Corrigendum: Oncolytic Adenovirus—A Nova for Gene-Targeted Oncolytic Viral Therapy in HCC
- 1Department of Medical Oncology, School of Medicine, Jinling Hospital, Nanjing University, Nanjing, China
- 2Department of Medical Oncology, Jinling Hospital, Nanjing Clinical School of Southern Medical University, Nanjing, China
- 3Department of Medical Oncology, Jinling Hospital, Nanjing, China
Hepatocellular carcinoma (HCC) is one of the most frequent cancers worldwide, particularly in China. Despite the development of HCC treatment strategies, the survival rate remains unpleasant. Gene-targeted oncolytic viral therapy (GTOVT) is an emerging treatment modality—a kind of cancer-targeted therapy—which creates viral vectors armed with anti-cancer genes. The adenovirus is a promising agent for GAOVT due to its many advantages. In spite of the oncolytic adenovirus itself, the host immune response is the determining factor for the anti-cancer efficacy. In this review, we have summarized recent developments in oncolytic adenovirus engineering and the development of novel therapeutic genes utilized in HCC treatment. Furthermore, the diversified roles the immune response plays in oncolytic adenovirus therapy and recent attempts to modulate immune responses to enhance the anti-cancer efficacy of oncolytic adenovirus have been discussed.
Introduction
Hepatocellular carcinoma (HCC) is one of the most frequent cancers worldwide and the third leading cause of cancer death. Even though great signs of progress have been made in standard therapies such as surgical removal, chemotherapy, and radiotherapy, the outcome remains disappointing. For this reason, the search for a new therapeutic approach is necessary. There is a bright new area in cancer-targeted therapy; “Gene Targeted Oncolytic Viral Therapy” (GTOVT) is considered a promising HCC therapy where oncolytic viruses are able to infect and kill cancer cells with no harm for normal cells (1). To date, a number of clinical trials have been conducted on oncolytic virotherapy for the treatment of HCC, as shown in Table 1. It is hoped that it will be used clinically in the future.
Oncolytic virotherapy was first reported a century ago, in 1912, by De Pace. He accidentally found that cervical cancer regressed in a patient who received Pasteur's attenuated rabies vaccine following a dog bite (2). In 2015, T-VEC (ImlygicTM), the oncolytic herpes virus, was approved in the western world for the treatment of advanced malignant melanoma (3) and became the first clinically used virus agent. In addition to the herpes virus, adenovirus, measles virus, reovirus, and Newcastle disease virus are used in oncolytic virotherapy. Among them, adenovirus vectors based on serotype 5 (Ad5) had been used widely due to several well-established advantages (4). First, a high titer of virus production can be easily produced [an average 1012 viral particles per milliliter (vp/ml) on each purification when 109~1010(vp/ml) is used in clinic]. Second, they transduce both dividing and non-dividing cells efficiently. Most importantly, adenovirus backbones are flexible for genetic modifications. Thus, the adenovirus became the most feasible agent owing to its high degree of customizability (5, 6).
Oncolytic adenovirus anti-cancer efficacy is mostly determined by the host immune response. As for a pathogen, the adenovirus triggers a robust host immune response after infection. This kind of immune response is considered a “double-edged sword” as an oncolytic adenovirus stimulates both the anti-virus and anti-tumor immune response (7). On one hand, the host anti-virus immune response should be reduced to guarantee oncolytic virus cancer-killing efficacy. On the other hand, the anti-cancer immune response elicited by the oncolytic adenovirus expands its anti-cancer efficacy, including the metastasis side (8). In order to achieve the largest cancer-killing efficacy, it is necessary for GTOVT to construct an oncolytic adenovirus by genetic or non-genetic modification to reduce immunogenicity and to equip immunostimulatory molecules. In this review, we specifically summarized the recent progress of the oncolytic adenovirus utilized in HCC treatment, including direct oncolytic virotherapy and virus-based immunotherapy.
Genetic Modifications of Oncolytic Adenovirus
Adenoviruses (Ads) are non-enveloped double-stranded DNA (dsDNA) viruses belonging to the Adenoviridae family (9). The Adenoviridae family is divided into seven species (A to G) (10) and includes 57 serotypes of the human virus. As for DNA viruses, the adenovirus has sufficient genome insertion capacity that is capable of carrying therapeutic genes of sizes of about 30–38 kb. Importantly, it could be expanded farther, up to 7.5 kb, by deleting viral genes such as the E3 gene (11). The Ads genome consists of four early genes (E1–E4), which are responsible for virus replication, and five late genes (L1–L5), code capsid proteins. The brief outline of each viral gene function is shown in figure below (Figure 1). At the onset of virus replication, the E1 gene is first expressed and then modulates other the expression of other virus genes. Thus, E1 gene deletion is commonly used in generating replication-deficient adenovirus vectors (11).
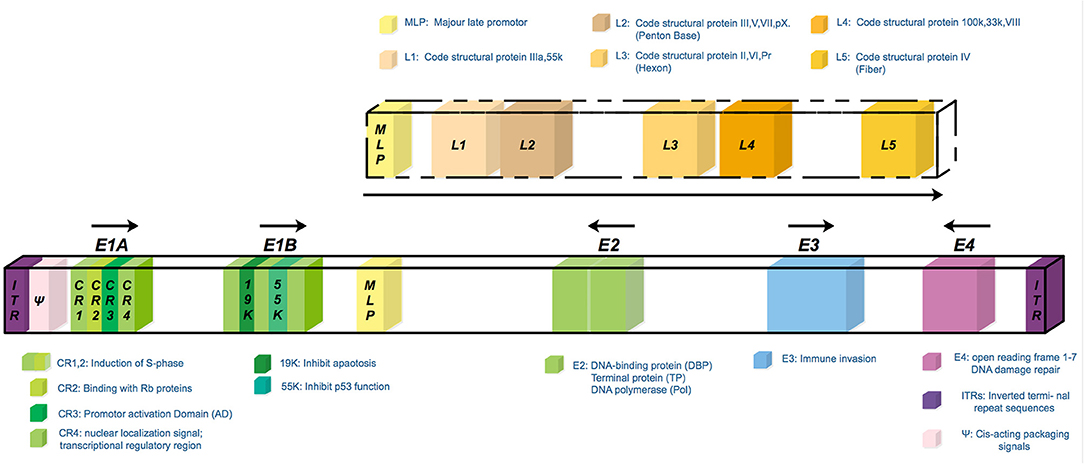
Figure 1. The scheme of the wild type serotype 5 adenovirus genome. The genome consists of four early transcription elements (E1, E2, E3, and E4) and five late expression genes (L1–L5) associated with adenoviral particle assembly. The E1 gene is required for the activation of the transcription of early genes; the E2 gene is required for virus DNA replication; the E3 gene is required for the modulation and evasion of the host's immune response, prevention of untimely cell death through apoptosis, and efficient cell lysis once new particle assembly is complete; the E4 gene is involved in virus RNA metabolism and transport, preferential downregulation of host-cell protein synthesis, and enhancement of virus DNA replication. The deletion of both the E1 and E3 genes can accommodate up to 7.5 kb of foreign DNA and is commonly used in gene therapy. E4 gene deletion further reduces induction of vector-specific immune responses and minimizes the outgrowth of replication-competent viruses in packaging cell lines.
Adenovirus infection is dependent on cellular receptors that mediate the adenovirus's partial transfer into human cells (10) (Figure 2). In general, adenoviruses from specious A, C, E, and F use the coxsackievirus-Ad receptor (CAR), while serotypes from species B and D tend to use alternative receptors such as CD46, CD80, CD86, and DSG-2 (12). They all depend on Integrins as the same secondary receptor (13). Considering CAR is widely expressed in human epithelium cells, especially in the respiratory tract (14), wild type adenoviruses are not feasible agents for oncolytic virotherapy with the lack of tumor cell selectivity. Thus, a lot of efforts have been made to artificially modify adenoviruses for the purpose of gaining both tumor cell targeting and killing efficacy (15).
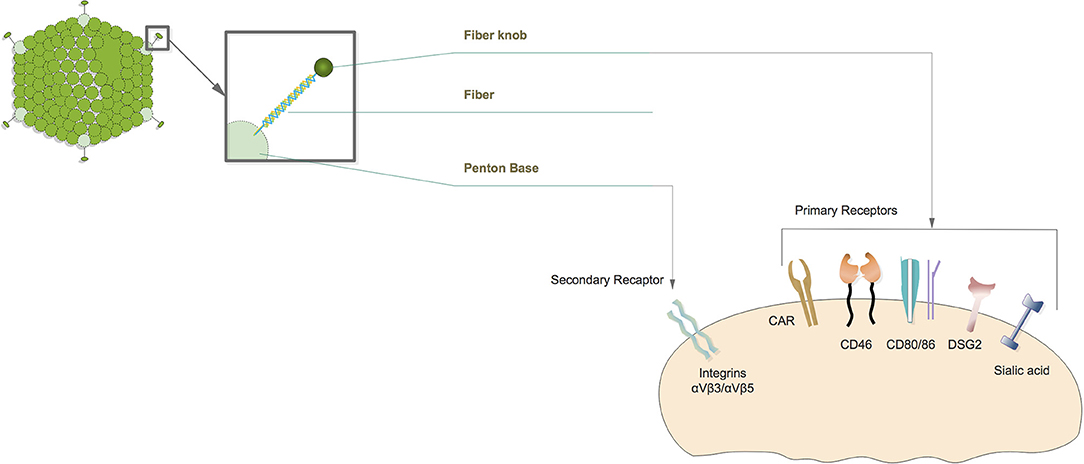
Figure 2. Diagram of oncolytic adenovirus infection. Oncolytic adenovirus infections initiated by fiber knob interact with the primary receptor on the cell membrane. Primary receptors are different according to adenovirus serotype. Afterwards, the penton base directly binds to secondary receptors that complete virus infection.
Genetic Modifications to Achieve Cancer Selectivity
Cancer selectivity is an indispensable feature of oncolytic adenoviruses to ensure effective anti-cancer efficacy while avoiding unwanted side effects. Several studies have reported that tumor selectivity can be accomplished by deleting a part of viral genes (Figure 3). For example, the conversed region 2 (CR2) of the adenovirus E1A gene coding protein displaces RB and the RB-related proteins from the E2F transcription family in infected cells (16), which consequently derives quiescent cells entering S-phase (17). This process is essential for virus replication in normal cells. However, CR2 is dispensable in cancer cells due to them having sufficient free E2Fs (16). Zhang et al. had therefore constructed the AdCV101 with 24 bp deleted (922–946) in the CR2 region of the E1A gene. Their results showed that the AdCV101 exhibited less cytotoxicity in normal cells in comparison with HCC cell lines (17).
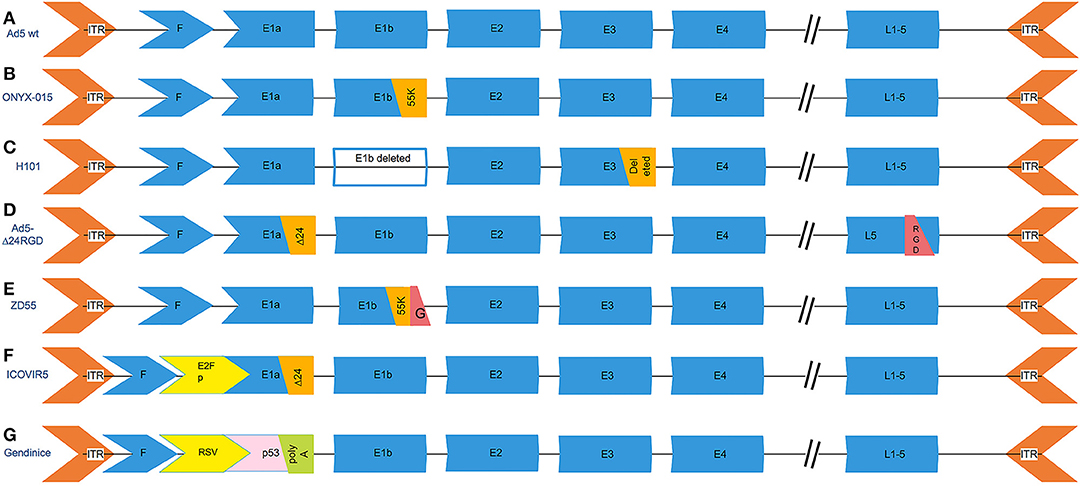
Figure 3. The genetic modifications of common oncolytic adenovirus vectors. (A) The genetic scheme of wild type serotype 5 adenovirus. (B) ONYX-015, the E1b-55k (2496-3323) region that is capable of replication in p53-deficient human tumor cells was deleted. (C) H101, deleting the entire E1B gene and a 78.3–85.8 nm gene segment in the E3 region, which is responsible for the code of the adenovirus death protein. (D) Ad5-Δ24RGD carries a 24-bp (919–943) deletion in the E1A region that is responsible for binding Rb protein and RGD motif insertion into the fiber. (E) ZD55 deleted the E1B 55-kD gene and armed with foreign gene. (F) ICOVIR5, the oncolytic adenovirus in which the endogenous E1A promoter has been replaced by the human E2F-1 promoter insulated with the DM-1. (G) Gendicine is a recombinant human serotype 5 adenovirus in which the E1 region is replaced by a human wild-type p53 expression cassette. Gendicine is a recombinant human serotype 5 adenovirus in which the E1 region is replaced by a human wild-type p53 expression cassette. The p53 gene is driven by a Rous sarcoma virus (RSV) promoter with a bovine growth hormone (BGH) poly(A) tail.
Furthermore, the E1B gene encodes two distinct tumor antigens, the 19-kDa (18) and 55-kDa proteins. The E1B-19 kDa protein is a homolog of Bcl-2 related apoptosis inhibitors (19–22). It has been demonstrated that the E1B-19KDa protein promotes cellular transformation by inhibiting E1A-induced apoptosis in a p53-independent manner (16). The E1B-55KDa protein is also capable of arresting the E1A apoptotic effect through p53-stabilization. Meanwhile, the E1B-55kDa protein forms a complex with the E4-orf6 gene product. Together, they mediate the viral mRNA accumulation and inhibit host cell mRNA transport and translation (23). leiOXYN-15, the E1B-55 kDa gene-deleted adenovirus, exhibited an excellent anti-cancer effect in clinical trials in head and neck cancer (24, 25). At first, its tumor selectivity was thought to be a consequence of inactivating mutations or deletions of the p53 gene in cancer cells (26, 27). However, OXYN-015 replication had been observed in several tumor cell lines that retain wild-type p53 sequences (28). These results made the precise role of p53 in ONYX- 015 selectivity more controversial. Later on, O'Shea et al. confirmed that the difference in RNA export between normal and tumor cells is a determinant of ONYX-015 oncolytic selectivity but not p53 inactivation (28). In addition, Sharon et al. explored that the E1b-55K domain is required for the inhibition of the host DNA damage response, which is important for adenovirus replication in HepG2 cells, and 2-Aminopurine can compensate for this domain loss (29).
However, the tumor selectivity of oncolytic adenoviruses that is produced simply by deleting viral genes is not qualified for avoiding unwanted tissue damage. Recently, cancer-specific promoters have become the most popular implement that controls oncolytic adenoviruses strictly targeted to cancer cells. In comparison to AdCN101, AdCN102, and AdCN103 exhibit an impressive reduction of virus replication in normal cells that were driven by an hTERT promoter (17). For liver cancer in particular, CV890, which was regulated by an AFP-promoter, selectively replicates in AFP-producing cells (30). The recently used oncolytic adenoviral vectors targeting HCC and their promoters are listed in the Table 2. In addition to the promoter itself, Kim et al. inserted a transcription enhancer to the distal region of a promoter to enhance transcriptional activity without specific loss (47). The apolipoprotein E (ApoE) promoter compensated for liver-specific PEPCK promoter transcription activity, which was hampered by a trans-splicing ribozyme (48).
In addition to active transduction selectivity, further ablation of the native tropism (for example, CAR-expressing normal cells) and/or modification of the vector to infect cells that are naturally not a target (for example, non-CAR-expressing cancer cells) is required. Fiber modification is commonly used to direct oncolytic adenovirus tumor tropism with the purpose of facilitating adenovirus transduction. The attachment of the globular knob domain on the adenovirus capsid protein to the cell surface CAR is the initial step of virus internalization (49). Thus, it is a basal element that mediates oncolytic adenovirus tumor-killing efficacy. Stepanenk et al. had classified recent modifications on the adenovirus vector fiber knob into five categories: (1) an incorporation of a targeting peptide into the fiber knob domain (the HI loop and/or C-terminus); (2) fiber knob serotype switching, or pseudotyping, by constructing chimeric fibers consisting of the knob domain derived from an alternate serotype (e.g., Ad5/3 or Ad5/35 chimeras [63#]), which binds to a receptor(s) other than CAR (e.g., desmoglein 2/DSG2 and/or CD46); (3) “fiber complex mosaicism,” an approach that combines serotype chimerism with peptide ligand(s) incorporation (e.g., Ad5/3-RGD); 4) “dual fiber mosaicism” by expressing two separate fibers with distinct receptor-binding capabilities on the same viral particle (e.g., Ad5-5/3 or Ad5-5/σ1); (5) fiber xenotyping by replacing the knob and shaft domains of wild-type Ad5 fiber protein with the fibrin itrimerization domain of a T4 bacteriophage or σ1 attachment protein of a reovirus (49). These modifications not only facilitate oncolytic adenovirus infection but may alleviate anti-virus immune responses that reduce virus immunogenicity by altering capsid antigens. However, it certainly had its limitation that the removal or exchange of virulence factors often limits virus replication (50).
Genetic Modifications to Increase Cancer Cell Killing Efficacy
Other than cancer selectivity, oncolytic adenoviruses have one more indispensable feature, which is a strong cancer cell-killing efficacy. As described above, adenoviruses have enough genome capacity for a therapeutic gene vector. Furthermore, an increasing amount of research has reported that oncolytic adenoviruses are capable of infecting and expressing exogenous functional genes with no target cells. The functional/effector genes can be classified in four categories by their functions: (1) Tumor-suppressor gene (51, 52); (2) Cytotoxic gene; (3) Immune-modulating gene (53); (4) Tumor-antigens (54). The following is the detailed introduction of genes carried by oncolytic adenoviral vectors for HCC treatment.
Tumor Suppressor Genes
Vestigial-like (VGLL) proteins are transcriptional co-factors that have four members, VGLL 1–4. Among them, VGLL 4 has been studied exclusively. VGLL4 inhibits the YAP-TEAD transcription complex through direct competition with YAP for the binding of TEAD, which is the key member of the Hpo pathway (31). The widespread overexpression/overactivation of YAP has attracted much interest in VGLL4 as a therapeutic agent (55). It had been explored that VGLL4 has a tumor suppressor efficacy in lung, gastric, colorectal, and breast cancer (56–58). In addition to the transcription co-factor, Jin et al. found it is also a member of the binding Inhibitors of apoptosis proteins (IAP), which negatively regulate apoptosis (56). This evidence indicated that overexpression of VGLL4 in cancer cells might exhibit an anti-tumor effect, not only through YAP-dependent gene induction but also by the apoptosis pathway. Xie et al. had constructed an oncolytic virus Ad-sp-VGLL4 carrying the VGLL4 gene which regulated by survivin promotor that target to HCC cell line. As expected, Ad-sp-VGLL4 possesses a strong anti-tumor capacity. Their study first reported that over-expression of VGLL4 arrests the HCC cell cycle at the G2/M phase and restrains the S phase simultaneously. Therefore, there is a potential that Ad-sp-VGLL4 can be utilized for the treatment of HCC therapy (55). In addition to VGLL4, the other IAP-binding protein, X-linked inhibitor of apoptosis (XIAP)-associated factor 1 (XAF-1), is also regarded as a tumor suppressor. It had been discerned that X-linked IAP (XIAP) prevents the activities of caspase-3,−7, and−9 via directly binding to these caspases (59). The XAF-1 specifically inhibits XIAP and induces cancer cell apoptosis (60). Li et al. discovered that the co-delivery of XAF-1 and TNF-α via an adenovirus synergistically inhibits the apoptosis and proliferation of MHCC97L cells (39). They utilized 2A self-cleaved peptides to link XAF-1 and TNF-α coding sequences in one ORF, which is transcribed into one mRNA molecule, whereas it is translated into two different, function-independent proteins (61). Furthermore, it had been demonstrated that the constitutive expression of XIAP is also related to HCC resistance to Tumor necrosis factor-related apoptosis-inducing ligand (TRAIL) (42, 62, 63). Thus, Wei et al. utilized two independent oncolytic adenoviruses, AFP-D55-SOCS3 and AFP-D55-TRAIL (2:3 ratio), as a combined therapy for HCC. Their study showed that the restoration of SOCS-3 by an adenovirus antagonized TRAIL resistance by downregulating XIAP (64). SOCS-3 is a member of suppressors of cytokine signaling (SOCS) family, which consisting of eight members (CIS, SOCS-1 to 7) is well-investigated. The SOCSs, especially CIS, SOCS-1, SOCS-2, and SOCS-3, form a classical negative feedback circuit, inhibiting cytokine signaling pathways that are responsible for their induction. Particularly, SOCS-1 inhibits the JAK/STAT3 pathway by straightly binding to the JAK protein, while SOCS-3 binds to the activated receptor (38). As is well-known, JAK/STAT3 pathway activation is implicated in carcinogenesis. Therefore, AdCN305-SOCS1, the recombinant adenovirus expressing the SOSC-1 gene, exhibits an anticancer effect by inhibiting STAT3 phosphorylation and downregulating survivin, cyclin D1, Bcl-xL, and C-myc (65).
p53 is determined as a novel tumor-associated gene which mutations or deletions are related to most of the cancer formation. Since the frequency of p53 gene mutation/deletion is as high as 50.0% (average, 30.0%) in HCC (51), converting abnormal conformations of mutant p53 to normal p53 or enhancing the apoptosis of tumor cells by providing the exogenous p53 gene and the associated genes becomes a promising therapeutic strategy. The recombinant human wild-type p53-carrying adenovirus (Gendicine) is a pioneer gene therapy product approved for clinical use in China (66, 67). Aspp2 (apoptosis stimulating protein of p53-2) is one of the p53 binding proteins that enhances the p53 pro-apoptotic function (68). Interestingly, overexpression of Aspp2 presented anti-tumor effects in both a P53-dependent and independent manner (69). Aspp2 is therefore, to a certain extent, regarded as a tumor suppressor. Liu et al. demonstrated that Aspp2 increased colorectal cancer sensitivity to chemotherapy, especially to oxaliplatin, via inhibiting autophagy in a p53-independent pathway (70). Later on, they had generated a recombinant human adenovirus vector carrying the Aspp2 gene for use in HCC treatment in combination with oxaliplatin and found that Assp2-Ad inhibits hepatocarcinoma, independently of p53, in coordination with oxaliplatin (51).
Cytotoxic Genes
There are three types of primary intracellular antioxidant enzymes in mammalian cells: Superoxide dismutase (SOD), catalase, and peroxides. The SODs convert O-2 into H2O2, whereas the catalases and peroxidases convert H2O2 into water. The antioxidant enzymes are essential for preventing or repairing the damage caused by reactive oxygen species (71). It had been demonstrated that O-2 may act as a second messenger molecule to promote cell proliferation due to its generation markedly increased in ras-transfected fibroblasts (40). Huang et al. had found that the transduction of adenoviral MnSOD alone is enough to kill ras-transformed cells. They generated a dual-regulated AD55-Mn-SOD adenovirus, on the basis of a ZD55 backbone with E1B55-kDa-deletion of Ad5, and the E1a is regulated by a-fetoprotein (AFP) promoter and expresses superoxide dismutase (Mn-SOD). The AD55-Mn-SOD exhibited dramatical anti-cancer effects in HCC cells by activating the caspase apoptotic pathway (72). Furthermore, there were several studies reported that Mn-SOD gene therapy showed a novel anti-tumor effect also in pancreatic and colorectal cancer (71, 73).
However, the injection of AD55-MnSOD cannot achieve the complete inhibition of tumor cell growth, whether in tumor cells or at the animal level. Thus, it is necessary to explore the combination of this with other therapeutic strategies, for example, dual-gene-viral therapy, conventional chemotherapy, or immunotherapy (72).
Modulation of the Host Immune Response Triggered by Oncolytic Adenovirus Therapeutics
Despite its increasing advantages, gene-targeted oncolytic virus therapy is not popularized. This is because the current study found that gene monotherapy based on an oncolytic adenovirus is insufficient for tumor eradication. That the oncolytic adenovirus limited anti-tumor efficacy is attributed to several reasons. First, the majority of the human population had anti-adenovirus immunity, which significantly hampers the oncolytic adenovirus efficacy by causing rapid virus clearance after systemic administration (74). Second, the virus-mediated inflammatory response may cause severe complications that impairs safety (75). Third, the viral antigens might be presented by target cells after virus transfection and may ultimately induce a cytotoxic cellular response (76). In short, the adenovirus-triggered host immune response is one of the determining factors for its anti-cancer efficacy.
As far as virus-mediated immune responses are concerned, they include both host anti-virus and anti-tumor immune responses. The virus-based immunotherapy is the delivery of virus vectors harboring immune stimulatory factors to alleviate anti-virus immune responses as well as boost anti-tumor immunity in the tumor milieu.
Anti-virus Immune Response
Viral vectors could trigger a host anti-virus immune response, which intensely limits their applicability in several ways. First, viral vectors stimulate the expression of neutralizing antibodies (NAbs) against structural components due to their intrinsic immunogenicity (77, 78). The Pre-existing NAbs dramatically inhibit adenoviral vectors efficacy, and this leads to therapy termination. Second, intravenously administered adenoviruses are rapidly cleared by the mononuclear phagocytic system (MPS) in the liver and spleen (4).
To tackle this problem, multiple strategies, such as switching Ad serotype, fiber-nob modification, and constructing a hybrid vector system combining viral and non-viral carriers, are obliged to avoid virus-mediated immune responses Among them, serotype switching is the least reliable because each type of Ads has multiple surface-exposed capsid proteins containing neutralizing epitopes (79). An increasing amount of studies are committed to constructing a hybrid vector system with non-genetic modifications. The aim of the hybrid vector system is to construct adenoviruses “invisible” to immunogenic effector cells and components to thereby prolong blood circulation time and reduce immunogenicity (80), for instance, Polymeric adenoviruses engineered chemically or physically with numerous polymers coating on the virus surface. Commonly used chemically conjugated polymers, like poly(ethylene) glycol (PEG) (81) and the arginine-grafted bioreducible polymer (ABP) (82) have higher serum stability, while physically engineered polymers have better transduction efficiency. Oncolytic adenoviruses coated by polymers through electronic interaction are easy to manipulate since the cationic polymers with anionic adenovirus can form Ad/polymer complexes without a chemical reaction. Furthermore, it had been demonstrated that net cationic surface-charged Ads increase virus cellular uptake and transgene expression (83). However, this character causes non-specific viral uptake that might result in an unwanted side effect (80). Chen et al. engineered the oncolytic adenovirus PLC-ZD55-IL-24 and were intended to combine the advantages of both. The cationic calcium phosphate, which had been demonstrated to induce adenovirus cellular uptake and viral gene expression (84), was coated on an ionic virus capsid by electronic interaction. Then, to remedy the limitation of cationic polymers that lack specificity, the virus surface is stabilized by a neutrally charged PEG via Dioleoylphosphatydicacid (DOPA)er. Not only the negligible immune response was observed following the systemic administration of PLC-ZD55-IL-24 but it showed an efficient tumor-targeting ability and anti-tumor effect against HCC (85).
Anti-tumor Immune Response
Interestingly, immunosuppressed patients generally respond better to oncolytic virus therapy than those with an intact immune system and that this higher oncolytic activity is often associated with unacceptable toxicity. However, suppression of the adaptive immune response is a double-edged sword. To our knowledge, tumor immune evasion is the main obstacle restricting anti-tumor therapies. Tumor cells escape from immune responses in various ways, including through the secretion of immune-suppressing cytokines, the deficient expression of immune-modulating cytokines, and the recruitment of immune-inhibitory cells. Oncolytic adenoviruses provide a new strategy that combine tumor-debulking activity and the anti-tumor immune response. This combination therapy not only facilitates virus anti-tumor activity in the primary cancer milieu, but it also targets the metastatic lesion by activating the anti-tumor immune response. In order to modulate adaptive anti-tumor immunity, immune stimulatory, and/or co-stimulatory cytokines expressing Ads have been investigated in HCC immunotherapy.
IL-24 is one of the most potent immune-stimulatory cytokines and is a member of the IL-10 class-II cytokine family. In addition to modulating the immune response, IL-24 also showed anti-proliferative effects and induced cell apoptosis even with the genetic defects including the alteration of p53, Rb, and p16/INK4a (86, 87). Its anti-proliferative effect had been found in variety of cancer types, such as ovarian cancer (88, 89), lung carcinoma (90, 91), breast cancer (92), pancreatic cancer (93), glioma (94), prostate cancer (95), and colon cancer (96). SG600-IL24 is IL-24 expressing an oncolytic adenovirus generated based on the ZD55 backbone with a telomerase reverse transcriptase promoter (TERTp) that controls the E1gene and Hypoxia regulatory elements (HRE) that control the E1a and E1b gene (43). Xue et al. had studied the ability and function of SG600-IL24 to express IL-24 in HCC cell lines. Their study showed that SG600-IL24 can selectively suppress the proliferation and induce apoptosis of HCC cell lines in vitro but not normal liver cell line L02 in a p53-independent manner (97).
Liu et al. had developed the Cancer-targeting dual gene-viro-therapy (CTGVT-DG) strategy, which combines two tumor-suppressor genes that may have a compensative or synergetic effect (98). They investigated the combined therapy with IL-24 and the tumor necrosis factor-related apoptosis-inducing ligand (TRAIL), which was considered as a promising anti-tumor agent expressed in two Ads, respectively (99). Their study showed that a combination of two anti-tumor genes (IL-24 with TRAIL) may be a promising strategy for gene-viro therapy, which exhibits a synergistic anti-tumor effect (98). Furthermore, the combination therapy of Ad-ΔB/IL-12 with Ad-ΔB/TRAIL exhibits an enhanced anti-tumor immune response due to IL-12 being able to upregulate the TRAIL expression of NK cells, resulting in IFN-γ-dependent NK cell-related tumor metastasis inhibition (45, 100). Co-therapy with IL-12 and TRAIN complements TRAIL mono therapy poor pharmacokinetic property and induce HCC cells sensitivity to TRAIL's apoptotic effect (101). In addition, the enhanced anti-tumor efficacy of SG600-IL24 was observed in combination with IFN-α (102).
However, it had been found that some tumor cells over-expressed anti-apoptotic protein Bcl-2 and antagonized the IL-24 function (44). Thus, to recover IL-24 pro-apoptotic efficacy, Lou et al. constructed an AdCN205-IL-24-miR-34a that expressed both IL-24 and miRNA-34a (103). Previous studies demonstrated that miRNA-34a directly regulates the Bcl-2 (104). Significant induced tumor suppression and reduced expression of Bcl-2 had been observed after AdCN205-IL-24-miR-34a infection in comparison with AdCN205-IL-24 or AdCN205-miR-34a alone in vitro and vivo. In conclusion, downregulation of Bcl-2 induced by miRNA-34a can overcome tumor cell resistance to IL-24 and enhanced its anti-tumor effect (103).
In addition to IL-24 and IL-12, Sun et al. investigated whether recombinant adenoviruses expressing IL-2 (rAd-IL-2) as a gene immunotherapy agent could optimize the prognosis of HCC patients (53). IL-2 treatment was the first immunotherapy approved by the US Food and Drug Administration for use in melanomas (105). Recently, it had been demonstrated that oncolytic adenoviruses express interleukin-2 (IL-2), and the tumor necrosis factor alpha (TNF-a) can achieve an anti-tumor immunomodulatory effect similar to lymphodepletion. Importantly, using an oncolytic adenovirus is much safer than Lymphodepleting preconditioning with high-dose chemotherapy (106). According to Sun et al.'s study, rAd-IL-2 exhibits a significant induced anti-tumor immune response by recruiting CD4+ and CD8+T cells, increasing the interferon-γ release, and stimulating cytotoxic T lymphocyte responses in the HCC tumor model (53).
Oncolytic Adenovirus in Pre-Clinical Studies
Thanks to the advantages of oncolytic adenoviruses, a number of pre-clinical studies have been conducted on HCC treatment. As early as 2006, it was reported that improved recurrence-free survival and the overall survival had shown in advanced HCC patients receiving adjuvant ADV-TK (adenovirus vector expressing herpes simplex virus thymidine kinase) Gene Therapy after liver transplantation, as opposed to those who received liver transplantations alone (107). The feasibility and safety of intra-tumoral administration of an adenoviral vector encoding for HSV-TK were assessed in phase 1 clinical trials in HCC patients (108). Recently, the preliminary results from the phase 2 clinic trial declared that the double-dose adenovirus-mediated adjuvant therapy improved the outcome of liver transplantation in patients with advanced HCC (109). The other ongoing clinical trials are detailed in Table 1. Besides the anti-tumor properties of the oncolytic adenovirus itself, its combination with other agents has been studied and found to increase the cancer-killing efficacy. For example, the synergistic efficacy of a chemodrug, such as 5-FU, Gemcitabine, doxorubicin, and Paclitaxel (PTX), used in combination with an oncolytic adenovirus has been recorded (110). A phase 3 clinic trial of Hepatic artery infusion chemotherapy (HAIC) of FOLFOX in combination with oncolytic adenovirus in HCC treatment is under recruitment (NCT03780049). Furthermore, the compatibility of oncolytic adenoviruses with immune stimulator factors, like IL-2,-12, and−24, and immune checkpoint inhibitors, like (PD-1)/CD137L (111), has been studied recently. The combination of the anti-PD-1 antibody (Pembrolizumab) and Ad5-Δ24-RGD (DNX-2401) is being evaluated in a Phase II clinical trial for the treatment of glioblastoma (NCT02798406). There is no data for the use in HCC at present.
Discussion
Gene-targeted oncolytic virus therapy (GTOVT) had innovated a brand-new area in cancer treatment that provides the possibility of targeting all cancer types by designing viral vectors. The adenovirus is the most promising agent used in GTOVT due to several good characteristics. Theoretically, gene-armed viral vectors could target all type of cancers, including both solid tumors and hematological malignant ones. In spite of clinical trials having confirmed the safety of viral agents in clinical use, oncolytic adenoviruses have not yet been used in clinic. This is due oncolytic adenoviruses being strictly inhibited during systemic delivery in vivo, which hampers their effectiveness. To overcome this shortcoming, bioengineering, molecular, and immunological approaches have been considered. For example, decorated oncolytic adenovirus vectors are produced by genetic &/or non-genetic (capsid modification by polymers, like PEG, etc.) tools to avoid neutralization by anti-virus antigens and serum components and to prevent sequestration by MPS in the liver and spleen, which is the other major reason for virus clearance from circulation. Furthermore, high interstitial pressure and a tight stromal extracellular matrix (ECM) blocks the virus spread into the tumor foci. These problems are partially solved by the oncolytic adenovirus being armed with functional genes, such as Hyaluronidase or Metalloproteinase, or delivered by MSCs as a virus carrier for inducing virus uptake. It should be noted that some transgene expression may result in the loss of viral fitness. All these stations result in premature the clearance of the oncolytic adenovirus through a substantial immune response (112). Therefore, the oncolytic Ad-based immunotherapeutics have attracted increased interests recently due to their ability to modulate the anti-tumor immune response and evade the anti-virus immune response. However, finding the balance between the anti-tumor immune response and anti-virus immune response is a key issue that remains unsolved.
Author Contributions
MA: manuscript drafting and figure design. TW and RW: manuscript editing and revision. XC: literature review. YL and CT: clinical trials review and table design. RW: research team management and oversight.
Funding
The work was supported by grants from the National Natural Science Foundation of China (No. 81772995 and 81472266) and the Excellent Youth Foundation of Jiangsu Province, China, BK20140032 (to RW). We apologize to all colleagues whose relevant contributions could not be cited due to space limitations.
Conflict of Interest
The authors declare that the research was conducted in the absence of any commercial or financial relationships that could be construed as a potential conflict of interest.
References
1. Ma B, Wang Y, Zhou X, Huang P, Zhang R, Liu T, et al. Synergistic suppression effect on tumor growth of hepatocellular carcinoma by combining oncolytic adenovirus carrying XAF1 with cisplatin. J Cancer Res Clin Oncol. (2015) 141:419–29. doi: 10.1007/s00432-014-1835-8
2. De Pace N. Sulla scomparsa di un enorme cancro vegetante del callo dell'utero senza cura chirurgica. Ginecologia. (1912) 1912:82–8.
3. Ungerechts G, Engeland CE, Buchholz CJ, Eberle J, Fechner H, Geletneky K, et al. Virotherapy research in Germany: from engineering to translation. Hum Gene Ther. (2017) 28:800–19. doi: 10.1089/hum.2017.138
4. Russell SJ, Peng KW, Bell JC. Oncolytic virotherapy. Nat Biotechnol. (2012) 30:658–70. doi: 10.1038/nbt.2287
5. Arnberg N. Adenovirus receptors: implications for targeting of viral vectors. Trends Pharmacol Sci. (2012) 33:442–8. doi: 10.1016/j.tips.2012.04.005
6. Panek WK, Kane JR, Young JS, Rashidi A, Kim JW, Kanojia D, et al. Hitting the nail on the head: combining oncolytic adenovirus-mediated virotherapy and immunomodulation for the treatment of glioma. Oncotarget. (2017) 8:89391–405. doi: 10.18632/oncotarget.20810
7. Marelli G, Howells A, Lemoine NR, Wang Y. Oncolytic viral therapy and the immune system: a double-edged sword against cancer. Front Immunol. (2018) 9:866. doi: 10.3389/fimmu.2018.00866
8. Hamid O, Hoffner B, Gasal E, Hong J, Carvajal RD. Oncolytic immunotherapy: unlocking the potential of viruses to help target cancer. Cancer Immunol Immunother. (2017) 66:1249–64. doi: 10.1007/s00262-017-2025-8
9. Provenzano PP, Hingorani SR. Hyaluronan, fluid pressure, and stromal resistance in pancreas cancer. Br J Cancer. (2013) 108:1–8. doi: 10.1038/bjc.2012.569
10. Nattress CB, Halldén G. Advances in oncolytic adenovirus therapy for pancreatic cancer. Cancer Lett. (2018) 434:56–69. doi: 10.1016/j.canlet.2018.07.006
11. Li S, Ou M, Wang G, Tang L. Application of conditionally replicating adenoviruses in tumor early diagnosis technology, gene-radiation therapy and chemotherapy. Appl Microbiol Biotechnol. (2016) 100:8325–35. doi: 10.1007/s00253-016-7806-z
12. Oliva S, Gambella M, Boccadoro M, Bringhen S. Systemic virotherapy for multiple myeloma. Expert Opin Biol Ther. (2017) 17:1375–87. doi: 10.1080/14712598.2017.1364359
13. Zhang Y, Bergelson JM. Adenovirus receptors. J Virol. (2005) 79:12125–31. doi: 10.1128/JVI.79.19.12125-12131.2005
14. Cohen CJ, Shieh JT, Pickles RJ, Okegawa T, Hsieh JT, Bergelson JM. The coxsackievirus and adenovirus receptor is a transmembrane component of the tight junction. Proc Natl Acad Sci USA. (2001) 98:15191–6. doi: 10.1073/pnas.261452898
15. Baker AT, Aguirre-Hernández C, Halldén G, Parker AL. Designer oncolytic adenovirus: coming of age. Cancers (Basel). (2018) 10:E201. doi: 10.3390/cancers10060201
16. Berk AJ. Recent lessons in gene expression, cell cycle control, and cell biology from adenovirus. Oncogene. (2005) 24:7673–85. doi: 10.1038/sj.onc.1209040
17. Zhang W, Cai R, Luo J, Wang Y, Cui Q, Wei X, et al. The oncolytic adenovirus targeting to TERT and RB pathway induced specific and potent anti-tumor efficacy in vitro and in vivo for hepatocellular carcinoma. Cancer Biol Ther. (2007) 6:1726–32. doi: 10.4161/cbt.6.11.4831
18. Rao L, Debbas M, Sabbatini P, Hockenbery D, Korsmeyer S, White E. The adenovirus E1A proteins induce apoptosis, which is inhibited by the E1B 19-kDa and Bcl-2 proteins. Proc Natl Acad Sci USA. (1992) 89:7742–6. doi: 10.1073/pnas.89.16.7742
19. Boyd JM, Malstrom S, Subramanian T, Venkatesh LK, Schaeper U, Elangovan B, et al. Adenovirus E1B 19 kDa and Bcl-2 proteins interact with a common set of cellular proteins. Cell. (1994) 79:341–51. doi: 10.1016/0092-8674(94)90202-X
20. Chiou SK, Tseng CC, Rao L, White E. Functional complementation of the adenovirus E1B 19-kilodalton protein with Bcl-2 in the inhibition of apoptosis in infected cells. J Virol. (1994) 68:6553–66.
21. Boulakia CA, Chen G, Ng FW, Teodoro JG, Branton PE, Nicholson DW, et al. Bcl-2 and adenovirus E1B 19 kDA protein prevent E1A-induced processing of CPP32 and cleavage of poly(ADP-ribose) polymerase. Oncogene. (1996) 12:529–35.
22. Han J, Modha D, White E. Interaction of E1B 19K with Bax is required to block Bax-induced loss of mitochondrial membrane potential and apoptosis. Oncogene. (1998) 17:2993–3005. doi: 10.1038/sj.onc.1202215
23. Kim J, Cho JY, Kim JH, Jung KC, Yun CO. Evaluation of E1B gene-attenuated replicating adenoviruses for cancer gene therapy. Cancer Gene Ther. (2002) 9:725–36. doi: 10.1038/sj.cgt.7700494
24. Kirn D, Hermiston T. McCormick F. ONYX-015: clinical data are encouraging. Nat Med. (1998) 4:1341–2. doi: 10.1038/3902
25. Khuri FR, Nemunaitis J, Ganly I, Arseneau J, Tannock IF, Romel L, et al. A controlled trial of intratumoral ONYX-015, a selectively-replicating adenovirus, in combination with cisplatin and 5-fluorouracil in patients with recurrent head and neck cancer. Nat Med. (2000) 6:879–85. doi: 10.1038/78638
26. McCormick F. ONYX-015 selectivity and the p14ARF pathway. Oncogene. (2000) 19:6670–2. doi: 10.1038/sj.onc.1204096
27. McCormick F. Interactions between adenovirus proteins and the p53 pathway: the development of ONYX-015. Semin Cancer Biol. (2000) 10:453–9. doi: 10.1006/scbi.2000.0336
28. O'Shea CC, Johnson L, Bagus B, Choi S, Nicholas C, Shen A, et al. Late viral RNA export, rather than p53 inactivation, determines ONYX-015 tumor selectivity. Cancer Cell. (2004) 6:611–23. doi: 10.1016/j.ccr.2004.11.012
29. Sharon D, Schümann M, MacLeod S, McPherson R, Chaurasiya S, Shaw A, et al. 2-aminopurine enhances the oncolytic activity of an E1b-deleted adenovirus in hepatocellular carcinoma cells. PLoS ONE. (2013) 8:e65222. doi: 10.1371/journal.pone.0065222
30. Li Y, Yu DC, Chen Y, Amin P, Zhang H, Nguyen N, et al. A hepatocellular carcinoma-specific adenovirus variant, CV890, eliminates distant human liver tumors in combination with doxorubicin. Cancer Res. (2001) 61:6428–36.
31. Zhang W, Gao Y, Li P, Shi Z, Guo T, Li F, et al. VGLL4 functions as a new tumor suppressor in lung cancer by negatively regulating the YAP-TEAD transcriptional complex. Cell Res. (2014) 24:331–43. doi: 10.1038/cr.2014.10
32. Lv SQ, Ye ZL, Liu PY, Huang Y, Li LF, Liu H, et al. 11R-P53 and GM-CSF expressing oncolytic adenovirus target cancer stem cells with enhanced synergistic activity. J Cancer. (2017) 8:199–206. doi: 10.7150/jca.16406
33. Zhang J, Lai W, Li Q, Yu Y, Jin J, Guo W, et al. A novel oncolytic adenovirus targeting Wnt signaling effectively inhibits cancer-stem like cell growth via metastasis, apoptosis and autophagy in HCC models. Biochem Biophys Res Commun. (2017) 491:469–77. doi: 10.1016/j.bbrc.2017.07.041
34. Yuan X, Zhang Q, Li Z, Zhang X, Bao S, Fan D, et al. Mesenchymal stem cells deliver and release conditionally replicative adenovirus depending on hepatic differentiation to eliminate hepatocellular carcinoma cells specifically. Cancer Lett. (2016) 381:85–95. doi: 10.1016/j.canlet.2016.07.019
35. Qian CY, Wang KL, Fang FF, Gu W, Huang F, Wang FZ, et al. Triple-controlled oncolytic adenovirus expressing melittin to exert inhibitory efficacy on hepatocellular carcinoma. Int J Clin Exp Pathol. (2015) 8:10403–11.
36. Gao Y, Zhu Y, Huang X, Ai K, Zheng Q, Yuan Z. Gene therapy targeting hepatocellular carcinoma by a dual-regulated oncolytic adenovirus harboring the focal adhesion kinase shRNA. Int J Oncol. (2015) 47:668–78. doi: 10.3892/ijo.2015.3047
37. Wang Y, Liu T, Huang P, Zhao H, Zhang R, Ma B, et al. A novel Golgi protein (GOLPH2)-regulated oncolytic adenovirus exhibits potent antitumor efficacy in hepatocellular carcinoma. Oncotarget. (2015) 6:13564–78. doi: 10.18632/oncotarget.3769
38. Larsen L, Röpke C. Suppressors of cytokine signalling: SOCS. APMIS. (2002) 110:833–44. doi: 10.1034/j.1600-0463.2002.1101201.x
39. Li K, Li X, Wu Z, Zheng L, Cui Y, Wang J, et al. Adenovirus encoding XAF-1 and TNFα in the same open reading frame efficiently inhibits hepatocellular cancer cells. Mol Med Rep. (2016) 13:5169–76. doi: 10.3892/mmr.2016.5193
40. Irani K, Xia Y, Zweier JL, Sollott SJ, Der CJ, Fearon ER, et al. Mitogenic signaling mediated by oxidants in Ras-transformed fibroblasts. Science. (1997) 275:1649–52. doi: 10.1126/science.275.5306.1649
41. He G, Lei W, Wang S, Xiao R, Guo K, Xia Y, et al. Overexpression of tumor suppressor TSLC1 by a survivin-regulated oncolytic adenovirus significantly inhibits hepatocellular carcinoma growth. J Cancer Res Clin Oncol. (2012) 138:657–70. doi: 10.1007/s00432-011-1138-2
42. Shin EC, Seong YR, Kim CH, Kim H, Ahn YS, Kim K, et al. Human hepatocellular carcinoma cells resist to TRAIL-induced apoptosis, and the resistance is abolished by cisplatin. Exp Mol Med. (2002) 34:114–22. doi: 10.1038/emm.2002.17
43. Xiao CW, Xue XB, Zhang H, Gao W, Yu Y, Chen K, et al. Oncolytic adenovirus-mediated MDA-7/IL-24 overexpression enhances antitumor activity in hepatocellular carcinoma cell lines. Hepatobiliary Pancreat Dis Int. (2010) 9:615–21.
44. Lebedeva IV, Sarkar D, Su ZZ, Kitada S, Dent P, Stein CA, et al. Bcl-2 and Bcl-x(L) differentially protect human prostate cancer cells from induction of apoptosis by melanoma differentiation associated gene-7, mda-7/IL-24. Oncogene. (2003) 22:8758–73. doi: 10.1038/sj.onc.1206891
45. Smyth MJ, Cretney E, Takeda K, Wiltrout RH, Sedger LM, Kayagaki N, et al. Tumor necrosis factor-related apoptosis-inducing ligand (TRAIL) contributes to interferon γ-dependent natural killer cell protection from tumor metastasis. J Exp Med. (2001) 193:661–70. doi: 10.1084/jem.193.6.661
46. Li J, Liu H, Li L, Wu H, Wang C, Yan Z, et al. The combination of an oxygen-dependent degradation domain-regulated adenovirus expressing the chemokine RANTES/CCL5 and NK-92 cells exerts enhanced antitumor activity in hepatocellular carcinoma. Oncol Rep. (2013) 29:895–902. doi: 10.3892/or.2012.2217
47. Kim YH, Kim KT, Lee SJ, Hong SH, Moon JY, Yoon EK, et al. Image-aided suicide gene therapy utilizing multifunctional hTERT-targeting adenovirus for clinical translation in hepatocellular carcinoma. Theranostics. (2016) 6:357–68. doi: 10.7150/thno.13621
48. van der Poel HG, McCadden J, Verhaegh GW, Kruszewski M, Ferrer F, Schalken JA, et al. A novel method for the determination of basal gene expression of tissue-specific promoters: an analysis of prostate-specific promoters. Cancer Gene Ther. (2001) 8:927–35. doi: 10.1038/sj.cgt.7700385
49. Stepanenko AA, Chekhonin VP. Tropism and transduction of oncolytic adenovirus 5 vectors in cancer therapy: focus on fiber chimerism and mosaicism, hexon and pIX. Virus Res. (2018) 257:40–51. doi: 10.1016/j.virusres.2018.08.012
50. Haralambieva I, Iankov I, Hasegawa K, Harvey M, Russell SJ, Peng KW. Engineering oncolytic measles virus to circumvent the intracellular innate immune response. Mol Ther. (2007) 15:588–97. doi: 10.1038/sj.mt.6300076
51. Liu X, Xu J, Wang S, Yu X, Kou B, Chai M, et al. Synergistic inhibitory effects on hepatocellular carcinoma with recombinant human adenovirus Aspp2 and oxaliplatin via p53-independent pathway in vitro and in vivo. Int J Oncol. (2017) 51:1291–9. doi: 10.3892/ijo.2017.4105
52. Sawahara H, Shiraha H, Uchida D, Kato H, Kato R, Oyama A, et al. Promising therapeutic efficacy of a novel reduced expression in immortalized cells/dickkopf-3 expressing adenoviral vector for hepatocellular carcinoma. J Gastroenterol Hepatol. (2017) 32:1769–77. doi: 10.1111/jgh.13757
53. Sun Y, Wu H, Chen G, Huang X, Shan Y, Shi H, et al. Genetically engineered recombinant adenovirus expressing interleukin2 for hepatocellular carcinoma therapy. Mol Med Rep. (2018) 17:300–6. doi: 10.3892/mmr.2017.7922
54. Zhang Q, Yuan XF, Lu Y, Li ZZ, Bao SQ, Zhang XL, et al. Surface expression of anti-CD3scfv stimulates locoregional immunotherapy against hepatocellular carcinoma depending on the E1A-engineered human umbilical cord mesenchymal stem cells. Int J Cancer. (2017) 141:1445–57. doi: 10.1002/ijc.30846
55. Xie W, Hao J, Zhang K, Fang X, Liu X. Adenovirus armed with VGLL4 selectively kills hepatocellular carcinoma with G2/M phase arrest and apoptosis promotion. Biochem Biophys Res Commun. (2018) 503:2758–63. doi: 10.1016/j.bbrc.2018.08.036
56. Jin HS, Park HS, Shin JH, Kim DH, Jun SH, Lee CJ, et al. A novel inhibitor of apoptosis protein (IAP)-interacting protein, Vestigial-like (Vgl)-4, counteracts apoptosis-inhibitory function of IAPs by nuclear sequestration. Biochem Biophys Res Commun. (2011) 412:454–9. doi: 10.1016/j.bbrc.2011.07.117
57. Jiao S, Wang H, Shi Z, Dong A, Zhang W, Song X, et al. A peptide mimicking VGLL4 function acts as a YAP antagonist therapy against gastric cancer. Cancer Cell. (2014) 25:166–80. doi: 10.1016/j.ccr.2014.01.010
58. Zhang Y, Shen H, Withers HG, Yang N, Denson KE, Mussell AL, et al. VGLL4 selectively represses YAP-dependent gene induction and tumorigenic phenotypes in breast cancer. Sci Rep. (2017) 7:6190. doi: 10.1038/s41598-017-06227-7
59. Zhu L, Cheng X, Ding Y, Shi J, Jin H, Wang H, et al. Bone marrow-derived myofibroblasts promote colon tumorigenesis through the IL-6/JAK2/STAT3 pathway. Cancer Lett. (2014) 343:80–9. doi: 10.1016/j.canlet.2013.09.017
60. Plenchette S, Cheung HH, Fong WG, LaCasse EC, Korneluk RG. The role of XAF1 in cancer. Curr Opin Investig Drugs. (2007) 8:469–76.
61. Szymczak AL, Workman CJ, Wang Y, Vignali KM, Dilioglou S, Vanin EF, et al. Correction of multi-gene deficiency in vivo using a single 'self-cleaving' 2A peptide-based retroviral vector. Nat Biotechnol. (2004) 22:589–94. doi: 10.1038/nbt957
62. Yamanaka T, Shiraki K, Sugimoto K, Ito T, Fujikawa K, Ito M, et al. Chemotherapeutic agents augment TRAIL-induced apoptosis in human hepatocellular carcinoma cell lines. Hepatology. (2000) 32:482–90. doi: 10.1053/jhep.2000.16266
63. Suzuki Y, Nakabayashi Y, Nakata K, Reed JC, Takahashi R. X-linked inhibitor of apoptosis protein (XIAP) inhibits caspase-3 and−7 in distinct modes. J Biol Chem. (2001) 276:27058–63. doi: 10.1074/jbc.M102415200
64. Wei RC, Cao X, Gui JH, Zhou XM, Zhong D, Yan QL, et al. Augmenting the antitumor effect of TRAIL by SOCS3 with double-regulated replicating oncolytic adenovirus in hepatocellular carcinoma. Hum Gene Ther. (2011) 22:1109–19. doi: 10.1089/hum.2010.219
65. Liu L, Li W, Wei X, Cui Q, Lou W, Wang G, et al. Potent antitumor activity of oncolytic adenovirus-mediated SOCS1 for hepatocellular carcinoma. Gene Ther. (2013) 20:84–92. doi: 10.1038/gt.2012.4
66. Tazawa H, Kagawa S, Fujiwara T. Advances in adenovirus-mediated p53 cancer gene therapy. Expert Opin Biol Ther. (2013) 13:1569–83. doi: 10.1517/14712598.2013.845662
67. Li Y, Li B, Li CJ, Li LJ. Key points of basic theories and clinical practice in rAd-p53 (Gendicine) gene therapy for solid malignant tumors. Expert Opin Biol Ther. (2015) 15:437–54. doi: 10.1517/14712598.2015.990882
68. Sullivan A, Lu X. ASPP: a new family of oncogenes and tumour suppressor genes. Br J Cancer. (2007) 96:196–200. doi: 10.1038/sj.bjc.6603525
69. Liu J, Li W, Deng M, Liu D, Ma Q, Feng X. Immunohistochemical determination of p53 protein overexpression for predicting p53 gene mutations in hepatocellular carcinoma: a meta-analysis. PLoS ONE. (2016) 11:e0159636. doi: 10.1371/journal.pone.0159636
70. Shi Y, Han Y, Xie F, Wang A, Feng X, Li N, et al. ASPP2 enhances Oxaliplatin (L-OHP)-induced colorectal cancer cell apoptosis in a p53-independent manner by inhibiting cell autophagy. J Cell Mol Med. (2015) 19:535–43. doi: 10.1111/jcmm.12435
71. Weydert C, Roling B, Liu J, Hinkhouse MM, Ritchie JM, Oberley LW, et al. Suppression of the malignant phenotype in human pancreatic cancer cells by the overexpression of manganese superoxide dismutase. Mol Cancer Ther. (2003) 2:361–9.
72. Huang F, Ma B, Wang Y, Xiao R, Kong Y, Zhou X, et al. Targeting gene-virus-mediated manganese superoxide dismutase effectively suppresses tumor growth in hepatocellular carcinoma in vitro and in vivo. Cancer Biother Radiopharm. (2014) 29:403–11. doi: 10.1089/cbr.2014.1642
73. Behrend L, Mohr A, Dick T, Zwacka RM. Manganese superoxide dismutase induces p53-dependent senescence in colorectal cancer cells. Mol Cell Biol. (2005) 25:7758–69. doi: 10.1128/MCB.25.17.7758-7769.2005
74. Nwanegbo E, Vardas E, Gao W, Whittle H, Sun H, Rowe D, et al. Prevalence of neutralizing antibodies to adenoviral serotypes 5 and 35 in the adult populations of The Gambia, South Africa, and the United States. Clin Diagn Lab Immunol. (2004) 11:351–7. doi: 10.1128/CDLI.11.2.351-357.2004
75. Raper SE, Chirmule N, Lee FS, Wivel NA, Bagg A, Gao GP, et al. Fatal systemic inflammatory response syndrome in a ornithine transcarbamylase deficient patient following adenoviral gene transfer. Mol Genet Metab. (2003) 80:148–58. doi: 10.1016/j.ymgme.2003.08.016
76. Yang Y, Jooss KU, Su Q, Ertl HC, Wilson JM. Immune responses to viral antigens versus transgene product in the elimination of recombinant adenovirus-infected hepatocytes in vivo. Gene Ther. (1996) 3:137–44.
77. Morral N, O'Neal W, Rice K, Leland M, Kaplan J, Piedra PA, et al. Administration of helper-dependent adenoviral vectors and sequential delivery of different vector serotype for long-term liver-directed gene transfer in baboons. Proc Natl Acad Sci USA. (1999) 96:12816–21. doi: 10.1073/pnas.96.22.12816
78. Tomita K, Sakurai F, Tachibana M, Mizuguchi H. Correlation between adenovirus-neutralizing antibody titer and adenovirus vector-mediated transduction efficiency following intratumoral injection. Anticancer Res. (2012) 32:1145–52.
79. Kaufmann JK, Nettelbeck DM. Virus chimeras for gene therapy, vaccination, and oncolysis: adenoviruses and beyond. Trends Mol Med. (2012) 18:365–76. doi: 10.1016/j.molmed.2012.04.008
80. Choi JW, Lee YS, Yun CO, Kim SW. Polymeric oncolytic adenovirus for cancer gene therapy. J Control Release. (2015) 219:181–91. doi: 10.1016/j.jconrel.2015.10.009
81. O'Riordan CR, Lachapelle A, Delgado C, Parkes V, Wadsworth SC, Smith AE, et al. PEGylation of adenovirus with retention of infectivity and protection from neutralizing antibody in vitro and in vivo. Hum Gene Ther. (1999) 10:1349–58. doi: 10.1089/10430349950018021
82. Kim PH, Kim J, Kim TI, Nam HY, Yockman JW, Kim M, et al. Bioreducible polymer-conjugated oncolytic adenovirus for hepatoma-specific therapy via systemic administration. Biomaterials. (2011) 32:9328–42. doi: 10.1016/j.biomaterials.2011.08.066
83. Pack DW, Hoffman AS, Pun S, Stayton PS. Design and development of polymers for gene delivery. Nat Rev Drug Discov. (2005) 4:581–93. doi: 10.1038/nrd1775
84. Kelly EJ, Russell SJ. MicroRNAs and the regulation of vector tropism. Mol Ther. (2009) 17:409–16. doi: 10.1038/mt.2008.288
85. Chen J, Gao P, Yuan S, Li R, Ni A, Chu L, et al. Oncolytic adenovirus complexes coated with lipids and calcium phosphate for cancer gene therapy. ACS Nano. (2016) 10:11548–60. doi: 10.1021/acsnano.6b06182
86. Huang EY, Madireddi MT, Gopalkrishnan RV, Leszczyniecka M, Su Z, Lebedeva IV, et al. Genomic structure, chromosomal localization and expression profile of a novel melanoma differentiation associated (mda-7) gene with cancer specific growth suppressing and apoptosis inducing properties. Oncogene. (2001) 20:7051–63. doi: 10.1038/sj.onc.1204897
87. Lebedeva IV, Su ZZ, Chang Y, Kitada S, Reed JC, Fisher PB. The cancer growth suppressing gene mda-7 induces apoptosis selectively in human melanoma cells. Oncogene. (2002) 21:708–18. doi: 10.1038/sj.onc.1205116
88. Gopalan B, Litvak A, Sharma S, Mhashilkar AM, Chada S, Ramesh R. Activation of the Fas-FasL signaling pathway by MDA-7/IL-24 kills human ovarian cancer cells. Cancer Res. (2005) 65:3017–24. doi: 10.1158/0008-5472.CAN-04-3758
89. Mahasreshti PJ, Kataram M, Wu H, Yalavarthy LP, Carey D, Fisher PB, et al. Ovarian cancer targeted adenoviral-mediated mda-7/IL-24 gene therapy. Gynecol Oncol. (2006) 100:521–32. doi: 10.1016/j.ygyno.2005.08.042
90. Kawabe S, Nishikawa T, Munshi A, Roth JA, Chada S, Meyn RE. Adenovirus-mediated mda-7 gene expression radiosensitizes non-small cell lung cancer cells via TP53-independent mechanisms. Mol Ther. (2002) 6:637–44. doi: 10.1006/mthe.2002.0714
91. Ramesh R, Ito I, Gopalan B, Saito Y, Mhashilkar AM, Chada S. Ectopic production of MDA-7/IL-24 inhibits invasion and migration of human lung cancer cells. Mol Ther. (2004) 9:510–8. doi: 10.1016/j.ymthe.2004.01.019
92. McKenzie T, Liu Y, Fanale M, Swisher SG, Chada S, Hunt KK. Combination therapy of Ad-mda7 and trastuzumab increases cell death in Her-2/neu-overexpressing breast cancer cells. Surgery. (2004) 136:437–42. doi: 10.1016/j.surg.2004.05.022
93. Lebedeva IV, Su ZZ, Sarkar D, Gopalkrishnan RV, Waxman S, Yacoub A, et al. Induction of reactive oxygen species renders mutant and wild-type K-ras pancreatic carcinoma cells susceptible to Ad.mda-7-induced apoptosis. Oncogene. (2005) 24:585–96. doi: 10.1038/sj.onc.1208183
94. Yacoub A, Mitchell C, Hong Y, Gopalkrishnan RV, Su ZZ, Gupta P, et al. MDA-7 regulates cell growth and radiosensitivity in vitro of primary (non-established) human glioma cells. Cancer Biol Ther. (2004) 3:739–51. doi: 10.4161/cbt.3.8.968
95. Lebedeva IV, Su ZZ, Sarkar D, Kitada S, Dent P, Waxman S, et al. Melanoma differentiation associated gene-7, mda-7/interleukin-24, induces apoptosis in prostate cancer cells by promoting mitochondrial dysfunction and inducing reactive oxygen species. Cancer Res. (2003) 63:8138–44.
96. Zhao L, Gu J, Dong A, Zhang Y, Zhong L, He L, et al. Potent antitumor activity of oncolytic adenovirus expressing mda-7/IL-24 for colorectal cancer. Hum Gene Ther. (2005) 16:845–58. doi: 10.1089/hum.2005.16.845
97. Xue XB, Xiao CW, Zhang H, Lu AG, Gao W, Zhou ZQ, et al. Oncolytic adenovirus SG600-IL24 selectively kills hepatocellular carcinoma cell lines. World J Gastroenterol. (2010) 16:4677–84. doi: 10.3748/wjg.v16.i37.4677
98. Liu X, Cao X, Wei R, Cai Y, Li H, Gui J, et al. Gene-viro-therapy targeting liver cancer by a dual-regulated oncolytic adenoviral vector harboring IL-24 and TRAIL. Cancer Gene Ther. (2012) 19:49–57. doi: 10.1038/cgt.2011.67
99. Wiley SR, Schooley K, Smolak PJ, Din WS, Huang CP, Nicholl JK, et al. Identification and characterization of a new member of the TNF family that induces apoptosis. Immunity. (1995) 3:673–82. doi: 10.1016/1074-7613(95)90057-8
100. El-Shemi AG, Ashshi AM, Na Y, Li Y, Basalamah M, Al-Allaf FA, et al. Combined therapy with oncolytic adenoviruses encoding TRAIL and IL-12 genes markedly suppressed human hepatocellular carcinoma both in vitro and in an orthotopic transplanted mouse model. J Exp Clin Cancer Res. (2016) 35:74. doi: 10.1186/s13046-016-0365-4
101. He SQ, Chen Y, Chen XP, Zhang WG, Wang HP, Zhang BX. Interleukin-12 enhanced tumor necrosis factor related apoptosis-inducing ligand TRAIL-induced apoptosis in human hepatocellular carcinoma by inhibiting expression of survivin. Zhonghua Wai Ke Za Zhi. (2003) 41:453–7.
102. Wang CJ, Xiao CW, You TG, Zheng YX, Gao W, Zhou ZQ, et al. Interferon-α enhances antitumor activities of oncolytic adenovirus-mediated IL-24 expression in hepatocellular carcinoma. Mol Cancer. (2012) 11:31. doi: 10.1186/1476-4598-11-31
103. Lou W, Chen Q, Ma L, Liu J, Yang Z, Shen J, et al. Oncolytic adenovirus co-expressing miRNA-34a and IL-24 induces superior antitumor activity in experimental tumor model. J Mol Med (Berl). (2013) 91:715–25. doi: 10.1007/s00109-012-0985-x
104. Zenz T, Mohr J, Eldering E, Kater AP, Bühler A, Kienle D, et al. miR-34a as part of the resistance network in chronic lymphocytic leukemia. Blood. (2009) 113:3801–8. doi: 10.1182/blood-2008-08-172254
105. Snyder A, Zamarin D, Wolchok JD. Immunotherapy of melanoma. Prog Tumor Res. (2015) 42:22–9. doi: 10.1159/000436998
106. Santos JM, Cervera-Carrascon V, Havunen R, Zafar S, Siurala M, Sorsa S, et al. Adenovirus coding for interleukin-2 and tumor necrosis factor alpha replaces lymphodepleting chemotherapy in adoptive T cell therapy. Mol Ther. (2018) 26:2243–54. doi: 10.1016/j.ymthe.2018.06.001
107. Li N, Zhou J, Weng D, Zhang C, Li L, Wang B, et al. Adjuvant adenovirus-mediated delivery of herpes simplex virus thymidine kinase administration improves outcome of liver transplantation in patients with advanced hepatocellular carcinoma. Clin Cancer Res. (2007) 13:5847–54. doi: 10.1158/1078-0432.CCR-07-0499
108. Sangro B, Mazzolini G, Ruiz M, Ruiz J, Quiroga J, Herrero I, et al. A phase I clinical trial of thymidine kinase-based gene therapy in advanced hepatocellular carcinoma. Cancer Gene Ther. (2010) 17:837–43. doi: 10.1038/cgt.2010.40
109. Zhu R, Weng D, Lu S, Lin D, Wang M, Chen D, et al. Double-dose adenovirus-mediated adjuvant gene therapy improves liver transplantation outcomes in patients with advanced hepatocellular carcinoma. Hum Gene Ther. (2018) 29:251–8. doi: 10.1089/hum.2017.114
110. Goradel NH, Mohajel N, Malekshahi ZV, Jahangiri S, Najafi M, Farhood B, et al. Oncolytic adenovirus: A tool for cancer therapy in combination with other therapeutic approaches. J Cell Physiol. (2019) 234:8636–46. doi: 10.1002/jcp.27850
111. Zhang Y, Zhang H, Wei M, Mou T, Shi T, Ma Y, et al. Recombinant adenovirus expressing a soluble fusion protein PD-1/CD137L subverts the suppression of CD8+ T cells in HCC. Mol Ther. (2019). doi: 10.1016/j.ymthe.2019.07.019. [Epub ahead of print].
Keywords: gene-targeted oncolytic viral therapy, adenovirus, HCC, immunotherapy, virus engineering
Citation: Abudoureyimu M, Lai Y, Tian C, Wang T, Wang R and Chu X (2019) Oncolytic Adenovirus—A Nova for Gene-Targeted Oncolytic Viral Therapy in HCC. Front. Oncol. 9:1182. doi: 10.3389/fonc.2019.01182
Received: 14 June 2019; Accepted: 21 October 2019;
Published: 08 November 2019.
Edited by:
Andrew Zloza, Rush University Medical Center, United StatesReviewed by:
Ning Wei, University of Pittsburgh, United StatesPraveen Bommareddy, Rutgers, The State University of New Jersey, United States
Copyright © 2019 Abudoureyimu, Lai, Tian, Wang, Wang and Chu. This is an open-access article distributed under the terms of the Creative Commons Attribution License (CC BY). The use, distribution or reproduction in other forums is permitted, provided the original author(s) and the copyright owner(s) are credited and that the original publication in this journal is cited, in accordance with accepted academic practice. No use, distribution or reproduction is permitted which does not comply with these terms.
*Correspondence: Rui Wang, d2FuZ3J1aTIxOEAxNjMuY29t