- 1Department of Clinical Medicine and Surgery, Molecular Biology, University Federico II, Naples, Italy
- 2Department of Clinical and Biological Sciences, University of Turin, Turin, Italy
- 3Section of Hematology, Department of Clinical and Experimental Medicine, University of Pisa, Pisa, Italy
More than 15 years ago, imatinib entered into the clinical practice as a “magic bullet”; from that point on, the prognosis of patients affected by chronic myeloid leukemia (CML) became comparable to that of aged-matched healthy subjects. The aims of treatment with tyrosine kinase inhibitors (TKIs) are for complete hematological response after 3 months of treatment, complete cytogenetic response after 6 months, and a reduction of the molecular disease of at least 3 logs after 12 months. Patients who do not reach their goal can switch to another TKI. Thus, the molecular monitoring of response is the main consideration of management of CML patients. Moreover, cases in deep and persistent molecular response can tempt the physician to interrupt treatment, and this “dream” is possible due to the quantitative PCR. After great international effort, today the BCR-ABL1 expression obtained in each laboratory is standardized and expressed as “international scale.” This aim has been reached after the establishment of the EUTOS program (in Europe) and the LabNet network (in Italy), the platforms where biologists meet clinicians. In the field of quantitative PCR, the digital PCR is now a new and promising, sensitive and accurate tool. Some authors reported that digital PCR is able to better classify patients in precise “molecular classes,” which could lead to a better identification of those cases that will benefit from the interruption of therapy. In addition, digital PCR can be used to identify a point mutation in the ABL1 domain, mutations that are often responsible for the TKI resistance. In the field of resistance, a prominent role is played by the NGS that enables identification of any mutation in ABL1 domain, even at sub-clonal levels. This manuscript reviews how the molecular tools can lead the management of CML patients, focusing on the more recent technical advances.
Introduction
It is well-known that more than 95% of cases of chronic myeloid leukemia (CML) are characterized by the presence of the Philadelphia chromosome (Ph'), the deleted chromosome 22 produced by the reciprocal (but not fully balanced) translocation between the long arms of chromosome 9 and 22. During this event, two genes, Abelson 1 (ABL1), located on chromosome 9, and the Breakpoint Cluster Region (BCR), on chromosome 22, generate a fusion gene called BCR-ABL1, that, along with the Philadelphia chromosome, is the main diagnostic marker of CML (1).
Breakpoints within the BCR gene may be located in three different regions; in particular, the more common breakpoints are located downstream of exon 13 or exon 14 (e13, e14 named subtype b2 and b3), and more rarely (2–3%) on exons 6, 8, or 19 (2, 3).
Breakpoints on the ABL1 gene are located on exon 2, upstream (subtype a2) or downstream (subtype a3), with two different fusion constructs; both of these genes encode a protein of 210 kDa (p210). Alternatively, breakpoints might occur between exon 1 of BCR and exon 2 of ABL1 (e1a2), thus encoding a protein of 190 kDa (p190), which is more frequently found in acute lymphoblastic leukemia (>75%), and more rarely in acute myeloid leukemia (2%) and in CML (<1%) (4, 5). Finally, the break downstream of exon 19 (e19-a2) can generate the “micro BCR/ABL1,” and its p230 protein, associated with a less aggressive “chronic neutrophilic leukemia” (6) (Figure 1).
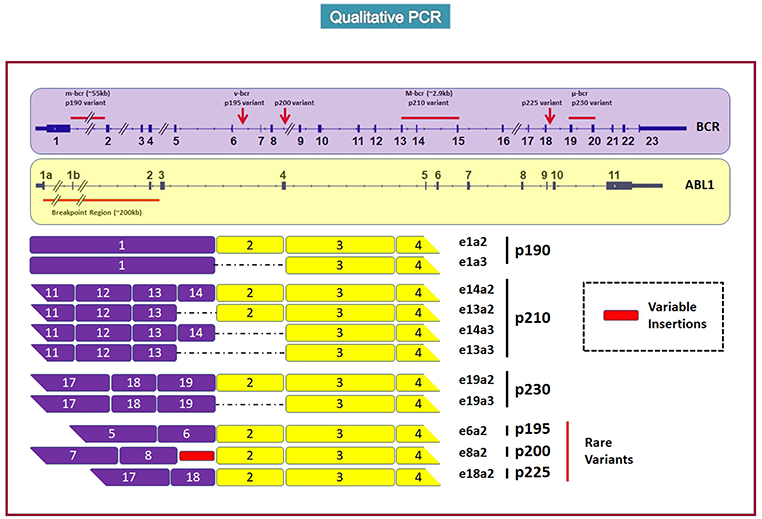
Figure 1. The figure represents the most frequent loci of rupture of the BCR and ABL1 genes (up part) and the consequently origined proteins (P190, P210, P230, rare rearrangements) taken from Weerkamp et al. (7).
BCR/ABL1 oncoproteins are constitutively active tyrosine kinases that promote the activation of different transduction pathway signals involved in cell growth and differentiation (RAS, RAF, JUN, MYC, STAT, AKT), able, therefore, to transform the hematopoietic stem cell in a neoplastic clone (8–11).
Many studies tried to describe the incidence of these different transcripts and to understand if proteins of different lengths might be responsible for different “phenotypes” of the disease. Recently, the prevalence of b2a2, b3a2 and the rare rearrangements have been assessed in more than 45,000 CML cases from 45 countries. b2a2 resulted to be the more frequent transcript (60%), followed by b3a2 (38%) and by “rare” rearrangements (occurring in only 2% of CML cases) (12).
The prognostic role of the different transcripts is still a matter of debate: in the scientific community, some authors support the hypothesis that b2a2 is associated with a lower rate of optimal responses (13–16), whereas other groups did not retrieve any prognostic differences (17). At the ASH meeting 2018, two different groups reported that the b3a2 form, compared to b2a2, seemed to be associated with a higher rate of deep molecular responses (DMR) (18) and of maintaining treatment-free-remission (TFR) (19).
Qualitative PCR is useful for detecting BCR-ABL1 and identifying the type of rearrangement (Figure 2); nevertheless, the type of encoded protein does not yet have a role in clinical practice, nor does it influence the choice of the first-line therapy. Conversely, the management of the CML patients is based on quantitative polymerase chain reaction (RQ-PCR), which allows us to stratify patients into “optimal responders” (who will continue the same treatment), “failed patients” (who need to immediately change therapy), and “warning cases” (who have to be closely followed to evaluate if and when to change the treatment), according to guidelines edited by the European Leukemia Network (ELN) (20, 21) or by the National Comprehensive Cancer Network NCCN (22).
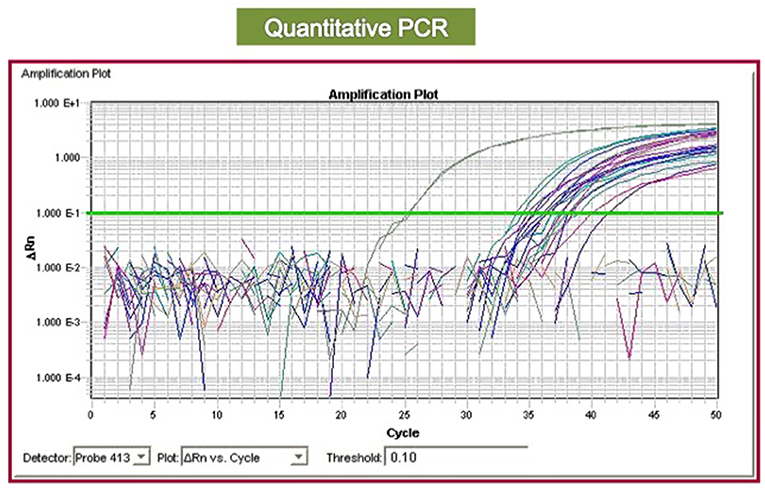
Figure 2. Quantitative PCR plot (RQ-PCR): the real-time amplification allows to measure the quantity of BCR-ABL1 transcript by using a reference curve. By the measure of the threshold cycle (the cycle corresponding to the point where the amplification signal overcomes the background) is possible to calculate the concentration of each sample.
Real-Time Quantitative PCR: Its Fundamental Role in Management of CML Patients
Molecular monitoring plays an essential role in the clinical management of CML patients, leading the majority of clinical decisions.
After the introduction of the tyrosine kinase inhibitors (TKIs) in the treatment of CML, RQ-PCR became the gold standard to follow the disease burden reduction kinetics and to allow an accurate prognostic stratification.
In the IRIS trial (the study that allowed imatinib to enter into the clinical routine as a “magic bullet against cancer”), RQ-PCR showed that the reduction of the BCR-ABL1/ABL1 ratio of at least 3 logarithms by 18 months represented an added value to the complete cytogenetic response (CCyR) in terms of long-term survival prediction (23). Interestingly, the “molecular load” significantly impacted on the 5-year event-free survival (EFS), which was 95% for cases in major molecular response (MMR = 3 logs of reduction) vs. 86% for cases with BCR-ABL1/ABL1 ratio between 0.1 and 1%, 62% for patients with BCR-ABL1/ABL1 ratio between 1% and 10%, and 58% for cases who still presented after 18 months a BCR-ABL1/ABL1 ratio >10% (23, 24). Moreover, cases with a sub-optimal response at 18 months (no MMR) had a significantly higher risk of losing CCyR (24 vs. 0%) (25), thus highlighting the fundamental need of an accurate and reliable molecular monitoring.
The continuous therapeutic improvement of the last 10 years has led to increasingly ambitious treatment endpoints (now culminating in the possibility of achieving TFR), which, in turn, need more and more refined definitions of DMR levels, corresponding to a reduction of the BCR-ABL1/ABL1 ratio of more than 4 logs (26, 27).
At an initial stage, the molecular quantitative approach was “coarse”: the 3 laboratories responsible for the molecular monitoring of patients enrolled in the IRIS trial, decided to mix 30 samples as “basal” (considered to carry 100% of the fusion gene); the reduction of the transcript was then measured from this value in logarithms (MR3 or MMR: reduction of BCR-ABL1/ABL1 ratio of 3 logs = 0.1%; MR4: reduction of BCR-ABL1/ABL1 ratio of 4 logs = 0.01%, MR4.5: reduction of BCR-ABL1/ABL1 ratio of 4.5 logs = 0.0032%; MR5: reduction of BCR-ABL1/ABL1 ratio of 5 logs = 0.001%) (28).
Moreover, until 2005 the quantification was still relative in the majority of labs: in fact, the transcript measured after 3 months of treatment was compared to the measurement initially obtained at diagnosis; the transcript was then measured after 6 months and compared to that of the third month and so on. It was a very difficult and time-consuming approach, especially for physicians. After that, the quantification became obsolete due to the introduction of a reference curve or of specific standards in the PCR reaction, which was definitely a success, but the standardization of the molecular tests was necessary to allow us to compare results deriving from different laboratories, thus introducing molecular response as the primary objective of the clinical trials.
In the scenario, in June 2007, collaboration between ELN and Novartis allowed the creation of the EUropean Treatment Outcome Study (EUTOS) consortium, with the purpose to create an international CML registry, standardize the molecular monitoring, design a path to cure, and become a network of excellence, able to optimize treatment of CML patients and to promote the cooperation among hematologists and scientists across all of Europe. Thirty-eight laboratories from 15 different countries began a process of methodological standardization of the BCR-ABL1 measure and performed periodic quality controls (https://www.eutos.org/content/home/index_eng.html). Among these laboratories, 3 Italian centers were also present (Naples, Orbassano-Turin and Bologna), which started to spread the European project across Italy: in 2008, the Italian network, initially called “X-file,” and then “LabNet,” began its activity, with the aim of creating a network of molecular laboratories distributed throughout the national territory, which could manage the molecular response in CML, for the benefit of all Italian clinical centers. The project included a series of educational meetings, where the methodological aspects and the clinical significance of the evaluation of the residual disease were discussed and presented, with the purpose of rapidly expanding the importance of the molecular monitoring of CML in the TKIs era. We have to consider that at that time, although the prognostic role of the molecular response was evident, the technologies in use were still under discussion. The molecular investigations were carried out only in a few centers all over the world, and that monitoring was recommended to always be carried out by the same laboratory. In the same years, the methodological characteristics had been the subject of a “consensus conference” held in Washington in October 2005, where the technical characteristics were established for an optimal monitoring of the residual disease. A series of recommendations were discussed to harmonize the methodologies used in various laboratories by introducing a conversion factor (the International Scale, IS), which then became the standard to express results (29).
The Italian network initially started in 14 centers; at first it was decided to use a single methodology and a single technological platform in all laboratories, and the results clearly suggested that under controlled conditions the results were very reproducible, thus paving the way to the use of RQ-PCR in clinical routine. The network grew up with a dual purpose: to diffuse, in a progressively larger group of Italian laboratories, the new technologies, and to give diagnostic support in clinical studies designed by the Italian cooperative group “GIMEMA.” A dedicated software is used as a communication tool between clinical centers and laboratories, and a platform harvesting all regional databases on CML was created. Nowadays, LabNet consists of 55 laboratories that regularly participate in activities (such as training, methodological updating and quality controls) and constitute a valid and recognized diagnostic support for the whole country. Today, LabNet, thanks to constant development of its web platform, is the interface where clinicians and biologists collaborate in the management of the CML patients; moreover, LabNet allows clinicians to evaluate RQ-PCR as an accurate surrogate marker of response and is also a valid tool for assessing the patients' adherence to treatment. In 2017, as a result of the great effort of the Italian community, more than 33,000 RQ-PCR tests for BCR-ABL1 have been performed, all laboratories were able to reach and maintain the MR4, and 69% of them attained the MR4.5, the backbone for a safe TFR (http://www.gimema.it/labnet-cml/) (Figure 3).
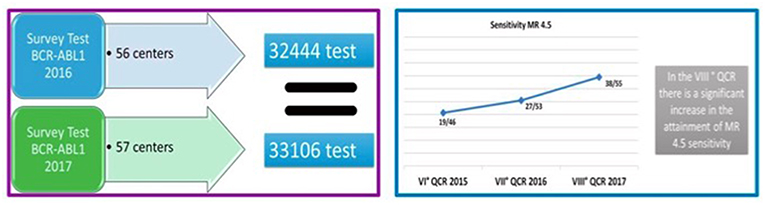
Figure 3. Increasing number of laboratories of the Italian LabNet network being able to attain a sensitivity level of MR 4.5 (BCR-ABL1/ABL1 ratio = 0.0032%).
Currently, in the field of RQ-PCR, there remain some debated issues: (1) if and how strict the molecular monitoring should be to help physicians' decision in order to do the “early switch” (change of TKI in cases not reaching a BCR-ABL1/ABL1 ratio ≤ 10% at 3 months); (2) whether or not the molecular techniques are really able to assess the stable DMR, a fundamental pre-requisite for TFR; 3) whether the definition of different “molecular classes” is reliable, which would allow physicians to offer TFR to the “optimal candidates” only, thus reducing the probability of TFR loss.
Concerning the role of the molecular monitoring by RQ-PCR and the “early switch,” the English group was the first one showing that BCR-ABL1/ABL1 ratio at 3 months <9.84% was associated with a longer overall survival (OS) (8-year OS, 93% for cases with ratio <9.84 vs. 57% for those with a ratio >9.84%) (29). The German group found similar results and the CML IV study confirmed a significant difference in terms of 5-year OS between cases with BCR-ABL1/ABL1 ratio lower or higher than 10% at 3 months (94 vs. 87%) (30). Interestingly, the predictive role of the Early Molecular Response (EMR), a reduction of at least 1 log in respect of diagnosis at 3 months of treatment, was effective not only for imatinib, but also when second-generation TKIs (dasatinib and nilotinib) were employed: in the ENESTnd trial, for example, where nilotinib was compared to imatinib as first-line treatment, EMR offered a clear advantage in terms of MR3 achievement and a lower probability of disease progression (31). The same results were obtained with dasatinib, both in terms of MR3 rate at 24 months (76% for cases with EMR vs. 16% for those with BCR-ABL1/ABL1 ratio at 3 months >10%) and of 3-year OS (96 vs. 86%) (32).
Overall, the data reported above, even if retrospective, concur in sustaining the fundamental role of EMR; but what would happen to patients not in EMR if clinicians would wait for the molecular result at the 6 month instead of changing TKI just at the third month? The Canadian group measured the 3-year freedom from treatment failure rate for patients that at 3 months were not in EMR and who recovered or for patients who did not show the optimal response at the sixth month. The worst outcome was observed for cases with a transcript persistently >10% at the sixth month; nevertheless, no differences were observed between cases already “optimal” at 3 months and those who became “optimal” only after 6 months. This suggests that PCR values at 6 months might be more relevant than those from the third month, at least in the imatinib setting (33).
At the ASH meeting held in 2018, the matter of the “early switch” was discussed again: 108 patients in sub-optimal response or failing imatinib were early switched to nilotinib; the authors sustained that the early switch (change at the third month) offered a significant advantage in terms of MR3 at 2 years (78% of cases who switched to nilotinib after 3 months, 55% for those who switched between 3 and 12 months and 30% for patients who switched after 12 months or later) (34). At the same meeting, the results from the DASCERN trial were also presented, where patients not in EMR were randomized to switch to dasatinib at the third month or to continue imatinib; the MR3 rate at 12 months was significantly higher for cases who immediately switched to dasatinib (29 vs. 13%), even if the 3-year OS and progression-free survival (PFS) were the same in the two cohorts (35).
Some authors suggested as a possible solution to this debate the measure of the slope of the BCR-ABL1 transcript reduction: indeed, in the group of cases with a BCR-ABL1/ABL1 ratio >10% at 3 months, the outcome was better for those who showed a halving time <79 days, that could represent a favorable reduction kinetics that could prelude to the achievement of the optimal response at the second time-point (6 months) (36, 37). Similarly, another promising approach could be the measurement of BCR-ABL1 transcript after only 4 weeks of treatment: in a series of 258 cases, the Receiver Operating Characteristic (ROC) curve showed that patients with a BCR-ABL1/ABL1 ratio <41% after 1 month of therapy had higher probabilities of achieving the optimal response at 3 months, DMR (56 vs. 29%) and presented longer EFS (93 vs. 85%) (38).
Regarding the other two main issues—the ability of RQ-PCR to prove the stability of DMR, and the reliability of the correct definition of the “molecular classes,” we have to consider that, although RQ-PCR is always used to monitor the response to TKIs, it may not be the best approach when the issue is TFR followed by molecular relapse in half of patients who had profound and long-lasting molecular responses at the time of TKI discontinuation (39–41). In fact, to date, there is some evidence that we cannot accurately and reproducibly monitor those patients who are able to stay out of treatment indefinitely. This is probably due to several factors, such as the limit of detection of our molecular monitoring technology, or the inherent differences in the biology of leukemia (42), and/or immune response (43) in different patients, or a combination of several still undefined factors.
Indeed, among the methodological limitations of the RQ-PCR, there may be sampling errors, low sensitivity, or low/absent transcriptional levels of BCR-ABL1 in the CML leukemic stem cell (LSC) that, when hidden in the hypoxic niche, does not synthetize the tyrosine kinase protein while it retains the fusion gene at DNA and RNA levels (44). For these reasons, researchers are always looking for increasingly sensitive techniques able to detect, with better accuracy and precision, the residual leukemic cells and to allow early identification of the patients who might be more likely to benefit from TFR.
The demonstration that the measure of the BCR-ABL1 transcript is not sufficient to identify the perfect candidate for TFR treatment comes from a recent work that compared the number of LSCs measured in the peripheral blood by flow cytometry (as CD34+/CD38-/CD26+ population) with that measured by RQ-PCR. On a series of 400 patients, the absolute value of LSCs, either measured on peripheral blood or on bone marrow samples, did not correlate with the BCR-ABL1/ABL1 ratio measured by RQ-PCR in peripheral blood, with about 30% of patients with undetectable BCR-ABL1 transcript levels still showing circulating CD26+ LSCs. Perhaps, molecular response could be considered as a picture of the transcriptionally active progenitor cells only (45).
Another “technical” hypothesis that could explain why half of patients rapidly lose TFR, is that there might be a mistake in categorizing patients; in other words, we would offer TFR to cases who are not in a real and persistent optimal response. This issue could perhaps be resolved by a new quantitative PCR, the digital PCR (dPCR).
The Third Generation of Quantitative PCR: The Digital PCR
Several publications reported that dPCR is better at detecting MRD compared to RQ-PCR (46, 47), and that it provides an improved precision even at low BCR-ABL1 transcript levels (46).
Today, dPCR represents one of the techniques that could be used for MRD monitoring, as it simplifies the standardization process and improves the sensitivity and precision of quantitative measurements. This method has already been employed in the hematological field by assessing mutations of JAK2 (48), B-RAF (49), DNMT3A (50) or by quantitating some fusion genes, such as PML-RARα (51) or BCR-ABL1 (52) or by testing the rearrangement of the immunoglobulin heavy chain (IGH) (53).
In the classical RQ-PCR, an absolute standard curve with known amounts of BCR-ABL1 is used to extrapolate the quantity of unknown samples by comparison between the amplification cycles of the standard references and of the samples (54). Nevertheless, the amplification may not always be perfectly efficient or variable, with, eventually, a lower sensitivity (55).
dPCR offers an advantage over the conventional PCR, because samples are divided into thousands or millions of nanoliter reactions and amplification is performed in many spatially separated microscopic wells (the droplets), with higher efficiency (56) (Figure 4).
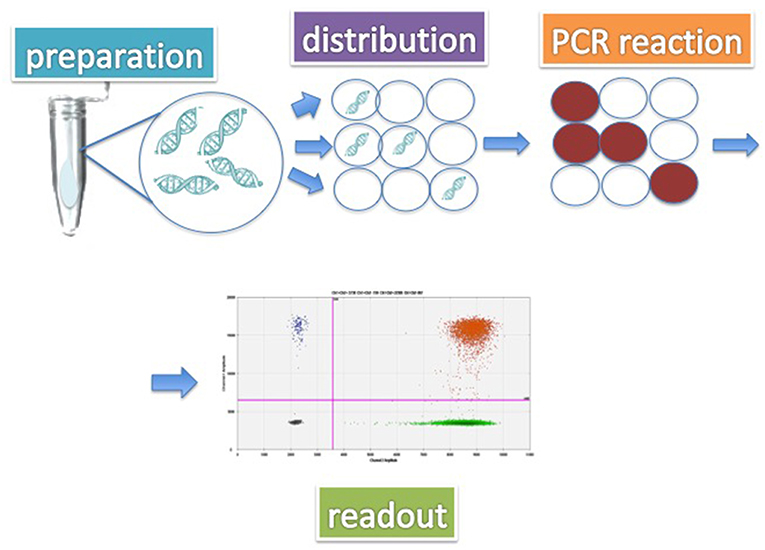
Figure 4. Digital PCR (dPCR) overview. The fundamental phases of the process are represented: (1) partitioning of the sample in thousand of drops; (2) amplification; (3) output and digital analysis.
The purpose of dPCR is to have one template molecule in each partition; therefore, after amplification, each partition can be evaluated as “positive” or “negative” (digital reading). Consequently, the absolute quantity of molecules can be determined without the need for a standard curve. One of the main advantages of dPCR is the precise quantification of the nucleic acids, which facilitates the measurement of small percentage differences; overall, dPCR has the potential to detect the presence of the BCR-ABL1 transcript with greater sensitivity and precision than RQ-PCR (57).
Several factors must be considered in the application of dPCR in CML: first, the “clinical” vs. the “analytical” sensitivity, which is expressed as the limit of detection (LoD) of an analyte, that indicates the lowest concentration detected with certainty at 95% of confidence interval (58). However, the “clinical” sensitivity of a test is defined as its ability to determine a log reduction of BCR-ABL1/ABL1 ratio (or ratio between BCR-ABL1 and another reference gene) expressed internationally compared to the baseline. When “clinical” sensitivity is considered, three factors must be taken into account: (1) dPCR remains susceptible to pre-analytical errors upstream of the process, such as sampling, RNA extraction and cDNA synthesis, as already occurs for RQ-PCR; (2) the quantification of the reference gene is still necessary to evaluate the processing qualities of the sample and the pre-PCR phases; (3) the use of a conversion factor remains a fundamental requirement for the expression of results according to the international scale, which is, in turn, the basis for the classification of molecular responses.
As reported in other hematological malignancies, such as non-Hodgkin's lymphomas (59) and acute lymphoblastic leukemia (60), similarly in CML dPCR may be more sensitive than RQ-PCR, but it is always susceptible to the specific assay design, pre-PCR processing and molecular dropout (target not detected despite being present in the reaction). Moreover, positive and negative controls are needed to evaluate false negative and false positive samples and to define the quantification thresholds.
The application of dPCR for the molecular monitoring in CML is a growing area of research: initial studies using both nanofluidic approach and dPCR showed that dPCR can measure transcript below 0.01% (46, 47), but not without some false negative results. An interesting study reported a robust quantification of BCR-ABL1 levels from 0.0032% (MR4.5) to 10% across three different dPCR platforms: Bio-Rad QX200, RainDance RainDrop System, and Applied Biosystems QuantStudio3D. This study successfully detected BCR-ABL1 down to MR5, thus demonstrating that dPCR approach was able to increase sensitivity detection levels (61) (Figure 5).
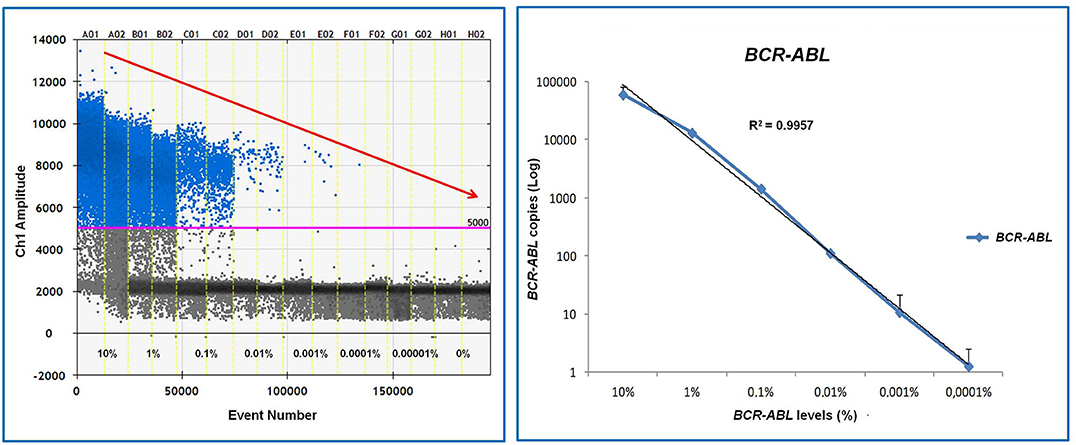
Figure 5. Assessment of detection performance of dPCR system in RNA samples obtained from serial dilutions of Ph'+ cells in Ph'-cells. As shown, dPCR is able to detect BCR-ABL1 mRNA up to 0.001% (MR5).
However, another study reported that BCR-ABL1 values between MR3 and MR5 generated by the Bio-Rad QX200 system were consistently higher than values generated by conventional RQ-PCR (62). Nevertheless, the concordance between dPCR and RQ-PCR was good, with values between 89 and 97%, according to the employed platform (61). Other authors confirmed the high sensitivity of dPCR: in the MR4.5 class, 76% of cases resulting negative by RQ-PCR were still positive by dPCR when the cut off for positivity was set at 3 droplets (63, 64). Moreover, at the European Hematology Association (EHA) meeting held in 2018, it has been reported that 16% of the cases enrolled in the EURO SKI trial, who did not show any BCR-ABL1 transcript when QR-PCR was performed on cDNA, resulted BCR-ABL1-positive when dPCR was performed on genomic DNA. Therefore, leaving the question unanswered as to what would be the best informative compartment for MRD assessment in CML (65).
Similarly, in another series of 230 patients enrolled in the ENEST first trial, 10% of cases defined as in MR4 by RQ-PCR resulted in MR3 by dPCR (62); this induces to hypothesize that at least one quarter of CML cases who lost TFR were effectively cases that had not been adequately scored by RQ-PCR. Different groups tried to identify a specific BCR-ABL1 value that would be significantly associated to the TFR loss; Nicolini et al. showed that the loss of TFR was conditioned by the duration of treatment with Imatinib (longer than 75 months was favorable) and by a cut off of 0.023% (measured by dPCR) (66). Another group reported that the probability of losing TFR was 53% in the cohort of cases, showing BCR-ABL1/ABL1 ratio >0.468 vs. 17% in the subgroup where the transcript values were <0.468 (67). Finally, age higher than 45 years and positive d-PCR at the moment of imatinib discontinuation were the two factors able to condition the loss of TFR in the ISAV study (68).
In conclusion, d-PCR seems to be a good, novel, sensitive and accurate tool for the molecular monitoring in CML; nevertheless, an international standardization is necessary before this technique enters routine molecular methods.
Next Generation Sequencing (NGS) and its Use in CML
The scenario of the new molecular technologies employed in CML has been recently enriched by the Next Generation Sequencing (NGS); this method is now frequently used for detecting ABL1 kinase domain (KD) mutations, that are one relevant mechanism of CML resistance to TKIs and that account for ~50% of acquired resistance in failing CML cases (69). Indeed, many different single KD mutations have been associated with resistance to imatinib, but also to nilotinib (Y253H, E255K, E255V, F359V, and F359C), dasatinib (V299L, T315A, F317L, F317I, F317V, and F317C) or bosutinib (Y253H, V299L, F317V) (70–77).
Even if these TKI-specific mutations are present in <10% of cases who do not reach the optimal response, it is relevant to identify and characterize them, in order to evaluate what kind of TKI has to be employed at the moment of the switch for resistance.
ABL1 KD mutations are commonly detected by sequencing BCR-ABL1 cDNA according to the Sanger's method. This technique, however, can only detect mutations present in a percentage higher than 10–20% (78) (Figure 6). Other techniques with greater sensitivity, such as High-Performance Liquid Chromatography (HPLC) (78) and mass-spectrometry (79) can identify mutations with a minimal mutation load <1% (Figure 4). In 2004, Soverini et al. (78) developed a new HPLC-based assay that they compared to the conventional Sanger. Both D-HPLC and Sanger detected ABL1 mutations in 12/27 patients; nevertheless, 2 cases, that have been scored as wild-type by Sanger, resulted mutated by HPLC. In 2010, Placzek et al. (79) set a novel biosensor in a cellular model for measuring ABL1 kinase activity; this sensitive method represented a new tool for mutational screening. Indeed, it was able to identify mutations by measuring ABL1 kinase activity that resulted reduced in mutated vs. the wild-type samples.
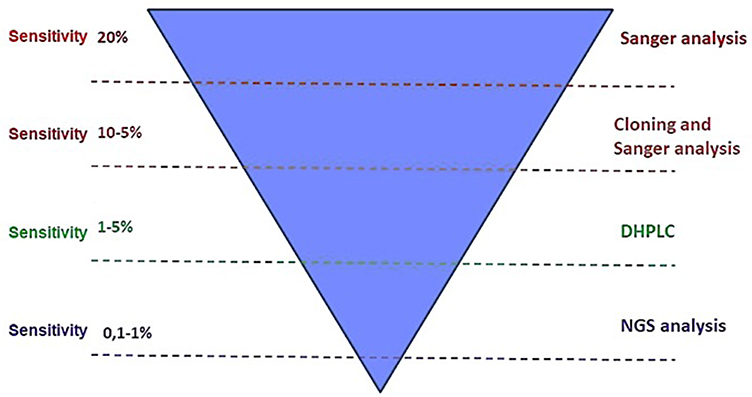
Figure 6. Sensitivity of different molecular biology methods for ABL1 kinase domain mutations detection: the deepest sensitivity is reached by Next Generation Sequencing (NGS) tools.
The “NGS” definition collectively refers to some high-throughput methods that can sequence large numbers of RNA or DNA molecules and can be digitally tabulated. These platforms result in very high numbers of sequencing reads and allow for discovery of sequences occurring at lower frequencies, even when observed in a heterogeneous background. An important feature of this technology is the massive quantity of information generated, and consequently, sophisticated bioinformatics and computational methods are required for analysis. Compared to the Sanger sequencing, which is characterized by a limit of sensitivity of approximately 10–20%, NGS reaches sensitivities of 1–10% and may reveal a complex clonal architecture consisting of a dynamic mix of polyclonal and compound mutations (80–82).
NGS includes two different strategies: (1) the “target capture” via probe hybridization or ligation, and (2) the “amplicon based enrichment” (ultra-deep sequencing [UDS] or the “amplicon” deep sequencing [ADS]). Hybridization-based NGS uses synthetic oligonucleotides specifically designed to target BCR and ABL1, followed by sequencing. Fusion junctions are predicted using bio-informatic packages designed to identify structural variants, including chromosome translocations, amplifications, inversions and deletions. These packages usually produce one or both types of reads: (1) split reads, that are single reads composed of material from two non-contiguous genomic regions directly mapping a fusion junction to a base pair resolution; and (2) discordant pairs of reads, in which there are individual reads in a pair map to different chromosome locations, indicating the presence of a structural rearrangement within the insert between them. When a sample has an expression level of BCR-ABL1 >10%, a mean read-depth of 50x is sufficient to map the fusion junction. The mapped genomic fusion junctions of each patient are unique and can be used as patient–specific markers for MRD monitoring if DNA or RNA at diagnosis are available. Amplicon deep-sequencing method utilizes a highly multiplexed amplicon generation strategy where multiple regions of interest are amplified and then sequenced at a depth of 100–10,000-fold greater than that offered by Sanger sequencing. Dedicated softwares assemble, align and map the sequenced reads to the reference sequence and perform variant detection. The quantitative feature is gained by sequencing targeted regions at depths of hundreds or thousands of reads, allowing the sensitive detection and quantification of rare events. ADS has been used for sensitive quantification of ABL1 mutations (83–86); overlapping primers designed to cover the KD within the fusion BCR-ABL1 transcript were used to amplify the domain by a nested PCR approach to enrich it for the fusion transcript. Amplicons from multiple samples are barcoded and clonally amplified for sequencing. Sequencing the amplicons on a high throughput platform allows sufficient depth of coverage (≥2,000 reads) per base to identify mutations at very high sensitivity (<1%).The application of amplicon deep sequencing for monitoring the genomic fusion junctions as a molecular marker is also a plausible quantification approach that is usually used to detect and track clonally-expanded lymphocytic populations in acute lymphoblastic leukemia (87, 88) and other malignancies (89–93). Whether amplicon deep-sequencing can compete with the sensitivity achieved by quantitative methods, such as RQ-PCR or d-PCR, is still unknown.
From the clinical point of view, it seems that ABL1 mutations could also be responsible for resistance when present at a low level, and that detection of multiple KD mutations is associated with an inferior response rate compared to patients with none or with single mutations (94).
Compound mutations (more than one mutation in a single molecule) have also emerged as a potential driver of acquired resistance to first- and second-generation TKIs, and show different resistance profiles compared with individual mutations, some of them also being resistant to ponatinib, the third-generation TKI able to overcome the negative prognostic impact of the majority of the ABL1 mutations, including the T315I (95). Compound mutations being resistant to ponatinib is still matter of debate: indeed, some authors sustain that compound mutations are always resistant (96, 97), whereas, others have demonstrated that only few combinations are really resistant to ponatinib. In particular, at the last ASH meeting, Soverini et al. (98) showed that compound mutations characterize only 3.5% of the chronic phases, 32% of the accelerate/blastic cases and 37% of cases progressed into Ph'-positive acute lymphoblastic leukemia. The most accurate and sensitive molecular method for detecting these compound mutations seems to be NGS; however, some studies conveyed that sequencing artifacts and PCR recombination can also lead to some, even if rare, false-positive compound mutations, with a possible over-estimation (99).
On the other hand, novel advances and refinements of the NGS-based sequencing approaches can be interesting: for example, the incorporation of unique molecular identifier tags into the NGS protocol demonstrated that sequencing errors can be identified and removed from analysis (94), resulting in the ability to distinguish compound mutations from PCR-artifacts or polyclonal mutations (different mutations on different CML cells). Thus, Deininger et al. (83) devised an algorithm to predict false-positive compound-mutation: this algorithm was based on the recombination rate, which was determined by measuring the frequency at which false-compound mutations were generated when RNA samples from two patients, each with already known distinct single ABL1 mutations, were mixed. Only compound mutations with an observed frequency greater than the false-compound mutation rate were considered “true” compound mutations.
Notwithstanding NGS is not yet fully standardized, as previously occurred for the RQ-PCR, an international attempt of harmonization has been performed in the last few years: the IRON II study was conducted by a consortium of 10 laboratories from 8 countries engaged in the standardization and validation of a common protocol for ABL1 mutation screening: a concordance between Sanger and NGS was found in 394/398 of the tested samples (100). As for the LabNet, this international project was exported to Italy, where a network of 4 laboratories (Bologna, Catania, Orbassano, Napoli) sharing a common NGS protocol and an optimized pipeline of data analysis was created and shared. With collaboration of 39 Italian clinical centers, 211 “failing” patients were assessed: NGS was able to detect a mutation in 45% of them, in comparison with 20% who resulted mutated by Sanger, and 36% of cases showed “low-burden” mutations. The most relevant finding was that 24% of patients already scored as “wild-type” by Sanger, resulted mutated by NGS. Furthermore, NGS found that mutations showed a clinically relevant profile for the choice of TKI, thus supporting a relevant clinical role for NGS in CML (101).
Conclusions
For many years, the assessment of MRD has been fundamental for better managing of treatment of CML patients with TKIs, and this process has been done in an easier way than in other hematological malignancies because the fundamental pathogenetic mechanism of this disease has been well clarified in the last few years, making the BCR-ABL1 transcript the principal target of all MRD tests (102).
The technique that is commonly adopted for measuring the BCR-ABL1 transcript is the quantitative PCR, which could be performed either by real-time or dPCR; the new published data have shown that dPCR could be more sensitive and accurate than RQ-PCR and, in future, we'll see if dPCR will replace RQ-PCR in the CML context. Moreover, the best target nucleic acid (mRNA or DNA) is still matter of debate, because it has been demonstrated that LSCs also persist in patients achieving DMR and during TFR. The availability of new molecular methods will probably provide the answer to this question.
Another hot field in the CML scenario is represented by the adoption of the NGS tools for evaluating the mutations of the ABL1 kinase domain, these being the mutations responsible for resistance to TKIs either in the chronic or in more advanced disease phases. Until now, the Sanger sequencing has been the more commonly adopted method, but NGS, with its higher sensitivity, is able to detect mutations at a sub-clonal level as well as compound mutations that are responsible for the resistance to ponatinib.
The use of NGS will likely be more frequently adopted for patients who fail the first line of therapy and for those who are in suboptimal response during further treatment lines. In the allogeneic transplantation setting, data produced with NGS are not yet available and Sanger is still the recommended technique.
Overall, in this review we have demonstrated the potential to manage many and new promising molecular tools that can help physicians to design, ab initio, a successful and patient-tailored treatment. Obviously, further harmonization of these molecular techniques by international projects is still necessary to speak a universal language.
It is clear that, the basis of successful management of CML patients will always be the strict collaboration between biologists, technicians, and physicians. The existing and future networks will help all of us to reach this goal.
Author Contributions
BI, EG, and SG wrote the manuscript. SE and FD revised the part of methodologies. CB revised the clinical parts.
Conflict of Interest Statement
The authors declare that the research was conducted in the absence of any commercial or financial relationships that could be construed as a potential conflict of interest.
Acknowledgments
Authors thank all patients and clinicians who believe in networking and in molecular biology, the biologists and technicians working in molecular laboratory of Orbassano, Pisa, Naples, all members of the Scientific Committee of the GIMEMA CML LAbNet, Dr. R. Cucci for her assistance, Dr. P. Rossi and Dr. S. Redihough for their language revision.
References
1. Sandberg AA, Gemmill RM, Hecht BK, Hecht F. The Philadelphia chromosome: a model of cancer and molecular cytogenetics. Cancer Genet Cytogenet. (1986) 21:129–46. doi: 10.1016/0165-4608(86)90039-7
2. Bartram CR, Kleihauer E, de Klein A, Grosveld G, Teyssier JR, Heisterkamp N, et al. C-abl and bcr are rearranged in a Ph1-negative CML patient. EMBO J. (1985) 4:683–6. doi: 10.1002/j.1460-2075.1985.tb03683.x
3. Heisterkamp N, Stam K, Groffen J, de Klein A, Grosveld G. Structural organization of the bcr gene and its role in the Ph' translocation. Nature. (1985) 315:758–61. doi: 10.1038/315758a0
4. Ben-Neriah Y, Daley GQ, Mes-Masson AM, Witte ON, Baltimore D. The chronic myelogenous leukemia-specific P210 protein is the product of the bcr/abl hybrid gene. Science. (1986) 233:212–4. doi: 10.1126/science.3460176
5. Chan LC, Karhi KK, Rayter SI, Heisterkamp N, Eridani S, Powles R, et al. A novel abl protein expressed in Philadelphia chromosome positive acute lymphoblastic leukaemia. Nature. (1987) 325:635–7. doi: 10.1038/325635a0
6. Verstovsek S, Lin H, Kantarjian H, Saglio G, De Micheli D, Pane F, et al. Neutrophilic-chronic myeloid leukemia: low levels of p230 BCR/ABL mRNA and undetectable BCR/ABL protein may predict an indolent course. Cancer. (2002) 94:2416–25. doi: 10.1002/cncr.10490
7. Weerkamp F, Dekking E, Ng YY, van der Velden VH, Wai H, Böttcher S, et al. Flow cytometric immunobead assay for the detection of BCR-ABL fusion proteins in leukemia patients. Leukemia. (2009) 23:1106–17. doi: 10.1038/leu.2009.93
8. De Keersmaecker K, Cools J. Chronic myeloproliferative disorders: a tyrosine kinase tale. Leukemia. (2006) 20:200–5. doi: 10.1038/sj.leu.2404064
9. Quintás-Cardama A, Cortes J. Molecular biology of bcr-abl1-positive chronic myeloid leukemia. Blood. (2009) 113:1619–30. doi: 10.1182/blood-2008-03-144790
10. Håkansson P, Segal D, Lassen C, Gullberg U, Morse HC 3rd, Fioretos T, et al. Identification of genes differentially regulated by the P210 BCR/ABL1 fusion oncogene using cDNA microarrays. Exp Hematol. (2004) 32:476–82. doi: 10.1016/j.exphem.2004.02.012
11. Zhao C, Chen A, Jamieson CH, Fereshteh M, Abrahamsson A, Blum J, et al. Hedgehog signalling is essential for maintenance of cancer stem cells in myeloid leukaemia. Nature. (2009) 458:776–9. doi: 10.1038/nature07737
12. Baccarani M, Castagnetti F, Gugliotta G, Rosti G, Soverini S, Albeer A, et al. International BCR-ABL study group. The proportion of different BCR-ABL1 transcript types in chronic myeloid leukemia. An international overview. Leukemia. (2019) 33:1173–83. doi: 10.1038/s41375-018-0341-4
13. Castagnetti F, Gugliotta G, Breccia M, Iurlo A, Levato L, Albano F, et al. GIMEMA CML working party. The BCR-ABL1 transcript type influences response and outcome in Philadelphia chromosome-positive 0chronic myeloid leukemia patients treated frontline with imatinib. Am J Hematol. (2017) 92:797–805. doi: 10.1002/ajh.24774
14. Hanfstein B, Lauseker M, Hehlmann R, Saussele S, Erben P, Dietz C, et al. Distinct characteristics of e13a2 versus e14a2 BCR-ABL1 driven chronic myeloid leukemia under first-line therapy with imatinib. Haematologica. (2014) 99:1441–7. doi: 10.3324/haematol.2013.096537
15. Pagnano KBB, Miranda EC, Delamain MT, Duarte GO, de Paula EV, Lorand-Metze I, et al. influence of bcr-abl transcript type on outcome in patients with chronic-phase chronic myeloid leukemia treated with imatinib. Clin Lymphoma Myeloma Leuk. (2017) 17:728–733. doi: 10.1016/j.clml.2017.06.009
16. Lucas CM, Harris RJ, Giannoudis A, Davies A, Knight K, Watmough SJ, et al. Chronic myeloid leukemia patients with the e13a2 BCR-ABL fusion transcript have inferior responses to imatinib compared to patients with the e14a2 transcript. Haematologica. (2009) 94:1362–7. doi: 10.3324/haematol.2009.009134
17. Pfirrmann M, Evtimova D, Saussele S, Castagnetti F, Cervantes F, Janssen J, et al. No influence of BCR-ABL1 transcript types e13a2 and e14a2 on long-term survival: results in 1494 patients with chronic myeloid leukemia treated with imatinib. J Cancer Res Clin Oncol. (2017) 143:843–50. doi: 10.1007/s00432-016-2321-2
18. Da Silva MS, Miranda EC, Torresan Delamain M, Oliveira Duarte G, Vergilio BR, Pascoal Lopes AB, et al. B3a2 transcript is an independent factor for the achievement of a deep molecular response in chronic phase - chronic myeloid leukemia patients treated with imatinib in first-line. Blood. (2018) 132:1749. doi: 10.1182/blood-2018-99-114036
19. Shanmuganathan N, Branford S, Yong ASM, Hiwase DK, Yeung DT, Ross DM, et al. The e13a2 BCR-ABL1 transcript is associated with higher rates of molecular recurrence after treatment-free remission attempts: retrospective analysis of the Adelaide cohort. Blood. (2018) 132:1731. doi: 10.1182/blood-2018-99-111083
20. Baccarani M, Deininger MW, Rosti G, Hochhaus A, Soverini S, Apperley JF, et al. European leukemianet recommendations for the management of chronic myeloid leukemia: Blood. (2013) 122:872–84. doi: 10.1182/blood-2013-05-501569
21. Baccarani M, Castagnetti F, Gugliotta G, Rosti G. A review of the European LeukemiaNet recommendations for the management of CML. Ann Hematol. (2015) 94 (Suppl 2):S141–7. doi: 10.1007/s00277-015-2322-2
22. Pallera A, Altman JK, Berman E, Abboud CN, Bhatnagar B, Curtin P, et al. NCCN guidelines insights: chronic myeloid leukemia, version 1. (2017). J Natl Compr Canc Netw. (2016) 14:1505–12. doi: 10.6004/jnccn.2016.0162
23. Hughes TP, Hochhaus A, Branford S, Müller MC, Kaeda JS, Foroni L, et al. Long-term prognostic significance of early molecular response to imatinib in newly diagnosed chronic myeloid leukemia: an analysis from the International Randomized Study of Interferon and STI571 (IRIS). Blood. (2010) 116:3758–65. doi: 10.1182/blood-2010-03-273979
24. O'Brien SG, Guilhot F, Larson RA, Gathmann I, Baccarani M, Cervantes F, et al. Imatinib compared with interferon and low-dose cytarabine for newly diagnosed chronic-phase chronic myeloid leukemia. N Engl J Med. (2003) 348:994–1004. doi: 10.1056/NEJMoa022457
25. Marin D, Milojkovic D, Olavarria E, Khorashad JS, de Lavallade H, Reid AG, et al. European LeukemiaNet criteria for failure or suboptimal response reliably identify patients with CML in early chronic phase treated with imatinib whose eventual outcome is poor. Blood. (2008) 112:4437–44. doi: 10.1182/blood-2008-06-162388
26. Mahon FX. Discontinuation of TKI therapy and 'functional' cure for CML. Best Pract Res Clin Haematol. (2016) 2016 308–313. doi: 10.1016/j.beha.2016.10.014
27. Takahashi N, Tauchi T, Kitamura K, Miyamura K, Saburi Y, Hatta Y, et al. Deeper molecular response is a predictive factor for treatment-free remission after imatinib discontinuation in patients with chronic phase chronic myeloid leukemia: the JALSG-STIM213 study. Int J Hematol. (2018) 107:185–93. doi: 10.1007/s12185-017-2334-x
28. Cross NC, White HE, Colomer D, Ehrencrona H, Foroni L, Gottardi E, et al. A. Laboratory recommendations for scoring deep molecular responses following treatment for chronic myeloid leukemia. Leukemia. (2015) 29:999–1003. doi: 10.1038/leu.2015.29
29. Marin D, Ibrahim AR, Lucas C, Gerrard G, Wang L, Szydlo RM, et al. Assessment of BCR-ABL1 transcript levels at 3 months is the only requirement for predicting outcome for patients with chronic myeloid leukemia treated with tyrosine kinase inhibitors. J Clin Oncol. (2012) 30:232–8. doi: 10.1200/JCO.2011.38.6565
30. Hanfstein B, Müller MC, Hehlmann R, Erben P, Lauseker M, Fabarius A, et al. Early molecular and cytogenetic response is predictive for long-term progression-free and overall survival in chronic myeloid leukemia (CML). Leukemia. (2012) 2096–102. doi: 10.1038/leu.2012.85
31. Hughes TP, Saglio G, Kantarjian HM, Guilhot F, Niederwieser D, Rosti G, et al. A Early molecular response predicts outcomes in patients with chronic myeloid leukemia in chronic phase treated with frontline nilotinib or imatinib. Blood. (2014) 123:1353–60. doi: 10.1182/blood-2013-06-510396
32. Jabbour E, Kantarjian HM, Saglio G, Steegmann JL, Shah NP, Boqué C, et al. Early response with dasatinib or imatinib in chronic myeloid leukemia: 3-year follow-up from a randomized phase 3 trial (DASISION). Blood. (2014) 123:494–500. doi: 10.1182/blood-2013-06-511592
33. Kim DD, Hamad N, Lee HG, Kamel-Reid S, Lipton JH. BCR/ABL level at 6 months identifies good risk CML subgroup after failing early molecular response at 3 months following imatinib therapy for CML in chronic phase. Am J Hematol. (2014) 89:626–32. doi: 10.1002/ajh.23707
34. Viboonjuntra P, Lekhakula A, Chansung K, Sirijerachai C, Niparuck P, Pauvilai T, et al. Impact of early switching to nilotinib as second line tki in patients with chronic myeloid leukemia who were intolerant to, suboptimal to and failed imatinib: the thai multi-centered study. Blood. (2018) 132:4267. doi: 10.1182/blood-2018-99-117787
35. Cortes JE, Jiang Q, Wang J, Weng J, Zhu H, Xiaoli L, et al. Dasatinib versus imatinib in patients (Pts) with chronic myeloid leukemia in chronic phase (CML-CP) who have not achieved an optimal response to 3 months of im/atinib therapy: dascern. Blood. (2018) 132:788.
36. Branford S, Yeung DT, Parker WT, Roberts ND, Purins L, Braley JA, et al. Prognosis for patients with CML and >10% BCR-ABL1 after 3 months of imatinib depends on the rate of BCR-ABL1 decline. Blood. (2014) 124:511–8. doi: 10.1182/blood-2014-03-566323
37. Fava C, Rege-Cambrin G, Dogliotti I, Gottardi E, Berchialla P, Di Gioacchino B, et al. early bcr-abl1 reduction is predictive of better event-free survival in patients with newly diagnosed chronic myeloid leukemia treated with any tyrosine kinase inhibitor. Clin Lymphoma Myeloma Leuk. (2016) 16 (Suppl:) S96–S100. doi: 10.1016/j.clml.2016.03.008
38. Song HY, Noh H, Choi SY, Lee SE, Kim SH, Kee KM, et al. BCR-ABL1 transcript levels at 4 weeks have prognostic significance for time-specific responses and for predicting survival in chronic-phase chronic myeloid leukemia patients treated with various tyrosine kinase inhibitors. Cancer Med. (2018) 10:5107–17. doi: 10.1002/cam4.1753
39. Cortes J, Rea D, Lipton JH. Treatment-free remission with first- and second-generation tyrosine kinase inhibitors. Am J Hematol. (2019) 94:346–357. doi: 10.1002/ajh.25342
40. Guru Murthy GS, Atallah E. Treatment-free remission in CML: the US Perspective. Curr Hematol Malig Rep. (2019) 14:56–61. doi: 10.1007/s11899-019-0496-8
41. Breccia M, Foà R. Current information and recommendations on the discontinuation of TKI inhibitors in chronic myeloid leukemia. Curr Oncol Rep. (2018) 20:23. doi: 10.1007/s11912-018-0669-y
42. Rinaldetti S, Pfirrmann M, Manz K, Guilhot J, Dietz C, Panagiotidis P, et al. Effect of ABCG2, OCT1, and ABCB1 (MDR1) gene expression on treatment-free remission in a EURO-SKI subtrial. Clin Lymphoma Myeloma Leuk. (2018) 18:266–271. doi: 10.1016/j.clml.2018.02.004
43. Caocci G, Martino B, Greco M, Abruzzese E, Trawinska MM, Lai S, et al. Killer immunoglobulin-like receptors can predict TKI treatment-free remission in chronic myeloid leukemia patients. Exp Hematol. (2015) 43:1015–18.e1. doi: 10.1016/j.exphem.2015.08.004
44. Rovida E, Marzi I, Cipolleschi MG, Dello Sbarba P. One more stem cell niche: how the sensitivity of chronic myeloid leukemia cells to imatinib mesylate is modulated within a “hypoxic” environment. Hypoxia (Auckl). (2014) 2:1–10. doi: 10.2147/HP.S51812
45. Bocchia M, Sicuranza A, Abruzzese E, Iurlo A, Sirianni S, Gozzini A, et al. Residual peripheral blood CD26+ leukemic stem cells in chronic myeloid leukemia patients during TKI therapy and during treatment-free remission. Front Oncol. (2018) 8:194. doi: 10.3389/fonc.2018.00194
46. Goh HG, Lin M, Fukushima T, Saglio G, Kim D, Choi S, et al. Sensitive quantitation of minimal residual disease in chronic myeloid leukemia using nanofluidic digital polymerase chain reaction assay. Leuk Lymphoma. (2011) 52:896–904. doi: 10.3109/10428194.2011.555569
47. Jennings LJ, George D, Czech J, Yu M, Joseph L. Detection and quantification of BCR-ABL1 fusion transcripts by droplet digital PCR J Mol Diagn JMD. (2014)16:174–9. doi: 10.1016/j.jmoldx.2013.10.007
48. Fontanelli G, Baratè C, Ciabatti E, Guerrini F, Grassi S, Del Re M, et al. Real-Time PCR and droplet digital PCR: two techniques for detection of the JAK2(V617F) mutation in Philadelphia-negative chronic myeloproliferative neoplasms. Int J Lab Hematol. (2015) 37:766–73. doi: 10.1111/ijlh.12404
49. Guerrini F, Paolicchi M, Ghio F, Ciabatti E, Grassi S, Salehzadeh S, et al. The droplet digital PCR: a new valid molecular approach for the assessment of B-RAFV600E mutation in hairy cell leukemia. Front Pharmacol. (2016) 13:363. doi: 10.3389/fphar.2016.00363
50. Brambati C, Galbiati S, Xue E, Toffalori C, Crucitti L, Greco R, et al. Droplet digital polymerase chain reaction for DNMT3A and IDH1/2 mutations to improve early detection of acute myeloid leukemia relapse after allogeneic hematopoietic stem cell transplantation. Haematologica. (2016) 101:e157–61. doi: 10.3324/haematol.2015.135467
51. Brunetti C, Anelli L, Zagaria A, Minervini A, Minervini CF, Casieri P, et al. Droplet digital PCR is a reliable tool for monitoring minimal residual disease in acute promyelocytic leukemia. J Mol Diagn. (2017). 19:437–44. doi: 10.1016/j.jmoldx.2017.01.004
52. Lund HL, Hughesman CB, McNeil K, Clemens S, Hocken K, Pettersson R, et al. Initial diagnosis of chronic myelogenous leukemia based on quantification of M-BCR status using droplet digital PCR. Anal Bioanal Chem. (2016) 408:1079–94. doi: 10.1007/s00216-015-9204-2
53. Drandi D, Ferrero S, Ladetto M. Droplet digital PCR for minimal residual disease detection in mature lymphoproliferative disorders. Methods Mol Biol. (2018) 1768:229–56. doi: 10.1007/978-1-4939-7778-9_14
54. Branford S, Hughes TP, Rudzki Z. Monitoring chronic myeloid leukaemia therapy by real-time quantitative PCR in blood is a reliable alternative to bone marrow cytogenetics. Br J Haematol. (1999) 107:587–99. doi: 10.1046/j.1365-2141.1999.01749.x
55. Freeman W, Walker S, Vrana K. Quantitative RT-PCR: pitfalls and potential. Biotechniques. (1999) 26: 124–125. doi: 10.2144/99261rv01
56. Vogelstein B, Kinzler K. Digital PCR. Proc Natl Acad Sci USA. (1999) 96:9236–241. doi: 10.1073/pnas.96.16.9236
57. Whale AS, Huggett JF, Cowen S, Speirs V, Shaw J, Ellison S, et al. Comparison of microfluidic digital PCR and conventional quantitative PCR for measuring copy number variation. Nucleic Acids Res. (2012) 40:e82. doi: 10.1093/nar/gks203
58. Bustin SA, Benes V, Garson JA, Hellemans J, Huggett J, Kubista M, et al. The MIQE guidelines: minimum information for publication of quantitative real-time PCR experiments. Clin Chem. (2009) 55:611–622. doi: 10.1373/clinchem.2008.112797
59. Cavalli M, De Novi LA, Della Starza I, Cappelli LV, Nunes V, Pulsoni A, et al. Comparative analysis between RQ-PCR and digital droplet PCR of BCL2/IGH gene rearrangement in the peripheral blood and bone marrow of early stage follicular lymphoma. Br J Haematol. (2017) 177:588–596. doi: 10.1111/bjh.14616
60. Nunes V, Cazzaniga G, Biondi A. An update on PCR use for minimal residual disease monitoring in acute lymphoblastic leukemia. Expert Rev Mol Diagn. (2017) 11:953–63. doi: 10.1080/14737159.2017.1377073
61. Alikian M, Whale AS, Akiki S, Piechocki K, Torrado C, Myint T, et al. RT-qPCR and RT-Digital PCR: a comparison of different platforms for the evaluation of residual disease in chronic myeloid leukemia. Clin Chem. (2017) 63:525–531. doi: 10.1373/clinchem.2016.262824
62. Franke G, Maier J, Wildenberger K, Cross M, Frank O, Giles F, et al. Quantification of BCR-ABL with digital PCR results in a significantly lower rate of deep molecular responses when compared to RT-qPCR in CML patients treated in the ENEST 1st trial. Blood. (2015) 126:135. doi: 10.1200/JCO.2016.34.15_suppl.7056
63. Goh HG, Kim D, Choi SY, Kim SH, Lee J, Lee YS, et al. Monitoring of dynamics of BCR-ABL transcripts over time using digital PCR assay in CP CML patients after achieving complete molecular remission. Blood. (2010) 116:1726
64. Andersen RF, Pallisgaard N. Exceeding MR5.0 sensitivity in routine BCR-ABL1 analysis using multiplex digital PCR. Blood. (2014) 124:4540.
65. Machova Polakova K, Zizkova H, Motlova E, Pecherkova P, Zuna J, Hovorkova L, et al. Patient-specific BCR-ABL1 genomic fusion analysis of minimal residual disease of CML patients eligible for TKI stopping significantly outperformed mRNA detection either by QPCR or digital PCR. EHA Library. (2018) 215432:PS1118.
66. Nicolini FE, Dulucq S, Guilhot J, Etienne G, Mahon FX. The evaluation of residual disease by digital PCR, and TKI duration are critical predictive factors for molecular recurrence after for stopping imatinib first-line in chronic phase CML Patients: results of the STIM2 study. Blood. (2018) 132:462. doi: 10.1182/blood-2018-99-113029
67. Bernardi S, Malagola M, Zanaglio C, Iurlo A, Bucelli C, Polverelli, et al. Comparative monitoring of minimal residual disease (MRD) By RT-quantitative (RT-qPCR) and digital PCR (dPCR) in Ph+ chronic myeloid leukemia (CML) patients treated with TKIs for recognition of stable deep molecular response (DMR) and identification of best candidates to TKIs treatment discontinuation. Blood. (2018) 132:3012. doi: 10.1182/blood-2018-99-112391
68. Mori S, Vagge E, le Coutre P, Abruzzese E, Martino B, Pungolino E, et al. Age and dPCR can predict relapse in CML patients who discontinued imatinib: the ISAV study. Am J Hematol. (2015) 90:910–4. doi: 10.1002/ajh.24120
69. Baccarani M, Soverini S, De Benedittis C. Molecular monitoring and mutations in chronic myeloid leukemia: how to get the most out of your tyrosine kinase inhibitor. Am Soc Clin Oncol Educ Book. (2014) 167–75. doi: 10.14694/EdBook_AM.2014.34.167
70. Gorre M, Mohammed M, Ellwood K, Hsu N, Paquette R, Rao P, et al. Clinical resistance to STI-571 cancer therapy caused by BCR-ABL gene mutation or amplification. Science. (2001) 293:876–80. doi: 10.1126/science.1062538
71. Von Bubnoff N, Schneller F, Peschel C, Duyster J. BCR-ABL gene mutations in relation to clinical resistance of philadelphia-chromosome-positive leukaemia to STI571: a prospective study. Lancet. (2002) 359:487–91. doi: 10.1016/S0140-6736(02)07679-1
72. Branford S, Rudzki Z, Walsh S, Parkinson I, Grigg A, Szer J, et al. Detection of BCR-ABL mutations in patients with CML treated with imatinib is virtually always accompanied by clinical resistance, and mutations in the ATP phosphate binding loop (P-Loop) are associated with a poor prognosis. Blood. (2003) 102:276–83. doi: 10.1182/blood-2002-09-2896
73. Shah N, Skaggs B, Branford S, Hughes T, Nicoll J, Paquette R, et al. Sequential ABL kinase inhibitor therapy selects for compound drug resistant BCR-ABL mutations with altered oncogenic potency. J Clin Invest. (2007) 117:2562–69. doi: 10.1172/JCI30890
74. Muller M, Cortes J, Kim D, Druker B, Erben P, Pasquini R, et al. Dasatinib treatment of chronic-phase chronic myeloid leukemia: analysis of responses according to preexisting BCR-ABL mutations. Blood. (2009) 114:4944–4953. doi: 10.1182/blood-2009-04-214221
75. Jabbour E, Morris V, Kantarjian H, Yin C, Burton E. Cortes, J. Characteristics and outcomes of patients with V299l BCR-ABL kinase domain mutation after therapy with tyrosine kinase inhibitors. Blood. (2012) 120:3382–83. doi: 10.1182/blood-2012-04-424192
76. Khoury H, Corte J, Kantarjian H, Gambacorti-Passerini C, Baccarani M, Kim D, et al. Bosutinib is active in chronic phase chronic myeloid leukemia after imatinib and dasatinib and/or nilotinib therapy failure. Blood. (2012) 119:3403–12. doi: 10.1182/blood-2011-11-390120
77. Soverini S, De Benedittis C, Machova Polakova K, Brouckova A, Horner D, Iacono M, et al. Unraveling the complexity of tyrosine kinase inhibitor-resistant populations by ultra-deep sequencing of the BCR-ABL kinase domain. Blood. (2013) 122:1634–48. doi: 10.1182/blood-2013-03-487728
78. Soverini S, Martinelli G, Amabile M, Poerio A, Bianchini M, Rosti G, et al. Denaturing-HPLC-based assay for detection of ABL mutations in chronic myeloid leukemia patients resistant to Imatinib. Clin Chem. (2004) 50:1205–13. doi: 10.1373/clinchem.2004.031112
79. Placzek EA, Plebanek MP, Lipchik AM, Kidd SR, Parker LL. A peptide biosensor for detecting intracellular Abl kinase activity using matrix-assisted laser desorption/ionization time-of-flight mass spectrometry. Anal Biochem. (2010) 397:73–8. doi: 10.1016/j.ab.2009.09.048
80. Parker WT, Lawrence R, Ho M, Irwin D, Scott H, Hughes T, et al. Sensitive detection of BCR-ABL1 mutations in patients with chronic myeloid leukemia after imatinib resistance is predictive of outcome during subsequent therapy. J Clin Oncol. (2011) 29:4250–59. doi: 10.1200/JCO.2011.35.0934
81. Khorashad J, Kelley T, Szankasi P, Mason C, Soverini S, Adrian L, et al. BCR-ABL1 compound mutations in tyrosine kinase inhibitor resistant CML: frequency and clonal relationships. Blood. (2013) 121:489–98. doi: 10.1182/blood-2012-05-431379
82. Zabriskie M. BCR-ABL1 compound mutations combining key kinase domain positions confer clinical resistance to ponatinib in Ph chromosome-positive leukemia. Cancer Cell. (2014) 26:428–42. doi: 10.1016/j.ccr.2014.07.006
83. Deininger M, Hodgson J, Shah N, Cortes J, Kim D, Nicolini F, et al. Compound mutations in BCR-ABL1 are not major drivers of primary or secondary resistance to ponatinib in CP-CML patients. Blood. (2016) 127:703–12. doi: 10.1182/blood-2015-08-660977
84. Benichou J, Ben-Hamo R, Louzoun Y, Efroni S. Rep-Seq uncovering the immunological repertoire through next-generation sequencing. Immunology. (2012) 135:183–91. doi: 10.1111/j.1365-2567.2011.03527.x
85. Merker JD, Valouev A, Gotlib J. Next-generation sequencing in hematologic malignancies: what will be the dividends? Adv Hematol. (2012) 3:333–39. doi: 10.1177/2040620712458948
86. Cagnetta A, Garuti A, Marani C, Cea M, Miglino M, Rocco I, et al. Evaluating treatment response of chronic myeloid leukemia: emerging science and technology. Curr Cancer Drug Targets. (2013). 13:779–90doi: 10.2174/15680096113139990084
87. Theunissen PMJ, de Bie M, van Zessen D, de Haas V, Stubbs AP, van der Velden VHJ. Next-generation antigen receptor sequencing of paired diagnosis and relapse samples of B-cell acute lymphoblastic leukemia: clonal evolution and implications for minimal residual disease target selection. Leuk Res. (2019) 76:98–104. doi: 10.1016/j.leukres.2018.10.009
88. Montaño A, Forero-Castro M, Marchena-Mendoza D, Benito R, Hernández-Rivas JM. New challenges in targeting signaling pathways in acute lymphoblastic leukemia by NGSApproaches: an update. Cancers (Basel). 2018 10:E110. doi: 10.3390/cancers10040110
89. Niu X, Chuang JC, Berry GJ, Wakelee HA Anaplastic Lymphoma Kinase Testing: IHC vs. FISH vs. NGS. Curr Treat Options Oncol. (2017) 18:71. doi: 10.1007/s11864-017-0513-x
90. Rosenquist R, Rosenwald A, Du MQ, Gaidano G, Groenen P, Wotherspoon A, et al. Clinical impact of recurrently mutated genes on lymphoma diagnostics: state-of-the-art and beyond. Haematologica. (2016) 101:1002–9. doi: 10.3324/haematol.2015.134510
91. Dubois S, Jardin F. The role of next-generation sequencing in understanding the genomic basis of diffuse large B cell lymphoma and advancing targeted therapies. Expert Rev Hematol. (2016) 2016:255–69. doi: 10.1586/17474086.2016.1130616
92. Bacher U, Shumilov E, Flach J, Porret N, Joncourt R, Wiedemann G, et al. Challenges in the introduction of next-generation sequencing (NGS) for diagnostics of myeloid malignancies into clinical routine use. Blood Cancer J. (2018) 8:113. doi: 10.1038/s41408-018-0148-6
93. Villamor N, López-Guillermo A, López-Otín C, Campo E. Next-generation sequencing in chronic lymphocytic leukemia. Semin Hematol. (2013) 50:286–95. doi: 10.1053/j.seminhematol.2013.09.005
94. Parker W, Phillis S, Yeung D, Hughes T, Scott H, Branford S. Many BCR-ABL1 compound mutations reported in chronic myeloid leukemia patients may actually be artifacts due to PCR-mediated recombination. Blood. (2014) 124:153–55. doi: 10.1182/blood-2014-05-573485
95. Müller MC, Cervantes F, Hjorth-Hansen H, Janssen JJWM, Milojkovic D, Rea D, et al. Ponatinib in chronic myeloid leukemia (CML): Consensus on patient treatment and management from a European expert panel. Crit Rev Oncol Hematol. (2017) 120:52–9. doi: 10.1016/j.critrevonc.2017.10.002
96. Kastner R, Zopf A, Preuner S, Pröll J, Niklas N, Foskett P, et al. Rapid identification of compound mutations in patients with Philadelphia-positive leukaemias by long-range next generation sequencing. Eur J Cancer. (2014) 50:793–800. doi: 10.1016/j.ejca.2013.11.030
97. Richters A, Ketzer J, Getlik M, Grütter C, Schneider R, Heuckmann JM, et al. Targeting gain of function and resistance mutations in Abl and KIT by hybrid compound design. J Med Chem. (2013). 56:5757–72. doi: 10.1021/jm4004076
98. Soverini S, Bavaro L, Martelli M, De Benedittis C, Papayannidis C, Percesepe A, et al. Next generation sequencing-based bcr-abl1 kinase domain mutation screening in de novo and tyrosine kinase inhibitor-resistant philadelphia chromosome-positive acute lymphoblastic leukemia: results of a prospective study. Blood. (2018) 132:4078. doi: 10.1182/blood-2018-99-117028
99. Parker WT, Yeung DT, Yeoman AL, Altamura HK, Jamison BA, Field CR, et al. The impact of multiple low-level BCR-ABL1 mutations on response to ponatinib. Blood. (2016) 127:1870–80. doi: 10.1182/blood-2015-09-666214
100. Soverini S, Ernst T, Kohlmann A, De Benedittis C, Alikian M, Foroni L, et al. Sensitivity, reproducibility and clinical utility of next-generation sequencing (NGS) for BCR-ABL1 Kinase domain mutation screening: results from the CML work package of the iron-II (Interlaboratory RObustness Of Next-Generation Sequencing) international study. Blood. (2013) 122:3824.
101. Soverini S, Bavaro L, De Benedittis C, Martelli M, Stella S, Vigneri P, et al. What's Next in CML - a prospective study evaluating sanger sequencing and next generation sequencing (NGS) for BCR-ABL1 kinase domain (KD) mutation screening. Blood. (2017) 130:248.
Keywords: CML, chronic myeloid leukemia, digital PCR, real-time PCR, NGS, mutations, ABL1, BCR-ABL1
Citation: Izzo B, Gottardi EM, Errichiello S, Daraio F, Baratè C and Galimberti S (2019) Monitoring Chronic Myeloid Leukemia: How Molecular Tools May Drive Therapeutic Approaches. Front. Oncol. 9:833. doi: 10.3389/fonc.2019.00833
Received: 05 March 2019; Accepted: 13 August 2019;
Published: 06 September 2019.
Edited by:
Pierluigi Porcu, Sidney Kimmel Cancer Center, United StatesReviewed by:
Christine Marie Stellrecht, University of Texas MD Anderson Cancer Center, United StatesLeland Metheny, University Hospitals of Cleveland, United States
Copyright © 2019 Izzo, Gottardi, Errichiello, Daraio, Baratè and Galimberti. This is an open-access article distributed under the terms of the Creative Commons Attribution License (CC BY). The use, distribution or reproduction in other forums is permitted, provided the original author(s) and the copyright owner(s) are credited and that the original publication in this journal is cited, in accordance with accepted academic practice. No use, distribution or reproduction is permitted which does not comply with these terms.
*Correspondence: Barbara Izzo, YmFyYmFyYS5penpvQHVuaW5hLml0
†These authors have contributed equally to this work