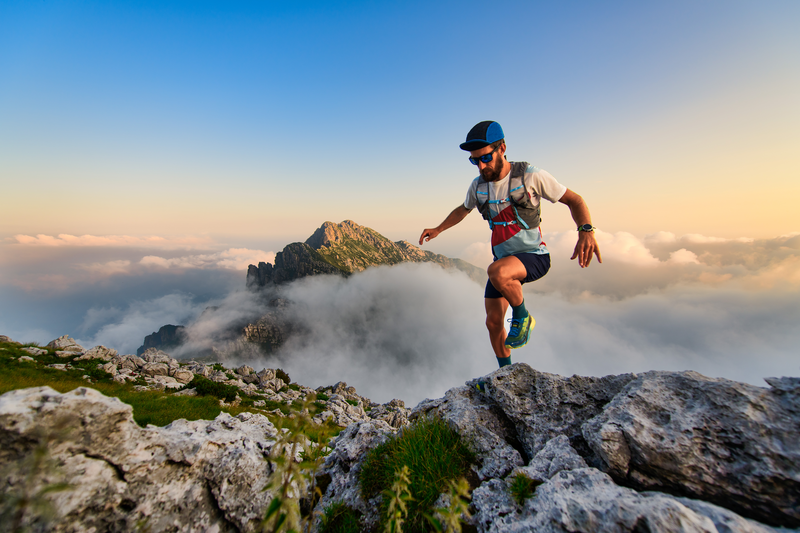
94% of researchers rate our articles as excellent or good
Learn more about the work of our research integrity team to safeguard the quality of each article we publish.
Find out more
OPINION article
Front. Oncol. , 10 January 2019
Sec. Cancer Molecular Targets and Therapeutics
Volume 8 - 2018 | https://doi.org/10.3389/fonc.2018.00667
Fifteen years ago, the question “Why do we lose the war on cancer?” sounded (1). After an interview with the world's leading oncologists, the author presented, in particular, explanations such as: “Cancer is a challenge like no other,” “A very tough set of problems,” and “Focus on individual cellular mechanisms to the near exclusion of what's happening in the organism as a whole.” One of the solutions to the problem would be “Changing the way we think about cancer.”
Until now, this radical turn has not occurred: the global cancer incidence is growing and many problems remain unsolved. As a result, the advice to change the way we think about cancer and cancer treatment has become even more relevant.
Cancer is usually viewed as a disease of old age, alongside diseases such as myocardial infarction, stroke, diabetes, Parkinson's, and Alzheimer's. However, there is a radical difference between cancer and these other diseases (2). All other diseases of old age arise due to loss of a specific function, for whatever reason, and loss of specific cells, whereas cancer is a gain of a new function and new specific cells. At the dawn of oncology, solid tumors were viewed as chaotic cell conglomerates, but currently a tumor is viewed as an organ (3–5), i.e., “an anatomically discrete collection of tissues integrated to perform specific functions” (3). The fact that, with very few exceptions (6), cancer is encountered in all animals (7, 8) suggests the great evolutionary significance of this organ. However, contrary to the general practice of biological and medical research, most cancer studies are performed without any connection to this organ's origin and function.
The function of the cancer organ is to kill the organism. Although this statement seems counter-intuitive, it is actually stating an obvious fact (2, 4, 9). As was suggested by Sommer, “cancer kills the individual and saves the species” (10), and this assignment determines its important role in evolution (8). Cancer-associated genes are essential; their mutant forms are harmful to fitness, and they are therefore subjected to strong negative selection (11). A computer simulation found that additional protections against cancer increase genetic predisposition to disease in the population (12). It was hypothesized that, in evolutionary terms, cancer serves as a guardian of the gene pool (2) or a quality control mechanism (13).
Many researchers do not share this view, and instead believe that cancer is selectively neutral, since most people whom it kills are in the postreproductive age (8). This objection seems not to be convincing. In reality, cancer always arises in an organism that is genetically compromised to some extent. Usually, driver mutations in the germinal cells and zygote induce cancer during the patient's reproductive age (the early-life cancer), whereas postzygotic mutations accumulating in somatic stem cells lead to progressive genetic mosaicism and, as a result, the postreproductive late-life cancer (14–19). Most likely, it is the early-life cancer that is subject to negative selection, thus preventing the spread of mutant alleles in the population.
However, this raises the question of why the late-life cancer incidence is so high. Perhaps the overwhelming, though selectively neutral, late-life cancer is accounted for by the phenomenon of antagonistic pleiotropy (20). It has been suggested that a beneficial early-life program can continue to function at an old age (even if useless and harmful) merely “by inertia,” i.e., due to the inefficiency of evolutionary mechanisms at a postreproductive age (4). Thus, the harmful late-life cancer may be an evolutionary tradeoff for the beneficial early-life cancer. In addition, aging-related disorders of angiogenesis, metabolism, and apoptosis create favorable conditions for cancer progression (21).
It seems likely that, in the wild, where this phenomenon has evolved over millions of years, the proportion of older individuals in the population is small (as most perish due to hunger, cold, injuries, diseases, and predators), and the selectively active early-life cancer dominates. In contrast, the current human population has overcome the calamities of the wild and achieved an unprecedented increase in life expectancy (22), and the proportion of older individuals is significant. In this situation, the selectively active inherited and familial cancers that comprise < 10% of all cancer cases (23) are overshadowed by the many-fold greater numbers of selectively neutral sporadic late-life cancers. The statistics of cancer incidence of only one, far from typical, representative of the animal world (Homo sapiens) are able to obscure the evolutionary role of cancer in general. In this manner, cancer probably performs at the population level the same function that apoptosis does at the cellular level (24, 25).
More important, however, is the practical side of this issue. In the current framework, several hallmarks of cancer are known, which are allegedly necessary and sufficient for the formation of a tumor (26, 27). Together, they explain the development of a tumor, but not that of a malignant tumor. Indeed, cancer death is, in general, due to systemic damages rather than local effects of the primary tumor growth or even the metastases (5). A well-known list of hallmarks seems to reflect a predominantly in vitro-centric view of cancer, whereas it leaves a conceptual gap between the cancer cell phenotype and the cancer disease in the in vivo-centric perception of cancer, i.e., between the cancer hallmarks and multiple paraneoplastic syndromes (28–30). It is the latter, and not the metastases themselves, that are usually fatal. Hence, we have to admit that the known hallmarks of cancer, most of which are also inherent in benign tumors (31), are necessary but insufficient to completely describe the oncological process.
There is an opinion that an individual cancer cell carries no recognizable molecules or structures that make it consistently distinguishable from a normal cell (32). However, it should be recognized that the unique and extremely important hallmark of a cancer cell is its ability to systemically ruin the body (2, 4, 9). It is strange enough that such an obvious ability (in essence, a killer function) has not yet acquired a specific name of its own. There are local and generalized manifestations of tumor growth; their relative contribution varies depending on the tumor type and location. A growing tumor can affect the body locally by squeezing the brain, squeezing the vessels, causing bleeding, or promoting obturation or perforation of a hollow organ. These local manifestations are relatively well treated with surgery or irradiation, whereas the systemic effects of a tumor are not always amenable to treatment and are the cause of death of the majority of cancer patients (cancer cell dissemination makes their elimination unfeasible and a lethal outcome often unavoidable).
The deadly effects of the “incognito” function are realized through the cancer secretome (33–36), which includes extracellular vesicles (37–42), miRNAs (43), free DNA (44), and cancer-related neurogenetic factors (45). With their help, the tumor forms its own and premetastatic niches (46–48); provides itself with a blood supply (49), energy supply (50), and innervation (51); controls the microenvironment; and recruits normal cells. In the same way, a tumor induces systemic changes, such as anemia (52), coagulopathy (53), chronic inflammation (54), metabolic abnormalities (55), anorexia, cachexia (56, 57), and NETosis (58, 59). Individual components contributing to the systemic effects are expressed to varying degrees in different forms of cancer, but on the whole, the oncologic process develops as a programmed and coordinated attack of many specialized cells (including non-transformed cells) that disturbs the organismal integrity. Collectively, these data suggest that it is the special killer function that makes the tumor malignant.
The primary goal in oncology has been, and still is, to efficiently and effectively kill cancer cells (60). If we imagine, however, using this strategy to combat external human enemies (poisonous animals, for example), it becomes clear that it is fundamentally flawed. First, the task of total extermination is hardly feasible in practice; second, the off-target damages are practically unavoidable; and, finally, it is fraught with disastrous consequences for humanity due to unacceptable ecological imbalances. Nevertheless, this is the strategy currently being used in the war against cancer, for want of a better one, and cancer patients face precisely these consequences. The low efficacy and side effects of today's cancer therapy offer grounds for criticism and require alternative solutions (61–67).
In the fight against external enemies (poisonous animals), we apply a much more effective and less devastating strategy of neutralization of poisons by specific antidotes. There are a number of interesting examples of using a similar anticancer strategy in experimental mouse models: (i) oral administration of soda solution neutralizes intratumor acidity and suppresses tumor growth (68, 69); (ii) antibodies to interleukin-23 raise the immune response (70); (iii) sympathectomy blocks prostate tumor development at an early stage, and a blockade of cholinergic receptors blocks metastasis (51) [of note, an epidemiologic study indicated a decrease in mortality in prostate cancer patients receiving beta-blockers (71)]; (iv) administration of DNase I dissolves NETs, which have a high DNA content, and restores perfusion in the kidney and heart (58, 59); and (v) the TLR7/8/9 antagonist IMO-8503 inhibits cancer-induced cachexia (72). However, no matter how promising these studies may be, they are rather fragmented.
Obviously, the possibility of developing an effective antidote is determined, first of all, by the knowledge of the mechanism of poison action. Unfortunately, we still do not have a clear understanding of the disturbances the tumor introduces into the host ecosystem, without which restoration of normal homeostasis is hardly possible. Key questions remain unanswered: What is the mechanism of malignancy? Is it the same for different types of cancer (and are paraneoplastic syndromes its side effects)? Or, on the contrary, is the mechanism specific for each form of cancer? What is the relative contribution of various components of the cancer secretome? Which metabolic pathways, and in which tissues, are their key targets?
The main maladies of contemporary mankind, aging and cancer, are both deeply rooted in the biology of multicellular organisms. This explains why fighting both is so difficult. Their study requires a systemic approach integrating the molecular, cellular, tissue, and organism levels. This opportunity has appeared relatively recently and is actively used in relation to aging. New high-throughput “omics” technologies (genomics, metagenomics, methylomics, transcriptomics, metabolomics, proteomics, etc.) are now revealing information about biological pathways that change with age in different organs and tissues (73). As for cancer, the research focuses, in general, on the tumor itself and its microenvironment. There are no attempts to apply the “omics” technologies to the tumor-bearing organism for investigation of biological pathways that change in different organs and tissues with tumor progression. Supplementing the reductionist methods traditionally used in cancer research with a holistic approach that can provide vital information for disease prevention and treatment is urgently needed.
As for the identification of putative “poisons” (i.e., mediators of the killer function), one can suggest the high utility of the mouse model of parabiosis that more than 30 years ago gave evidence of the humoral mediation of cancer-associated cachexia (74, 75). This model has a number of important advantages: (i) isolation, in short-term experiments, of only systemic effects (eliminating local effects); (ii) avoidance of the chronic effects of tumor growth fraught with various side effects; and (iii) the possibility to identify active humoral factors and their immediate targets. This model has improved in recent years to provide important information concerning carcinogenesis (76–80).
In parallel with the evolution of concepts on the nature of cancer, a rethinking of the tumor/host relationship has occurred. Early ideas about their antagonism and the life-and-death struggle have been exchanged for the realization of their synergy and paradoxical “love, not war” relationship (4). Indeed, without the multifaceted involvement of many normal cells in the oncological process, the tumor could neither arise nor metastasize. This gives grounds for considering “carcinogenesis as collective rather than individual ‘guilt,' and puts the blame on the whole cellular community rather than on a single cell” (19).
Clarifying the details of this suicidal liaison can help identify principally new drugs aimed at blocking it, devoid of the side effects of the currently used cytotoxic drugs. In this way, simple and effective solutions may be found, akin to the application of soda to normalize tissue pH (69, 70) or DNase I to dissolve NETs (58, 59).
Since the structure and function of each organ are tightly interconnected, one can suggest that the blockage of the killer function may by itself help achieve the long-awaited goal of complete elimination of tumor organ. As Sidney Farber noticed: “It is not necessary, in order to make great progress in the cure of cancer, for us to have the full solution of all the problems of basic research. The history of medicine is replete with examples of cures obtained years, decades, and even centuries before the mechanism of action was understood for these cures—from vaccination, to digitalis, to aspirin” [cited by (1)].
The author confirms being the sole contributor of this work and has approved it for publication.
The author declares that the research was conducted in the absence of any commercial or financial relationships that could be construed as a potential conflict of interest.
The author is grateful for the support by the N.N. Blokhin Cancer Research Center.
miRNAs, microRNAs; NETs, neutrophil extracellular traps; NETosis, neutrophil extracellular traps in peripheral vessels causing organ failure.
1. Leaf C. Why we're losing the war on cancer (and how to win it). Fortune (2004) 149:76–82, 84–6, 88.
2. Lichtenstein AV. On evolutionary origin of cancer. Cancer Cell Int. (2005) 5:5. doi: 10.1186/1475-2867-5-5
3. Bissell MJ, Radisky D. Putting tumours in context. Nat Rev Cancer (2001) 1:46–54. doi: 10.1038/35094059
4. Lichtenstein AV. Cancer: shift of the paradigm. Med Hypotheses (2008) 71:839–50. doi: 10.1016/j.mehy.2008.07.041
5. Egeblad M, Nakasone ES, Werb Z. Tumors as organs: complex tissues that interface with the entire organism. Dev Cell (2010) 18:884–901. doi: 10.1016/j.devcel.2010.05.012
6. Seluanov A, Gladyshev VN, Vijg J, Gorbunova V. Mechanisms of cancer resistance in long-lived mammals. Nat Rev Cancer (2018) 18:433–41. doi: 10.1038/s41568-018-0004-9
7. Greaves M. Darwinian medicine: a case for cancer. Nat Rev Cancer (2007) 7:213–21. doi: 10.1038/nrc2071
8. Leroi AM, Koufopanou V, Burt A. Opinion: cancer selection. Nat Rev Cancer (2003) 3:226–31. doi: 10.1038/nrc1016
9. Lichtenstein AV. Cancer research: a hurdle race. Biochemistry (2014) 79:385–90. doi: 10.1134/S0006297914050010
10. Sommer SS. Does cancer kill the individual and save the species? Hum Mutat. (1994) 3:166–9. doi: 10.1002/humu.1380030214
11. Thomas MA, Weston B, Joseph M, Wu W, Nekrutenko A, Tonellato PJ. Evolutionary dynamics of oncogenes and tumor suppressor genes: higher intensities of purifying selection than other genes. Mol Biol Evol. (2003) 20:964–8. doi: 10.1093/molbev/msg110
12. Frank SA. Genetic variation in cancer predisposition: mutational decay of a robust genetic control network. Proc Natl Acad Sci USA. (2004) 101:8061–5. doi: 10.1073/pnas.0400561101
14. Stratton MR, Campbell PJ, Futreal PA. The cancer genome. Nature (2009) 458:719–24. doi: 10.1038/nature07943
15. Fernandez LC, Torres M, Real FX. Somatic mosaicism: on the road to cancer. Nat Rev Cancer (2016) 16:43–55. doi: 10.1038/nrc.2015.1
16. Forsberg LA, Gisselsson D, Dumanski JP. Mosaicism in health and disease - clones picking up speed. Nat Rev Genet. (2017) 18:128–42. doi: 10.1038/nrg.2016.145
17. Tomasetti C, Li L, Vogelstein B. Stem cell divisions, somatic mutations, cancer etiology, and cancer prevention. Science (2017) 355:1330–4. doi: 10.1126/science.aaf9011
18. Lichtenstein AV. Cancer: bad luck or punishment? Biochemistry (2017) 82:75–80. doi: 10.1134/S0006297917010084
19. Lichtenstein AV. Genetic mosaicism and cancer: cause and effect. Cancer Res. (2018) 78:1375–8. doi: 10.1158/0008-5472.CAN-17-2769
20. Kirkwood TB. Understanding the odd science of aging. Cell (2005) 120:437–47. doi: 10.1016/j.cell.2005.01.027
21. Beheshti A, Benzekry S, McDonald JT, Ma L, Peluso M, Hahnfeldt P, et al. Host age is a systemic regulator of gene expression impacting cancer progression. Cancer Res. (2015) 75:1134–43. doi: 10.1158/0008-5472.CAN-14-1053
24. Umansky SR. The genetic program of cell death. Hypothesis and some applications: transformation, carcinogenesis, ageing. J Theor Biol. (1982) 97:591–602. doi: 10.1016/0022-5193(82)90360-5
25. Ameisen JC. On the origin, evolution, and nature of programmed cell death: a timeline of four billion years. Cell Death Differ. (2002) 9:367–93. doi: 10.1038/sj.cdd.4400950
26. Hanahan D, Weinberg RA. The hallmarks of cancer. Cell (2000) 100:57–70. doi: 10.1016/S0092-8674(00)81683-9
27. Hanahan D, Weinberg RA. Hallmarks of cancer: the next generation. Cell (2011) 144:646–74. doi: 10.1016/j.cell.2011.02.013
28. Finora K. Common paraneoplastic syndromes. Clin Tech Small Anim Pract. (2003) 18:123–6. doi: 10.1053/svms.2003.36629
29. Posner JB. Immunology of paraneoplastic syndromes: overview. Ann N Y Acad Sci. (2003) 998:178–86. doi: 10.1196/annals.1254.018
31. Lazebnik Y. What are the hallmarks of cancer? Nat Rev Cancer (2010) 10:232–3. doi: 10.1038/nrc2827
32. Sonnenschein C, Soto AM. The death of the cancer cell. Cancer Res. (2011) 71:4334–7. doi: 10.1158/0008-5472.CAN-11-0639
33. Balkwill F. Cancer and the chemokine network. Nat Rev Cancer (2004) 4:540–50. doi: 10.1038/nrc1388
34. Kulbe H, Levinson NR, Balkwill F, Wilson JL. The chemokine network in cancer–much more than directing cell movement. Int J Dev Biol. (2004) 48:489–96. doi: 10.1387/ijdb.041814hk
35. Kulbe H, Thompson R, Wilson JL, Robinson S, Hagemann T, Fatah R, et al. The inflammatory cytokine tumor necrosis factor-α generates an autocrine tumor-promoting network in epithelial ovarian cancer cells. Cancer Res. (2007) 67:585–92. doi: 10.1158/0008-5472.CAN-06-2941
36. Wels J, Kaplan RN, Rafii S, Lyden D. Migratory neighbors and distant invaders: tumor-associated niche cells. Genes Dev. (2008) 22:559–74. doi: 10.1101/gad.1636908
37. Rak J. Extracellular vesicles - biomarkers and effectors of the cellular interactome in cancer. Front Pharmacol. (2013) 4:21. doi: 10.3389/fphar.2013.00021
38. Coppe JP, Patil CK, Rodier F, Sun Y, Munoz DP, Goldstein J, et al. Senescence-associated secretory phenotypes reveal cell-nonautonomous functions of oncogenic RAS and the p53 tumor suppressor. PLoS Biol. (2008) 6:2853–68. doi: 10.1371/journal.pbio.0060301
39. Khwaja FW, Svoboda P, Reed M, Pohl J, Pyrzynska B, Van Meir EG. Proteomic identification of the wt-p53-regulated tumor cell secretome. Oncogene (2006) 25:7650–61. doi: 10.1038/sj.onc.1209969
40. Kim S, Takahashi H, Lin WW, Descargues P, Grivennikov S, Kim Y, et al. Carcinoma-produced factors activate myeloid cells through TLR2 to stimulate metastasis. Nature (2009) 457:102–6. doi: 10.1038/nature07623
41. Gusachenko ON, Zenkova MA, Vlassov VV. Nucleic acids in exosomes: disease markers and intercellular communication molecules. Biochemistry (2013) 78:1–7. doi: 10.1134/S000629791301001X
42. Kharaziha P, Ceder S, Li Q, Panaretakis T. Tumor cell-derived exosomes: a message in a bottle. Biochim Biophys Acta (2012) 1826:103–11. doi: 10.1016/j.bbcan.2012.03.006
43. Cooks T, Pateras IS, Jenkins LM, Patel KM, Robles AI, Morris J, et al. Mutant p53 cancers reprogram macrophages to tumor supporting macrophages via exosomal miR-1246. Nat Commun. (2018) 9:771. doi: 10.1038/s41467-018-03224-w
44. Wen F, Shen A, Choi A, Gerner EW, Shi J. Extracellular DNA in pancreatic cancer promotes cell invasion and metastasis. Cancer Res. (2013) 73:4256–66. doi: 10.1158/0008-5472.CAN-12-3287
45. Ayala GE, Dai H, Powell M, Li R, Ding Y, Wheeler TM, et al. Cancer-related axonogenesis and neurogenesis in prostate cancer. Clin Cancer Res. (2008) 14:7593–603. doi: 10.1158/1078-0432.CCR-08-1164
46. Barcellos-Hoff MH, Lyden D, Wang TC. The evolution of the cancer niche during multistage carcinogenesis. Nat Rev Cancer (2013) 13:511–8. doi: 10.1038/nrc3536
47. Kaplan RN, Riba RD, Zacharoulis S, Bramley AH, Vincent L, Costa C, et al. VEGFR1-positive haematopoietic bone marrow progenitors initiate the pre-metastatic niche. Nature (2005) 438:820–7. doi: 10.1038/nature04186
48. Kaplan RN, Rafii S, Lyden D. Preparing the “soil”: the premetastatic niche. Cancer Res. (2006) 66:11089–93. doi: 10.1158/0008-5472.CAN-06-2407
49. Bergers G, Benjamin LE. Tumorigenesis and the angiogenic switch. Nat Rev Cancer (2003) 3:401–10. doi: 10.1038/nrc1093
50. Luo Y, Yoneda J, Ohmori H, Sasaki T, Shimbo K, Eto S, et al. Cancer usurps skeletal muscle as an energy repository. Cancer Res. (2014) 74:330–40. doi: 10.1158/0008-5472.CAN-13-1052
51. Magnon C, Hall SJ, Lin J, Xue X, Gerber L, Freedland SJ, et al. Autonomic nerve development contributes to prostate cancer progression. Science (2013) 341:1236361. doi: 10.1126/science.1236361
52. Spivak JL. The anaemia of cancer: death by a thousand cuts. Nat Rev Cancer (2005) 5:543–55. doi: 10.1038/nrc1648
53. Rak J, Yu JL, Luyendyk J, Mackman N. Oncogenes, trousseau syndrome, and cancer-related changes in the coagulome of mice and humans. Cancer Res. (2006) 66:10643–6. doi: 10.1158/0008-5472.CAN-06-2350
54. Roxburgh CSD, McMillan DC. Cancer and systemic inflammation: treat the tumour and treat the host. Br J Cancer (2014) 110:1409–12. doi: 10.1038/bjc.2014.90
55. Borniger JC, Walker II WH, Emmer KM, Zhang N, Zalenski AA, et al. A role for hypocretin/orexin in metabolic and sleep abnormalities in a mouse model of non-metastatic breast cancer. Cell Metabol. (2018) 28:118–29. doi: 10.1016/j.cmet.2018.04.021
56. Danai LV, Babic A, Rosenthal MH, Dennstedt EA, Muir A, Lien EC, et al. Altered exocrine function can drive adipose wasting in early pancreatic cancer. Nature (2018) 558:600–4. doi: 10.1038/s41586-018-0235-7
57. Guttridge DC. A TGF-β pathway associated with cancer cachexia. Nat Med. (2015) 21:1248–9. doi: 10.1038/nm.3988
58. Cedervall J, Zhang Y, Huang H, Zhang L, Femel J, Dimberg A, et al. Neutrophil extracellular traps accumulate in peripheral blood vessels and compromise organ function in tumor-bearing animals. Cancer Res. (2015) 75:2653–62. doi: 10.1158/0008-5472.CAN-14-3299
59. Cedervall J, Zhang Y, Olsson AK. Tumor-induced NETosis as a risk factor for metastasis and organ failure. Cancer Res. (2016) 76:4311–5. doi: 10.1158/0008-5472.CAN-15-3051
60. Huang S. The war on cancer: lessons from the war on terror. Front Oncol. (2014) 4:293. doi: 10.3389/fonc.2014.00293
61. Gillies RJ, Verduzco D, Gatenby RA. Evolutionary dynamics of carcinogenesis and why targeted therapy does not work. Nat Rev Cancer (2012) 12:487–93. doi: 10.1038/nrc3298
62. Brock A, Huang S. Precision oncology: between vaguely right and precisely wrong. Cancer Res. (2017) 77:6473–9. doi: 10.1158/0008-5472.CAN-17-0448
63. Enriquez-Navas PM, Kam Y, Das T, Hassan S, Silva A, Foroutan P, et al. Exploiting evolutionary principles to prolong tumor control in preclinical models of breast cancer. Sci Transl Med. (2016) 8:327ra24. doi: 10.1126/scitranslmed.aad7842
64. Zhang J, Cunningham JJ, Brown JS, Gatenby RA. Integrating evolutionary dynamics into treatment of metastatic castrate-resistant prostate cancer. Nat Commun. (2017) 8:1816. doi: 10.1038/s41467-017-01968-5
65. Ibrahim-Hashim A, Abrahams D, Enriquez-Navas PM, Luddy K, Gatenby RA, Gillies RJ. Tris-base buffer: a promising new inhibitor for cancer progression and metastasis. Cancer Med. (2017) 6:1720–9. doi: 10.1002/cam4.1032
66. Maley CC, Aktipis A, Graham TA, Sottoriva A, Boddy AM, Janiszewska M, et al. Classifying the evolutionary and ecological features of neoplasms. Nat Rev Cancer (2017) 17:605–19. doi: 10.1038/nrc.2017.69
67. DeGregori J. Connecting cancer to its causes requires incorporation of effects on tissue microenvironments. Cancer Res. (2017) 77:6065–8. doi: 10.1158/0008-5472.CAN-17-1207
68. Estrella V, Chen T, Lloyd M, Wojtkowiak J, Cornnell HH, Ibrahim-Hashim A, et al. Acidity generated by the tumor microenvironment drives local invasion. Cancer Res. (2013) 73:1524–35. doi: 10.1158/0008-5472.CAN-12-2796
69. Persi E, Duran-Frigola M, Damaghi M, Roush WR, Aloy P, Cleveland JL, et al. Systems analysis of intracellular pH vulnerabilities for cancer therapy. Nat Commun. (2018) 9:2997. doi: 10.1038/s41467-018-05261-x
70. Langowski JL, Zhang X, Wu L, Mattson JD, Chen T, Smith K, et al. IL-23 promotes tumour incidence and growth. Nature (2006) 442:461–5. doi: 10.1038/nature04808
71. Grytli HH, Fagerland MW, Fossa SD, Tasken KA, Haheim LL. Use of beta-blockers is associated with prostate cancer-specific survival in prostate cancer patients on androgen deprivation therapy. Prostate (2013) 73:250–60. doi: 10.1002/pros.22564
72. Calore F, Londhe P, Fadda P, Nigita G, Casadei L, Marceca G, et al. The TLR7/8/9 antagonist IMO-8503 inhibits cancer-induced cachexia. Cancer Res. (2018) 78:6680–90. doi: 10.1158/0008-5472.CAN-17-3878
73. Valdes AM, Glass D, Spector TD. Omics technologies and the study of human ageing. Nat Rev Genet. (2013) 14:601–7. doi: 10.1038/nrg3553
74. Mordes JP, Rossini AA. Tumor-induced anorexia in the Wistar rat. Science (1981) 213:565–7. doi: 10.1126/science.6941477
75. Norton JA, Moley JF, Green MV, Carson RE, Morrison SD. Parabiotic transfer of cancer anorexia/cachexia in male rats. Cancer Res. (1985) 45:5547–52.
76. Duyverman AM, Kohno M, Duda DG, Jain RK, Fukumura D. A transient parabiosis skin transplantation model in mice. Nat Protoc. (2012) 7:763–70. doi: 10.1038/nprot.2012.032
77. Franklin RA, Li MO. Determining leukocyte origins using parabiosis in the PyMT breast tumor model. Bio Protoc. (2015) 5:e1567. doi: 10.21769/BioProtoc.1567
78. Garzia L, Kijima N, Morrissy AS, De AP, Guerreiro-Stucklin A, Holgado BL, et al. A hematogenous route for medulloblastoma leptomeningeal metastases. Cell (2018) 172:1050–62. doi: 10.1016/j.cell.2018.01.038
79. Pradeep S, Kim SW, Wu SY, Nishimura M, Chaluvally-Raghavan P, Miyake T, et al. Hematogenous metastasis of ovarian cancer: rethinking mode of spread. Cancer Cell (2014) 26:77–91. doi: 10.1016/j.ccr.2014.05.002
Keywords: war on cancer, hallmarks of cancer, killer function, tumor/host relationships, cancer therapy, treatment of cancer
Citation: Lichtenstein AV (2019) Strategies of the War on Cancer: To Kill or to Neutralize? Front. Oncol. 8:667. doi: 10.3389/fonc.2018.00667
Received: 25 September 2018; Accepted: 17 December 2018;
Published: 10 January 2019.
Edited by:
Suzie Chen, Rutgers University, The State University of New Jersey, United StatesReviewed by:
Lital Keinan-Boker, University of Haifa, IsraelCopyright © 2019 Lichtenstein. This is an open-access article distributed under the terms of the Creative Commons Attribution License (CC BY). The use, distribution or reproduction in other forums is permitted, provided the original author(s) and the copyright owner(s) are credited and that the original publication in this journal is cited, in accordance with accepted academic practice. No use, distribution or reproduction is permitted which does not comply with these terms.
*Correspondence: Anatoly V. Lichtenstein, YWxpY2h0QG1haWwucnU=
Disclaimer: All claims expressed in this article are solely those of the authors and do not necessarily represent those of their affiliated organizations, or those of the publisher, the editors and the reviewers. Any product that may be evaluated in this article or claim that may be made by its manufacturer is not guaranteed or endorsed by the publisher.
Research integrity at Frontiers
Learn more about the work of our research integrity team to safeguard the quality of each article we publish.