- Metabolic Disease Branch, National Institute of Diabetes and Digestive and Kidney Diseases, National Institutes of Health, Bethesda, MD, United States
Background: Epidemiological data reveal that treatment with lithium, a mood stabilizer, is associated with decreased incidence and mortality of certain cancer types, such as melanoma. Therefore, repositioning of lithium as an anticancer agent has emerged as a promising strategy in oncology. Since lithium affects the physiology of several endocrine tissues, the goal of this study was to analyze the role of lithium in the pathogenesis and treatment of tumors of the endocrine system.
Methods: The databases of PubMed, EMBASE, MEDLINE, were searched from January 1970 through February 2019 for articles including the keywords “lithium and”—“thyroid cancer,” “thyroid nodule,” “parathyroid adenoma,” “parathyroid carcinoma,” “pituitary adenoma,” “pituitary neuroendocrine tumor,” “neuroendocrine tumor,” “carcinoid,” “adrenal adenoma,” “adrenal carcinoma,” “pheochromocytoma/paraganglioma.” Preclinical in vitro and in vivo studies as well as case series, retrospective cohort studies and prospective trials were selected for the analysis.
Results: Treatment with lithium has been associated with a higher prevalence of thyroid enlargement, hypothyroidism and increased calcium levels due to parathyroid adenoma or hyperplasia, as one of the mechanisms of its action is to stimulate proliferation of normal follicular thyroid and parathyroid cells via activation of the Wnt signaling pathway. Supratherapeutic concentrations of lithium decrease the activity of glycogen synthase kinase-3β (GSK-3β), leading to cell cycle arrest in several in vitro cancer models including medullary thyroid cancer (TC), pheochromocytoma/paraganglioma and carcinoid. Growth inhibitory effects of lithium in vivo have been documented in medullary TC xenograft mouse models. Clinically, lithium has been used as an adjuvant agent to therapy with radioactive iodine (RAI), as it increases the residence time of RAI in TC.
Conclusion: Patients chronically treated with lithium need to be screened for hypothyroidism, goiter, and hyperparathyroidism, as the prevalence of these endocrine abnormalities is higher in lithium-treated patients than in the general population. The growth inhibitory effects of lithium in medullary TC, pheochromocytoma/paraganglioma and carcinoid were achieved with supratherapeutic concentrations of lithium thus limiting its translational perspective. Currently available clinical data on the efficacy of lithium in the therapy of endocrine tumors in human is limited and associated with conflicting results.
Introduction
Lithium (lithos for stone in Greek) was discovered in the nineteenth century and it's salt—lithium carbonate has been widely used to treat bipolar disorder (1). Interestingly, patients chronically exposed to lithium carbonate were also characterized by a lower incidence of Alzheimer disease (2). These clinical observations prompted an investigation of the pleiotropic effects of lithium carbonate in human physiology and pathology. One of the important findings was that lithium affects glycogen synthase kinase-3β (GSK-3β) signaling pathway. GSK-3β functions as an important regulatory kinase and its hyperactivity have been linked to several disorders such as Alzheimer disease, schizophrenia (3), diabetes (4), and cancer (5, 6). Therefore, the reposition of lithium as an inhibitor of GSK-3β has emerged as a potential tool in cancer treatment. This interest is further supported by functional studies documenting the effect of lithium on numerous other pathways, including the WNT/β-catenin pathway (7), activation of CREB (cAMP-response-element-binding protein), interaction with TORC1 (1, 8) immunomodulation of lymphokine-activated killer cells and its effects on cell metabolism (glycolysis) (9–11). All of these cellular processes play an important role in pathogenesis of tumors of the endocrine system (12–20). Most of the studies analyzing the effects of lithium in the management of cancers have been primarily focused on thyroid cancer. Besides endocrine tumors, there are few reports where lithium use was associated with growth inhibition of melanoma (9, 21), prostate (22), and hepatocellular carcinoma cells (23, 24). In a retrospective cohort study, lithium exposure was associated with reduced melanoma risk and mortality (25). In contrary to this, lithium exposure increased the incidence of renal tumors in the lithium-treated patients (26).
The therapy of a subset of endocrine cancers is very challenging and the goal of this review is to provide an overview of the potential utility of lithium in the management of endocrine tumors based on published pre-clinical and clinical data.
Lithium and Benign and Malignant Thyroid Nodules
Epidemiology
As the use of lithium for the manic state of the bipolar disorder increased, so did the appearance of goiters and hypothyroidism, suggesting a role of lithium in the synthesis and storage of thyroid hormones (27). The prevalence of goiter has been reported to be as common as 4–60% (28–33) and hypothyroidism was observed in up to 6–52% of patients taking lithium (28, 34–46) while its prevalence in general population varies between 4.8–28 and 4.6–9.5%, respectively (47–49). On the other hand, lithium can also cause damage to the thyroid cell with consequent signs and symptoms of thyroiditis (28, 50). Interestingly, lithium is concentrated by the thyroid at levels 3–4 times higher than in the circulation (51). Lithium was found to increase the intrathyroidal iodine content as well as to inhibit release of thyroid hormones from the thyroid into the circulation due to altered tubulin polymerization in thyrocytes (28, 52, 53). In fact, the latter property has been used to treat patients with hyperthyroidism. Similar mechanisms have been proposed to be utilized to extend the retention of radioactive iodine (RAI) within the thyroid gland to treat either benign toxic multinodular goiters, Graves' disease or differentiated thyroid cancer (54–60). The application of lithium as adjuvant therapy for metastatic thyroid cancer is particularly interesting in view of the fact that the incidence of differentiated thyroid cancer (DTC) is rising more rapidly than any other type of cancer and that RAI-non responsive metastatic DTC is characterized by a poor outcome (61, 62).
Mechanism of Lithium Action—Pre-clinical Evidence
Since therapy with lithium has been associated with a high prevalence of goiter, there have been several studies evaluating the growth stimulatory effects of lithium on normal follicular cells. In vivo studies in rat models indicated that exposure to lithium resulted in an increase in follicular diameter and a decrease in follicle cell height (63). Functional in vitro models revealed that Wnt/β-catenin signaling may be important in lithium-associated goiter, as lithium significantly increased human thyrocyte proliferation mediated by Wnt/β-catenin pathway (64–66). Wnt/ β-catenin pathway plays a crucial role in organ formation during embryonic development, stem cell renewal and cell survival (67, 68). Aberrant activation of this pathway has been seen in many cancers. This pathway can regulate multiple target genes which play important roles in various physiological processes like proliferation, transformation and differentiation (67, 68). Gilbert-Sirieix et al. documented that the Wnt/β-catenin pathway regulates expression of one of the most important transcription factors in the thyroid gland, thyroid-transcription factor 1 (TTF1), in the papillary thyroid cancer (PTC) cell line TPC1 and in patient-derived PTC tumors (20). The activation of Wnt signaling by lithium chloride at the concentration of 5 and 20 mM induced TTF-1 gene and protein expression, suggesting a role of lithium in tumor differentiation (20). This observation is particularly important in view of the fact that de-differentiation is often observed in thyroid cancer, during which tumors progressively lose the expression of thyroid-specific genes such as sodium-iodine symporter (NIS), paired-box gene 8 (PAX8) or thyroglobulin (Tg) (69). Dupain et al. observed that treatment with 20 mM lithium resulted in upregulation of thyroid-specific genes TTF1 and PAX8 in TPC1 cell line, but not in other cell lines (e.g., BHP 10-3, and ARO) (70). TTF-1 and PAX8 are thyroid-specific transcription factors and are crucial for thyroid development and function. These transcription factors have been known to regulate the expression of various thyroid specific genes which include Tg, NIS thyroid peroxidase (TPO), and thyrotropin receptor (TSHR) (71). The low levels of TTF-1 and loss of its nuclear localization in thyroid cancer have been associated with dedifferentiation and increased malignancy, whereas the role of PAX8 in thyroid cancer is still controversial (71, 72). The lithium-mediated induction in the expression of thyroid-specific transcription factors upon Wnt activation, suggest these transcription factors as downstream targets of Wnt/β-catenin pathway in thyroid cancer cells (Figure 1).
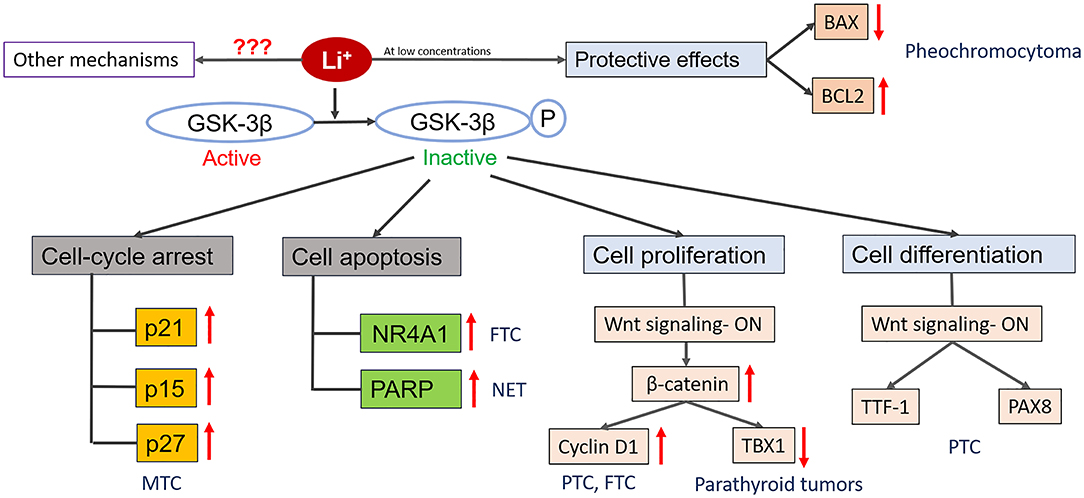
Figure 1. Diagrammatic representation of known mechanisms of action of lithium in endocrine cancers. Lithium treatment inactivates GSK-3β by phosphorylating it. Inactivation of GSK-3β can promote cell death by increasing the expression of cyclin-dependent kinase inhibitors (p21, p15, p27) that promotes cell cycle arrest and/or by increasing the expression of transcription factors (NR4A1) which induce expression of pro-apoptotic genes. Depending upon the cell types and concentration, lithium can also promote cellular proliferation by activating Wnt/β-catenin signaling pathway and its downstream mediators which have the ability to regulate the expression of various genes that play important role in cell growth and survival (e.g., Cyclin D1, TBX1). Lithium treatment can also promoter cell differentiation by increasing the expression of tissue specific transcription factors (TTF-1, PAX8). Also, lithium treatment at low concentration provide protective effects to the cells against toxic compounds by inhibiting the expression of BAX (pro-apoptotic gene) and promoting the expression of BCL2 (anti-apoptotic gene). Besides these, there might be other mechanisms of action involved which are currently unknown.
Another study also documented the role of lithium in the differentiation of a follicular thyroid cancer (FTC) model. FTC is characterized by a significantly lower expression of Nuclear Receptor Subfamily 4 (NR4A1) compared with benign follicular adenomas (73). NR4A1 receptors have emerged as important molecular switches in processes associated with carcinogenesis, including apoptosis, DNA repair, proliferation, migration, inflammation, and metabolism. NR4A1 has been shown to be lower in various tumor types in comparison to normal tissues. The Wnt signaling pathway is one of the upstream mechanism of NR4A1 regulation (74). Lithium treatment resulted in restoration of NR4A1 expression in FTC cell line as well as inhibition of cell growth and induction of apoptosis in vitro (73). Besides NR4A1, lithium treatment upregulated the expression of FOSB which is a downstream target of Wnt signaling pathway. This suggests that upregulation of NR4A1 by lithium treatment is possibly associated with activation of Wnt signaling pathway. Wnt signaling pathway is known to be regulated by GSK-3β (75) and lithium treatment has been shown to inhibit activity of GSK-3β (76). The exact mechanisms by which lithium inhibits GSK-3β and Wnt regulates NR4A1 are not known. Moreover, one of the cell lines used in this above mentioned study was WRO, and its classification as a model for human FTC has been questioned in subsequent studies (77).
Since lithium is a potential differentiation stimulus, several studies have tested its role as an RAI uptake enhancer in in vitro models via induction of NIS. The main shortcoming of this approach is that cancer cells lack the machinery responsible for iodine organification and therefore intracellular RAI is rapidly released (78). Liu et al. analyzed the role of lithium in a rat follicular cell line intrinsically expressing NIS (FRTL5), and a follicular thyroid cancer cell line FTC133 stably transfected with NIS (60). Lithium in a concentration ranging between 0.5 and 2 mM did not affect RAI efflux nor residence time in these models (60). Similarly, Elisei et al. used anaplastic and medullary thyroid cancer models stably transfected with NIS and showed that treatment with 10 mM of lithium chloride was not associated with increased RAI retention (78).
More encouraging results have been found in both in vitro and in vivo models of medullary thyroid cancer (MTC). Lithium at concentrations ranging between 10 and 30 mM, through inhibition of GSK-3β signaling, led to significant inhibition of MTC cell line growth and decreased production of neuroendocrine markers (79). The growth inhibitory effects of lithium were associated with increase in the levels of cyclin-dependent kinase inhibitors (p21, p27, and p15) leading to cell cycle arrest. The lithium mediated growth inhibition was aslo confirmed in an in vivo xenograft model of MTC (79). Moreover, these effects were achieved with therapeutic concentrations in mice sera, ranging between 0.2 and 1 mEq/l, thus reflecting the therapeutic window in humans. Adler et al. documented an additive effect of treatment with lithium in combination with the histone deacetylase inhibitor valproic acid in inhibition of growth and induction of apoptosis of TT (MTC cell line) cells in vitro (14).
Overall, based on in vitro experiments, there is evidence that lithium affects GSK-3β and Wnt/β-catenin signaling in a cell type-dependent manner—with stimulation of proliferation of normal follicular cells and inconsistent inhibition of cell growth in thyroid cancer cell lines (Figure 1; Table 1). The majority of pre-clinical studies utilized in vitro models exposed to supra-therapeutic concentrations of lithium, limiting its translational perspective. The only in vivo data documenting growth inhibitory effects of lithium are available for an MTC xenograft model. The role of lithium as a differentiation stimulus and RAI-retention enhancer is limited and needs to be clarified based on in vivo studies.
Clinical Evidence
Lithium and Benign Thyroid Nodules/Goiter
Turner et al. documented increased thyroidal retention of RAI in patients with hyperthyroidism treated with lithium (54) (Table 2). Data from two large randomized studies performed in Italy revealed that lithium enhanced the effectiveness of RAI therapy resulting in a more prompt control of the disease in patients with large goiters (55, 56). The authors also claimed that lithium diminished RAI-induced release of thyroid hormones into the circulation, an observation confirmed by another group (58), suggesting that adjuvant lithium therapy could be employed in the RAI therapy for goiters, particularly in elderly patients with high cardiovascular risk. Martin et al. also showed that cure was twice as likely in patients with toxic multinodular goiter receiving RAI with adjuvant lithium compared with RAI therapy alone (80). However, another randomized study concluded that the role of lithium as adjuvant therapy in patients with large goiters was insignificant (57). The latter study was the largest one involving patients with goiters (Table 2), suggesting that the role of lithium as an adjunct to RAI therapy might be either minimal or not significant. The discrepancy in the results of above-mentioned studies might be due to varying RAI dosages implemented in therapy, different thyroid volumes of included patients, varying doses, and duration of exposure to lithium, different iodine nutritional status, to name just a few. Interestingly, exposure to lithium has been associated with morphological changes of the thyrocytes, namely pronounced pleomorphism of the epithelial cells and marked nuclear changes (81). These features might be interpreted in cytology specimens received from fine-needle aspiration biopsy of thyroid nodules as atypia of uncertain significance (82). Therefore, lithium may need to be added to the list of medications causing cytologic atypia.
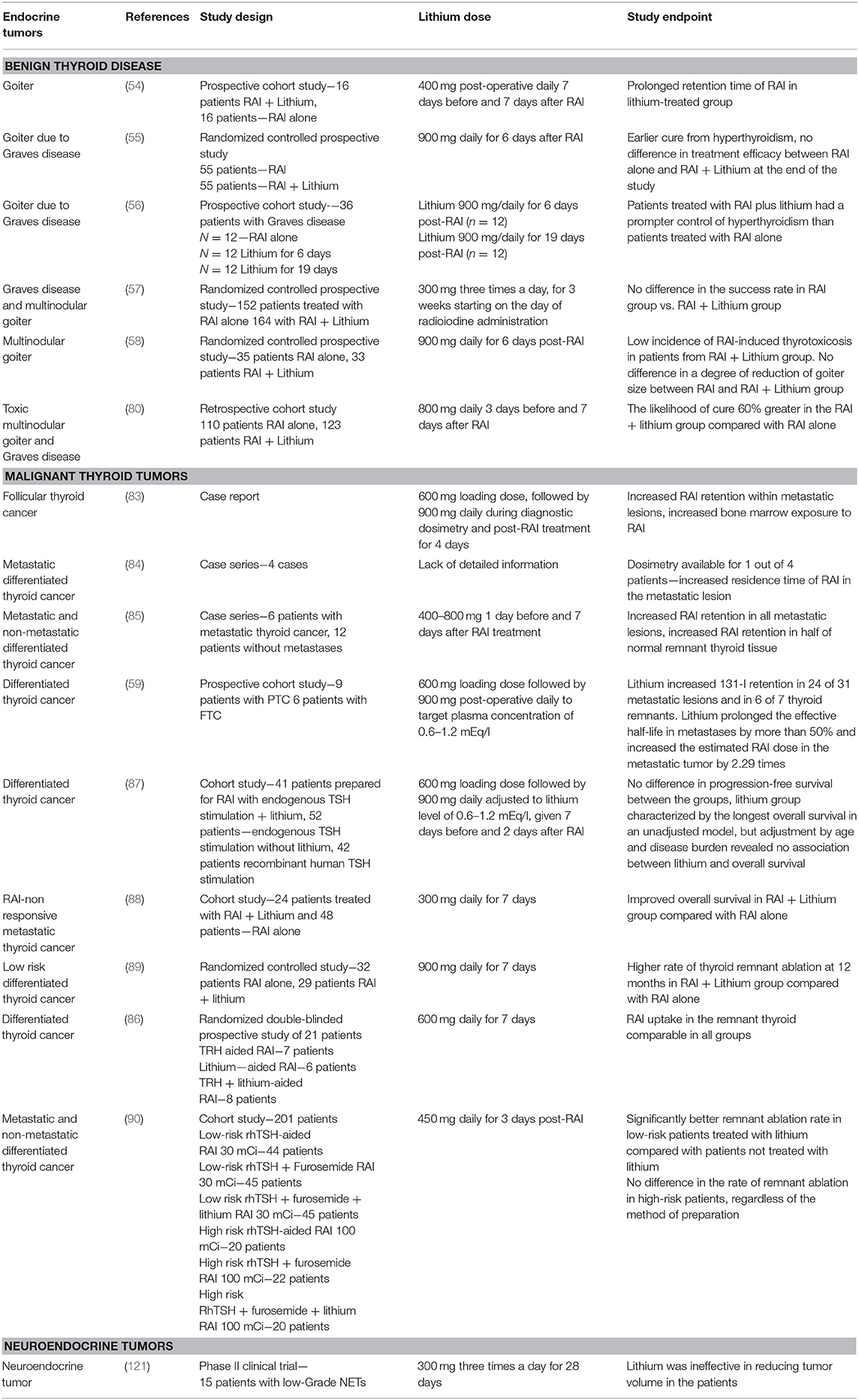
Table 2. Clinical studies utilizing lithium in benign thyroid disorders and malignant thyroid nodules.
Lithium and Thyroid Cancer
One of the first studies utilizing adjunctive lithium therapy in patients with thyroid cancer were case reports of metastatic thyroid cancer associated with hyperthyroidism due to hormonal overproduction by DTC (83, 84). The effects of addition of lithium were quantified with tumor and whole-body dosimetry. Adjunctive lithium therapy resulted in a longer retention time of RAI within the metastatic lesions but also increased exposure of the bone marrow to radiation (83). Moreover, achievement of therapeutic lithium levels of 0.6–1.2 mEq/l was associated with side effects, including nausea and vomiting. Interestingly, Pons et al. showed that although RAI retention was significantly increased in all metastatic lesions in DTC patients with distant metastases, it was not significantly increased in the remnant normal thyroid tissue in ~50% of low-risk patients (85). This observation was further confirmed by Ang et al. who showed a lack of enhanced retention of RAI in thyroid remnant tissue after exposure to lithium (86).
Another case series from the National Institute of Health, United States of America including 15 patients with metastatic DTC found that lithium increased RAI retention in 24 of 31 metastatic lesions and in 6 of 7 thyroid remnants. Lithium prolonged the effective half-life in metastases by more than 50% and increased the estimated RAI dose in the metastatic tumor by 2.29-fold (59). However, the long-term efficacy of adjunctive lithium therapy in this cohort was not available until recently, when our group summarized the National Institute of Health experience with lithium-aided RAI therapy for metastatic DTC (87). There was no difference in progression-free survival in patients treated with lithium-aided RAI compared with patients treated with RAI alone. Although therapy with lithium was associated with improved overall survival in unadjusted models, adjustment by clinically relevant factors affecting overall survival such as age and disease burden revealed no added benefit of lithium. The main factors affecting the outcome were patient age and disease burden, while the method of preparation for RAI therapy was not associated with improved progression-free or overall survival (87). This study was characterized by the largest to date cohort of dosimetry-based lithium-aided therapy of metastatic thyroid cancer and relatively decent duration of follow of a median 5 years. In a smaller cohort, 24 Korean patients with metastatic RAI-refractory DTC underwent combined treatment with RAI and lithium and the treatment efficacy was compared with 48 patients treated solely with RAI. The comparison analysis was performed using propensity score matching (88). The overall survival was significantly better in lithium-aided RAI treated patients, but the analysis was not adjusted by other factors affecting the outcome such as age, number, and location of metastatic foci (88).
In contrast to patients with high-risk metastatic thyroid cancer, addition of lithium to low dose RAI for thyroid remnant ablation in low-risk DTC patients without distant metastases has proven to be beneficial. Yamazaki et al. performed a randomized controlled study in Brazil involving 32 patients treated with 30 mCi of RAI alone and 29 patients treated with RAI with addition of lithium. One year follow up studies revealed a higher thyroid remnant ablation rate in the RAI + lithium group compared with RAI alone, as documented by undetectable stimulated thyroglobulin and negative 123I whole-body scan (89). Similar findings were observed in another study conducted in Italy by Barbaro et al. on thyroid ablation in low-risk DTC patients, while this study found no difference in remnant ablation in high-risk patients treated with 100 mCi of RAI, regardless of whether lithium treatment was added (90). It is worthwhile to speculate that the higher efficacy of lithium-aided RAI therapy in low-risk patients might be due to the higher level of NIS expression in low-risk patients, particularly in normal remnant tissue, compared with relatively lower NIS expression in tissues derived from distant metastases of high-risk patients (91). Lithium-induced extension of RAI residence time in metastatic lesions might not be sufficient to deliver tumoricidal RAI dose to the high-risk tumors. It might be interesting to design a study utilizing combination therapy with NIS inducing agents such as MEK or BRAF inhibitors with lithium as an adjunct to RAI therapy of high-risk DTC patients.
Despite very promising pre-clinical data in in vitro and in vivo MTC models, a clinical trial utilizing lithium monotherapy in MTC was terminated, and 3 out of 5 enrolled patients died of disease (NCT00582712).
Overall, therapy with lithium was well-tolerated. Gastrointestinal disturbances (nausea, vomiting, diarrhea) were reported most commonly in up to 10–20% of patients (85). Although lithium treatment has been reported to increase remnant ablation rate in low-risk patients with DTC, its utility in this setting may be limited as current guidelines recommend against RAI in thyroid cancer confined to the thyroid gland based upon the observed lack of benefit of RAI therapy on mortality and morbidity in low-risk thyroid cancer patients. Additive lithium therapy in high-risk patients has not been proven to improve long-term outcomes in patients with metastatic DTC.
Lithium and Parathyroid Tumors
Epidemiology
Hyperparathyroidism due to benign parathyroid adenomas overproducing parathyroid hormone (PTH) is one of the most common endocrine disorders. Treatment with lithium has been associated with 4–6 higher likelihood of hyperparathyroidism compared with its incidence in the general population (92). Most studies have shown that lithium-induced hyperparathyroidism is associated with the presence of a solitary parathyroid adenoma (92–96). However, lithium-induced polyglandular hyperplasia has also been described (95–97).
The molecular signature of lithium-induced parathyroid adenomas reveals that gross chromosomal alterations occur rarely. In most cases, the tumorigenic pathway is independent of multiple endocrine neoplasia gene (MEN1) and genes at 1p34.3 and 1q21-q32, suggesting a unique etiology of development of these tumors (98).
The management of lithium-induced parathyroid adenomas is predominantly surgical, however, calcimimetics have been also successfully used, particularly in the pediatric population (96, 97, 99–101).
Mechanism of Lithium Action—Pre-clinical Evidence
Calcium sensing receptors (CaSRs) are present in parathyroid glands, renal tubules, and bones. Lithium is known to shift the CaSR set point in parathyroid cells, raising the threshold of serum calcium necessary to inhibit PTH secretion, resulting in an increase in parathyroid cell proliferation and increased PTH synthesis and secretion (94). One of the potential mechanisms of stimulation of parathyroid cell proliferation by lithium might be the activation of the WNT/β-catenin signaling pathway. Verdelli et al. in an in vitro model of parathyroid adenoma-derived cells showed that lithium-induced activation of WNT/β-catenin signaling led to downregulation of the transcription factor TBX1, which is expressed in adult parathyroid cells and deregulated in parathyroid tumors (Figure 1) (102). Similar findings of activation of Wnt/β-catenin signaling pathway leading to downregulation of TBX1 were observed by Corbetta et al. in HEK293 cells (103). TBX1 deficiency may potentially contribute to the low proliferative index of parathyroid tumors (102). In fact, Saxe et al. in a study utilizing primary cultures of parathyroid adenomas showed that therapeutic concentrations of lithium of 2 mM lead to enhanced proliferation of parathyroid cells in vitro (104). Lithium has been also shown to stimulate release of PTH in vitro (105). To summarize, pre-clinical in vitro studies consistently show that lithium induces proliferation and PTH secretion in parathyroid cells (Table 1).
Tumors of the Adrenal Cortex and Medulla
Epidemiology
Adrenocortical tumors are common in occurrence with most of the tumors being benign and non-functional adrenocortical adenomas (106). A very small proportion of adrenal tumors known as adrenocortical carcinomas (ACC) are malignant in nature and can cause significant morbidity and mortality (107). ACC is a rare malignancy with an incidence of 0.7–2.0 cases/million inhabitants per year (108). Similar to ACC, pheochromocytomas, and paragangliomas which are tumors of adrenal medulla are considered rare tumors affecting around 3 per million people annually (109). Currently, there is no patient-based information available pertaining to the effects of lithium on ACC, pheochromocytomas, and paragangliomas.
Mechanism of Action—Pre-clinical Evidence
At present, there is only one pre-clinical study where the effects of lithium were analyzed on adrenal cortex tumors. The study reported dose-dependent inhibition of apoptosis associated with a reduction in DNA fragmentation in tumor as well as adjacent normal tissues procured from the patients with adrenal cortex tumors. In contrast to this, lithium treatment caused enhanced DNA fragmentation in the adrenocortical tissue of patients with Cushing disease (110). This study was very preliminary in nature as the conclusions were drawn from the analysis of DNA fragmentation observed in the agarose gels and the study utilized supraphysiological concentrations of lithium (5, 10, and 50 mM).
Up until now the effects of lithium on pheochromocytomas have been studied on a single cell line that is derived from a rat pheochromocytoma (PC12). Lithium treatment in PC12 cells at a concentration equivalent to the levels detected in the serum of lithium-treated patients (0.5 mM) resulted in an increase in cell number (111). This increase in cell number was not associated with an increase in proliferation as no significant difference in DNA synthesis was observed. Rather, lithium protected PC12 cells from thapsigargin and trimethylation-induced cell death. A higher lithium concentration (5 mM) resulted in a reduction of cell number (111). In another study, lithium treatment had a concentration-dependent cytotoxic effect on PC12 cells. These cytotoxic effects were associated with interactions of lithium ions to amyloid-β monomers, resulting in the formation of β -sheet fibrils which induced toxic effects within the cells (112).
A similar growth suppression effect of lithium was observed in a study where PC12 cells were exposed to supraphysiological concentrations of lithium (5, 10, and 30 mM) (16). Growth suppression at these concentrations was associated with inhibition of GSK-3β activity via its phosphorylation in a concentration-dependent manner and reduction in the production of vasoactive hormones. The exact mechanism by which lithium phosphorylates and inactivates GSK3 is, however, not known.
Lithium treatment (1.2 mM) has been shown to have protective effects against morphine-induced cell death in PC12 cells. The protective effects of lithium were associated with decreased expression of a pro-apoptotic gene (Bax) and increased expression of an anti-apoptotic gene (BCL-2), resulting in reduced apoptosis (Figure 1) (113). A similar study reported the cytoprotective effects of lithium against a β-amyloid peptide in PC12 cells pretreated with lithium (2 mM) for 7 days in association with increased BCL-2 protein levels (114). Another study showed that lithium treatment at 1 mM concentration for 5 days protected PC12 cells from hydrogen peroxide-induced cell death by increasing nuclear translocation of nuclear factor E2-related factor 2 (Nrf2) protein (115). Altogether, these studies suggest protective rather than anti-cancer effects of lithium at therapeutic concentrations. The studies where cytotoxic effects were observed in PC12 cells were conducted at very high lithium concentrations and therefore lack translational significance (Table 1).
Other Endocrine Tumors
Epidemiology
The other endocrine tumors include neuroendocrine tumors (NETs) and pituitary adenomas (also known as pituitary neuroendocrine tumors, PitNETs). NETs are a heterogeneous group of tumors which have both neuro- and endocrine properties. NETs affect 2–5 people per 100,000 population annually, but its incidence is increasing every year (116). At present, there is very limited information available regarding the effects of lithium on NETs.
PitNETs are the common neoplasms arising from the pituitary gland, with an estimated prevalence of around 17% (117). PitNETs are histologically diverse based on their cell of origin and the type of hormone secreted (118). PitNETs are mostly benign in nature but can lead to substantial morbidity by hypersecretion of pituitary hormones. More than 50% of PitNETS are functional tumors and secrete hormones (117). Currently, there is no patient-based information available pertaining to the effects of lithium on PitNETs.
Mechanism of Action—Pre-clinical Evidence
In an in vitro study performed on NET cell lines- gastrointestinal (BON-1) and pulmonary carcinoid (NCI-H727) cells, lithium treatment at 20 mM concentration inactivated GSK-3β, inhibited cellular proliferation and caused cell cycle arrest in both cell lines. These effects were even more pronounced when lithium treatment (15 mM) was combined with either valproic acid (3 mM) or suberoyl bis-hydroxamic acid (40 μM), both of which activate the Notch signaling pathway (119). In another study utilizing the same cell lines, lithium caused a dose-dependent reduction in cell growth through inactivation of GSK-3β and increased expression of ADP-ribose polymerase, while suppressing serotonin and chromogranin A cellular levels (120). These studies suggest an anti-cancer effect of lithium in NET cells, however, they are very preliminary in nature and the observations need to be validated through in vivo models at therapeutic concentrations. At present, there are no in-vitro or in-vivo studies where the effects of lithium have been investigated in PitNETs.
Clinical Evidence
There is only one clinical trial to date that has been conducted in United States of America by the National Cancer Institute (NCI) to analyze the effects of lithium on patients with NETs. The trial included 15 patients with low-grade NETs that were given 300 mg lithium orally 3 times daily for 28 days followed by measurement of tumor response. The study reported that lithium was ineffective in demonstrating any objective responses in these patients and the trial was discontinued early (121).
Summary and Conclusions
Patients chronically treated with lithium need to be screened for hypothyroidism, goiter, and hyperparathyroidism, as prevalence of these endocrine abnormalities is higher in lithium-treated patients than in the general population. The growth inhibitory effects of lithium in non-medullary TC, pheochromocytoma/paraganglioma and carcinoid were achieved with supratherapeutic concentrations of lithium in vitro, thus limiting its translational perspective. Although pre-clinical in vivo models of growth inhibitory effects of lithium in MTC were promising, the only clinical trial focused on lithium therapy enrolled only five patients and was ended before the endpoints were met, thus limiting any conclusions of lithium therapy efficacy in MTC. Currently available clinical data on the efficacy of lithium in therapy of endocrine tumors in humans is limited and associated with conflicting results. The most robust data were obtained from clinical trials utilizing combination of lithium and RAI therapy in patients with goiters and thyroid cancer. The lithium dosage utilized in these studies were comparable to the doses used for bipolar and depressive disorders, which ranges from 0.4–2.0g per day (122). Even though treatment with lithium has been associated with the extended retention of RAI in RAI-avid tissues, there is no long-term clinical benefit of lithium therapy in thyroid cancer. Future studies may focus on the potential synergistic role of the combination therapy with NIS-inducing agents such as MEK or BRAF inhibitors to enhance RAI uptake and lithium as an agent extending RAI retention time in the management of metastatic thyroid cancer. More pre-clinical in vivo data utilizing therapeutic concentrations of lithium in monotherapy and combination therapy is needed before translation of lithium from bench to the bedside for the management endocrine tumors is pursued.
Author Contributions
JK-G: review concept and design. JK-G, ST, and AT: manuscript preparation. JK-G and ST: manuscript review.
Funding
NIDDK intramural funding program ZIE DK 047053-12.
Conflict of Interest
The authors declare that the research was conducted in the absence of any commercial or financial relationships that could be construed as a potential conflict of interest.
References
1. Mota de Freitas D, Leverson BD, Goossens JL. Lithium in medicine: mechanisms of action. Met Ions Life Sci. (2016) 16:557–84. doi: 10.1007/978-3-319-21756-7_15
2. Kessing LV, Sondergard L, Forman JL, Andersen PK. Lithium treatment and risk of dementia. Arch Gen Psychiatry. (2008) 65:1331–5. doi: 10.1001/archpsyc.65.11.1331
3. Cole AR. Glycogen synthase kinase 3 substrates in mood disorders and schizophrenia. FEBS J. (2013) 280:5213–27. doi: 10.1111/febs.12407
4. Amar S, Belmaker RH, Agam G. The possible involvement of glycogen synthase kinase-3 (GSK-3) in diabetes, cancer and central nervous system diseases. Curr Pharm Des. (2011) 17:2264–77. doi: 10.2174/138161211797052484
5. McCubrey JA, Steelman LS, Bertrand FE, Davis NM, Sokolosky M, Abrams SL, et al. GSK-3 as potential target for therapeutic intervention in cancer. Oncotarget. (2014) 5:2881–911. doi: 10.18632/oncotarget.2037
6. Maurer U, Preiss F, Brauns-Schubert P, Schlicher L, Charvet C. GSK-3 - at the crossroads of cell death and survival. J Cell Sci. (2014) 127:1369–78. doi: 10.1242/jcs.138057
7. Xia Y, Wu S. Tissue inhibitor of metalloproteinase 2 inhibits activation of the beta-catenin signaling in melanoma cells. Cell Cycle. (2015) 14:1666–74. doi: 10.1080/15384101.2015.1030557
8. Heinrich A, Boer U, Tzvetkov M, Oetjen E, Knepel W. Stimulation by lithium of the interaction between the transcription factor CREB and its co-activator TORC. Biosci Rep. (2009) 29:77–87. doi: 10.1042/BSR20080116
9. Penso J, Beitner R. Lithium detaches hexokinase from mitochondria and inhibits proliferation of B16 melanoma cells. Mol Genet Metab. (2003) 78:74–8. doi: 10.1016/S1096-7192(02)00203-2
10. Cisternas P, Zolezzi JM, Martinez M, Torres VI, Wong GW, Inestrosa NC. Wnt-induced activation of glucose metabolism mediates the in vivo neuroprotective roles of Wnt signaling in Alzheimer disease. J Neurochem. (2019) 149:54–72. doi: 10.1111/jnc.14608
11. Alsady M, de Groot T, Kortenoeven MLA, Carmone C, Neijman K, Bekkenkamp-Grovenstein M, et al. Lithium induces aerobic glycolysis and glutaminolysis in collecting duct principal cells. Am J Physiol Renal Physiol. (2018) 314:F230–9. doi: 10.1152/ajprenal.00297.2017
12. Jin Z, Cheng X, Feng H, Kuang J, Yang W, Peng C, et al. Apatinib inhibits angiogenesis via suppressing Akt/GSK3beta/ANG signaling pathway in anaplastic thyroid cancer. Cell Physiol Biochem. (2017) 44:1471–84. doi: 10.1159/000485583
13. Zhong Z, Hu Z, Jiang Y, Sun R, Chen X, Chu H, et al. Interleukin-11 promotes epithelial-mesenchymal transition in anaplastic thyroid carcinoma cells through PI3K/Akt/GSK3beta signaling pathway activation. Oncotarget. (2016) 7:59652–63. doi: 10.18632/oncotarget.10831
14. Adler JT, Hottinger DG, Kunnimalaiyaan M, Chen H. Inhibition of growth in medullary thyroid cancer cells with histone deacetylase inhibitors and lithium chloride. J Surg Res. (2010) 159:640–4. doi: 10.1016/j.jss.2008.08.004
15. Tissier F, Cavard C, Groussin L, Perlemoine K, Fumey G, Hagnere AM, et al. Mutations of beta-catenin in adrenocortical tumors: activation of the Wnt signaling pathway is a frequent event in both benign and malignant adrenocortical tumors. Cancer Res. (2005) 65:7622–7. doi: 10.1158/0008-5472.CAN-05-0593
16. Kappes A, Vaccaro A, Kunnimalaiyaan M, Chen H. Lithium ions: a novel treatment for pheochromocytomas and paragangliomas. Surgery. (2007) 141:161–5. Discussion 165. doi: 10.1016/j.surg.2006.12.005
17. Guan H, Liang W, Liu J, Wei G, Li H, Xiu L, et al. Transmembrane protease serine 4 promotes thyroid cancer proliferation via CREB phosphorylation. Thyroid. (2015) 25:85–94. doi: 10.1089/thy.2014.0155
18. Thakur S, Daley B, Gaskins K, Vasko VV, Boufraqech M, Patel D, et al. Metformin targets mitochondrial glycerophosphate dehydrogenase to control rate of oxidative phosphorylation and growth of thyroid cancer in vitro and in vivo. Clin Cancer Res. (2018) 24:4030–43. doi: 10.1158/1078-0432.CCR-17-3167
19. Bikas A, Jensen K, Patel A, Costello J Jr, McDaniel D, Klubo-Gwiezdzinska J, et al. Glucose-deprivation increases thyroid cancer cells sensitivity to metformin. Endocr Relat Cancer. (2015) 22:919–32. doi: 10.1530/ERC-15-0402
20. Gilbert-Sirieix M, Makoukji J, Kimura S, Talbot M, Caillou B, Massaad C, et al. Wnt/beta-catenin signaling pathway is a direct enhancer of thyroid transcription factor-1 in human papillary thyroid carcinoma cells. PLoS ONE. (2011) 6:e22280. doi: 10.1371/journal.pone.0022280
21. Ballin A, Aladjem M, Banyash M, Boichis H, Barzilay Z, Gal R, et al. The effect of lithium chloride on tumour appearance and survival of melanoma-bearing mice. Br J Cancer. (1983) 48:83–7. doi: 10.1038/bjc.1983.160
22. Sun A, Shanmugam I, Song J, Terranova PF, Thrasher JB, Li B. Lithium suppresses cell proliferation by interrupting E2F-DNA interaction and subsequently reducing S-phase gene expression in prostate cancer. Prostate. (2007) 67:976–88. doi: 10.1002/pros.20586
23. Erdal E, Ozturk N, Cagatay T, Eksioglu-Demiralp E, Ozturk M. Lithium-mediated downregulation of PKB/Akt and cyclin E with growth inhibition in hepatocellular carcinoma cells. Int J Cancer. (2005) 115:903–10. doi: 10.1002/ijc.20972
24. Wang XM, Li J, Feng XC, Wang Q, Guan DY, Shen ZH. Involvement of the role of Chk1 in lithium-induced G2/M phase cell cycle arrest in hepatocellular carcinoma cells. J Cell Biochem. (2008) 104:1181–91. doi: 10.1002/jcb.21693
25. Asgari MM, Chien AJ, Tsai AL, Fireman B, Quesenberry CP Jr. Association between lithium use and melanoma risk and mortality: a population-based study. J Invest Dermatol. (2017) 137:2087–91. doi: 10.1016/j.jid.2017.06.002
26. Zaidan M, Stucker F, Stengel B, Vasiliu V, Hummel A, Landais P, et al. Increased risk of solid renal tumors in lithium-treated patients. Kidney Int. (2014) 86:184–90. doi: 10.1038/ki.2014.2
27. Bocchetta A, Loviselli A. Lithium treatment and thyroid abnormalities. Clin Pract Epidemiol Ment Health. (2006) 2:23. doi: 10.1186/1745-0179-2-23
28. Lazarus JH. Lithium and thyroid. Best Pract Res Clin Endocrinol Metab. (2009) 23:723–33. doi: 10.1016/j.beem.2009.06.002
29. Perrild H, Hegedus L, Baastrup PC, Kayser L, Kastberg S. Thyroid function and ultrasonically determined thyroid size in patients receiving long-term lithium treatment. Am J Psychiatry. (1990) 147:1518–21. doi: 10.1176/ajp.147.11.1518
30. Bauer M, Blumentritt H, Finke R, Schlattmann P, Adli M, Baethge C, et al. Using ultrasonography to determine thyroid size and prevalence of goiter in lithium-treated patients with affective disorders. J Affect Disord. (2007) 104:45–51. doi: 10.1016/j.jad.2007.01.033
31. Ozpoyraz N, Tamam L, Kulan E. Thyroid abnormalities in lithium-treated patients. Adv Ther. (2002) 19:176–84. doi: 10.1007/BF02848693
32. Caykoylu A, Capoglu I, Unuvar N, Erdem F, Cetinkaya R. Thyroid abnormalities in lithium-treated patients with bipolar affective disorder. J Int Med Res. (2002) 30:80–4. doi: 10.1177/147323000203000112
33. Schiemann U, Hengst K. Thyroid echogenicity in manic-depressive patients receiving lithium therapy. J Affect Disord. (2002) 70:85–90. doi: 10.1016/S0165-0327(00)00374-8
34. Kallner G, Petterson U. Renal, thyroid and parathyroid function during lithium treatment: laboratory tests in 207 people treated for 1-30 years. Acta Psychiatr Scand. (1995) 91:48–51. doi: 10.1111/j.1600-0447.1995.tb09741.x
35. Kirov G. Thyroid disorders in lithium-treated patients. J Affect Disord. (1998) 50:33–40. doi: 10.1016/S0165-0327(98)00028-7
36. Deodhar SD, Singh B, Pathak CM, Sharan P, Kulhara P. Thyroid functions in lithium-treated psychiatric patients: a cross-sectional study. Biol Trace Elem Res. (1999) 67:151–63. doi: 10.1007/BF02784070
37. Kusalic M, Engelsmann F. Effect of lithium maintenance therapy on thyroid and parathyroid function. J Psychiatry Neurosci. (1999) 24:227–33.
38. Johnston AM, Eagles JM. Lithium-associated clinical hypothyroidism. Prevalence and risk factors. Br J Psychiatry. (1999) 175:336–9. doi: 10.1192/bjp.175.4.336
39. Gracious BL, Findling RL, Seman C, Youngstrom EA, Demeter CA, Calabrese JR. Elevated thyrotropin in bipolar youths prescribed both lithium and divalproex sodium. J Am Acad Child Adolesc Psychiatry. (2004) 43:215–20. doi: 10.1097/00004583-200402000-00018
40. Kirov G, Tredget J, John R, Owen MJ, Lazarus JH. A cross-sectional and a prospective study of thyroid disorders in lithium-treated patients. J Affect Disord. (2005) 87:313–7. doi: 10.1016/j.jad.2005.03.010
41. Aliasgharpour M, Abbassi M, Shafaroodi H, Razi F. Subclinical hypothyroidism in lithium-treated psychiatric patients in Tehran, Islamic Republic of Iran. East Mediterr Health J. (2005) 11:329–33.
42. Fagiolini A, Kupfer DJ, Scott J, Swartz HA, Cook D, Novick DM, et al. Hypothyroidism in patients with bipolar I disorder treated primarily with lithium. Epidemiol Psichiatr Soc. (2006) 15:123–7. doi: 10.1017/S1121189X00004322
43. Bocchetta A, Cocco F, Velluzzi F, Del Zompo M, Mariotti S, Loviselli A. Fifteen-year follow-up of thyroid function in lithium patients. J Endocrinol Invest. (2007) 30:363–6. doi: 10.1007/BF03346311
44. Vanderpump MP, Tunbridge WM, French JM, Appleton D, Bates D, Clark F, et al. The incidence of thyroid disorders in the community: a twenty-year follow-up of the Whickham Survey. Clin Endocrinol (Oxf). (1995) 43:55–68. doi: 10.1111/j.1365-2265.1995.tb01894.x
45. Kuman Tuncel O, Akdeniz F, Ozbek SS, Kavukcu G, Unal Kocabas G. Thyroid function and ultrasonography abnormalities in lithium-treated bipolar patients: a cross-sectional study with healthy controls. Noro Psikiyatr Ars. (2017) 54:108–15. doi: 10.5152/npa.2017.12457
46. Barbesino G. Drugs affecting thyroid function. Thyroid. (2010) 20:763–70. doi: 10.1089/thy.2010.1635
47. Mesele M, Degu G, Gebrehiwot H. Prevalence and associated factors of goiter among rural children aged 6-12 years old in Northwest Ethiopia, cross-sectional study. BMC Public Health. (2014) 14:130. doi: 10.1186/1471-2458-14-130
48. Hollowell JG, Staehling NW, Flanders WD, Hannon WH, Gunter EW, Spencer CA, et al. Serum TSH, T(4), and thyroid antibodies in the United States population (1988 to 1994): National Health and Nutrition Examination Survey (NHANES III). J Clin Endocrinol Metab. (2002) 87:489–99. doi: 10.1210/jcem.87.2.8182
49. Canaris GJ, Manowitz NR, Mayor G, Ridgway EC. The Colorado thyroid disease prevalence study. Arch Intern Med. (2000) 160:526–34. doi: 10.1001/archinte.160.4.526
50. Miller KK, Daniels GH. Association between lithium use and thyrotoxicosis caused by silent thyroiditis. Clin Endocrinol. (2001) 55:501–8. doi: 10.1046/j.1365-2265.2001.01381.x
51. Berens SC, Wolff J, Murphy DL. Lithium concentration by the thyroid. Endocrinology. (1970) 87:1085–7. doi: 10.1210/endo-87-5-1085
52. Kohrle J. Local activation and inactivation of thyroid hormones: the deiodinase family. Mol Cell Endocrinol. (1999) 151:103–19. doi: 10.1016/S0303-7207(99)00040-4
53. Berens SC, Bernstein RS, Robbins J, Wolff J. Antithyroid effects of lithium. J Clin Invest. (1970) 49:135767. doi: 10.1172/JCI106352
54. Turner JG, Brownlie BE, Rogers TG. Lithium as an adjunct to radioiodine therapy for thyrotoxicosis. Lancet. (1976) 1:614–5. doi: 10.1016/S0140-6736(76)90419-0
55. Bogazzi F, Bartalena L, Brogioni S, Scarcello G, Burelli A, Campomori A, et al. Comparison of radioiodine with radioiodine plus lithium in the treatment of Graves' hyperthyroidism. J Clin Endocrinol Metab. (1999) 84:499–503. doi: 10.1210/jc.84.2.499
56. Bogazzi F, Bartalena L, Campomori A, Brogioni S, Traino C, De Martino F, et al. Treatment with lithium prevents serum thyroid hormone increase after thionamide withdrawal and radioiodine therapy in patients with Graves' disease. J Clin Endocrinol Metab. (2002) 87:4490–5. doi: 10.1210/jc.2002-020580
57. Bal CS, Kumar A, Pandey RM. A randomized controlled trial to evaluate the adjuvant effect of lithium on radioiodine treatment of hyperthyroidism. Thyroid. (2002) 12:399–405. doi: 10.1089/105072502760043486
58. Vannucchi G, Chiti A, Mannavola D, Dazzi D, Rodari M, Tadayyon S, et al. Radioiodine treatment of non-toxic multinodular goitre: effects of combination with lithium. Eur J Nucl Med Mol Imaging. (2005) 32:1081–8. doi: 10.1007/s00259-005-1818-8
59. Koong SS, Reynolds JC, Movius EG, Keenan AM, Ain KB, Lakshmanan MC, et al. Lithium as a potential adjuvant to 131I therapy of metastatic, well differentiated thyroid carcinoma. J Clin Endocrinol Metab. (1999) 84:912–6. doi: 10.1210/jc.84.3.912
60. Liu YY, van der Pluijm G, Karperien M, Stokkel MP, Pereira AM, Morreau J, et al. Lithium as adjuvant to radioiodine therapy in differentiated thyroid carcinoma: clinical and in vitro studies. Clin Endocrinol. (2006) 64:617–624. doi: 10.1111/j.1365-2265.2006.02515.x
61. Klubo-Gwiezdzinska J, Wartofsky L. The role of molecular diagnostics in the management of indeterminate thyroid nodules. J Clin Endocrinol Metab. (2018) 103:3507–10. doi: 10.1210/jc.2018-01081
62. Haugen BR, Alexander EK, Bible KC, Doherty GM, Mandel SJ, Nikiforov YE, et al. 2015 American Thyroid Association management guidelines for adult patients with thyroid nodules and differentiated thyroid cancer: the American Thyroid Association guidelines task force on thyroid nodules and differentiated thyroid cancer. Thyroid. (2016) 26:1–133. doi: 10.1089/thy.2015.0020
63. Heltne CE, Ollerich DA. Morphometric and electron microscopic studies of goiter induced by lithium in the rat. Am J Anat. (1973) 136:297–303. doi: 10.1002/aja.1001360304
64. Klein PS, Melton DA. A molecular mechanism for the effect of lithium on development. Proc Natl Acad Sci USA. (1996) 93:8455–9. doi: 10.1073/pnas.93.16.8455
65. Hedgepeth CM, Conrad LJ, Zhang J, Huang HC, Lee VM, Klein PS. Activation of the Wnt signaling pathway: a molecular mechanism for lithium action. Dev Biol. (1997) 185:82–91. doi: 10.1006/dbio.1997.8552
66. Rao AS, Kremenevskaja N, Resch J, Brabant G. Lithium stimulates proliferation in cultured thyrocytes by activating Wnt/beta-catenin signalling. Eur J Endocrinol. (2005) 153:929–38. doi: 10.1530/eje.1.02038
67. Jeong WJ, Ro EJ, Choi KY. Interaction between Wnt/beta-catenin and RAS-ERK pathways and an anti-cancer strategy via degradations of beta-catenin and RAS by targeting the Wnt/beta-catenin pathway. NPJ Precis Oncol. (2018) 2:5. doi: 10.1038/s41698-018-0049-y
68. Krishnamurthy N, Kurzrock R. Targeting the Wnt/beta-catenin pathway in cancer: update on effectors and inhibitors. Cancer Treat Rev. (2018) 62:50–60. doi: 10.1016/j.ctrv.2017.11.002
69. Elisei R, Pinchera A, Romei C, Gryczynska M, Pohl V, Maenhaut C, et al. Expression of thyrotropin receptor (TSH-R), thyroglobulin, thyroperoxidase, and calcitonin messenger ribonucleic acids in thyroid carcinomas: evidence of TSH-R gene transcript in medullary histotype. J Clin Endocrinol Metab. (1994) 78:867–71. doi: 10.1210/jcem.78.4.8157713
70. Dupain C, Ali HM, Mouhoub TA, Urbinati G, Massaad-Massade L. Induction of TTF-1 or PAX-8 expression on proliferation and tumorigenicity in thyroid carcinomas. Int J Oncol. (2016) 49:1248–58. doi: 10.3892/ijo.2016.3617
71. Kimura S. Thyroid-specific transcription factors and their roles in thyroid cancer. J Thyroid Res. (2011) 2011:710213. doi: 10.4061/2011/710213
72. Lopez-Campistrous A, Thiesen A, Gill AJ, Ghosh S, McMullen TP. Loss of nuclear localization of thyroid transcription factor 1 and adverse outcomes in papillary thyroid cancer. Hum Pathol. (2019) 91:36–42. doi: 10.1016/j.humpath.2019.06.002
73. Camacho CP, Latini FR, Oler G, Hojaij FC, Maciel RM, Riggins GJ, et al. Down-regulation of NR4A1 in follicular thyroid carcinomas is restored following lithium treatment. Clin Endocrinol. (2009) 70:475–83. doi: 10.1111/j.1365-2265.2008.03349.x
74. Mohan HM, Aherne CM, Rogers AC, Baird AW, Winter DC, Murphy EP. Molecular pathways: the role of NR4A orphan nuclear receptors in cancer. Clin Cancer Res. (2012) 18:3223–8. doi: 10.1158/1078-0432.CCR-11-2953
75. Wu D, Pan W. GSK3: a multifaceted kinase in Wnt signaling. Trends Biochem Sci. (2010) 35:161–8. doi: 10.1016/j.tibs.2009.10.002
76. Stambolic V, Ruel L, Woodgett JR. Lithium inhibits glycogen synthase kinase-3 activity and mimics wingless signalling in intact cells. Curr Biol. (1996) 6:1664–8. doi: 10.1016/S0960-9822(02)70790-2
77. Saiselet M, Floor S, Tarabichi M, Dom G, Hebrant A, van Staveren WC, et al. Thyroid cancer cell lines: an overview. Front Endocrinol. (2012) 3:133. doi: 10.3389/fendo.2012.00133
78. Elisei R, Vivaldi A, Ciampi R, Faviana P, Basolo F, Santini F, et al. Treatment with drugs able to reduce iodine efflux significantly increases the intracellular retention time in thyroid cancer cells stably transfected with sodium iodide symporter complementary deoxyribonucleic acid. J Clin Endocrinol Metab. (2006) 91:2389–95. doi: 10.1210/jc.2005-2480
79. Kunnimalaiyaan M, Vaccaro AM, Ndiaye MA, Chen H. Inactivation of glycogen synthase kinase-3beta, a downstream target of the raf-1 pathway, is associated with growth suppression in medullary thyroid cancer cells. Mol Cancer Ther. (2007) 6:1151–8. doi: 10.1158/1535-7163.MCT-06-0665
80. Martin NM, Patel M, Nijher GM, Misra S, Murphy E, Meeran K. Adjuvant lithium improves the efficacy of radioactive iodine treatment in Graves' and toxic nodular disease. Clin Endocrinol. (2012) 77:621–7. doi: 10.1111/j.1365-2265.2012.04385.x
81. Fauerholdt L, Vendsborg P. Thyroid gland morphology after lithium treatment. Acta Pathol Microbiol Scand A. (1981) 89:339–41. doi: 10.1111/j.1699-0463.1981.tb00230.x
82. Pusztaszeri M, Ibrahim Y, Malacarne S, Meyer P. Lithium-induced atypia in fine-needle aspiration biopsy of thyroid nodules. Cytopathology. (2014) 25:35.
83. Gershengorn MC, Izumi M, Robbins J. Use of lithium as an adjunct to radioiodine therapy of thyroid carcinoma. J Clin Endocrinol Metab. (1976) 42:105–11. doi: 10.1210/jcem-42-1-105
84. Briere J, Pousset G, Darsy P, Guinet. [The advantage of lithium in association with iodine 131 in the treatement of functioning metastasis of the thyroid cancer (author's transl)]. Ann Endocrinol. (1974) 35:281–2.
85. Pons F, Carrio I, Estorch M, Ginjaume M, Pons J, Milian R. Lithium as an adjuvant of iodine-131 uptake when treating patients with well-differentiated thyroid carcinoma. Clin Nucl Med. (1987) 12:644–7. doi: 10.1097/00003072-198708000-00014
86. Ang ES, Teh HS, Sundram FX, Lee KO. Effect of lithium and oral thyrotrophin-releasing hormone (TRH) on serum thyrotrophin (TSH) and radioiodine uptake in patients with well differentiated thyroid carcinoma. Singapore Med J. (1995) 36:606–8.
87. Luo H, Tobey A, Auh S, Cochran C, Zemskova M, Reynolds J, et al. The effect of lithium on the progression-free and overall survival in patients with metastatic differentiated thyroid cancer undergoing radioactive iodine therapy. Clin Endocrinol (Oxf). (2018) 89:481–8. doi: 10.1111/cen.13806
88. Lim I, Park J, Kim H, Byun B, Kim B, Choi C, et al. Usefulness of combined therapy with 131I and lithium in patients with metastatic radioiodine-refractory thyroid cancer. Thyroid. (2014) 24:A20.
89. Yamazaki CA, Padovani RP, Biscolla RP, Ikejiri ES, Marchetti RR, Castiglioni ML, et al. Lithium as an adjuvant in the postoperative ablation of remnant tissue in low-risk thyroid carcinoma. Thyroid. (2012) 22:1002–6. doi: 10.1089/thy.2011.0372
90. Barbaro D, Grosso M, Boni G, Lapi P, Pasquini C, Orsini P, et al. Recombinant human TSH and ablation of post-surgical thyroid remnants in differentiated thyroid cancer: the effect of pre-treatment with furosemide and furosemide plus lithium. Eur J Nucl Med Mol Imaging. (2010) 37:242–9. doi: 10.1007/s00259-009-1254-2
91. Tavares C, Coelho MJ, Eloy C, Melo M, da Rocha AG, Pestana A, et al. NIS expression in thyroid tumors, relation with prognosis clinicopathological and molecular features. Endocr Connect. (2018) 7:78–90. doi: 10.1530/EC-17-0302
92. Awad SS, Miskulin J, Thompson N. Parathyroid adenomas versus four-gland hyperplasia as the cause of primary hyperparathyroidism in patients with prolonged lithium therapy. World J Surg. (2003) 27:486–8. doi: 10.1007/s00268-002-6824-4
93. Pamathy G, Jayarajah U, Wangmo T, Banagala ASK. Lithium-induced symptomatic hypercalcemia and hyperparathyroidism in a patient with bipolar affective disorder: a case report and review of literature. Indian J Psychol Med. (2018) 40:378–80. doi: 10.4103/IJPSYM.IJPSYM_305_17
94. Haden ST, Stoll AL, McCormick S, Scott J, Fuleihan Ge-H. Alterations in parathyroid dynamics in lithium-treated subjects. J Clin Endocrinol Metab. (1997) 82:2844–8. doi: 10.1210/jc.82.9.2844
95. Nordenstrom J, Elvius M, Bagedahl-Strindlund M, Zhao B, Torring O. Biochemical hyperparathyroidism and bone mineral status in patients treated long-term with lithium. Metabolism. (1994) 43:1563–7. doi: 10.1016/0026-0495(94)90017-5
96. Jarhult J, Ander S, Asking B, Jansson S, Meehan A, Kristoffersson A, et al. Long-term results of surgery for lithium-associated hyperparathyroidism. Br J Surg. (2010) 97:1680–5. doi: 10.1002/bjs.7199
97. Ibrahim Y, Mohamed SE, Deniwar A, Al-Qurayshi ZH, Kandil E. Lithium-Associated Hyperparathyroidism: a Pooled analysis. ORL J Otorhinolaryngol Relat Spec. (2015) 77:273–80. doi: 10.1159/000431230
98. Dwight T, Kytola S, Teh BT, Theodosopoulos G, Richardson AL, Philips J, et al. Genetic analysis of lithium-associated parathyroid tumors. Eur J Endocrinol. (2002) 146:619–27. doi: 10.1530/eje.0.1460619
99. Gandhi K, Athanassaki ID, Karaviti LP, Suresh D. Calcimimetics in a pediatric case of lithium induced hyperparathyroidism: new application of an old drug in pediatrics. Endocr Rev. (2014) 35.
100. Marti JL, Yang CS, Carling T, Roman SA, Sosa JA, Donovan P, et al. Surgical approach and outcomes in patients with lithium-associated hyperparathyroidism. Ann Surg Oncol. (2012) 19:3465–71. doi: 10.1245/s10434-012-2367-6
101. Norlen O, Sidhu S, Sywak M, Delbridge L. Long-term outcome after parathyroidectomy for lithium-induced hyperparathyroidism. Br J Surg. (2014) 101:1252–6. doi: 10.1002/bjs.9589
102. Verdelli C, Avagliano L, Guarnieri V, Cetani F, Ferrero S, Vicentini L, et al. Expression, function, and regulation of the embryonic transcription factor TBX1 in parathyroid tumors. Lab Invest. (2017) 97:1488–99. doi: 10.1038/labinvest.2017.88
103. Corbetta S, Verdelli C, Vaira V, Meregalli M, Belicchi M, Terranegra A, et al. Wnt/beta-catenin regulates the T-box transcription factor TBX1, the candidate gene of 22Q11.2 microdeletion/digeorge syndrome, in human parathyroid tumors. Endocr Rev. (2011) 32. doi: 10.1210/endo-meetings.2011.PART3.P18.P3-47
104. Saxe A, Gibson G. Effect of lithium on incorporation of bromodeoxyuridine and tritiated thymidine into human parathyroid cells. Arch Surg. (1993) 128:865–9. doi: 10.1001/archsurg.1993.01420200039007
105. Birnbaum J, Klandorf H, Giuliano A, Van Herle A. Lithium stimulates the release of human parathyroid hormone in vitro. J Clin Endocrinol Metab. (1988) 66:1187–91. doi: 10.1210/jcem-66-6-1187
106. Else T, Kim AC, Sabolch A, Raymond VM, Kandathil A, Caoili EM, et al. Adrenocortical carcinoma. Endocr Rev. (2014) 35:282–326. doi: 10.1210/er.2013-1029
107. Gaujoux S, Weinandt M, Bonnet S, Reslinger V, Bertherat J, Dousset B. Surgical treatment of adrenal carcinoma. J Visc Surg. (2017) 154:335–43. doi: 10.1016/j.jviscsurg.2017.06.010
108. Libe R. Adrenocortical carcinoma (ACC): diagnosis, prognosis, and treatment. Front Cell Dev Biol. (2015) 3:45. doi: 10.3389/fcell.2015.00045
109. Turchini J, Cheung VKY, Tischler AS, De Krijger RR, Gill AJ. Pathology and genetics of phaeochromocytoma and paraganglioma. Histopathology. (2018) 72:97–105. doi: 10.1111/his.13402
110. Pushkarev VM, Tronko ND, Kostyuchenko NN, Mikosha AS. Effect of o,p'-DDD and Li+ on apoptotic DNA fragmentation in conventionally normal and tumour tissues of human adrenal cortex. Ukr Biokhim Zh (1999). (2007) 79:44–9.
111. Fabrizi C, De Vito S, Somma F, Pompili E, Catizone A, Leone S, et al. Lithium improves survival of PC12 pheochromocytoma cells in high-density cultures and after exposure to toxic compounds. Int J Cell Biol. (2014) 2014:135908. doi: 10.1155/2014/135908
112. Yang W, Yan Z, Hongjing Z, Dexiang L, Zengxun L, Xiaojing C, et al. The toxic effect of lithium ion on neurons (PC12 cells) and Abeta42 molecules. Biol Trace Elem Res. (2014) 159:410–5. doi: 10.1007/s12011-014-9949-z
113. Sahebgharani M, Nejati M, Sepehrizadeh Z, Khorramizadeh MR, Bahrololoumi-Shapourabadi M, Hashemi-Bozchlou S, et al. Lithium chloride protects PC12 pheochromocytoma cell line from morphine-induced apoptosis. Arch Iran Med. (2008) 11:639–48. doi: 10.1016/j.toxlet.2007.05.204
114. Wei H, Leeds PR, Qian Y, Wei W, Chen R, Chuang D. beta-amyloid peptide-induced death of PC 12 cells and cerebellar granule cell neurons is inhibited by long-term lithium treatment. Eur J Pharmacol. (2000) 392:117–23. doi: 10.1016/S0014-2999(00)00127-8
115. Rizak J, Tan H, Zhu H, Wang JF. Chronic treatment with the mood-stabilizing drug lithium up-regulates nuclear factor E2-related factor 2 in rat pheochromocytoma PC12 cells in vitro. Neuroscience. (2014) 256:223–9. doi: 10.1016/j.neuroscience.2013.10.036
116. Kunz PL. Carcinoid and neuroendocrine tumors: building on success. J Clin Oncol. (2015) 33:1855–63. doi: 10.1200/JCO.2014.60.2532
117. Mehta GU, Lonser RR. Management of hormone-secreting pituitary adenomas. Neuro Oncol. (2017) 19:762–73. doi: 10.1093/neuonc/now130
118. Theodros D, Patel M, Ruzevick J, Lim M, Bettegowda C. Pituitary adenomas: historical perspective, surgical management and future directions. CNS Oncol. (2015) 4:411–29. doi: 10.2217/cns.15.21
119. Adler JT, Hottinger DG, Kunnimalaiyaan M, Chen H. Combination therapy with histone deacetylase inhibitors and lithium chloride: a novel treatment for carcinoid tumors. Ann Surg Oncol. (2009) 16:481–6. doi: 10.1245/s10434-008-0194-6
120. Greenblatt DY, Ndiaye M, Chen H, Kunnimalaiyaan M. Lithium inhibits carcinoid cell growth in vitro. Am J Transl Res. (2010) 2:248–53.
121. Lubner SJ, Kunnimalaiyaan M, Holen KD, Ning L, Ndiaye M, Loconte NK, et al. A preclinical and clinical study of lithium in low-grade neuroendocrine tumors. Oncologist. (2011) 16:452–7. doi: 10.1634/theoncologist.2010-0323
122. Oruch R, Elderbi MA, Khattab HA, Pryme IF, Lund A. Lithium: a review of pharmacology, clinical uses, and toxicity. Eur J Pharmacol. (2014) 740:464–73. doi: 10.1016/j.ejphar.2014.06.042
123. Zatz M, Reisine TD. Lithium induces corticotropin secretion and desensitization in cultured anterior pituitary cells. Proc Natl Acad Sci USA. (1985) 82:1286–90. doi: 10.1073/pnas.82.4.1286
Keywords: lithium, endocrine tumors, mechanism of action, pre-clinical evidence, clinical evidence
Citation: Thakur S, Tobey A and Klubo-Gwiezdzinska J (2019) The Role of Lithium in Management of Endocrine Tumors—A Comprehensive Review. Front. Oncol. 9:1092. doi: 10.3389/fonc.2019.01092
Received: 31 July 2019; Accepted: 04 October 2019;
Published: 18 October 2019.
Edited by:
Benyi Li, University of Kansas Medical Center, United StatesReviewed by:
Zongbing You, Tulane University, United StatesHui-Ju Hsieh, University of Texas MD Anderson Cancer Center, United States
Copyright © 2019 Thakur, Tobey and Klubo-Gwiezdzinska. This is an open-access article distributed under the terms of the Creative Commons Attribution License (CC BY). The use, distribution or reproduction in other forums is permitted, provided the original author(s) and the copyright owner(s) are credited and that the original publication in this journal is cited, in accordance with accepted academic practice. No use, distribution or reproduction is permitted which does not comply with these terms.
*Correspondence: Joanna Klubo-Gwiezdzinska, joanna.klubo-gwiezdzinska@nih.gov
†These authors have contributed equally to this work