interest.
References
1. Adams J, Kauffman M. Development of the proteasome inhibitor Velcade (Bortezomib). Cancer Invest. (2004) 22:304–11. doi: 10.1081/CNV-120030218
2. Voorhees PM, Dees EC, O'Neil B, Orlowski RZ. The proteasome as a target for cancer therapy. Clin Cancer Res. (2003) 9:6316–25.
3. Curran MP, McKeage K. Bortezomib: a review of its use in patients with multiple myeloma. Drugs. (2009) 69:859–88. doi: 10.2165/00003495-200969070-00006
4. Bartel DP. MicroRNAs: target recognition and regulatory functions. Cell. (2009) 136:215–33. doi: 10.1016/j.cell.2009.01.002
5. Sayed D, Abdellatif M. MicroRNAs in development and disease. Physiol Rev. (2011) 91:827–87. doi: 10.1152/physrev.00006.2010
6. Eacker SM, Dawson TM, Dawson VL. Understanding microRNAs in neurodegeneration. Nat Rev Neurosci. (2009) 10:837–41. doi: 10.1038/nrn2726
7. Farazi TA, Spitzer JI, Morozov P, Tuschl T. miRNAs in human cancer. J Pathol. (2011) 223:102–15. doi: 10.1002/path.2806
8. Han M, Toli J, Abdellatif M. MicroRNAs in the cardiovascular system. Curr Opin Cardiol. (2011) 26:181–9. doi: 10.1097/HCO.0b013e328345983d
9. Gambari R, Fabbri E, Borgatti M, Lampronti I, Finotti A, Brognara E, et al. Targeting microRNAs involved in human diseases: a novel approach for modification of gene expression and drug development. Biochem Pharmacol. (2011) 82:1416–29. doi: 10.1016/j.bcp.2011.08.007
10. Calame K. MicroRNA-155 function in B Cells. Immunity. (2007) 27:825–7. doi: 10.1016/j.immuni.2007.11.010
11. Elton TS, Selemon H, Elton SM, Parinandi NL. Regulation of the MIR155 host gene in physiological and pathological processes. Gene. (2013) 532:1–12. doi: 10.1016/j.gene.2012.12.009
12. Faraoni I, Antonetti FR, Cardone J, Bonmassar E. miR-155 gene: a typical multifunctional microRNA. Biochim Biophys Acta. (2009) 1792:497–505. doi: 10.1016/j.bbadis.2009.02.013
13. O'Connell RM, Rao DS, Baltimore D. microRNA regulation of inflammatory responses. Annu Rev Immunol. (2012) 30:295–312. doi: 10.1146/annurev-immunol-020711-075013
14. Sonkoly E, Janson P, Majuri ML, Savinko T, Fyhrquist N, Eidsmo L, et al. MiR-155 is overexpressed in patients with atopic dermatitis and modulates T-cell proliferative responses by targeting cytotoxic T lymphocyte-associated antigen 4. J Allergy Clin Immunol. (2010) 126:581-9.e5–20. doi: 10.1016/j.jaci.2010.05.045
15. Moore CS, Rao VT, Durafourt BA, Bedell BJ, Ludwin SK, Bar-Or A, et al. miR-155 as a multiple sclerosis-relevant regulator of myeloid cell polarization. Ann Neurol. (2013) 74:709–20. doi: 10.1002/ana.23967
16. Clark AK, Old EA, Malcangio M. Neuropathic pain and cytokines: current perspectives. J Pain Res. (2013) 6:803–14. doi: 10.2147/JPR.S53660
17. Mika J, Zychowska M, Popiolek-Barczyk K, Rojewska E, Przewlocka B. Importance of glial activation in neuropathic pain. Eur J Pharmacol. (2013) 716:106–19. doi: 10.1016/j.ejphar.2013.01.072
18. Moalem G, Tracey DJ. Immune and inflammatory mechanisms in neuropathic pain. Brain Res Rev. (2006) 51:240–64. doi: 10.1016/j.brainresrev.2005.11.004
19. Miller RJ, Jung H, Bhangoo S, White FA. Cytokine and chemokine regulation of sensory neuron function. In: Canning BJ, Spina DV, editors. Handbook of Experimental Pharmacology. Berlin Heidelberg: Springer (2009). p. 417–49.
20. Carozzi VA, Canta A, Chiorazzi A. Chemotherapy-induced peripheral neuropathy: what do we know about mechanisms? Neurosci Lett. (2015) 596:90–107. doi: 10.1016/j.neulet.2014.10.014
21. Zhang J, Su YM, Li D, Cui Y, Huang ZZ, Wei JY, et al. TNF-alpha-mediated JNK activation in the dorsal root ganglion neurons contributes to Bortezomib-induced peripheral neuropathy. Brain Behav Immun. (2014) 38:185–91. doi: 10.1016/j.bbi.2014.01.020
22. Li ZY, Zhang YP, Zhang J, Zhang SB, Li D, Huang ZZ, et al. The possible involvement of JNK activation in the spinal dorsal horn in bortezomib-induced allodynia: the role of TNF-alpha and IL-1beta. J Anesth. (2016) 30:55–63. doi: 10.1007/s00540-015-2077-x
23. Chiorazzi A, Canta A, Meregalli C, Carozzi V, Sala B, Oggioni N, et al. Antibody against tumor necrosis factor-alpha reduces bortezomib-induced allodynia in a rat model. Anticancer Res. (2013) 33:5453−9.
24. Andersson DA, Gentry C, Moss S, Bevan S. Transient receptor potential A1 is a sensory receptor for multiple products of oxidative stress. J Neurosci. (2008) 28:2485–94. doi: 10.1523/JNEUROSCI.5369-07.2008
25. Bandell M, Story GM, Hwang SW, Viswanath V, Eid SR, Petrus MJ, et al. Noxious cold ion channel TRPA1 is activated by pungent compounds and bradykinin. Neuron. (2004) 41:849–57. doi: 10.1016/S0896-6273(04)00150-3
26. Bautista DM, Movahed P, Hinman A, Axelsson HE, Sterner O, Hogestatt ED, et al. Pungent products from garlic activate the sensory ion channel TRPA1. Proc Natl Acad Sci USA. (2005) 102:12248–52. doi: 10.1073/pnas.0505356102
27. Jordt SE, Bautista DM, Chuang HH, McKemy DD, Zygmunt PM, Hogestatt ED, et al. Mustard oils and cannabinoids excite sensory nerve fibres through the TRP channel ANKTM1. Nature. (2004) 427:260–5. doi: 10.1038/nature02282
28. Sawada Y, Hosokawa H, Matsumura K, Kobayashi S. Activation of transient receptor potential ankyrin 1 by hydrogen peroxide. Eur J Neurosci. (2008) 27:1131–42. doi: 10.1111/j.1460-9568.2008.06093.x
29. Kwan KY, Allchorne AJ, Vollrath MA, Christensen AP, Zhang DS, Woolf CJ, et al. TRPA1 contributes to cold, mechanical, and chemical nociception but is not essential for hair-cell transduction. Neuron. (2006) 50:277–89. doi: 10.1016/j.neuron.2006.03.042
30. Story GM, Peier AM, Reeve AJ, Eid SR, Mosbacher J, Hricik TR, et al. ANKTM1, a TRP-like channel expressed in nociceptive neurons, is activated by cold temperatures. Cell. (2003) 112:819–29. doi: 10.1016/S0092-8674(03)00158-2
31. Zhao M, Isami K, Nakamura S, Shirakawa H, Nakagawa T, Kaneko S. Acute cold hypersensitivity characteristically induced by oxaliplatin is caused by the enhanced responsiveness of TRPA1 in mice. Mol Pain. (2012) 8:55. doi: 10.1186/1744-8069-8-55
32. Nassini R, Fusi C, Materazzi S, Coppi E, Tuccinardi T, Marone IM, et al. The TRPA1 channel mediates the analgesic action of dipyrone and pyrazolone derivatives. Br J Pharmacol. (2015) 172:3397–3411. doi: 10.1111/bph.13129
33. Li C, Deng T, Shang Z, Wang D, Xiao Y. Blocking TRPA1 and TNF-alpha signal improves bortezomib-induced neuropathic pain. Cell Physiol Biochem. (2018) 51:2098–110. doi: 10.1159/000495828
34. Duan Z, Li J, Pang X, Wang H, Su Z. Blocking mammalian target of rapamycin (mTOR) alleviates neuropathic pain induced by chemotherapeutic bortezomib. Cell Physiol Biochem. (2018) 48:54–62. doi: 10.1159/000491662
35. Miao F, Wang R, Cui G, Li X, Wang T, Li X. Engagement of microRNA-155 in exaggerated oxidative stress signal and TRPA1 in the dorsal horn of the spinal cord and neuropathic pain during chemotherapeutic oxaliplatin. Neurotox Res. (2019) 36:712–23. doi: 10.1007/s12640-019-00039-5
36. Chaplan SR, Bach FW, Pogrel JW, Chung JM, Yaksh TL. Quantitative assessment of tactile allodynia in the rat paw. J Neurosci Methods. (1994) 53:55–63. doi: 10.1016/0165-0270(94)90144-9
38. Pasetto LM, D'Andrea MR, Rossi E, Monfardini S. Oxaliplatin-related neurotoxicity: How and why? Crit Rev Oncol Hematol. (2006) 59:159–68. doi: 10.1016/j.critrevonc.2006.01.001
39. Hoskin PJ. Radiotherapy. In: Clinical pain management: Cancer Pain. London: Hodder Arnold (2008). p. 251–5.
40. Portenoy RK. Treatment of cancer pain. Lancet. (2011) 377:2236–47. doi: 10.1016/S0140-6736(11)60236-5
41. Lin X, You Y, Wang J, Qin Y, Huang P, Yang F. MicroRNA-155 deficiency promotes nephrin acetylation and attenuates renal damage in hyperglycemia-induced nephropathy. Inflammation. (2015) 38:546–54. doi: 10.1007/s10753-014-9961-7
42. Bluml S, Bonelli M, Niederreiter B, Puchner A, Mayr G, Hayer S, et al. Essential role of microRNA-155 in the pathogenesis of autoimmune arthritis in mice. Arthritis Rheum. (2011) 63:1281–8. doi: 10.1002/art.30281
43. Jin HM, Kim TJ, Choi JH, Kim MJ, Cho YN, Nam KI, et al. MicroRNA-155 as a proinflammatory regulator via SHIP-1 down-regulation in acute gouty arthritis. Arthritis Res Ther. (2014) 16:R88. doi: 10.1186/ar4531
44. Singh UP, Murphy AE, Enos RT, Shamran HA, Singh NP, Guan H, et al. miR-155 deficiency protects mice from experimental colitis by reducing T helper type 1/type 17 responses. Immunology. (2014) 143:478–89. doi: 10.1111/imm.12328
45. Cardoso AL, Guedes JR, Pereira de Almeida L, Pedroso de Lima MC. miR-155 modulates microglia-mediated immune response by down-regulating SOCS-1 and promoting cytokine and nitric oxide production. Immunology. (2012) 135:73–88. doi: 10.1111/j.1365-2567.2011.03514.x
46. Yasukawa H, Sasaki A, Yoshimura A. Negative regulation of cytokine signaling pathways. Annu Rev Immunol. (2000) 18:143–64. doi: 10.1146/annurev.immunol.18.1.143
47. Chinen T, Kobayashi T, Ogata H, Takaesu G, Takaki H, Hashimoto M, et al. Suppressor of cytokine signaling-1 regulates inflammatory bowel disease in which both IFNgamma and IL-4 are involved. Gastroenterology. (2006) 130:373–88. doi: 10.1053/j.gastro.2005.10.051
48. Hanada T, Yoshida H, Kato S, Tanaka K, Masutani K, Tsukada J, et al. Suppressor of cytokine signaling-1 is essential for suppressing dendritic cell activation and systemic autoimmunity. Immunity. (2003) 19:437–50. doi: 10.1016/S1074-7613(03)00240-1
49. Tan Y, Yang J, Xiang K, Tan Q, Guo Q. Suppression of microRNA-155 attenuates neuropathic pain by regulating SOCS1 signalling pathway. Neurochem Res. (2015) 40:550–60. doi: 10.1007/s11064-014-1500-2
50. Andersen HH, Duroux M, Gazerani P. MicroRNAs as modulators and biomarkers of inflammatory and neuropathic pain conditions. Neurobiol Dis. (2014) 71:159–68. doi: 10.1016/j.nbd.2014.08.003
51. Li H, Huang Y, Ma C, Yu X, Zhang Z, Shen L. MiR-203 involves in neuropathic pain development and represses Rap1a expression in nerve growth factor differentiated neuronal PC12 cells. Clin J Pain. (2015) 31:36–43. doi: 10.1097/AJP.0000000000000070
52. Sakai A, Suzuki H. Nerve injury-induced upregulation of miR-21 in the primary sensory neurons contributes to neuropathic pain in rats. Biochem Biophys Res Commun. (2013) 435:176–81. doi: 10.1016/j.bbrc.2013.04.089
53. Shi G, Shi J, Liu K, Liu N, Wang Y, Fu Z, et al. Increased miR-195 aggravates neuropathic pain by inhibiting autophagy following peripheral nerve injury. Glia. (2013) 61:504–12. doi: 10.1002/glia.22451
54. Shim HS, Bae C, Wang J, Lee KH, Hankerd KM, Kim HK, et al. Peripheral and central oxidative stress in chemotherapy-induced neuropathic pain. Mol Pain. (2019) 15:1–11. doi: 10.1177/1744806919840098
Keywords: microRNA-155, bortezomib, chemotherapeutics, multiple myeloma, neuropathy
Citation: Duan Z, Zhang J, Li J, Pang X and Wang H (2020) Inhibition of microRNA-155 Reduces Neuropathic Pain During Chemotherapeutic Bortezomib via Engagement of Neuroinflammation. Front. Oncol. 10:416. doi: 10.3389/fonc.2020.00416
Received: 25 November 2019; Accepted: 10 March 2020;
Published: 31 March 2020.
Edited by:
David A. Gewirtz, Virginia Commonwealth University, United StatesReviewed by:
Francesco De Logu, University of Florence, ItalyAmarjit Luniwal, North American Science Associates Inc., United States
Copyright © 2020 Duan, Zhang, Li, Pang and Wang. This is an open-access article distributed under the terms of the Creative Commons Attribution License (CC BY). The use, distribution or reproduction in other forums is permitted, provided the original author(s) and the copyright owner(s) are credited and that the original publication in this journal is cited, in accordance with accepted academic practice. No use, distribution or reproduction is permitted which does not comply with these terms.
*Correspondence: Hushan Wang, d2FuZ2h1c2hhbkBhb2wuY29t
†These authors have contributed equally to this work and share first authorship