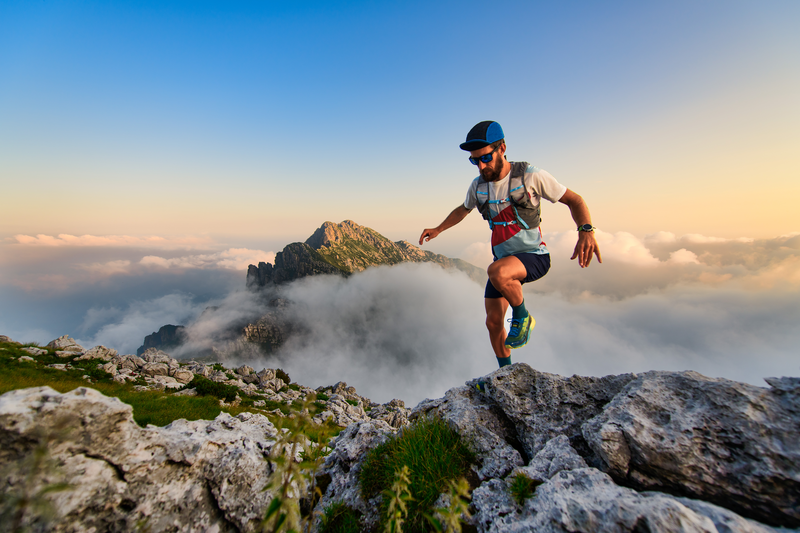
94% of researchers rate our articles as excellent or good
Learn more about the work of our research integrity team to safeguard the quality of each article we publish.
Find out more
ORIGINAL RESEARCH article
Front. Oncol. , 14 May 2019
Sec. Radiation Oncology
Volume 9 - 2019 | https://doi.org/10.3389/fonc.2019.00391
Due to the advantages of charged particles compared to conventional radiotherapy, a vast increase is noted in the use of particle therapy in the clinic. These advantages include an improved dose deposition and increased biological effectiveness. Metastasis is still an important cause of mortality in cancer patients and evidence has shown that conventional radiotherapy can increase the formation of metastasizing cells. An important pathway involved in the process of metastasis is the Hedgehog (Hh) signaling pathway. Recent studies have demonstrated that activation of the Hh pathway, in response to X-rays, can lead to radioresistance and increased migratory, and invasive capabilities of cancer cells. Here, we investigated the effect of X-rays, protons, and carbon ions on cell survival, migration, and Hh pathway gene expression in prostate cancer (PC3) and medulloblastoma (DAOY) cell lines. In addition, the potential modulation of cell survival and migration by the Hh pathway inhibitor GANT61 was investigated. We found that in both cell lines, carbon ions were more effective in decreasing cell survival and migration as well as inducing more significant alterations in the Hh pathway genes compared to X-rays or protons. In addition, we show here for the first time that the Hh inhibitor GANT61 is able to sensitize DAOY medulloblastoma cells to particle radiation (proton and carbon ion) but not to conventional X-rays. This important finding demonstrates that the results of combination treatment strategies with X-ray radiotherapy cannot be automatically extrapolated to particle therapy and should be investigated separately. In conclusion, combining GANT61 with particle radiation could offer a benefit for specific cancer types with regard to cancer cell survival.
Over the past decades an increased use of charged particles in radiotherapy has been observed. This is due to the advantages that charged particles, such as protons and carbon ions, offer compared to conventional X-rays. More specifically, due to the physical properties of these particles, the tumor can be more precisely targeted while the healthy tissues receive a lower dose (1, 2). In addition, carbon ions are biologically more effective compared to X-rays. This is reflected by the relative biological effectiveness or RBE value for carbon ions which, on average, is around 2–3 (1, 3). Although it is assumed that protons have an RBE of ~1.1, and thus the same or a similar effect as X-rays, recent evidence shows that protons, at given conditions, are able to induce a different biological response in cancer cells compared to X-rays (4–7).
Many advances have been made to enhance the therapeutic index in conventional radiotherapy. Nevertheless, radioresistant tumors require high radiation doses and the level of dose that can be delivered to obtain local tumor control is limited by the tolerance of the surrounding healthy tissues to these high doses. One option to overcome this problem is to sensitize cancer cells to radiation, by means of chemical or molecular agents. This strategy has already been exploited in the context of conventional radiotherapy (8, 9). However, limited data exists about the combination of particle therapy with such agents. Moreover, carbon ion therapy can also be useful to treat radioresistant tumors due to the higher RBE of carbon ions.
Cancer patients diagnosed with metastasis, either before, during or after treatment, often have a worse prognosis. Several studies, both in vitro and in vivo, have observed an increased migratory potential of cancer cells after X-ray irradiation (10–13). On the other hand, particle irradiation has been found to mostly decrease their migratory potential (6, 14–18). Previous research of our group showed that carbon ions had a greater effect on global gene expression than X-rays and that genes involved in motility were more suppressed after carbon ions than after X-rays (19, 20).
It is known that several molecular pathways are involved in the process of metastasis, including the Hedgehog (Hh) signaling pathway. This pathway is important in early development as well as tissue repair and regeneration in adults and becomes activated after binding of the Hh ligands to the membrane receptor patched 1 (PTCH1). As a consequence, the inhibition by PTCH1 on Smoothened (SMO) is released and this leads, in the end, to the translocation of the Glioma-associated oncogene homolog (GLI) proteins to the nucleus and the regulation of the Hh target genes (21). These target genes are involved in angiogenesis, migration, invasion, cell cycle progression, and apoptosis. Aberrant signaling of the Hh pathway has been implicated in the development and progression of several tumor types (21, 22), cancer cell metastasis and resistance to X-ray radiation (23–25). Chen et al. observed that the addition of soluble sonic hedgehog (SHH) to hepatocellular cancer cells resulted in increased resistance to X-ray irradiation. In addition, exposing these cells to X-ray radiation was found to activate the Hh pathway (26, 27). Other in vitro and in vivo studies have also reported a link between radioresistance and the Hh pathway (28–30). A clinical study by Sims-Mourtada et al. found that esophageal cancer patients with an active Hh pathway could sustain the repopulation of esophageal cancer cells after chemo-irradiation (31). Overall, these studies clearly demonstrate the association between X-ray radiation and Hh pathway activation. Moreover, an active Hh pathway can lead to resistance to X-rays. To the best of our knowledge no data are available on the effect of particle irradiation on Hh pathway activation and the corresponding role in radioresistance.
Several different inhibitors of the Hh pathway have been developed, with SMO-inhibitors vismodegib and sonidegib being the only ones approved by the Food and Drug Administration. Unfortunately, resistance to SMO-inhibitors is often observed (32). Therefore, inhibiting the Hh pathway downstream of SMO might be more successful. One such downstream inhibitor is GANT61 (Gli-ANTagonist) which is an inhibitor of GLI1/2 (33). Combining radiotherapy with Hh inhibitors as a possible way to sensitize cancer cells to radiation, has already been investigated in vitro and in vivo in combination with X-rays (29, 34–36). In addition, several clinical papers have also reported the combination of vismodegib with X-ray radiotherapy in patients with basal cell carcinomas (37–41). However, research on the specific combination of GANT61 with X-ray irradiation is still limited and not available in combination with particle irradiation (30, 34, 42).
The aim of this study was to investigate the effect of X-ray, proton and carbon ion irradiation on cell survival, migration and Hh pathway gene expression. In addition, we explored the potential of the Hh inhibitor GANT61 as an effective modulator of radiosensitivity and migration of cancer cells for the different radiation types. Both prostate cancer and medulloblastoma cells were used in this in vitro study, because both tumor types are good indications for particle therapy (43) and the Hh pathway plays an important role in either the initiation or progression of these tumor types (44).
Prostate cancer cells (PC3) and pediatric medulloblastoma cells (DAOY) were obtained from the American Type Culture Condition (ATCC, Molsheim Cedex, France). PC3 cells were cultured in minimal essential medium (Life technologies, Carlsbad, CA, USA) supplemented with 10% fetal bovine serum (FBS; Gibco, Life Technologies, Ghent, Belgium). DAOY cells were cultured in Eagle's Minimal Essential Medium (ATCC) supplemented with 10% FBS. All cell cultures were maintained in a humidified incubator (37°C and 5% CO2). Regular mycoplasma tests were performed on both cell lines. More information about the genetic background of both cell lines can be found on the website of the supplier (www.atcc.org).
For inhibition of the Hh pathway, the GLI1/2 inhibitor GANT61 was used at a concentration of 10 μM (Selleck Chemicals, Houston, TX, USA). Stock solutions were prepared by dissolving GANT61 in dimethyl sulfoxide (DMSO), then aliquoted and stored at −20°C. Control conditions were treated with the drug solvent. The drug was added 72 h before irradiation with either radiation type. During irradiation, the drug was still present in the medium until further processing of the cells was needed. After processing, GANT61 was removed.
Irradiation of the cells with X-rays was performed at the SCK•CEN facility (Mol, Belgium) using an Xstrahl 320 kV generator (250 kV, 12 mA, 3.8 mm Al, and 1.4 mm Cu). Cell culture flasks were horizontally irradiated with different X-rays doses [0, 0.25, 0.5, 2, 4, and 6 Gray (Gy)] at a calculated dose rate of 0.5 Gy/min.
Proton irradiation was performed at the iThemba LABS facility in South-Africa. Prior to the irradiation campaign, both cell lines were shipped from Belgium to South-Africa on dry-ice and cultured at iThemba LABS. Several days before the proton beam time was scheduled, cells were seeded in 12.5 cm2 flasks (Falcon, VWR; Leuven, Belgium). Just before irradiation, cell culture flasks were completely filled with medium and placed vertically in a Perspex phantom. The phantom consists of several Perspex plates with a cut-out for a culture flask to hold the cells at the specific depth, allowing a positioning accuracy of 0.1 mm. In order to irradiate the cells at a water equivalent depth (WED) of 85.0 mm that corresponds to a position near the middle of the SOBP, the required thickness of the total block of Perspex upstream of the measurement position was calculated by means of the numerical thick-target formalism proposed in Zhang and Newhauser (45) and the proton range and mass stopping power data given in Berger et al. (46). In addition, the wall of the cell culture flasks were accounted for in the WED calculations using a measured value for the density of the polystyrene material. Irradiations were performed with a 200 MeV proton beam, 10 cm field diameter, and a 50 mm SOBP with R50 range of 120 mm in water. The physical depth-dose profile of the proton beam was measured with a Markus™ ionization chamber (model 30-329) along the central axis of the Perspex phantom. These physical dose measurements were used to determine the output factor for the radiobiology experiments at the WED of 85.0 mm, which corresponds to an LET of 3.96 ± 0.20 keV/μm (47). The applied doses were 0.25, 0.5, 2, 4 and, 6 Gy. Due to the limited availability of the proton beam time, no samples for gene expression analysis, and migration assays could be obtained for the combination of proton irradiation with GANT61, for both cell lines.
Carbon ion beam time was granted by the iPAC committee of the Grand Accélérateur National d'Ions Lourds (GANIL; Caen, France). The cells were transported by car in a transportable incubator in completely filled flasks from Belgium to France. Afterwards the culture medium was replaced and the cells were placed overnight in a humidified incubator. Several days before irradiation the cells were seeded in 12.5 cm2 flasks (Falcon, VWR; Leuven, Belgium). Since irradiation was performed in a vertical position (perpendicular to the horizontal carbon ion beam), the culture flasks were completely filled with medium just before the start of the irradiation. Irradiation of the cells was performed with an initial carbon ion beam energy of 95 MeV/u (LET = 73 keV/μm) near the middle of the SOBP. The doses applied were 0, 0.25, 0.5, 1, 2, 3, and 4 Gy. Carbon ion dosimetry was performed as previously described (48).
After irradiation of the cells in culture flasks, cells were trypsinized and seeded in triplicate in six-well plates at low densities. Fourteen days after seeding, cells were fixed with 6% glutaraldehyde and 0.5% crystal violet (Sigma). Colonies of more than 50 cells were counted with a ColCount colony counter (Oxford Optronix, Oxford, UK). To calculate the surviving fraction (SF), the following formula was used:
The survival curve was fitted to the LQ model using the following formula:
in this formula α and β are radiation sensitivity parameters, D is the irradiation dose. At least three independent experiments were performed with three replicates per experiment. Due to experimental limitations, we were not able to obtain a larger number of control samples (compared to irradiated samples). Therefore, it will not be possible to detect slight changes in the survival of cells at lower doses such as 0.5 Gy. The RBE was calculated at a 10% survival by dividing the dose of X-rays at SF10 by the corresponding dose of proton or carbon ions at SF10. In order to determine the effect of the drug the sensitizer enhancingratio (SER10) was calculated by taking the ratio of the doses to reach 10% survival for control cells over the treated cells.
RNA was isolated at 8 h and 24 h after irradiation using the Allprep DNA/RNA kit (Qiagen, Venlo, The Netherlands) according to the manufacturer's protocol. After measuring the RNA concentration with the DropSense 16 (Trinean, Ghent, Belgium), RNA was reverse transcribed to cDNA using the GoScript™ Reverse Transcription System (Promega, Leiden, The Netherlands). Finally, qPCR reactions were performed by the 7,500 Fast Real Time PCR system (Applied Biosystems, Foster City, CA, USA). Primers for the Hh pathway genes (SHH, PTCH1, SMO, GLI1, GLI2, GLI3, and SUFU) and target genes of the Hh pathway (CYCLIND1, VEGFA, BCL-2, SNAIL, and MMP9) can be found in Table 1. Specific target genes of the Hh pathway were selected based on their involvement in cell cycle regulation (CYCLIND1), apoptosis (BCL-2), or migration/invasion (VEGFA, SNAIL, and MMP-9).
To investigate the migratory potential of cancer cells after irradiation, the Boyden chamber assay (Neuroprobe, Gaithersburg, MD, USA) was used. Briefly, cells were seeded in serum-free medium in the upper wells of the Boyden chamber 24 h after irradiation. The lower wells were filled with medium containing 0.1% FBS. The upper wells were separated from the lower wells by means of a polycarbonate membrane with 8 μm pores (Neuroprobe). For PC3 cells the membrane was precoated with collagen I (Life Technologies, Carlsbad, CA, USA). After allowing the cells to migrate for 16 h, the cells were fixed with methanol and stained with 0.5% crystal violet. Images were captured with a Leica MZ12 microscope and the migrated cells were counted in five separate fields with Image J.
Statistical analysis was performed using GraphPad Prism 7 (GraphPad Software, Inc., La Jolla, USA). For the in vitro experiments, a one-way ANOVA with Tukey's multiple comparison test or a two-tailed student's t-test was performed. P-values ≤ 0.05 were considered statistically significant.
All radiation types (X-rays, protons, and carbon ions) were able to induce a dose-dependent decrease in cancer cell survival (Figure 1). Proton irradiation induced a similar survival profile as X-rays, both in PC3 and DAOY cells. This was also reflected in the calculated RBE of protons at 10% survival (RBE10) which was 0.94 for PC3 cells and 1.06 for DAOY cells. In contrast, carbon ions were more effective in decreasing the survival of PC3 and DAOY cells compared to both X-rays and protons. The calculated RBE10 for PC3 cells was 1.93 and 2.57 for DAOY cells. More information about the radiation sensitivity parameters α and β of both cell lines can be found in Table 2.
Figure 1. Colony survival assay of PC3 and DAOY cells irradiated with X-rays, protons or carbon ions. Surviving fraction of PC3 (A) and DAOY (B) cells after irradiation with different doses, calculated 14 days after start of colony survival. Means ± SEM of three independent experiments performed in triplicate.
The investigated genes had a varied expression in response to the different radiation types (Table 3). In PC3 cells it is clear that carbon ions were able to induce more significant changes in the Hh pathway genes compared to protons and X-rays. Moreover, 8 h after carbon ion irradiation, all significant changes that are observed in the Hh pathway genes show an increased expression whereas at 24 h the majority of Hh pathway genes are significantly decreased. Significant decreases were observed at all doses of carbon ions for SHH, PTCH1, SMO, and SUFU. In contrast, very few significant changes in gene expression were observed after irradiation with X-rays and protons. Furthermore, 8 h after X-ray irradiation, most of the Hh pathway genes show a decreased expression whereas at 24 h this has shifted to an overall increased expression. At both time points after proton irradiation, the only significant change observed was at 24 h where GLI3 showed a significant decreased expression for all the radiation doses. In contrast, for X-rays a significant increase in GLI3 expression is observed at 24 h. The target genes of the Hh pathway also showed most significant changes after carbon ion irradiation. Specifically, at 24 h after carbon ion a persistent decrease in VEGFA could be observed. For X-rays and protons, an overall downregulation was observed at 8 h whereas an overall upregulation was observed at 24 h.
In DAOY cells, carbon ions induced much more significant changes in the expression of the investigated genes compared to X-rays and protons, for both 8 h and 24 h time points (Table 4). For the Hh pathway genes an overall decreased expression (non-significant) was observed both at 8 h and 24 h after X-rays. Moreover, a persistent decreasing trend could be observed for SHH, PTCH1, SMO, GLI1, GLI2. At 8 h after proton irradiation a decreased expression of the Hh pathway genes was observed. However, at 24 h most of these genes showed an increased expression. For carbon ions, only SHH demonstrated a persistent significant increased expression. The other genes of the Hh pathway showed a variable expression at 8 h after carbon ions whereas at 24 h most genes showed downregulation. All target genes of the Hh pathway, except for CCND1, showed a decreased expression 8 h after X-ray radiation. Only SNAIL and MMP9 showed a persistent decreased expression after X-rays. CCND1 showed a persistent increased expression after carbon ion radiation. Target genes of the Hh pathway mostly showed opposite responses at 8 h and 24 h after proton irradiation. For example, CCND1 had an increased expression at 8 h and a decreased expression at 24 h after proton irradiation. In contrast, BCL-2 and VEGFA showed an overall decreased expression at 8 h and an overall increased expression at 24 h after exposure to protons.
For both PC3 and DAOY cells, a general dose-dependent decrease in migration was observed for all types of radiation (Figure 2). In PC3 cells, protons were able to decrease the migration (except at 0.25 Gy), however the observed decrease was less pronounced than that of X-rays (Figure 2A). Carbon ions were significantly more effective in decreasing the migration of PC3 cells at low doses (0.25 and 0.5 Gy) compared to X-rays. In comparison to protons, carbon ions were overall more significantly effective in decreasing the migration of PC3 cells. In DAOY cells, protons were able to significantly decrease migration at a dose of 0.5 and 2 Gy in respect to similar X-ray doses (Figure 2B). In addition, carbon ions significantly decreased migration of DAOY cells at all doses compared to X-rays.
Figure 2. Migration of PC3 and DAOY cells after X-ray, proton, or carbon ion irradiation. The Boyden chamber assay was performed in order to assess the migratory potential of PC3 (A) and DAOY (B) cells. The migration assays was started 24 h after different doses of X-rays, protons, or carbon ions. Means ± SEM of three independent experiments performed in triplicate. *p ≤ 0.05 vs. control cells; **p ≤ 0.01; ***p ≤ 0.001; ****p ≤ 0.0001.
Survival of PC3 cells was not affected by the addition of GANT61 in combination with any of the radiation types (Figure 3). This was also reflected by a negligible sensitizer enhancing ratio at 10% survival (SER10) of 1.09, 0.98, and 1.07 for X-rays, protons, and carbon ions, respectively.
Figure 3. Effect of GANT61 in combination with radiation on the survival of PC3 cells. Colony survival curves of PC3 cells pre-treated for 72 h with GANT61 (10μM) and irradiated with different doses of X-rays (A), protons (B), or carbon ions (C). Means ± SEM of three independent experiments performed in triplicate.
In DAOY cells, GANT61 was able to sensitize the cells to proton and carbon ion radiation. Significant sensitization was observed at 4 Gy for protons and at 2 and 3 Gy for carbon ions. However, GANT61 was not able to sensitize DAOY cells to X-rays (Figure 4). The SER10 for X-rays, protons, and carbon ions was 1.07, 1.40, and 1.38, respectively.
Figure 4. Effect of GANT61 in combination with radiation on the survival of DAOY cells. Colony survival curves of DAOY cells pre-treated for 72 h with GANT61 (10μM) and irradiated with different doses of X-rays (A), protons (B), or carbon ions (C). Means ± SEM of three independent experiments performed in triplicate. *p ≤ 0.05 vs. control cells; **p ≤ 0.01.
The majority of Hh pathway genes showed a decreased expression after the combination of GANT61 with X-rays at 8 h post-irradiation (Table 5). The combination of GANT61 with carbon ions induced much more significant changes in the expression of the Hh pathway genes compared to the combination of GANT61 with X-rays. Moreover, 8 h after carbon ion irradiation, GANT61 was able to induce a significant decreased expression in the majority of the Hh pathway genes compared to DMSO treated cells. However, this was only persistent at 24 h after 0.5 Gy of carbon ions. For 2 Gy of carbon ions the opposite effect was observed after 24 h, with an increased expression in the majority of the Hh pathway genes. A significant decrease in SUFU gene expression was observed at 8 h after both X-rays and GANT61 and carbon ion and GANT61. The target genes of the Hh pathway showed an overall persistent decreased expression post-irradiation. In PC3 cells, the combination of GANT61 with either X-rays or carbon ions (Table 5) induced much more significant changes in gene expression as compared to irradiation alone (Table 3). In addition, opposite effects are observed after carbon ions compared to the combination of carbon ions with GANT61. Specifically for SUFU, which shows an increased expression at 8 h after carbon ions and a decreased expression at 24 h. In contrast, the combination of GANT61 and carbon ions significantly decreased the expression of SUFU at 8 h whereas an increase was observed at 24 h.
Table 5. Gene expression (qPCR) in PC3 cells after GANT61 treatment (10μm) of GANT61 in combination with radiation compared to DMSO treated cells.
In DAOY cells, the combination of GANT61 with X-rays had an overall increasing effect on the expression of the Hh pathway genes at 8 h and 24 h after treatment (Table 6). Furthermore, 24 h after treatment a significant decreased expression in SMO and GLI1 was observed. For carbon ions, GANT61 was able to significantly increase the expression of most of the Hh pathway genes at 8 h post-irradiation, whereas at 24 h after carbon ion irradiation (2 and 4 Gy) all Hh pathway genes were significantly downregulated. The target genes of the Hh pathway showed an increased expression at 8 h post-irradiation (X-rays and carbon ions) whereas at 24 h post-irradiation the majority of genes were significantly downregulated. BCL-2 showed a significant and persistent decreased expression at 8 h and 24 h after X-ray and GANT61 treatment. Comparing the effect of irradiation alone (Table 4) with the effect of GANT61 and irradiation demonstrated that the combination treatment induced much more significant changes compared to radiation alone. Moreover, X-rays alone downregulated the expression of SNAIL and VEGFA at 8 h post-irradiation whereas a significant increased expression was observed at 8 h after GANT61 and X-rays. For carbon ions we observed significant increases in SHH, PTCH1, CCND1, BCL-2, and VEGFA at 24 h post-irradiation. In contrast, the addition of GANT61 to carbon ions resulted in a significant downregulation of the aforementioned genes.
Table 6. Gene expression (qPCR) in DAOY cells after GANT61 treatment (10μM) in combination with radiation compared to DMSO treated cells.
Carbon ions were able to significantly decrease migration compared to X-rays in DMSO-treated PC3 cells at all doses except 0 Gy (Figure 5). The combination of X-rays and GANT61 was able to significantly decrease the migration of PC3 cells in comparison to DMSO treated controls at 2 and 4 Gy. In contrast, an increase in migration was observed after the combination of GANT61 with carbon ions compared to DSMO treated controls (Figure 5). However, at 0 and 0.25 Gy the combination of GANT61 with carbon ions was significantly superior at decreasing PC3 migration in comparison to GANT61 with X-rays.
Figure 5. Migration of PC3 cells after the combination of GANT61 with irradiation. The Boyden chamber assay was performed in order to assess the migratory potential of PC3 cells after the combination of GANT61 (72 h pre-incubation; 10μM) with X-rays or with carbon ions. Means ± SEM of 2–3 independent experiments performed in triplicate. *p ≤ 0.05 vs. control cells; **p ≤ 0.01; ***p ≤ 0.001; ****p ≤ 0.0001.
Migration of DAOY cells was significantly more suppressed after carbon ion radiation compared to X-ray radiation at 2 and 4 Gy (Figure 6). The combination of X-rays with GANT61 was able to significantly decrease the migration in comparison to X-rays alone at all doses except at 0.25 Gy. The combination of GANT61 with carbon ions was only able to significantly decrease the migration of DAOY cells at lower doses (0.25, 0.5 Gy) in comparison to carbon ions alone.
Figure 6. Migration of DAOY cells after the combination of GANT61 with irradiation. The Boyden chamber assay was performed in order to assess the migratory potential of DAOY cells after the combination of GANT61 (72 h pre-incubation; 10μM) with X-rays or with carbon ions. Means ± SEM of 2–3 independent experiments performed in triplicate. *p ≤ 0.05 vs. control cells; **p ≤ 0.01; ***p ≤ 0.001; ****p ≤ 0.0001.
With the increasing use of particle therapy in the clinic it is important to enhance our knowledge on the molecular mechanisms occurring in response to particle radiation as this might be different as compared to conventional X-ray therapy. Furthermore, combining radiotherapy with targeted therapies can sensitize cancer cells to radiation, thereby decreasing the radiation dose needed to obtain tumor control. Our previous studies showed that carbon ions are able to induce much more changes in global gene expression compared to X-rays in prostate cancer cells (20). In addition, carbon ions were found to be more effective than X-rays in decreasing the expression of genes involved in motility (19).
Previous studies showed that active Hh signaling can lead to resistance of cancer cells to X-ray radiation (28–30). Moreover, X-ray radiation can also activate the Hh pathway (26, 27). Finally, this pathway has also been linked to the migration and invasion of cancer cells. However, to the best of our knowledge, no investigations have been performed on the effect of particle radiation on the Hh pathway. Therefore, we investigated the effect of X-rays, proton, and carbon ion radiation on cell survival, migration, and Hh pathway gene expression. In addition, the potential modulating effect of the Hh inhibitor GANT61 on these endpoints was analyzed.
The effect of particle radiation on the survival of several cancer cells has already been investigated (49). However, there are no studies available that compare the effect of X-rays, protons, and carbon ions on cell survival in DAOY or PC3 cells in one single study. We observed that carbon ions were more effective in decreasing cell survival of both cell lines compared to either X-rays or protons. This is in line with other studies that observed similar findings in chordoma, colon carcinoma, and prostate cancer cells (19, 50). In addition, we observed that protons induced a similar survival curve as 250 kV X-rays. This was also reflected in the RBE10 of protons of 0.94 for PC3 cells and 1.06 for DAOY cells. The clinical RBE of protons is currently set to be ~1.1, however until today there is still much debate on whether this is correct or should be adjusted (51, 52). One of the arguments is that the RBE depends on several factors such as biological endpoint, linear energy transfer (LET), radiation dose, and tissue type. This is also clear from our RBE results, more specifically the difference in RBE between PC3 and DAOY cells, which reflects the variation between different cancer types.
We have investigated here for the first time the potential of the Hh inhibitor GANT61 to modulate the radiosensitivity of PC3 and DAOY cells in combination with proton and carbon ion irradiation. Our results indicate a radiosensitizing effect of GANT61 in combination with protons and carbon ions in DAOY cells, but not in combination with X-rays. In contrast, we could not observe a radiosensitizing effect of GANT61 in PC3 cells for any of the radiation types used in this study. So far there have only been a few studies investigating the combination of GANT61 with radiation, all of which have used X-ray radiation. Zhou et al. showed that GANT61 was able to sensitize renal cancer cells to X-rays (42). Another study by Gonnissen et al. investigated the effect of GANT61 in combination with X-rays in different prostate cancer cell lines. They observed a radiosensitizing effect in 22RV1 cells, but not in the DUI145 or PC3 cells (30, 34). This is in accordance with our results for PC3 cells where we also did not observe a radiosensitizing effect of GANT61 in combination with X-rays. GANT61 has been shown to induce sensitivity to X-ray radiation in a p53 dependent manner. Therefore, wild-type (WT) p53 cell lines will be sensitized to X-ray irradiation by GANT61, whereas cell lines with mutated p53 will not show any sensitization to X-ray irradiation (34). Both PC3 and DAOY cells have mutated p53 and this could explain why both cell lines do not show sensitization to X-ray radiation (53). On the other hand, it has been shown that particle radiation can induce radiosensitization in a p53 independent manner (54). This could explain the GANT61-induced sensitivity to proton and carbon ion radiation in DAOY cells. However, this could not be observed in the PC3 cells. One potential explanation might be that the basal Hh pathway activity is relatively higher in DAOY then in PC3 cells (data not shown). Since in PC3 cells only very low levels GLI1, a marker for Hh pathway activity, were observed, we could expect that Hh inhibition would not affect the cells much. In addition, gene expression at 24 h after the combination of carbon ion radiation + GANT61 showed significant decreases for the Hh pathway genes and its target genes in DAOY cells (2 and 4 Gy), whereas such a strong significant decrease was not observed in PC3 cells after the combination of carbon ion + GANT61 (except for at a 0.5 Gy dose). Another possible explanation could be that other mechanisms play a role in the response to the combination treatment. Moreover, GANT61 influences other target genes or processes, which we did not investigate here but that could have an effect and might explain the differences observed between both cell lines. Specifically, GANT61 targets not only cell cycle progression, migration, or apoptosis (as we have investigated the expression of some of the genes involved in these processes), but also DNA damage repair, autophagy, inflammatory response, limitless replicative potential, and CSC's are targeted by GANT61 (33).
In this study we showed that X-rays, protons, and carbon ions each induce their own expression profile of the Hh pathway and some selected Hh target genes. Moreover, we observed that, both in PC3 and DAOY cells, carbon ions were more effective in significantly altering the expression of the Hh pathway genes and target genes in comparison to X-rays or protons. This is in line with several microarray studies that investigated gene expression after X-ray and carbon ion irradiation. In these studies carbon ions were able to induce much more significant alterations in global gene expression compared to X-rays (20, 55, 56). Microarray studies comparing protons with X-rays are very scarce. One study by Girdhani et al. demonstrated that protons induced a dose-dependent downregulation in VEGF, IL-6, IL-8, and HIF1A expression in a panel of different cell types whereas X-rays showed a dose-dependent upregulation of the expression of these genes (4, 57). Next to the radiation-type dependent response of the Hh pathway gene expression, we also observed a cell-type dependent expression of the investigated genes. For example, at 24 h after X-ray irradiation an overall increasing trend is observed in the Hh pathway gene expression in PC3 cells whereas in DAOY cells the majority of Hh pathway genes show a downregulated expression. The opposite is observed 24 h after carbon ion radiation, which is most prominent for SHH and PTCH1 which both are significantly downregulated in PC3 cells, whereas they are significantly upregulated in DAOY cells. For PC3, VEGFA was downregulated at 8 h after proton radiation whereas an upregulation was observed 24 h after protons and 8 h and 24 h after X-rays. These results are in line with a study by Girdhani et al. where X-rays induced an increased expression of VEGFA, whereas protons decreased the expression of VEGFA (4). This is in contrast to what we observed in DAOY cells, where VEGFA showed an overall decreased expression at 8 h after X-rays and protons whereas an overall increased expression was observed at 24 h. However, it should be noted that in the study by Girdhani et al. only the time point of 6 h post-irradiation was investigated. The increased expression of CCND1 observed in PC3 and DAOY cells at 8 h after X-ray or carbon ion exposure is similar to what Fushimi and colleagues observed (58). In this study, an oral squamous cell carcinoma cell line was irradiated with either X-rays, carbon ions, or neon ions. They reported that both X-rays and carbon ions upregulated the expression of CCND1 with carbon ions inducing a higher upregulation than X-rays. A study by Chun et al. observed a downregulation of MMP9, SNAIL1, and VEGFA after proton irradiation in liver cancer cells (59). This is in line with what we observed 8 h after proton radiation in PC3 cells (except for SNAIL) and in DAOY cells (except for MMP9). Again it should be noted that this study investigated gene expression at 6 h whereas we investigated at 8 h post-irradiation.
The combination of GANT61 with carbon ions was able to suppress even more the downregulation in the Hh pathway genes in PC3 and DAOY cells compared to DMSO treated controls. This decreased expression was more pronounced after carbon ions than after X-rays. At 24 h after carbon ion radiation a significant downregulation in Hh pathway gene expression was observed in DAOY cells. This could explain the radiosensitizing effect of GANT61 that we observed in response to carbon ions. In contrast to the PC3 cells, where the Hh pathway genes shows less repression at 24 h after carbon ions and no radiosensitizing effect was observed. We also observed cell-type dependent changes in gene expression after the combination treatment. Moreover, PC3 cells showed a decreased expression at 8 h after GANT61 and X-ray exposure, whereas DAOY cells showed an increased expression at 8 h and 24 h after X-ray and GANT61 exposure. The addition of GANT61 to either X-rays or carbon ions results in a significant downregulation of BCL-2 in almost all investigated doses in both PC3 and DAOY cells. Since BCL-2 is known to promote cell survival, this could indicate that the addition of GANT61 to radiation affects cell survival even more than radiation alone. As previously mentioned, we were not able to obtain samples for gene expression after proton radiation and GANT61 treatment. It would therefore be interesting to gather this data in the future to see whether a similar effect is seen as with carbon ions and if this could be linked to the radiosensitization observed for protons.
Contradictory results exist concerning the effect of X-ray radiation on the migration of cancer cells, with some studies showing a decrease in migration while others show an increase in migration (10). In contrast, it is believed that carbon ions are more effective in decreasing the migration and invasion of cancer cells (14, 60). Our results show a dose-dependent decrease in migration after X-rays, protons as well as carbon ions, with carbon ions inducing a more pronounced decrease compared to X-rays and protons in both cell lines. This is in line with previous studies showing a decrease in migratory potential of DAOY cells after X-ray and carbon ion radiation (61). However, there are also some studies demonstrating an increased migration of DAOY cells in response to X-rays (62, 63). Moreover, we observed an increased migration of DAOY cells after a 0.5 Gy dose of protons. There have been some papers that observed an increased migration in response to proton irradiation, however this was at much higher doses (3, 4, and 8 Gy) (6, 64). For X-ray irradiation it is known that sublethal doses can increase the migration of cells, due to increased MMP activity and changes in BCL-2 family protein expression toward an apoptosis-resistant phenotype (11, 65). In line with these findings, it could also be that sublethal doses of proton irradiation might enhance the migration of cells. It would therefore be interesting to also investigate the expression of the migration related genes or MMP activity at lower proton doses (0.1–0.25 Gy).
No studies have been performed to investigate the effect of protons on DAOY cells or the effect of X-rays, protons, or carbon ions on PC3 cells. Based on our results, the migratory potential of DAOY cells appeared to be more affected than PC3 cells by irradiation.
The combination of GANT61 and radiation exposure on the migration of cancer cells has not been investigated so far. We show here for the first time that the combination of GANT61 with X-rays in PC3 and DAOY cells, is able to decrease migration more effectively than X-rays alone. We also investigated Hh target genes involved in cell migration (SNAIL, VEGFA, and MMP9) and found that both SNAIL and VEGF expression was decreased 24 h after the combination of X-ray and GANT61 exposure. This downregulation could possibly explain the significant decrease in migration of PC3 cells treated with X-rays and GANT61 compared to DMSO treated cells. In contrast, the combination of carbon ions and GANT61 increases the migration of PC3 cells at 2 and 4 Gy, which could be linked to the increased expression of VEGFA (2 Gy) and MMP9 (2 and 4 Gy) at 24 h after GANT61 and carbon ion irradiation. In contrast, SNAIL shows a decreased expression at 2 and 4 Gy 24 h after the combination treatment. The combination of GANT61 with carbon ions was only able to significantly decrease the migration of DAOY cells for carbon ion doses up to 0.5 Gy compared to DMSO treated controls. For DAOY cells, SNAIL, VEGF, and MMP9 expression was decreased 24 h after the combination of X-rays and GANT61 as well as the combination of carbon ions and GANT61 (except SNAIL at 2 Gy after X-ray and GANT61 exposure and MMP9 at 0.5 Gy after carbon ion and GANT61 exposure). This decrease could explain the decreased migration we observed for DAOY cells after the addition of GANT61 when compared to the DMSO treated controls for both X-rays and carbon ions. Unfortunately, we were not able to obtain the migration samples for the proton and GANT61 combination in this study. It would however be interesting to gather this data since medulloblastoma is a good candidate for proton therapy and, metastasis is often observed in these patients.
In conclusion, we show that carbon ions are more effective in decreasing cell survival, decreasing migration, and inducing more significant alterations in the Hh pathway genes in PC3 and DAOY cells as compared to X-rays and protons. In addition, we show here for the first time that the Hh inhibitor GANT61 is able to sensitize DAOY medulloblastoma cells to particle radiation (proton and carbon ion) but not to conventional X-rays. This is an important finding since it suggests that the results that were previously obtained from combination treatment strategies with X-ray therapy cannot be automatically extrapolated to particle therapy and should be investigated separately. However, our experiments have to be expanded to other cell lines of different tumor types to understand whether our findings are also relevant for other tumor types or not.
KK performed experiments at SCK•CEN, GANIL, and iThemba LABS. MM and SI designed the experimental set-up. NB, BB, and MV helped with experiments performed at GANIL. MM and RV helped with experiments performed at GANIL and iThemba LABS. CV helped with experiments performed at iThemba LABS. AJ helped with qPCR experiments. SB and KH contributed to the design of the work, as well as with interpretation of obtained data. All co-authors critically reviewed and approved the final version to be submitted to this Journal.
KK is a recipient of a SCK•CEN-KUL PhD grant. KK, NB, MV, and BB received a Horizon2020 travel grant (No. 654002) for experiments at GANIL. KK received an EU CONCERT travel grant and Belgian Hadron therapy Consortium (BHTC) travel grant, whereas MM received an FWO travel grant to perform experiments at iThemba LABS in South-Africa. KH is a clinical research fellow of the Research Foundation Flanders, Belgium.
The authors declare that the research was conducted in the absence of any commercial or financial relationships that could be construed as a potential conflict of interest.
We would like to thank the iPAC committee of the Grand Accélérateur National d'Ions Lourds (GANIL, Caen, France) for the carbon ion beam time granted (P1006-H) and the staff of the LARIA, CIRIL (GANIL) for allowing us access to and use of their facility. We would also like to thank the PAC committee of iThemba LABS (Cape Town, South-Africa) for the proton beam time granted and the staff of the Radiation Biophysics Division. We also thank Bart Marlein, Michel Doms, and Raf Aerts for their continued assistance during the X-irradiations at SCK•CEN.
1. Durante M, Loeffler JS. Charged particles in radiation oncology. Nat Rev Clin Oncol. (2010) 7:37–43. doi: 10.1038/nrclinonc.2009.183
2. Suit H, DeLaney T, Goldberg S, Paganetti H, Clasie B, Gerweck L, et al. Proton vs. carbon ion beams in the definitive radiation treatment of cancer patients. Radiother Oncol. (2010) 95:3–22. doi: 10.1016/j.radonc.2010.01.015
3. Weyrather WK, Debus J. Particle beams for cancer therapy. Clin Oncol (R Coll Radiol). (2003) 15, S23–8.
4. Girdhani S, Lamont C, Hahnfeldt P, Abdollahi A, Hlatky L. Proton irradiation suppresses angiogenic genes and impairs cell invasion and tumor growth. Radiat Res. (2012) 178:33–45. doi: 10.1667/RR2724.1
5. Girdhani S, Sachs R, Hlatky L. Biological effects of proton radiation: an update. Radiat Prot Dosimetry. (2015) 166:334–8. doi: 10.1093/rpd/ncv178
6. Jasinska-Konior K, Pochylczuk K, Czajka E, Michalik M, Romanowska-Dixon B, Swakon J, et al. Proton beam irradiation inhibits the migration of melanoma cells. PLoS ONE. (2017) 12:e0186002. doi: 10.1371/journal.pone.0186002
7. Lupu-Plesu M, Claren A, Martial S, N'Diaye PD, Lebrigand K, Pons N, et al. Effects of proton versus photon irradiation on (lymph)angiogenic, inflammatory, proliferative and anti-tumor immune responses in head and neck squamous cell carcinoma. Oncogenesis. (2017) 6:e354. doi: 10.1038/oncsis.2017.56
8. Kim BM, Hong Y, Lee S, Liu P, Lim JH, Lee YH, et al. Therapeutic implications for overcoming radiation resistance in cancer therapy. Int J Mol Sci. (2015) 16:26880–913. doi: 10.3390/ijms161125991
9. Maier P, Hartmann L, Wenz F, Herskind C. Cellular pathways in response to ionizing radiation and their targetability for tumor radiosensitization. Int J Mol Sci. (2016) 17:1–32 doi: 10.3390/ijms17010102
10. Moncharmont C, Levy A, Guy JB, Falk AT, Guilbert M, Trone JC, et al. Radiation-enhanced cell migration/invasion process: a review. Crit Rev Oncol Hematol. (2014) 92:133–42. doi: 10.1016/j.critrevonc.2014.05.006
11. Wild-Bode C, Weller M, Rimner A, Dichgans J, Wick W. Sublethal irradiation promotes migration and invasiveness of glioma cells: implications for radiotherapy of human glioblastoma. Cancer Res. (2001) 61:2744−50.
12. Su WH, Chuang PC, Huang EY, Yang KD. Radiation-induced increase in cell migration and metastatic potential of cervical cancer cells operates via the K-Ras pathway. Am J Pathol. (2012) 180:862–71. doi: 10.1016/j.ajpath.2011.10.018
13. Vilalta M, Rafat M, Graves EE. Effects of radiation on metastasis and tumor cell migration. Cell Mol Life Sci. (2016) 73:2999–3007. doi: 10.1007/s00018-016-2210-5
14. Fujita M, Imadome K, Shoji Y, Isozaki T, Endo S, Yamada S, et al. Carbon-ion irradiation suppresses migration and invasiveness of human pancreatic carcinoma cells MIAPaCa-2 via Rac1 and RhoA degradation. Int J Radiat Oncol Biol Phys. (2015) 93:173–80. doi: 10.1016/j.ijrobp.2015.05.009
15. Moncharmont C, Guy JB, Wozny AS, Gilormini M, Battiston-Montagne P, Ardail D, et al. Carbon ion irradiation withstands cancer stem cells' migration/invasion process in Head and Neck Squamous Cell Carcinoma (HNSCC). Oncotarget. (2016) 7:47738–49. doi: 10.18632/oncotarget.10281
16. Walenta S, Mueller-Klieser W. Differential superiority of heavy charged-particle irradiation to X-rays: studies on biological effectiveness and side effect mechanisms in multicellular tumor and normal tissue models. Front Oncol. (2016) 6:30. doi: 10.3389/fonc.2016.00030
17. Kwon YS, Lee KS, Chun SY, Jang TJ, Nam KS. Suppressive effects of a proton beam on tumor growth and lung metastasis through the inhibition of metastatic gene expression in 4T1 orthotopic breast cancer model. Int J Oncol. (2016) 49:336–42. doi: 10.3892/ijo.2016.3520
18. Lee KS, Lee DH, Chun SY, Nam KS. Metastatic potential in MDA-MB-231 human breast cancer cells is inhibited by proton beam irradiation via the Akt/nuclear factor-kappaB signaling pathway. Mol Med Rep. (2014) 10:1007–12. doi: 10.3892/mmr.2014.2259
19. Suetens A, Moreels M, Quintens R, Soors E, Buset J, Chiriotti S, et al. Dose- and time-dependent gene expression alterations in prostate and colon cancer cells after in vitro exposure to carbon ion and X-irradiation. J Radiat Res. (2015) 56:11–21. doi: 10.1093/jrr/rru070
20. Suetens A, Moreels M, Quintens R, Chiriotti S, Tabury K, Michaux A, et al. Carbon ion irradiation of the human prostate cancer cell line PC3: A whole genome microarray study. Int J Oncol. (2014) 44:1056–72. doi: 10.3892/ijo.2014.2287
21. Gonnissen A, Isebaert S, Haustermans K. Hedgehog signaling in prostate cancer and its therapeutic implication. Int J Mol Sci. (2013) 14:13979–4007. doi: 10.3390/ijms140713979
22. Karhadkar SS, Bova GS, Abdallah N, Dhara S, Gardner D, Maitra A, et al. Hedgehog signalling in prostate regeneration, neoplasia and metastasis. Nature. (2004) 431:707–12. doi: 10.1038/nature02962
23. Chang L, Zhao D, Liu HB, Wang QS, Zhang P, Li CL, et al. Activation of sonic hedgehog signaling enhances cell migration and invasion by induction of matrix metalloproteinase-2 and−9 via the phosphoinositide-3 kinase/AKT signaling pathway in glioblastoma. Mol Med Rep. (2015) 12:6702–10. doi: 10.3892/mmr.2015.4229
24. Magistri P, Battistelli C, Strippoli R, Petrucciani N, Pellinen T, Rossi L, et al. SMO inhibition modulates cellular plasticity and invasiveness in colorectal cancer. Front Pharmacol. (2017) 8:956. doi: 10.3389/fphar.2017.00956
25. Yue D, Li H, Che J, Zhang Y, Tseng HH, Jin JQ, et al. Hedgehog/Gli promotes epithelial-mesenchymal transition in lung squamous cell carcinomas. J Exp Clin Cancer Res. (2014) 33:34. doi: 10.1186/1756-9966-33-34
26. Chen YJ, Lin CP, Hsu ML, Shieh HR, Chao NK, Chao KS. Sonic hedgehog signaling protects human hepatocellular carcinoma cells against ionizing radiation in an autocrine manner. Int J Radiat Oncol Biol Phys. (2011) 80:851–9. doi: 10.1016/j.ijrobp.2011.01.003
27. Chen YJ, Sims-Mourtada J, Izzo J, Chao KS. Targeting the hedgehog pathway to mitigate treatment resistance. Cell Cycle. (2007) 6:1826–30. doi: 10.4161/cc.6.15.4545
28. Shafaee Z, Schmidt H, Du W, Posner M, Weichselbaum R. Cyclopamine increases the cytotoxic effects of paclitaxel and radiation but not cisplatin and gemcitabine in Hedgehog expressing pancreatic cancer cells. Cancer Chemother Pharmacol. (2006) 58:765–70. doi: 10.1007/s00280-006-0227-4
29. Zeng J, Aziz K, Chettiar ST, Aftab BT, Armour M, Gajula R, et al. Hedgehog pathway inhibition radiosensitizes non-small cell lung cancers. Int J Radiat Oncol Biol Phys. (2013) 86:143–9. doi: 10.1016/j.ijrobp.2012.10.014
30. Gonnissen A, Isebaert S, McKee CM, Muschel RJ, Haustermans K. The effect of metformin and GANT61 combinations on the radiosensitivity of prostate cancer cells. Int J Mol Sci. (2017) 18. doi: 10.3390/ijms18020399
31. Sims-Mourtada J, Izzo JG, Apisarnthanarax S, Wu TT, Malhotra U, Luthra R, et al. Hedgehog: an attribute to tumor regrowth after chemoradiotherapy and a target to improve radiation response. Clin Cancer Res. (2006) 12:6565–72. doi: 10.1158/1078-0432.ccr-06-0176
32. Danhof R, Lewis K, Brown M. Small molecule inhibitors of the hedgehog pathway in the treatment of basal cell carcinoma of the skin. Am J Clin Dermatol. (2018) 19:195–207. doi: 10.1007/s40257-017-0319-4
33. Gonnissen A, Isebaert S, Haustermans K. Targeting the Hedgehog signaling pathway in cancer: beyond Smoothened. Oncotarget. (2015) 6:13899–913. doi: 10.18632/oncotarget.4224
34. Gonnissen A, Isebaert S, McKee CM, Dok R, Haustermans K, Muschel RJ. The hedgehog inhibitor GANT61 sensitizes prostate cancer cells to ionizing radiation both in vitro and in vivo. Oncotarget. (2016) 7:84286–98. doi: 10.18632/oncotarget.12483
35. Teichman J, Dodbiba L, Thai H, Fleet A, Morey T, Liu L, et al. Hedgehog inhibition mediates radiation sensitivity in mouse xenograft models of human esophageal adenocarcinoma. PLoS ONE. (2018) 13:e0194809. doi: 10.1371/journal.pone.0194809
36. Tsai CL, Hsu FM, Tzen KY, Liu WL, Cheng AL, Cheng JC. Sonic Hedgehog inhibition as a strategy to augment radiosensitivity of hepatocellular carcinoma. J Gastroenterol Hepatol. (2015) 30:1317–24. doi: 10.1111/jgh.12931
37. Franco AI, Eastwick G, Farah R, Heyboer M, Lee M, Aridgides P. Upfront radiotherapy with concurrent and adjuvant vismodegib is effective and well-tolerated in a patient with advanced, multifocal basal cell carcinoma. Case Rep Dermatol Med. (2018) 2018:2354146 doi: 10.1155/2018/2354146
38. Schulze B, Meissner M, Ghanaati S, Burck I, Rodel C, Balermpas P. Hedgehog pathway inhibitor in combination with radiation therapy for basal cell carcinomas of the head and neck: first clinical experience with vismodegib for locally advanced disease. Strahlenther Onkol. (2016) 192:25–31. doi: 10.1007/s00066-015-0902-7
39. Pollom EL, Bui TT, Chang AL, Colevas AD, Hara WY. Concurrent vismodegib and radiotherapy for recurrent, advanced basal cell carcinoma. JAMA Dermatol. (2015) 151:998–1001. doi: 10.1001/jamadermatol.2015.0326
40. Raleigh DR, Algazi A, Arron ST, Neuhaus IM, Yom SS. Induction Hedgehog pathway inhibition followed by combined-modality radiotherapy for basal cell carcinoma. Br J Dermatol. (2015) 173:544–6. doi: 10.1111/bjd.13748
41. Tang N, Ratner D. Implementation of systemic Hedgehog inhibitors in daily practice as neoadjuvant therapy. J Natl Compr Canc Netw. (2017) 15:537–43. doi: 10.6004/jnccn.2017.0051
42. Zhou J, Wu K, Gao D, Zhu G, Wu D, Wang X, et al. Reciprocal regulation of hypoxia-inducible factor 2alpha and GLI1 expression associated with the radioresistance of renal cell carcinoma. Int J Radiat Oncol Biol Phys. (2014) 90:942–51. doi: 10.1016/j.ijrobp.2014.06.065
43. Combs SE, Zips D. “Clinical rationale and indications for particle therapy,” in Advances in Radiation Therapy, Guckenberger M, editor. Karger (2018). doi: 10.1159/000486997
44. Curran T. Reproducibility of academic preclinical translational research: lessons from the development of Hedgehog pathway inhibitors to treat cancer. Open Biol. 8:180098. doi: 10.1098/rsob.180098
45. Zhang R, Newhauser WD. Calculation of water equivalent thickness of materials of arbitrary density, elemental composition and thickness in proton beam irradiation. Phys Med Biol. (2009) 54:1383–95. doi: 10.1088/0031-9155/54/6/001
46. Berger MJ, Inokuti M, Andersen HH, Bichsel H, Powers D, Seltzer SM, et al. Report 49. J Int Com Rad Units Measur. (1993) os25:258–79. doi: 10.1093/jicru/os25.2.Report49
47. Debrot E, Tran L, Chartier L, Bolst D, Guatelli S, Vandevoorde C, et al. SOI microdosimetry and modified MKM for evaluation of relative biological effectiveness for a passive proton therapy radiation field. Phys Med Biol. (2018) 63:235007. doi: 10.1088/1361-6560/aaec2f
48. Durantel F, Balanzat E, Cassimi A, Chevalier F, Ngono-Ravache Y, Madi T, et al. Dosimetry for radiobiology experiments at GANI. Nuclear Instruments Methods Phys Res Sec A. (2016) 816:70–7. doi: 10.1016/j.nima.2016.01.052
49. Friedrich T, Scholz U, Elsasser T, Durante M, Scholz M. Systematic analysis of RBE and related quantities using a database of cell survival experiments with ion beam irradiation. J Radiat Res. (2013) 54:494–514. doi: 10.1093/jrr/rrs114
50. Fujisawa H, Genik PC, Kitamura H, Fujimori A, Uesaka M, Kato TA. Comparison of human chordoma cell-kill for 290 MeV/n carbon ions versus 70 MeV protons in vitro. Radiat Oncol. (2013) 8:91. doi: 10.1186/1748-717x-8-91
51. Jones B. Why RBE must be a variable and not a constant in proton therapy. Br J Radiol. (2016) 89:20160116. doi: 10.1259/bjr.20160116
52. Paganetti H. Relative biological effectiveness (RBE) values for proton beam therapy. Variations as a function of biological endpoint, dose, and linear energy transfer. Phys Med Biol. (2014) 59:R419–72. doi: 10.1088/0031-9155/59/22/r419
53. Suetens A, Konings K, Moreels M, Quintens R, Verslegers M, Soors E, et al. Higher initial DNA damage and persistent cell cycle arrest after carbon ion irradiation compared to X-irradiation in prostate and colon cancer cells. Front Oncol. (2016) 6:87. doi: 10.3389/fonc.2016.00087
54. Masunaga S, Uzawa A, Hirayama R, Matsumoto Y, Sakurai Y, Tanaka H, et al. The Effect of p53 status of tumor cells on radiosensitivity of irradiated tumors with carbon-ion beams compared with γ-rays or reactor neutron beams. World J Oncol. (2015) 6:398–409. doi: 10.14740/wjon941w
55. Fokas E, You A, Juricko J, Engenhart-Cabillic R, An HX. Genetic alterations after carbon ion irradiation in human lung adenocarcinoma cells. Int J Oncol. (2011) 38:161–8. doi: 10.3892/ijo-00000835
56. Yamamoto N, Ikeda C, Yakushiji T, Nomura T, Katakura A, Shibahara T, et al. Genetic effects of X-ray and carbon ion irradiation in head and neck carcinoma cell lines. Bull Tokyo Dent Coll. (2007) 48:177–85. doi: 10.2209/tdcpublication.48.177
57. Girdhani S, Sachs R, Hlatky L. Biological effects of proton radiation: what we know and don't know. Radiat Res. (2013) 179:257–72. doi: 10.1667/rr2839.1
58. Fushimi K, Uzawa K, Ishigami T, Yamamoto N, Kawata T, Shibahara T, et al. Susceptible genes and molecular pathways related to heavy ion irradiation in oral squamous cell carcinoma cells. Radiother Oncol. (2008) 89:237–244. doi: 10.1016/j.radonc.2008.04.015
59. S.-Chun Y, Kim S, K.-Nam S, Lee KS. Anti-metastatic potential of a proton beam is regulated by p38 MAPK/c-Fos signaling pathway in TPA-treated HepG2 human hepatocellular carcinoma. Biomed Pharmacother. (2018) 99:904–12. doi: 10.1016/j.biopha.2018.01.134
60. Fujita M, Yamada S, Imai T. Irradiation induces diverse changes in invasive potential in cancer cell lines. Semin Cancer Biol. (2015)35:45–52. doi: 10.1016/j.semcancer.2015.09.003
61. Rieken S, Rieber J, Brons S, Habermehl D, Rief H, Orschiedt L, et al. Radiation-induced motility alterations in medulloblastoma cells. J Rad Res. (2015) 56:430–436. doi: 10.1093/jrr/rru120
62. Asuthkar S, Nalla AK, Gondi CS, Dinh DH, Gujrati M, Mohanam S, et al. Gadd45a sensitizes medulloblastoma cells to irradiation and suppresses MMP-9–mediated EM. Neuro-Oncol. T. (2011) 13:1059–73. doi: 10.1093/neuonc/nor109
63. Nalla AK, Asuthkar S, Bhoopathi P, Gujrati M, Dinh DH, Rao JS. Suppression of uPAR retards radiation-induced invasion and migration mediated by integrin β1/FAK signaling in medulloblastoma. PLoS ONE. (2010) 5:e13006. doi: 10.1371/journal.pone.0013006
64. Zaboronok A, Isobe T, Yamamoto T, Sato E, Takada K, Sakae T, et al. Proton beam irradiation stimulates migration and invasion of human U87 malignant glioma cells. J Radiat Res. (2014) 55:283–7. doi: 10.1093/jrr/rrt119
Keywords: particle therapy, proton, carbon ion, radiosensitization, Hedgehog pathway, migration, gene expression, GANT61
Citation: Konings K, Vandevoorde C, Belmans N, Vermeesen R, Baselet B, Walleghem MV, Janssen A, Isebaert S, Baatout S, Haustermans K and Moreels M (2019) The Combination of Particle Irradiation With the Hedgehog Inhibitor GANT61 Differently Modulates the Radiosensitivity and Migration of Cancer Cells Compared to X-Ray Irradiation. Front. Oncol. 9:391. doi: 10.3389/fonc.2019.00391
Received: 07 November 2018; Accepted: 26 April 2019;
Published: 14 May 2019.
Edited by:
Anatoly Dritschilo, Georgetown University, United StatesReviewed by:
Emanuele Scifoni, Istituto Nazionale di Fisica Nucleare (INFN), ItalyCopyright © 2019 Konings, Vandevoorde, Belmans, Vermeesen, Baselet, Walleghem, Janssen, Isebaert, Baatout, Haustermans and Moreels. This is an open-access article distributed under the terms of the Creative Commons Attribution License (CC BY). The use, distribution or reproduction in other forums is permitted, provided the original author(s) and the copyright owner(s) are credited and that the original publication in this journal is cited, in accordance with accepted academic practice. No use, distribution or reproduction is permitted which does not comply with these terms.
*Correspondence: Marjan Moreels, bWFyamFuLm1vcmVlbHNAc2NrY2VuLmJl
Disclaimer: All claims expressed in this article are solely those of the authors and do not necessarily represent those of their affiliated organizations, or those of the publisher, the editors and the reviewers. Any product that may be evaluated in this article or claim that may be made by its manufacturer is not guaranteed or endorsed by the publisher.
Research integrity at Frontiers
Learn more about the work of our research integrity team to safeguard the quality of each article we publish.