- 1Department of Medical and Surgical Sciences, University of Bologna, Bologna, Italy
- 2Center for Applied Biomedical Research (CRBA), S. Orsola-Malpighi Hospital, University of Bologna, Bologna, Italy
- 3Department of Agricultural Sciences, University of Naples, Portici, Italy
- 4Food Quality and Design Group, Wageningen University, Wageningen, Netherlands
- 5Department of Pharmacy and Biotechnology, University of Bologna, Bologna, Italy
- 6Pathology Unit, S. Orsola-Malpighi Hospital, University of Bologna, Bologna, Italy
- 7Gastroenterology Unit, S. Orsola-Malpighi Hospital, University of Bologna, Bologna, Italy
Objectives: Unhealthy dietary patterns have been associated with colorectal cancer (CRC) onset while Mediterranean Diet (MD) has been proposed for CRC prevention. This study evaluated the effect of a Mediterranean Diet Mix (MD-MIX) on colonic tumors development in A/J mice fed a low-fat (LFD) or a high-fat western diet (HFWD), and injected with the procarcinogen azoxymethane (AOM).
Materials and Methods: Forty A/J male mice were randomly assigned into four feeding arms (10 mice/arm; LFD, LFD-MD-MIX, HFWD, HFWD-MD-MIX) to be treated with AOM. Ten mice were exposed to the diets alone (Healthy LFD and Healthy HFWD) to be used as control. Tumor incidence and multiplicity were evaluated at sacrifice. Mucosal fatty acid content and urinary phenolic compounds were assayed by mass spectrometry. Apoptosis was evaluated by TUNEL assay and gene expression markers. Cell proliferation was evaluated by Ki67 immunohistochemistry. Microbiota composition was assessed at different time points by 16S RNA sequencing.
Results: A tumor incidence of 100% was obtained in AOM-treated mice. The MD-MIX supplementation was able to reduce the number of colonic lesions in both LFD and HFWD-fed mice and to induce apoptosis, in particular in the LFD-MD-MIX arm. Moreover, a preventive effect on low-grade dysplasia and macroscopical lesions (>1 mm) development was found in HFWD-fed mice together with a regulation of the AOM-driven intestinal dysbiosis.
Conclusions: MD-MIX was able to counteract CRC development in mice under different dietary backgrounds through the regulation of apoptosis and gut microbiota.
Introduction
Colorectal cancer (CRC) remains one of the most frequently diagnosed malignancies worldwide with 1.8 million new cases estimated in 2018 (1). Globally, CRC incidence greatly diverge among different geographic areas reaching the highest rate of incidence in developed countries where a high-fat western dietary pattern is largely adopted (2). In particular, the highest CRC incidence rates are found in Australia/New Zealand, Northern America, Eastern Asia and some parts of Europe (e.g., Hungary and Norway) (1).
A clear relationship between long-term unhealthy dietary habits and increased risk of developing CRC is widely recognized (3), while prudent dietary patterns have been associated to CRC prevention (4). Among healthy dietary patterns, a pescovegetarian diet has been correlated with an overall lower CRC incidence highlighting the importance of fruits, vegetables, cereals, and fish consumption for CRC risk reduction (5). Moreover, it is known that Mediterranean Diet (MD), which is characterized by a daily consumption of whole grains, legumes, nuts, olive oil, fish, fruits, and vegetables protects against colonic adenoma recurrence and CRC risk in different cohort studies (6–8). Importantly, data obtained from the European Prospective Investigation into Cancer and nutrition (EPIC) study involving 10 European countries and about 520,000 healthy subjects (aged 25–70 years) showed that adherence to Mediterranean Diet was associated with a reduction of CRC risk (8–11%) (9). The preventive effects of these prudent dietary patterns on CRC are mainly associated with the high content of polyphenols and ω-3 polyunsaturated fatty acids (ω-3-PUFAs) (10–12). Some of the phenolic compounds commonly found in the MD are: catechins (from apples and walnuts), ferulic acid (from whole grain), naringenin (from tomatoes), and hydroxytyrosol (from olive oil) (13). We previously demonstrated that polyphenols extracted from annurca apple as well as the ω-3-PUFA Eicosapentaenoic acid as free fatty acid (EPA-FFA) were able to exert chemopreventive properties in the ApcMin/+ mouse model of familial adenomatous polyposis (14, 15). Also, the anticancer effect of walnut consumption have been recently demonstrated in the Azoxymethane (AOM) mouse model of CRC (16).
There is mounting evidence that the gut microbiota composition is critically influenced by dietary habits and that an imbalance in the relative abundance of specific bacterial strains may have a pivotal role in CRC initiation and progression (17). Recently, the impact of MD on the gut microbiota structure has been investigated in humans demonstrating that the beneficial effects associated with MD may be driven by changes in the gut microbiota composition (18, 19).
Although the anticarcinogenic properties of single food-derived compounds have been evaluated in CRC (20), the combined effect of food bioactives, typically consumed in the MD regimen, has not been investigated so far.
In this study, we hypothesized that extracts from MD components in combination with the fish-derived EPA-FFA (referred as MD-MIX) may be able to counteract the development of CRC in AOM-treated mice undergoing different dietary protocols. We found that the MD-MIX prevented the development of colonic malignant lesions mainly acting on apoptosis in mice fed a low-fat diet (LFD) or modulating the gut microbiota structure in mice fed a high-fat western diet (HFWD).
Materials and Methods
Mediterranean Diet Mix Composition
The Mediterranean Diet Mix (MD-MIX) was obtained by combining the ω-3 PUFA EPA-FFA (provided in the diet as outlined in the next paragraph) and a mix of bioactive phytochemicals extracts resembling the main categories constituting the Mediterranean diet and contributing to the total phytochemicals intake (Annurca apples 25%, cherry tomatoes 25%, walnuts 15%, wholegrain wheat 15%, and olive oil 20%). Phytochemicals sources and quantity were selected in order to have a good balance of the different families of polyphenols (flavanols, anthocyanins, ellagitannins, secoiridoids).
The polyphenol profile of the mix of extracts was characterized (see Supplementary Methods) and its concentration was expressed as equivalents of the most represented polyphenols: catechins (from apples and walnuts), ferulic acid (from wholegrain wheat), naringenin (from tomatoes), and hydroxytyrosol (from olive oil) (Supplementary Table 1). To preserve polyphenols stability a stock solution (1 mmol/L; 80:20 water: ethanol) was prepared, aliquoted and stored at −80°C until use.
Animals and Diets
Animal experiments were performed in accordance with the guidelines and regulations of the University of Bologna Animal Welfare Committee. The research protocol was approved by the Italian Institute of Health (approval number 203/2016-PR).
Fifty A/J male mice (5 weeks old) were purchased from Jackson Lab (Sacramento, CA, USA) and housed in individually ventilated cages (five mice/cage) in a temperature and humidity controlled animal facility with a 12 h light/dark cycle.
After a short period of acclimatization (1 week), mice were randomly assigned to the following feeding protocols (Figure 1): (1) low-fat diet (LFD: 12% calories from fat; normal calcium, cellulose, and vitamin D3 content; 3.75 kcal/g) and drinking water (Healthy LFD; n = 5); (2) high-fat western diet (HFWD: 45% calories from fat; low calcium, cellulose, vitamin D3 content; 4.98 kcal/g), and drinking water (Healthy HFWD; n = 5); (3) LFD and drinking water containing the extracts vehicle ethanol (LFD n = 10); (4) LFD in which soybean oil was substituted for 1% highly-purified EPA-FFA (ALFA, SLA Pharma AG, Switzerland), and drinking the mix of extracts (LFD-MD-MIX n = 10); (5) HFWD and drinking water containing the extracts vehicle ethanol (HFWD n = 10); (6) HFWD in which soybean oil was substituted for 1% highly-purified EPA-FFA and drinking the mix of extracts (HFWD-MD-MIX n = 10). Diets were formulated by Charles River (Lecco, Italy; see Supplementary Table 2). The beverage containing the mix of extracts was freshly prepared every day by diluting the stock solution 1:100 in water (final concentration: 10 μmol/L polyphenol equivalents). The final ethanol concentration was 0.2%. For carcinogenesis induction, LFD, LFD-MD-MIX, HFWD, and HFWD-MD-MIX arms (16-week old mice) received 8-weekly intraperitoneal injections of AOM (#A5486; Sigma-Aldrich; St. Louis, MO) under mild sedation (Figure 1). The same AOM lot (#SLBN5975V) was used for the entire experiment. Moreover, to guarantee its stability, the reagent was aliquoted and stored at −80°C until use. The first AOM injection was performed at 10 mg/kg, while all the following were performed at 7.5 mg/kg in order to reduce the risk of mortality observed after the first injection. Healthy LFD and Healthy HFWD arms did not receive AOM injections.
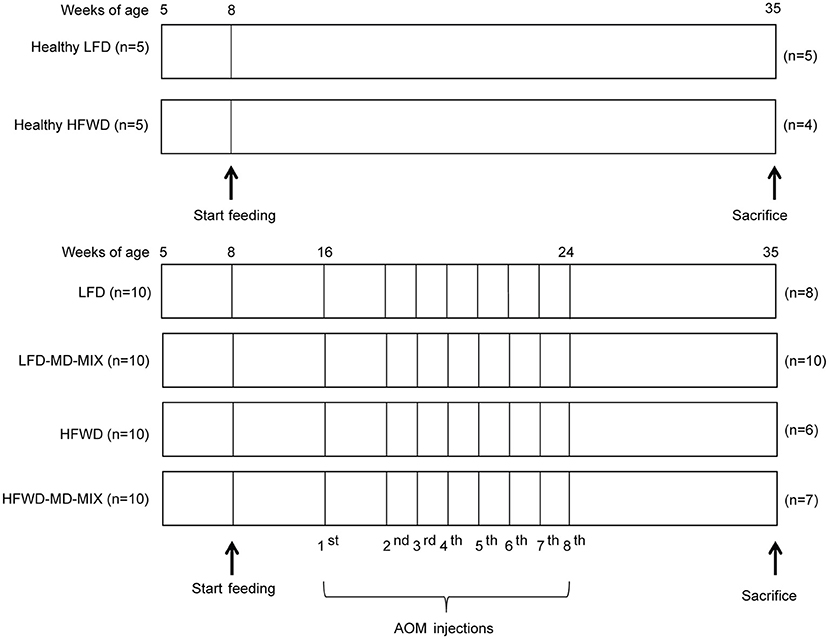
Figure 1. Feeding and carcinogenesis protocol. After acclimation (age 8 weeks) feeding protocols were started and maintained for 27 weeks. From week 16 LFD, LFD-MD-MIX, HFWD, and HFWD-MD-MIX mice were subjected to eight intraperitoneal AOM injections. The number of mice “n” in each arm is shown.
Body weight was monitored weekly, while rectal bleeding and diarrhea daily. Nineteen weeks after the first AOM injection, mice were euthanized through isofluorane overdose followed by cardiac puncture. Urine was collected at sacrifice by bladder aspiration and stored at −80°C. The colon was immediately removed, opened longitudinally, washed with ice-cold phosphate buffered saline, and its length was recorded. The number, location and size of macroscopical lesions was determined using a stereomicroscope and measured using a digital caliper. Fresh tissue samples from normal colonic mucosa (1 cm length) were collected from middle colon and stored at −80°C for molecular analyses or formalin-fixed and paraffin-embedded (FFPE) for immunohistochemistry (IHC). Then, the remaining colon was fixed in Bouin solution and paraffin-embedded to be processed for histopathology (21).
Histopathology
Histopathological evaluation was performed by one expert blinded pathologist (TB) on multiple hematoxylin and eosin stained sections obtained from the whole colon of each mouse. Dysplastic areas were classified as low-grade dysplasia (LGD), high-grade dysplasia (HGD), or adenocarcinoma (AdenoK).
Mucosal Fatty Acid Analysis
Mucosal fatty acid content was determined by gas chromatography-mass spectrometry (GC-MS) on fresh tissue samples as previously described (22).
Determination of Urinary Polyphenols
Polyphenols were extracted from urine samples as previously reported (23) with minor modifications (see Supplementary Methods). The molecular formula and the selected ion for quantified polyphenols are reported in Supplementary Table 3.
Immunohistochemistry and TUNEL Assay
For IHC, FFPE colonic tissues were sectioned at 4 μm thickness and processed as previously reported, using Ki67 primary antibody (1:400 Cell Signaling Technology #9027). The percentage of Ki67+ cells was obtained dividing the number of positive nuclei on total nuclei on 15 colonic crypts (when possible longitudinal crypts were selected). TUNEL assay was performed using DeadEnd™ Fluorometric TUNEL System (Promega, Madison, WI, USA) on FFPE normal colonic tissues. For quantitative analysis of TUNEL, ImageJ software (https://imagej.nih.gov/ij/), coupled with the Color Deconvolution plug-in, was used to quantify the percentage of positively stained nuclear area as previously (24).
RNA Extraction and Quantitative Real-Time PCR
Total RNA was extracted from fresh normal colonic tissues using TRIzol reagent (Invitrogen™, Thermo Fisher Scientific) and RNA concentration was measured using the Nanodrop 1,000 spectrophotometer (Thermo Fisher Scientific). Then, total RNA was reverse transcribed using the High-Capacity RNA-to-cDNA™ Kit (Applied Biosystems™, Thermo Fisher Scientific) according to the manufacturer's instructions. Quantitative real-time PCR (q-PCR) reactions were performed on ICycler thermal cycler (Biorad, Hercules, USA) using the SYBR®Select Master Mix for CFX and PrimeTime qPCR Primers for Bax (Mm.PT.58.14012210) and Bcl2 (Mm.PT.58.7362966) (Integrated DNA Technologies, Coralville, USA) genes. Gapdh was used as reference gene for mRNA normalization. Fold induction levels were obtained using the 2−ΔΔCt method by normalizing against the reference gene. Data were plotted as Bax/Bcl2 ratio.
Microbiota Analysis and Bioinformatics
For microbiota analysis fecal samples were collected from mice at different time points: 9 (T1: 1 week after the beginning of the feeding protocol), 17 (T2: 1 week after the first AOM injection), and 35 (T3: at the sacrifice) weeks of age. Feces from mice grouped in the same cage were pooled at each time point and total bacterial DNA was extracted using the QIAmp DNA Stool Mini Kit (Qiagen, Hilden, Germany). Microbiota data were obtained by sequencing the V3-V4 region of the 16S rRNA gene by using an Illumina platform. For each sample, the V3-V4 region of the 16S rRNA gene was PCR amplified using primers carrying Illumina overhang adapter sequences (25). Amplicons were purified with a magnetic bead-based clean-up system (Agencourt AMPure XP; Beckman Coulter, CA). Indexed libraries were prepared by limited-cycle PCR using Nextera technology and cleaned up with AMPure XP magnetic beads. Libraries were pooled at equimolar concentrations (4 nM), denatured and diluted to 6 pM before loading onto the MiSeq flow cell. A 2 × 300 bp paired end protocol was used, according to the manufacturer's instructions (Illumina, San Diego, CA). Raw sequences were processed using a pipeline combining PANDAseq and QIIME (26, 27). High-quality reads, as selected using the default values in QIIME, were binned into operational taxonomic units (OTUs) according to the taxonomic threshold of 97% using UCLUST (28), through an open-reference strategy. Taxonomy was assigned using the RDP classifier against Greengenes database (May 2013 release). The percentages of OTUs assigned to the different bacterial phylogenetic groups (from genus to phylum) were calculated as relative abundances for all samples. Average abundances were calculated for each time point and for all diet combinations. Values for bacterial genera present at a relative abundance >0.05% in at least one sample were used to produce a heatmap with horizontal scaling, plotting the z score (i.e., number of standard deviations from the mean, from −3 to 3) for each time point. The heatmap was generated using the function heatplot within the R package MADE4.
Beta diversity was estimated by computing weighted UniFrac distances, which were used for Principal Coordinates Analysis (PCoA) performed using vegan package in R statistical software.
Statistical Analysis
Statistical analysis was performed using Graphpad 6.0 Software (GraphPad Software Inc., CA, USA). The mean differences among groups were calculated using one-way two-tailed ANOVA followed by Tukey's multiple comparison post-hoc test. P < 0.05 were considered statistically significant.
Results
MD-MIX Counteracts CRC Development
The primary endpoint of this study was to evaluate the chemopreventive effect of the MD-MIX on colon carcinogenesis in AOM-injected A/J mice fed a LFD or HFWD. The A/J strain was chosen given its high sensitivity to AOM-driven colon carcinogenesis (29). Tumor incidence was 100% in AOM-exposed mice, while no tumors were observed in both LFD and HFWD healthy mice indicating that HFWD alone is not sufficient to induce tumor development during a lifespan of 27 weeks. Representative images of colons at sacrifice are reported in Figure 2A. Importantly, MD-MIX feeding significantly reduced tumor multiplicity in the LFD-MD-MIX group respect to the LFD arm and also protected against colonic lesions development in HFWD-fed mice (Figure 2B). Noteworthy, HFWD group showed a significant higher number of lesions compared to LFD-MD-MIX arm (Figure 2B). Analyzing the effect of the diet (LFD or HFWD) or MIX on the number of lesions, we found that the MIX significantly affects the number of lesions in mice fed both diets with no interaction between diet and MIX. Regarding lesion size, HFWD arm displayed a significant higher number of lesions larger than 1 mm compared to LFD and LFD-MD-MIX arms (Figure 2C). Treatment with the MD-MIX in HFWD-fed mice was associated with a significant lower number of lesions larger than 1 mm while no significant differences in terms of lesions smaller than 1 mm were observed among all groups (Figure 2C). Interestingly, histological analysis showed a higher number of LGD lesions in the HFWD arm, which was partially counteracted by the MD-MIX (Figure 2D). No significant changes were observed in the advanced lesions (high grade dysplasia and adenocarcinoma) among all groups (Figure 2E).
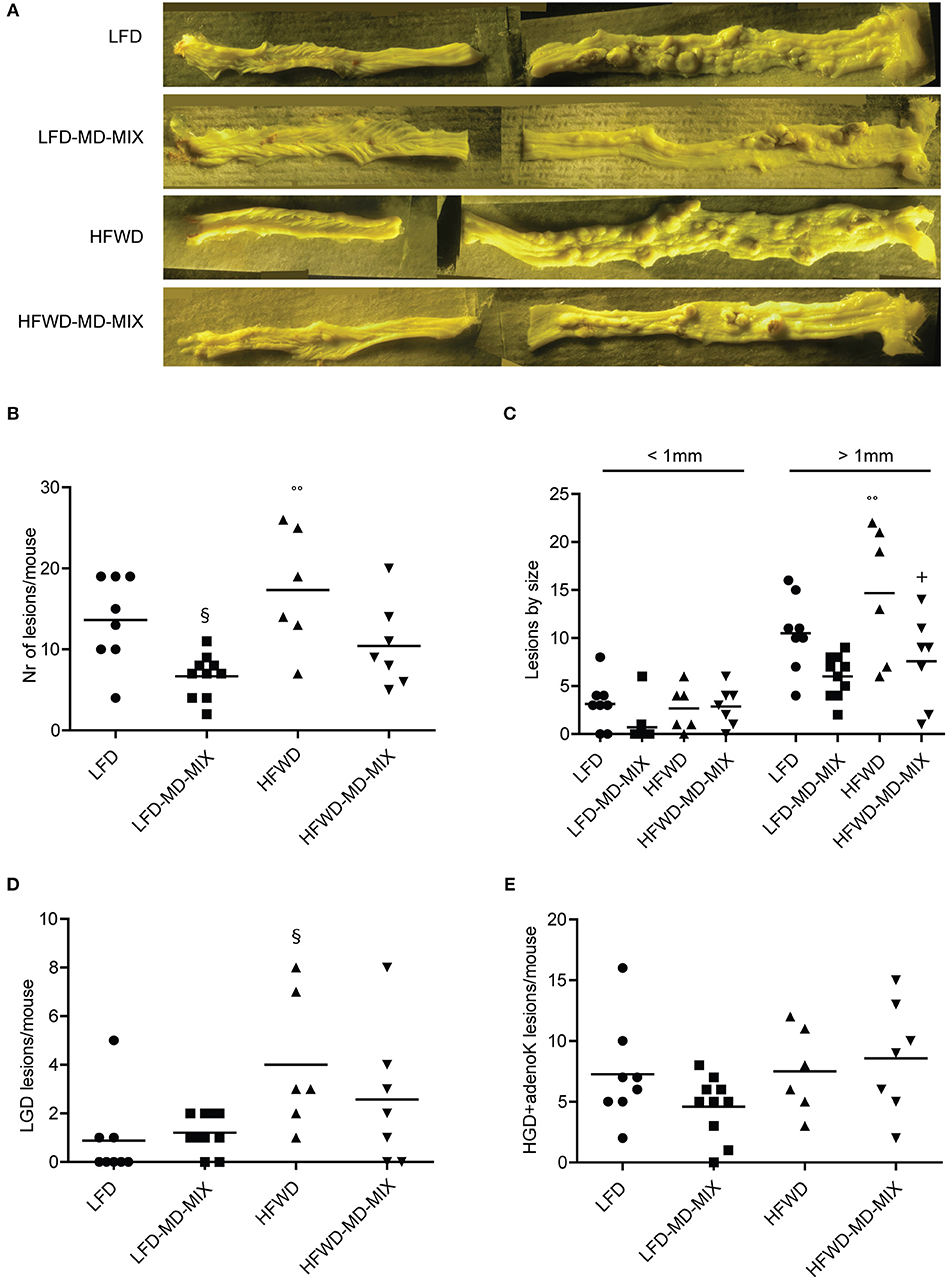
Figure 2. Effect of MD-MIX on tumor multiplicity, size and histological distribution of colonic lesions. (A) Representative images of colons fixed in Bouin's solution; (B) Tumor multiplicity (number of macroscopic lesions/mouse) (ANOVA p = 0.0027); (C) Number of macroscopic colonic lesions by size (ANOVA p = 0.0052 for lesions >1 mm); (D) Number of low grade dysplasia (LGD) lesions/mouse (ANOVA p = 0.0329); (E) Number of high grade dysplasia+ adenocarcinoma lesions (HGD+adenoK)/mouse. Data are shown as individual values. n = 8 LFD; n = 10 LFD-MD-MIX; n = 6 HFWD; n = 7 HFWD-MD-MIX. P-values were determined using one-way two-tailed ANOVA followed by Tukey's multiple comparison post-hoc test. §p < 0.05 vs. LFD; °°p < 0.01 vs. LFD-MD-MIX; +p < 0.05 vs. HFWD.
Colon length, which provides an indication of the severity of colonic inflammation (30), was significantly reduced upon AOM treatment in both LFD and HFWD groups compared with healthy LFD mice. Noteworthy, the exposure to the MD-MIX partially counteracted colon length shortening induced by the AOM treatment (Supplementary Figure 1).
Taken together our data demonstrate a protective effect of the MD-MIX against AOM-driven colon carcinogenesis.
Effect of Dietary Protocols on Body Weight, Colonic EPA Incorporation and Urinary Polyphenol Content
We did not observe a significant weight increase in mice fed a HFWD compared with LFD-fed mice (Supplementary Figure 2). Moreover, AOM exposition was associated with a significant weight reduction in all treated mice independently from the diet (Supplementary Figure 2).
Colonic EPA incorporation into cellular membranes and urinary polyphenols were measured to evaluate the bioavailability of EPA and polyphenols in MD-MIX treated mice and to investigate the effect of diets (LFD vs. HFWD) and colonic carcinogenesis (AOM treated vs. healthy mice) on their metabolism. As shown in Figure 3, LFD-MD-MIX and HFWD-MD-MIX arms displayed a significant increase in the percentage content of EPA in colonic cell membranes compared to all other arms. Noteworthy, EPA levels in LFD-MD-MIX group were also significantly higher than those in HFWD-MD-MIX arm.
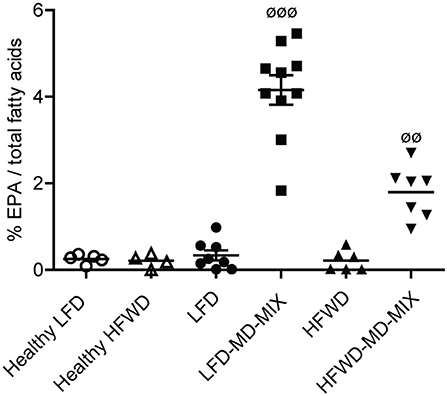
Figure 3. EPA incorporation on colonic tissues. Eicosapentaenoic acid (EPA; C20:5 n-3) levels in colonic tissues expressed as percentage of total fatty acids (ANOVA p < 0.0001). Data are shown as individual values. n = 5 Healthy LFD; n = 4 Healthy HFWD; n = 8 LFD; n = 10 LFD-MD-MIX; n = 6 HFWD; n = 7 HFWD-MD-MIX. P-values were obtained using one-way two-tailed ANOVA followed by Tukey's multiple comparison post-hoc test.øøp < 0.01 HFWD-MD-MIX vs. all groups;øøøp < 0.001 LFD-MD-MIX vs. all groups.
Fourteen polyphenols were retrieved in urine samples (Supplementary Table 4). Unexpectedly, HFWD, but not LFD feeding, was associated with an increased urinary polyphenol content which is detectable both in healthy and AOM-treated mice. Moreover, MD-MIX exposure led to a further increase in urinary polyphenol concentration in HFWD mice, but not in LFD arm (Supplementary Table 4).
These results indicate that EPA was efficiently incorporated in colonic epithelial membranes upon EPA-FFA supplementation in particular in LFD-fed mice and that urinary polyphenols excretion was increased under HFWD regimen.
Effect of MD-MIX on Apoptosis and Cell Proliferation
We then evaluated whether the MD-MIX could modulate apoptosis and cell proliferation. TUNEL staining demonstrated an induction of apoptosis in both dietary regimens treated with MD-MIX, although a stronger effect was observed in LFD-MD-MIX arm (Figures 4A,B). These data were partially confirmed by Bax/Bcl2 ratio showing an increase in LFD-MD-MIX arm (Supplementary Figure 3). No significant differences in Ki67 staining were observed among all groups (Supplementary Figure 4).
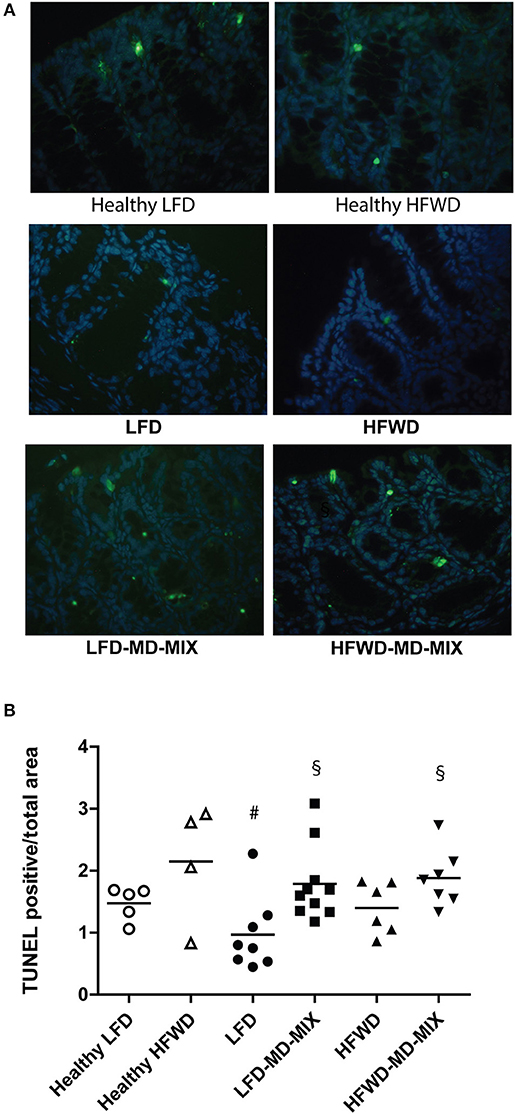
Figure 4. MD-MIX effects on cell apoptosis. (A) TUNEL representative images of colon from LFD and HFWD-fed mice (left and right column, respectively; magnification 40 X). (B) MD-MIX increased apoptosis (ANOVA p = 0.0122). Data are plotted as individual values. n = 5 Healthy LFD; n = 4 Healthy HFWD; n = 8 LFD; n = 10 LFD-MD-MIX; n = 6 HFWD; n = 7 HFWD-MD-MIX. Statistical significance was obtained using one-way two-tailed ANOVA followed by Tukey's multiple comparison post-hoc test. #p < 0.05 vs. Healthy HFWD; §p < 0.05 vs. LFD.
These results suggest that the MD-MIX may exert antineoplastic effects through a modulation of apoptosis.
Effect of AOM and MD-MIX on Gut Microbiota Structure
The pivotal role of the diet and colon carcinogenesis in modulating the gut microbiota composition has been described (31, 32). Thus, we evaluated whether AOM treatment under different dietary regimens may induce changes in specific bacterial populations. The 16S rRNA amplicons obtained from DNA samples extracted from pooled mice feces were sequenced, resulting in 226,372 high quality sequences, ranging between 2,166 and 5,802 with an average value of 4,527 sequences per sample. Reads were clustered into 7,791 operational taxonomic units (OTUs) based on 97% similarity.
AOM exposure caused a gut microbiota imbalance in both LFD and HFWD-fed mice (Figure 5). Indeed, we found an early increase in Lactobacillaceae members (in particular the genus Lactobacillus) at T2 in both LFD and HFWD arms (from 0 to 22% of the entire ecosystem in average for both diets), which is mostly maintained at T3 (14 and 22% for LFD and HFWD, respectively). Noteworthy, LFD fed mice exposed to AOM supplementation with MD-MIX caused a further increase of Lactobacillaceae (T2: 22% LFD vs. 30% LFD-MD-MIX; T3: 14% LFD vs. 18% LFD-MD-MIX), while this effect was counteracted by MD-MIX treatment in HFWD-fed mice (T2: 22% HFWD vs. 2% HFWD-MD-MIX; T3: 22% HFWD vs. 6% HFWD-MD-MIX). Members of the Firmicutes (in particular the genus Oscillospira) followed an inverse pattern respect to Lactobacillaceae. Indeed, a decrease in the genus Oscillospira was observed in LFD, LFD-MD-MIX, and HFWD arms from T1 (8.3% in average) to T3 (2.12% in average) whereas it was increased in the HFWD-MD-MIX group (from 8.3 to 12.7%). Moreover, we detected an increase in the relative abundance of Erysipelotrichaceae (in particular the genus Allobaculum) in both LFD-MD-MIX (from 4.9% at T1 to 33% at T3) and HFWD arms (from 5.7% at T1 to 21.3% at T3), while a reduction was observed in HFWD-MD-MIX group (from 8.03% at T1 at 2.65% at T3). Interestingly, the MD-MIX seems to counteract the AOM dependent microbiome unbalances only upon the HFWD regimen. PCoA, based on weighted Unifrac distances among gut microbiota profiles at OTUs level, confirmed this trend in HFWD-fed mice. In fact, PCoA showed that fecal samples at all three time-points (T1, T2, and T3) from mice supplemented with the MD-MIX plotted together in the left side independently from the AOM injection. On the contrary, samples from HFWD-fed mice taken at T2 and T3 showed a progressive drift toward the right side of the plot and far from the area in which the samples from healthy mice resided (Supplementary Figure 5).
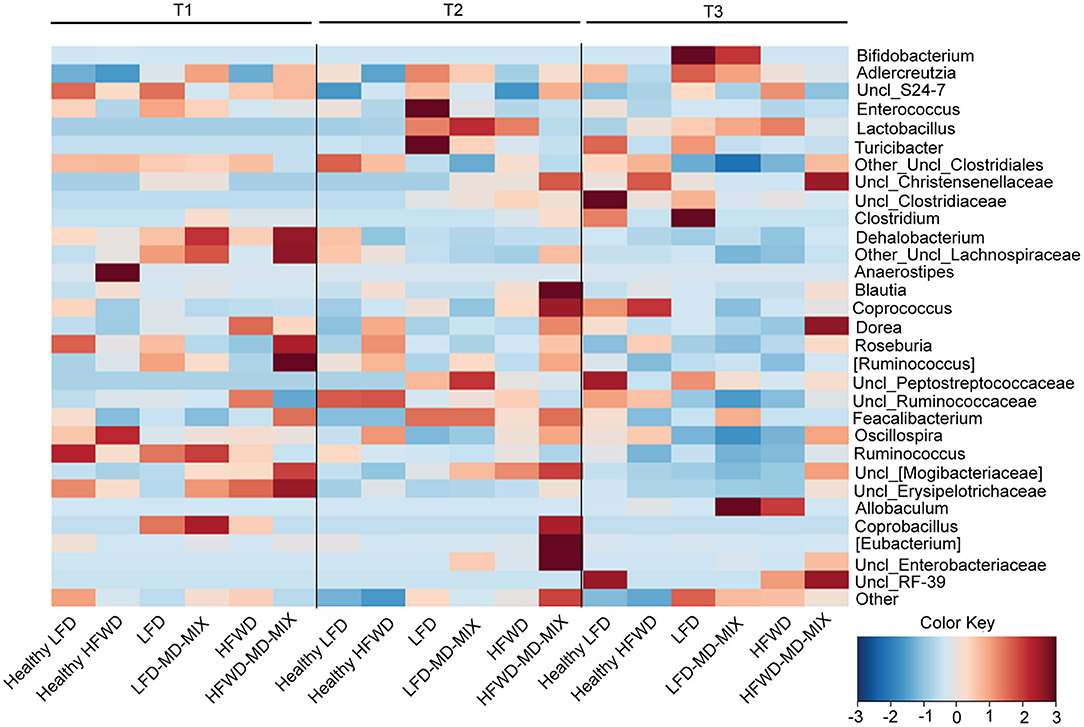
Figure 5. Impact of diets, AOM-treatment and MD-MIX on gut microbiota composition. Genus level average fecal microbiota profiles at time points T1, T2, and T3 for all combinations of diet (LFD and HFWD) and supplementation (MD-MIX), expressed as heatmap with horizontal scaling. Bacterial genera present at a relative abundance >0.05% in at least one sample are plotted. The color scale from blue to brown indicates the z score (i.e., number of standard deviations from the mean, from −3 to 3) for each time point. The heatmap has been generated using the function heatplot within the R package MADE4.
Overall, our data suggests that the impact of MD-MIX on the AOM-associated gut microbiota dysbiosis is strictly dietary dependent.
Discussion
In this study, we aimed at testing the potential chemopreventive effects of a polyphenol extract obtained from MD components, in combination with EPA-FFA (MD-MIX), in mice subjected to AOM injections and different diets.
Importantly, we found a reduction in tumor multiplicity in mice exposed to AOM and treated with the MD-MIX. In particular, our results showed that dietary supplementation with the MD-MIX may provide protection against early carcinogenic events, also in mice exposed to HFWD.
The impact of dietary habits on CRC risk has been extensively investigated. In particular, there is a great interest in identifying an effective dietary regimen that could counteract the adverse events associated with long-term unhealthy dietary habits and the widespread development of obesity, including CRC. However, many observational human studies conducted to evaluate how food habits may affect obesity and CRC risk often suffer from confounding factors thus providing inconsistent results (32). The MD is recognized as a healthy dietary pattern. The effect of MD on CRC risk has been extensively discussed in a recent review (8). Overall, a protective effect of MD pattern on CRC risk has emerged from case-control studies and meta-analyses, although data obtained from cohort studies were less consistent. These discrepancies appeared to be mainly due to different factors including: indexes used to quantify the adherence to MD, age, sex, and tumor site. Importantly MD was found to be more protective against the development of distal CRC in young adults, particularly inhabiting the MD geographic area (8).
To the best of our knowledge this is the first study aiming to approximate a MD regimen in mice and to clarify its impact on CRC onset. Despite being less obesity-prone compared to other mice strains (i.e., C57BL/6J), we opted to use the A/J strain for its increased susceptibility to AOM-induced colon carcinogenesis (29). Indeed we obtained a 100% tumor incidence in AOM-treated mice, while no tumors were observed in healthy mice fed with HFWD alone. These results are consistent with previous literature data showing that a long-term exposure to high-fat diet is necessary to obtain colonic malignant lesions in the absence of a mutagenic agent (33).
Being chemoprevention the main objective of this study, all the analyses performed to evaluate the molecular mechanisms that precede cancer development in this setting, were conducted on non-neoplastic colonic mucosa.
In order to evaluate EPA and polyphenols bioavailability, EPA incorporation in colonic tissues and urinary excretion of polyphenols were assessed. Data showed that mice treated with the MD-MIX efficiently incorporated EPA in colon tissues, independently from the diet, although LFD-MD-MIX group showed a higher EPA percentage respect to HFWD-MD-MIX arm. Thus, it is possible that HFWD, containing a higher percentage of linoleic acid compared with LFD may result in a lower EPA incorporation. Importantly, we found a higher level of apoptosis in the MD-MIX treated mice, particularly in LFD-MD-MIX. Different dietary components present in the MD extract have been associated with apoptosis induction (34). Among these, omega-3 are known to have pro-apoptotic properties in CRC (15, 22, 35). In addition, we observed a higher polyphenol bioavailability in HFWD than LFD arms exposed to the MD-MIX. Since it is known that insoluble dietary fibers can speed intestinal transit time and bind polyphenols in the intestine (36), we hypothesized that the higher content of cellulose in LFD compared with HFWD might affect polyphenols absorption.
The role of the gut microbiota in the pathogenesis of CRC is widely demonstrated (37, 38), and it is known that dietary habits may cause relevant differences in the gut microbiota structure (39). Recently, the beneficial effect of the consumption of foods typical of a MD regimen on gut microbiota composition have been highlighted in an Italian (18) and a Spanish cohort (40).
Interestingly, in our study, despite the chemopreventive effects of MD-MIX in both LFD and HFWD arms, we observed that the MD-MIX effect in counteracting the AOM-associated gut microbiota dysbioses was strictly dietary dependent. This leads us to hypothesize that the impact of MD-MIX on the gut microbiota may act with distinct mechanisms based on dietary regimens, as previously suggested (41). Indeed, although AOM exposure led to a rapid increase in the relative abundance of Lactobacillaceae family in both LFD and HFWD arms, MD-MIX was able to redress this dysbiotic drift only in mice fed HFWD. The increase in Lactobacillaceae observed in AOM-treated mice happened concomitantly to a decrease in members of the Firmicutes phylum (mainly Oscillospira and Uncl_Clostridiales). Importantly, HFWD-MD-MIX group showed Firmicutes profile similar to healthy HFWD mice with increased abundance of Oscillospira genus belonging to the Ruminococcaceae family. Recent findings in a Spanish cohort living in the Mediterranean area showed an inverse association among Oscillospira abundance with BMI and dietary intake of proteins and cholesterol indicating that changes in specific bacterial strains may reflect differences in nutrient consumption (40). Moreover, increased levels of Oscillospira have been also associated with MD consumption in non-human primates respect to Western-diet consumers (42).
An overgrowth of the Allobaculum genera, a member of Erysipelothricaceae, was found in HFWD arm at T3. A hyperproliferation of Allobaculum-like bacteria has been observed in fecal samples of rats treated with the carcinogen 1,2-dimethyl hydrazine which is a metabolic precursor of AOM (43). In addition, increased levels of Erysipelotrichaceae, in particular genera Allobaculum in mice fed a low-fat diet have been reported (41, 44), while controversial results regarding changes in members of Erysipelotrichi class of bacteria in mice undergoing a high-fat diet or western dietary pattern have been described (45, 46). As observed for Lactobacillus and Oscillospira, the HFWD-MD-MIX maintained a healthy-like abundance of Erysipelotrichi as well. Interestingly, in the context of the LFD regimen, the MD-MIX treatment strengthens the increase in Erysipelotrichi, consolidating the dysbiotic potential of AOM.
Our data indicate that the effects of MD-MIX on the AOM-driven gut microbiota dysbiosis are strictly dependent on the underlying dietary regimen. Indeed, while MD-MIX keeps the gut microbiome ecosystem in an eubiotic configuration under HFWD exposure, it consolidates an AOM dependent dysbiotic gut microbiota signature in the LFD fed groups.
In conclusion, our findings demonstrated that the supplementation with bioactives typical of MD could be effective as a chemopreventive approach in subjects who adhere to either a balanced or a western dietary regimens. Further clinical studies involving human subjects are warranted to clarify the role of MD on CRC prevention and to investigate the potential combined effect of dietary supplementation in association with a pharmacological strategy to obtain a stronger protection.
Data Availability
All datasets generated for this study are included in the manuscript and/or the supplementary files.
Ethics Statement
This study was carried out in accordance with the recommendations of University of Bologna Animal Welfare Committee. The protocol was approved by the Italian Institute of Health (203/2016-PR).
Author Contributions
GP and AP: conducting experiments, drafting of the manuscript, acquisition of data, analysis, and interpretation of the data, statistical analysis. MB and CM: technical support and acquisition of data. PV, VF, EB, MC, and PB: technical support, acquisition of data, analysis and interpretation of data. TB: analysis and interpretation of data. AM and AB: technical support, acquisition of data. MP: technical support. FB: critical revision of the manuscript for important intellectual content. LR: study concept and design, study supervision, analysis and interpretation of data, obtained funding.
Funding
Italian Association for Cancer Research [Investigator Grant IG14281 to LR] and Divo Bartolini Foundation. The study sponsor did not have a role in the study design, collection, analysis, interpretation of the data, and in the writing of the report.
Conflict of Interest Statement
The authors declare that the research was conducted in the absence of any commercial or financial relationships that could be construed as a potential conflict of interest.
Acknowledgments
We thank Dr. Chiara Fazio for the initial animal protocol assistance.
Supplementary Material
The Supplementary Material for this article can be found online at: https://www.frontiersin.org/articles/10.3389/fonc.2019.00140/full#supplementary-material
References
1. Bray F, Ferlay J, Soerjomataram I, Siegel RL, Torre LA, Jemal A. Global cancer statistics 2018: GLOBOCAN estimates of incidence and mortality worldwide for 36 cancers in 185 countries. CA Cancer J Clin. (2018) 68:394–424. doi: 10.3322/caac.21492
2. Siegel RL, Miller KD, Fedewa SA, Ahnen DJ, Meester RGS, Barzi A, et al. Colorectal cancer statistics, 2017. CA Cancer J Clin. (2017) 67:177–93. doi: 10.3322/caac.21395
3. Torres Stone RA, Waring ME, Cutrona SL, Kiefe CI, Allison J, Doubeni CA. The association of dietary quality with colorectal cancer among normal weight, overweight and obese men and women: a prospective longitudinal study in the USA. BMJ Open. (2017) 7:e015619. doi: 10.1136/bmjopen-2016-015619
4. Aleksandrova K, Pischon T, Jenab M, Bueno-de-Mesquita HB, Fedirko V, Norat T, et al. Combined impact of healthy lifestyle factors on colorectal cancer: a large European cohort study. BMC Med. (2014) 12:168. doi: 10.1186/s12916-014-0168-4
5. Orlich MJ, Singh PN, Sabaté J, Fan J, Sveen L, Bennett H, et al. Vegetarian dietary patterns and the risk of colorectal cancers. JAMA Intern Med. (2015) 175:767–76. doi: 10.1001/jamainternmed.2015.59
6. Cottet V, Bonithon-Kopp C, Kronborg O, Santos L, Andreatta R, Boutron-Ruault M-C, et al. Dietary patterns and the risk of colorectal adenoma recurrence in a European intervention trial. Eur J Cancer Prev. (2005) 14:21–9. doi: 10.1097/00008469-200502000-00004
7. Rosato V, Guercio V, Bosetti C, Negri E, Serraino D, Giacosa A, et al. Mediterranean diet and colorectal cancer risk: a pooled analysis of three Italian case–control studies. Br J Cancer. (2016) 115:862–5. doi: 10.1038/bjc.2016.245
8. Donovan MG, Selmin OI, Doetschman TC, Romagnolo DF. Mediterranean diet: prevention of colorectal cancer. Front Nutr. (2017) 4:59. doi: 10.3389/fnut.2017.00059
9. Bamia C, Lagiou P, Buckland G, Grioni S, Agnoli C, Taylor AJ, et al. Mediterranean diet and colorectal cancer risk: results from a European cohort. Eur J Epidemiol. (2013) 28:317–28. doi: 10.1007/s10654-013-9795-x
10. Pericleous M, Mandair D, Caplin ME. Diet and supplements and their impact on colorectal cancer. J Gastrointest Oncol. (2013) 4:409–23. doi: 10.3978/j.issn.2078-6891.2013.003
11. Song M, Zhang X, Meyerhardt JA, Giovannucci EL, Ogino S, Fuchs CS, et al. Marine ω-3 polyunsaturated fatty acid intake and survival after colorectal cancer diagnosis. Gut. (2017) 66:1790–6. doi: 10.1136/gutjnl-2016-311990
12. Song M, Nishihara R, Cao Y, Chun E, Qian ZR, Mima K, et al. Marine ω-3 polyunsaturated fatty acid intake and risk of colorectal cancer characterized by tumor-infiltrating T cells. JAMA Oncol. (2016) 61:135–49. doi: 10.1001/jamaoncol.2016.0605
13. Manach C, Scalbert A, Morand C, Rémésy C, Jiménez L. Polyphenols: food sources and bioavailability. Am J Clin Nutr. (2004) 79:727–47. doi: 10.1093/ajcn/79.5.727
14. Fini L, Piazzi G, Daoud Y, Selgrad M, Maegawa S, Garcia M, et al. Chemoprevention of intestinal polyps in ApcMin/+ mice fed with western or balanced diets by drinking annurca apple polyphenol extract. Cancer Prev Res. (2011) 4:907–15. doi: 10.1158/1940-6207.CAPR-10-0359
15. Fini L, Piazzi G, Ceccarelli C, Daoud Y, Belluzzi A, Munarini A, et al. Highly purified eicosapentaenoic acid as free fatty acids strongly suppresses polyps in Apc(Min/+) mice. Clin Cancer Res. (2010) 16:5703–11. doi: 10.1158/1078-0432.CCR-10-1990
16. Nakanishi M, Chen Y, Qendro V, Miyamoto S, Weinstock E, Weinstock GM, et al. Effects of walnut consumption on colon carcinogenesis and microbial community structure. Cancer Prev Res. (2016) 9:692–703. doi: 10.1158/1940-6207.CAPR-16-0026
17. Tjalsma H, Boleij A, Marchesi JR, Dutilh BE. A bacterial driver-passenger model for colorectal cancer: beyond the usual suspects. Nat Rev Microbiol. (2012) 10:575–82. doi: 10.1038/nrmicro2819
18. De Filippis F, Pellegrini N, Vannini L, Jeffery IB, La Storia A, Laghi L, et al. High-level adherence to a Mediterranean diet beneficially impacts the gut microbiota and associated metabolome. Gut. (2016) 65:1812–21. doi: 10.1136/gutjnl-2015-309957
19. Mitsou EK, Kakali A, Antonopoulou S, Mountzouris KC, Yannakoulia M, Panagiotakos DB, et al. Adherence to the Mediterranean diet is associated with the gut microbiota pattern and gastrointestinal characteristics in an adult population. Br J Nutr. (2017) 117:1645–55. doi: 10.1017/S0007114517001593
20. Song M, Garrett WS, Chan AT. Nutrients, foods, and colorectal cancer prevention. Gastroenterology. (2015) 148:1244–60.e16. doi: 10.1053/j.gastro.2014.12.035
21. Rodriguez-Palacios A, Kodani T, Kaydo L, Pietropaoli D, Corridoni D, Howell S, et al. Stereomicroscopic 3D-pattern profiling of murine and human intestinal inflammation reveals unique structural phenotypes. Nat Commun. (2015) 6:7577. doi: 10.1038/ncomms8577
22. Piazzi G, D'Argenio G, Prossomariti A, Lembo V, Mazzone G, Candela M, et al. Eicosapentaenoic acid free fatty acid prevents and suppresses colonic neoplasia in colitis-associated colorectal cancer acting on Notch signaling and gut microbiota. Int J Cancer. (2014) 135:2004–13. doi: 10.1002/ijc.28853
23. Urpi-Sarda M, Monagas M, Khan N, Llorach R, Lamuela-Raventós RM, Jáuregui O, et al. Targeted metabolic profiling of phenolics in urine and plasma after regular consumption of cocoa by liquid chromatography–tandem mass spectrometry. J Chromatogr A. (2009) 1216:7258–67. doi: 10.1016/j.chroma.2009.07.058
24. Daniel B, DeCoster MA. Quantification of sPLA2-induced early and late apoptosis changes in neuronal cell cultures using combined TUNEL and DAPI staining. Brain Res Brain Res Protoc. (2004) 13:144–50. doi: 10.1016/j.brainresprot.2004.04.001
25. Klindworth A, Pruesse E, Schweer T, Peplies J, Quast C, Horn M, et al. Evaluation of general 16S ribosomal RNA gene PCR primers for classical and next-generation sequencing-based diversity studies. Nucleic Acids Res. (2013) 41:e1. doi: 10.1093/nar/gks808
26. Masella AP, Bartram AK, Truszkowski JM, Brown DG, Neufeld JD. PANDAseq: paired-end assembler for illumina sequences. BMC Bioinformatics. (2012) 13:31. doi: 10.1186/1471-2105-13-31
27. Caporaso JG, Kuczynski J, Stombaugh J, Bittinger K, Bushman FD, Costello EK, et al. QIIME allows analysis of high-throughput community sequencing data. Nat Methods. (2010) 7:335–6. doi: 10.1038/nmeth.f.303
28. Edgar RC. Search and clustering orders of magnitude faster than BLAST. Bioinformatics. (2010) 26:2460–1. doi: 10.1093/bioinformatics/btq461
29. Bissahoyo A, Pearsall RS, Hanlon K, Amann V, Hicks D, Godfrey VL, et al. Azoxymethane is a genetic background-dependent colorectal tumor initiator and promoter in mice: effects of dose, route, and diet. Toxicol Sci. (2005) 88:340–5. doi: 10.1093/toxsci/kfi313
30. Suzuki R, Kohno H, Sugie S, Nakagama H, Tanaka T. Strain differences in the susceptibility to azoxymethane and dextran sodium sulfate-induced colon carcinogenesis in mice. Carcinogenesis. (2006) 27:162–9. doi: 10.1093/carcin/bgi205
31. Schulz MD, Atay Ç, Heringer J, Romrig FK, Schwitalla S, Aydin B, et al. High-fat-diet-mediated dysbiosis promotes intestinal carcinogenesis independently of obesity. Nature. (2014) 514:508–12. doi: 10.1038/nature13398
32. Buckland G, Bach A, Serra-Majem L. Obesity and the Mediterranean diet: a systematic review of observational and intervention studies. Obes Rev. (2008) 9:582–93. doi: 10.1111/j.1467-789X.2008.00503.x
33. Newmark HL, Yang K, Lipkin M, Kopelovich L, Liu Y, Fan K, et al. A Western-style diet induces benign and malignant neoplasms in the colon of normal C57Bl/6 mice. Carcinogenesis. (2001) 22:1871–5. doi: 10.1093/carcin/22.11.1871
34. Farinetti A, Zurlo V, Manenti A, Coppi F, Mattioli AV. Mediterranean diet and colorectal cancer: a systematic review. Nutrition. (2017) 43–4:83–8. doi: 10.1016/J.NUT.2017.06.008
35. Pot GK, Majsak-Newman G, Geelen A, Harvey LJ, Nagengast FM, Witteman BJ, et al. Fish consumption and markers of colorectal cancer risk: a multicenter randomized controlled trial. Am J Clin Nutr. (2009) 90:354–61. doi: 10.3945/ajcn.2009.27630
36. Padayachee A, Netzel G, Netzel M, Day L, Zabaras D, Mikkelsen D, et al. Binding of polyphenols to plant cell wall analogues–Part 2: phenolic acids. Food Chem. (2012) 135:2287–92. doi: 10.1016/j.foodchem.2012.07.004
37. Ahn J, Sinha R, Pei Z, Dominianni C, Wu J, Shi J, et al. Human gut microbiome and risk for colorectal cancer. J Natl Cancer Inst. (2013) 105:1907–11. doi: 10.1093/jnci/djt300
38. Flemer B, Lynch DB, Brown JMR, Jeffery IB, Ryan FJ, Claesson MJ, et al. Tumour-associated and non-tumour-associated microbiota in colorectal cancer. Gut. (2017) 66:633–43. doi: 10.1136/gutjnl-2015-309595
39. David LA, Maurice CF, Carmody RN, Gootenberg DB, Button JE, Wolfe BE, et al. Diet rapidly and reproducibly alters the human gut microbiome. Nature. (2013) 505:559–63. doi: 10.1038/nature12820
40. Garcia-Mantrana I, Selma-Royo M, Alcantara C, Collado MC. Shifts on gut microbiota associated to mediterranean diet adherence and specific dietary intakes on general adult population. Front Microbiol. (2018) 9:890. doi: 10.3389/fmicb.2018.00890
41. Cox LM, Cho I, Young SA, Anderson WHK, Waters BJ, Hung S-C, et al. The non-fermentable dietary fiber hydroxypropyl methylcellulose modulates intestinal microbiota. FASEB J. (2013) 27:692–702. doi: 10.1096/fj.12-219477
42. Nagpal R, Shively CA, Appt SA, Register TC, Michalson KT, Vitolins MZ, et al. Gut microbiome composition in non-human primates consuming a western or mediterranean diet. Front Nutr. (2018) 5:28. doi: 10.3389/fnut.2018.00028
43. Wei H, Dong L, Wang T, Zhang M, Hua W, Zhang C, et al. Structural shifts of gut microbiota as surrogate endpoints for monitoring host health changes induced by carcinogen exposure. FEMS Microbiol Ecol. (2010) 73:577–86. doi: 10.1111/j.1574-6941.2010.00924.x
44. Ravussin Y, Koren O, Spor A, LeDuc C, Gutman R, Stombaugh J, et al. Responses of Gut microbiota to diet composition and weight loss in lean and obese mice. Obesity. (2012) 20:738–47. doi: 10.1038/oby.2011.111
45. Turnbaugh PJ, Ridaura VK, Faith JJ, Rey FE, Knight R, Gordon JI. The effect of diet on the human gut microbiome: a metagenomic analysis in humanized gnotobiotic mice. Sci Transl Med. (2009) 1:6ra14. doi: 10.1126/scitranslmed.3000322
Keywords: colorectal cancer, chemoprevention, microbiota, Mediterranean diet, omega-3
Citation: Piazzi G, Prossomariti A, Baldassarre M, Montagna C, Vitaglione P, Fogliano V, Biagi E, Candela M, Brigidi P, Balbi T, Munarini A, Belluzzi A, Pariali M, Bazzoli F and Ricciardiello L (2019) A Mediterranean Diet Mix Has Chemopreventive Effects in a Murine Model of Colorectal Cancer Modulating Apoptosis and the Gut Microbiota. Front. Oncol. 9:140. doi: 10.3389/fonc.2019.00140
Received: 07 January 2019; Accepted: 18 February 2019;
Published: 12 March 2019.
Edited by:
Guangwen Cao, Second Military Medical University, ChinaReviewed by:
Hao Liu, Nanfang Hospital, Southern Medical University, ChinaZohreh Sanaat, Tabriz University of Medical Sciences, Iran
Li Liang, Southern Medical University, China
Copyright © 2019 Piazzi, Prossomariti, Baldassarre, Montagna, Vitaglione, Fogliano, Biagi, Candela, Brigidi, Balbi, Munarini, Belluzzi, Pariali, Bazzoli and Ricciardiello. This is an open-access article distributed under the terms of the Creative Commons Attribution License (CC BY). The use, distribution or reproduction in other forums is permitted, provided the original author(s) and the copyright owner(s) are credited and that the original publication in this journal is cited, in accordance with accepted academic practice. No use, distribution or reproduction is permitted which does not comply with these terms.
*Correspondence: Giulia Piazzi, Z2l1bGlhLnBpYXp6aTJAdW5pYm8uaXQ=
Luigi Ricciardiello, bHVpZ2kucmljY2lhcmRpZWxsb0B1bmliby5pdA==
†These authors have contributed equally to this work