- 1Section of Pathological Anatomy, School of Medicine, United Hospitals, Polytechnic University of the Marche Region, Ancona, Italy
- 2Department of Radiology and Imaging Sciences, Indiana University School of Medicine, Indianapolis, IN, United States
- 3Oncology Unit, Macerata Hospital, Macerata, Italy
- 4Department of Pathology and Surgery, Faculty of Medicine, Cordoba, Spain
- 5Division of Oncology, S. Orsola-Malpighi Hospital, Bologna, Italy
- 6Institute of Urology, School of Medicine, United Hospitals, Marche Polytechnic University, Ancona, Italy
The rising incidence rate of the cancer in the prostate gland has increased the demand for improved diagnostic, imaging, and therapeutic approaches. Prostate-specific membrane antigen (PSMA), with folate hydrolase and carboxypeptidase and, internalization activities, is highly expressed in the epithelial cells of the prostate gland and is strongly upregulated in prostatic adenocarcinoma, with elevated expression correlating with, metastasis, progression, and androgen independence. Recently, PSMA has been an active target of investigation by several approaches, including the successful utilization of small molecule inhibitors, RNA aptamer conjugates, PSMA-based immunotherapy, and PSMA-targeted prodrug therapy. Future investigations of PSMA in prostate cancer (PCa) should focus in particular on its intracellular activities and functions. The objective of this contribution is to review the current role of PSMA as a marker for PCa diagnosis, imaging, and therapy.
Introduction
Prostate-specific membrane antigen (PSMA) is a type 2 integral membrane glycoprotein with folate hydrolase and carboxypeptidase, and internalization activities. This internalization capability is increased up to 3-fold when PSMA is linked to anti-PSMA antibodies. PSMA expression is highest in prostate tissue (secretory acinar epithelium), but detectable levels of PSMA protein are also found in the kidney (proximal tubules), the small bowel (i.e., jejunal brush border), neuroglia (Schwann cells and astrocytes), and salivary glands (1, 2). Notably, PSMA is highly expressed in prostate cancer cells and the vessels of various non-prostatic solid tumors (it is not expressed in the normal vasculature) (3).
With the rise and evolution of several targeted approaches to examine prostate cancer using PSMA, the aim of this contribution is to review the current role of PSMA as a marker for PCa diagnosis, imaging, and therapy.
Expression And Role Of Psma In Pca
PSMA was originally discovered using the monoclonal antibody 7E11 obtained from the cell membrane of the LNCaP cell line (4). It has been shown by immunohistochemistry that expression of PSMA at the tissue level increases through the progression from normal prostate cells to high-grade prostatic intraepithelial neoplasia (HGPIN) and to PCa (3) (Figure 1). There exists a strong positive correlation between PSMA expression and Gleason score. Elevated PSMA expression is strongly correlated with a high serum PSA. These indications are associated with increased tumor angiogenesis and lack of ets-related gene (ERG) expression which leads to reduced vitamin D and androgen receptor expression (5). PSMA expression is regulated by the androgen receptor (AR). PSMA expression increases dramatically during androgen-deprivation therapy (6).
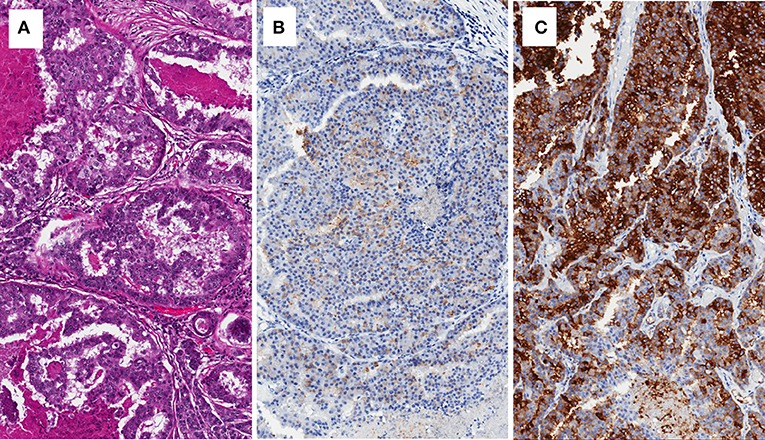
Figure 1. Brain metastasis of prostate cancer with cribriform pattern (A), showing low expression of PSA (B), and intense expression of PSMA (C).
Downregulation of PSMA expression by AR may be associated to the presence of an enhancer region although no androgen response elements have been identified (7).
PSMA expression is significantly correlated with prostate growth and differentiation (8). In particular, in vitro expression of PSMA is associated with an increased cellular folate content. This induces a proliferative property to cells expressing PSMA (9, 10). In addition, PSMA stimulates PCa cell proliferation, migration and survival through the phospho-p38 (P-p38) MAPK pathway in LNCaP cancer cells (11). Guo et al. demonstrated that PSMA knockdown in a LNCaP cell line was associated with not only the inhibition of the pathway of phosphatidylinositol 3-kinase/Akt signaling but also decreased cell proliferation, migration and survival (12).
PSMA is involved in the development of PCa metastases. Xu et al. evaluated four prostate cancer cell lines (i.e., DU145, LNCap, PC-3, and 22RV1) for metastasis-related genes potentially involved in PCa metastasis regulated by PSMA. In their study, CDH6, MMP3, and MTSS1 were seen as PSMA-related genes. Their expression was inversely related with the stage of cancer, thus suggesting their possible involvement in the suppression of PCa metastasis by PSMA (13).
PSMA-Based Imaging In Patients With PCa
Conventional imaging techniques, such as ultrasound, CT, bone scintigraphy and Magnetic Resonance Imaging (MRI), are at present utilized to detect primary PCa and its metastatic deposits. However, the limitation of such traditional imaging techniques and modalities is their low sensitivity in the detection of recurrent or/and metastatic PCa. Improved imaging modalities are needed to optimize the management of the patients with PCa.
Positron Emission Tomography (PET) and single photon emission computed tomography (SPECT) with emerging radiopharmaceuticals provide more accurate staging for primary cancer, detection of metastatic disease, and restaging of tumor recurrence. PSMA has received considerable attention as a useful marker for imaging purposes in patients with PCa (14, 15). Several PSMA-based approaches have been developed, including antibodies, nanobodies, and small molecule inhibitors.
Antibodies and Nanobodies
Indium-111 capromab pendetide (111In-capromab, ProstaScint®) was the first monoclonal antibody against PSMA used in PCa immunoscintigraphy. Correlation of scan results with tissue specimens showed that 111In-capromab detected soft tissue metastases, with an average negative predictive value of 70%, sensitivity of 60%, and positive predictive value of 60% (16–18). However, 111In-capromab lacks sensitivity because it recognizes an intracellular epitope of PSMA, thereby targeting only apoptotic/necrotic or damaged cells.
Unlike 111In-capromab, J591 is an antibody against the extracellular domain of PSMA. 111In-labeled J591 has been evaluated against conventional imaging techniques in the evaluation of bone metastases. 111In-labeled J591 identifies 93.7% of skeletal lesions detected by a conventional imaging technique. Thirteen out of Eighteen bone deposits detected only with 111In-labeled J591 were successively confirmed to be metastases (19). In a more recent study, J591 has been radiolabeled with 89Zr (20) and 64Cu (21) for PET imaging and demonstrate robust targeting of skeletal, nodal and soft tissue metastasis (22).
A new strategy in the development of high-contrast nuclear imaging is the utilization of specific antibody fragments, called nanobodies. Nanobodies contain antibody-derived smaller fragments (typically the variable domain alone of heavy chain antibodies) that largely retain the specific antigen binding properties of the original antibodies, but with more rapid pharmacokinetics and lower immunogenic potential. Evazalipour et al. compared the properties of different nanobodies radiolabeled with 99 m-Technetium (99 mTc) in PSMA+ LNCaP and PSMA− PC3 cell lines and in PSMA− and PSMA+ tumor-bearing xenografts through SPECT/micro-CT imaging and tissue analysis. Among the evaluated molecules, nanobody PSMA30 resulted in an important compound for future applications in PCa imaging trials (23).
Interesting results were also obtained with minibodies, i.e., IAB2M, an 80-kDa minibody genetically engineered from the parent antibody J591 that targets the extracellular domain of PSMA. A phase I dose-escalation study in patients with metastatic prostate cancer demonstrated PET imaging with 89Zr-Df-IAB2M is feasible and well tolerated, and targets both bone and soft-tissue disease (24).
Small Molecules
The identification of the functional (25) and structural (26) homology between N-acetylaspartylglutamate peptidase or NAAALDASE (for which a number of enzymatic inhibitors had been identified) (27, 28) and PSMA has been a major step forward for the development of PSMA-targeted radiotracers. Generally, small molecule PSMA inhibitors consist of zinc binding compounds linked to a glutamate isostere or glutamate. Phosphonate-, phosphate-, and phosphoramidates (1) and ureas (2) constitute the two main families of compounds. Based on NAALADASE homology, several compounds have been developed and labeled with 123I (20, 29, 30), 99mTc (21, 31), 18F (32), 111In (33), and 68Ga (34).
123I-MIP-1072 and 123I-MIP-1095 were the first small molecule inhibitors of PSMA adopted in the clinic. SPECT/CT using these compounds showed a rapid detection of PCa deposits in the bone, soft tissue, and prostate gland of men with metastatic PCa (35). A phase I trial on 131I-MIP-1095 in men with mCRPC is now active (NCT03030885).
Among the emerging PSMA small molecule inhibitors, N-(N-((S)-1,3-dicarboxypropyl) carbamoyl)-4-(18F)fluorobenzyl-L-cysteine (18F-DCFBC) is under evaluation in several ongoing studies. Using 18F-DCFBC, PSMA+ PC-3 PIP xenografts were early visualized with little radioactivity in the PSMA− isogenic PC-3 flu xenografts. After 2 h, the PC-3 PIP xenografts remained visible, with clearance of background radioactivity from kidneys, liver and blood (36, 37).
The use of 18F-DCFBC has been investigated in a few patients with Gleason scores between 7 and 9 and with radiological evidence of metastatic PCa. Bone scans or CT identified 21 lesions (5 bone and 16 lymph node lesions), while 32 lesions were visible with 18F-DCFBC PET. Ten of Eleven additional lesions were located in the bone and were suggestive of early bone deposits, indicating the potential of 18F-DCFBC PET in this subpopulation (38). Currently, the use of 18F-DCFBC PET/CT is under evaluation in a study enrolling patients scheduled for surgical prostate (Group 1), or with biochemical recurrence after surgery or radiotherapy (Group 2), or in metastatic PCa patients (Group 3) (NCT02190279). In addition, another ongoing phase I/II study is assessing the potential of 18F-DCFBC PET in the detection of primary PCa, nodal and bone metastases in men at initial diagnosis (NCT01496157) (Table 1).
As for the PSMA inhibitor 18F-DCFPyL (2-(3-{1-carboxy-5-((6-((18)F)fluoro-pyridine-3-carbonyl)-amino)-pentyl}-ureido)-pentanedioic acid), Chen et al. evaluated its use in immunocompromised mice utilizing isogenic PSMA PC3 PIP and PSMA- PC3 flu xenografts, suggesting that this agent could be viable and effective in this setting (32). A phase I study is now assessing the biodistribution and pharmacokinetic of 18F-DCFPyL in patients with advanced PCa (NCT02151760).
The early distinction between local disease and metastasis is crucial in the management of patients with PCa. 18F-choline can distinguish lesions with moderate to good sensitivity, but its activity is limited to patients with a PSA >1 ng/mL (39). The results obtained by 68Ga-labeled PSMA inhibitors showed a high potential in the detection of small recurrent PCa lesions in patients with low levels of serum PSA (40–42). Indeed, 68Ga-labeled PSMA inhibitors are characterized by accumulation in small metastatic deposits and a rapid clearance from the tissue in the background (43). Recently, a comparison between PET/CT and PET/MRI hybrid systems using a 68Ga-labeled PSMA compound for the detection of recurrent PCa has been performed. The results showed that Ga-PSMA PET/MRI was far more accurate in the detection of PCa and, at the same time, associated with lower radiation exposure (34).
Beyond 68Ga-labeled compounds, 99mTc-labeled inhibitors of PSMA have shown great promise in the detection of PCa lesions. Presently, a phase II study is testing 99mTc-MIP-1404 PSMA inhibitor in patients with high-risk PCa scheduled for radical prostatectomy (RP) surgery including extended pelvic lymph node (LN) dissection compared to histopathology (NCT01667536). Results are expected from the completed phase 3 trial proSPECT-AS (NCT02615067). Primary outcome measures of the study are sensitivity and specificity of 99mTc-MIP-1404 SPECT/CT image assessments to correctly detect clinically significant prostate cancer when compared to histopathology following either RP or prostate biopsy in men with newly diagnosed PCa whose biopsy indicates a histopathologic Gleason Score of ≤ 3 + 4.
Furthermore, BAY1075553 [2-PMPA analogs (2S, 4S)-2-18F-fluoro-4-(phosphonomethyl) pentanedioid acid] has demonstrated high uptake in PSMA+ LNCaP tumor xenografts (44). The phase I study showed that BAY1075553 was able to detect primary PCa, lymph node and bone metastases, although its high uptake with degenerative bone lesions may limit its use in assessing bone disease (45).
Worth mentioning is the registrational phase II/III OSPREY study (NCT02981368) that evaluated the diagnostic accuracy of 18F-DCFPyL PET/CT relative to histopathology, for detecting PCa in pelvic lymph nodes in patients with high risk localized prostate cancer who are planned for RP with lymphadenectomy, and in patients with locally recurrent or metastatic disease willing to undergo biopsy.
Imaging at Diagnosis of PCa
A number of recent studies has dealt with the use of PSMA-based imaging for the purpose of diagnosing primary PCa (Figure 2).
Fendler et al. assessed the accuracy of 68Ga-PSMA-11 PET/CT in identifying PCa at the initial diagnosis in men with biopsy-proven PCa (46). They found that the optimal SUVmax cutoff for distinction of histopathology-positive segments from histopathology-negative segments is of 6.5. With this approach they obtained 67% sensitivity, 92% specificity, 97% positive predictive value, and 72% accuracy.
Woythal et al. (47) evaluated the association of intraprostatic 68Ga-PSMA PET/CT features and PSMA immunohistochemical expression in 31 patients who underwent RP and preoperative 68Ga-PSMA-11 PET/ CT. 68Ga-PSMA-11 PET/CT demonstrated sensitivity and specificity of 87 and 97%, respectively, in the detection of PCa. However, there was no correlation between Gleason Score (GS) and the SUVmax of PCa.
On the other hand, Uprimny et al. (48) found that PCa with a GS of 6, 7a (3 + 4) and 7b (4 + 3) showed lower 68Ga-PSMA-11 uptake, with SUVmax of 5.9, 8.3, and 8.2, respectively, compared to men with a GS greater than 7 (median SUVmax: 21.2). In addition, men with a PSA of 10.0 ng/mL or above it showed a greater uptake than those patients with PSA levels below 10.0 ng/mL.
The correlation of intraprostatic PSMA uptake with clinical parameter, such as PSA value, GS and d'Amico risk score, was analyzed by Koerber et al. in 104 patients with newly diagnosed PCa (49). Results of this study indicated that men with higher PSA, higher d'Amico risk score and higher GS had greater intensity of PSMA uptake on PET/CT.
The comparison between the multiparametric Magnetic Resonance Imaging (mpMRI) and 68Ga-PSMA-11 PET/CT findings showed a concordance in the detection of intraprostatic tumor lesions, with the highest GS of 89.55%. By giving additional molecular imaging information to the mpMRI features, this method can be improved to avoid false-negative results or understaging tumors, in particular the detection of those with the highest GSs. In addition, PSMA PET/ MRI may prove useful in finding lower rates of indolent cancer detection and a great number of intermediate- and high-risk tumors.
Imaging at Staging of PCa
For pre-operatory staging, current guidelines recommend at least abdomino-pelvic cross-sectional imaging (MRI or CT) and a bone scan, for intermediate- and high-risk PCa (50) only. In a prospective study 30 patients with intermediate- and high-risk PCa underwent preoperative 68Ga-PSMA PET/CT followed by RP and extended pelvic LN dissection. Using pathology as reference, 68Ga-PSMA PET/CT showed a sensitivity of 64% for the evaluation of LN metastasis, with a 95% specificity, 88%, positive predictive value, and 82% negative predictive value (51).
In a prospective, phase II, single center study, Gorin et al. analyzed the diagnostic value of PSMA targeted 18F-DCFPyL PET/TC in the preoperative staging of 25 patients considered to be at high risk for having metastatic PCa, despite a negative conventional staging result. With this technique, they obtained a sensitivity and specificity of 71 and 88%, respectively, per patient analysis and 66 and 92% per LN packet analysis (52).
The retrospective study conducted by Maurer et al. (53) involved a 130 men with intermediate and high risk PCa staged with 68Ga-PSMA-PET/magnetic resonance tomography or PET/CT. The sensitivity, specificity and accuracy of 68Ga-PSMA-PET were 65.9, 98.9, and 88.5%, and those of morphological imaging were 43.9, 85.4, and 72.3%, respectively. Such figures are higher than those for traditional imaging techniques and other alternative PET tracers. Hence, the addition of 68Ga-PSMA PET to traditional approaches has the potential to replace current standard imaging, enabling more complete and accurate primary staging.
Imaging at Biochemical Recurrence of PCa
In men with biochemical recurrence (BCR) after RP or radiotherapy the detection rate of 68Ga-PSMA PET/CT increases with higher pre-scan PSA value. In the post-RP patients the rate of 68 Ga-PSMA PET/CT was 11.3, 26.6, 53.3, 79.1, and 95.5% for serum PSA levels of 0.01 to <0.2 ng/mL, 0.2 to <0.5 ng/mL, 0.5 to <1 ng/mL, 1 to <2 ng/mL, and ≥ 2 ng/mL, respectively. In the post-radiotherapy patients, the rate was 33.3% for PSA 0.01 to <0.5 ng/mL, 71.4% for PSA 0.5 to <1 ng/mL, 93.3% for PSA 1 to <2 ng/mL, and 100% for PSA ≥ 2 ng/mL (54). Such figures are in agreement with the meta-analysis data by Perera et al. (55). In that study, on per-patient analysis, the sensitivity and specificity of 68Ga-PSMA-11 PET were both 86%. On per-lesion analysis, the sensitivity and specificity were 80 and 97%. 68Ga-PSMA PET positivity increased with a shorter PSA doubling time.
Higher rates have been reported by Raucher et al. (56) in a cohort of men with PSA value between 0.2 and 1 ng/ml after RP. The rate of detection was 55% in men with “very low” serum PSA (0.2–0.5 ng/ml) and of 74% in patients with “low” PSA (0.5–1.0 ng <ml). In such investigation the most relevant predictors for 68GaPSMA-ligand PET/CT positivity in multivariable analysis were concurrent androgen deprivation therapy and serum PSA value. Identification of the sites of recurrent disease is of great importance, thus avoiding unnecessary localized treatments in patients of systemic recurrence and avoid the side effects of systemic treatments in men with localized recurrence (57, 58).
Table 1 summarizes the completed trials on PSMA and imaging. For additional trials please visit: https://clinicaltrials.gov.
18F-fluciclovine (Axumin®) (18F-FACBC) is an amino-acid targeting radiotracer and not a PSMA based PET/CT agent (59). The sensitivity of 18F-fluciclovine PET for identifying recurrent disease changes with PSA levels, with reported detection rates in the post-prostatectomy biochemical failure setting of 72.0% (for PSA values of less than 1 ng/mL) 83.3% (for PSA 1-2 ng/mL), and 100% for PSA levels of 2 or more ng/mL (60). In patients with pathologically enlarged lymph nodes, presence of true-positive lesions was noted in 29% patients with 18F-fluciclovine vs. 7% patients with CT (61, 62). A prospective study compared overall detection rate of 18F-FACBC and 11C-Choline PET/CT on 28 patients with biochemical relapse after RP. Anti-3-18F-FACBC PET/CT detected 60% additional tumor lesions including 5 (17.8%) additional patients (63).
PSMA-Targeting Strategies for PCa Therapy
PSMA has been widely utilized as a target antigen due to its constitutive or induced internalization property as well as to its high expression in PCa. Several strategies, including peptides, monoclonal antibodies and aptamers, have been utilized as nanoparticles or prodrugs to improve targeting efficiency in PCa cells. The discovery and development of anticancer aptamers may prove to be relevant contribution to PCa molecular imaging.
Aptamers are short DNA, RNA or peptide oligomers able to assume a specific and stable three-dimensional shape in vivo (64). Their high affinity and specificity, similar to antibodies, is achieved by a three-dimensional conformation complementary to the target surface. At this regard, Lupold et al. identified two RNA aptamers (A9 and A10) characterized with high binding affinity to PSMA, leading to the inhibition of its NAALADase/glutamate carboxypeptidase II activity (65). Successively, Xu et al. conjugated A10 aptamer on the surface of micelles, showing high drug uptake in PSMA+ cancer cells both in vitro and in vivo investigations (66).
PSMA can be used as target for delivery of therapeutic agents such as in antibody-drug conjugated (ADC) therapy. PSMA ADC is a fully human anti-PSMA monoclonal antibody conjugated to monomethyl auristatin E through a valine-citrulline linker.
Wang and his group assessed the antitumor activity of PSMA ADC in PCa cell lines in vitro and in a novel in vivo model of taxane-refractory human PCa. They observed that in vitro cytotoxic activity was efficient for PCa cells with increased PSMA expression (>105 molecules/cell; IC50 0.022 nmol/L). In addition, PSMA ADC showed high in vivo activity in treating xenograft tumors that have progressed on previous docetaxel therapy (67).
Petrylak et al. (68) reported data from a phase II trial based on PSMA-ADC at 2.5 mg/kg in patients with taxane-refractory metastatic castration-resistant PCa (CRPCa). Thirty-Nine Percent of the patients had been treated with both cabazitaxel and docetaxel, while 58% had received both enzalutamide and abiraterone. Dosing was started at 2.5 mg/kg and adjusted at 2.3 mg/kg for tolerability. The study demonstrated that PSA decline of 30% or more was observed in 36% (2.3 mg/kg) and 16% (2.5 mg/kg). Circulating tumor cell (CTC) decline of ≥50% was seen in 74% patients in both 2.3 and 2.5 mg/kg. Duration of therapy on 2.3 mg/kg was far longer than on 2.5 mg/kg, as well as the rate of serious adverse events (37 vs. 59%). Notably, PSA and CTC decline was associated with higher PSMA expression + CTC level, while PSA responses alone were correlated with lower neuroendocrine (NE) marker expression, thus suggesting that NE differentiation may have a role in this context. On the basis of such results, this study has been further extended (see NCT02020135).
Phage display technology has been used by researchers in the identification of peptide sequences, which can bind to PSMA and, at the same time, inhibit its enzymatic activity. Denmeade et al. conjugated a PSMA-specific peptide to an inhibitor (i.e., Thapsigargin) of the sarcoplasmic/endoplasmic reticulum calcium adenosine triphosphate (SERCA) pump. The type of pump shares the catalytic properties of ion-motive ATPases of the P-type family. It transports calcium ions from the cytoplasm into the sarco-endoplasmic reticulum. Its activity is needed for viability by all types of cells. The conjugate remains inactive until the PSMA-specific peptide is cleaved, thereby starting SERCA inhibition. In xenograft models, thapsigargin induced tumor regression at doses that appeared to be minimally toxic (69). Based on such findings, a phase I study is evaluating the Thapsigargin prodrug G-202 in patients with advanced PCa and other solid tumors (NCT01056029).
PSMA can be used in immunotherapy and radiotherapy approaches. “Adoptive immunotherapy based on infusion of designer T cells engineered with chimeric antigen receptors (CARs)” to potentiate their antitumor activity could serve as a highly specific modality for the treatment of cancer. Thus, PSMA×CD3 diabody is able to retarget human CD4+ and CD8+ lymphocytes to lyse PSMA-expressing C4-2 PCa cells. Other 1st and 2nd generation anti-PSMA designer T cells have shown their activity in both in vitro and in vivo studies (70). More recently, mouse-human chimeric IgG1 of 2C9 (KM2777) has been fused with C-terminus interleukin-2 (IL-2). In a xenograft tumor model using PSMA-expressing PCa cells, this fusion, KM2812, showed evident antitumor activity, with complete regression in some cases (71). Bispecific antibodies have been utilized in human clinical trials. A phase I trial has studying the safety of adoptive transfer of autologous T cells targeted to PSMA for the treatment of castrate metastatic PCa patients (NCT01140373). Vaccine is another very important area that utilizes PSMA as a target to increase cellular and humoral immune responses against tumor cells (72).
Concerning the potential role of PSMA targeted antivascular radiotherapy, Bandekar et al. has evaluated liposomes loaded with the α-particle generator 225Ac to kill in a selective manner PSMA positive PCa cells. In such study, anti-PSMA–targeted liposomes have been able to kill PSMA positive cells, including the endothelial cells expressing PSMA, thus suggesting their use for selective antivascular radiotherapy (73).
Therapy of Metastatic Castration Resistant PCa (CRPCa) With Radiopharmaceuticals
Recently, studies have explored the role of PSMA-based treatments with radiopharmaceuticals of metastatic castration resistant PCa (Figure 3). The first antibody-based radiotherapeutic was Yttrium-90 (90Y) capromab. Phase 1 (74) and Phase 2 (75) studies were unsuccessful for significant toxicity and lack of efficacy.
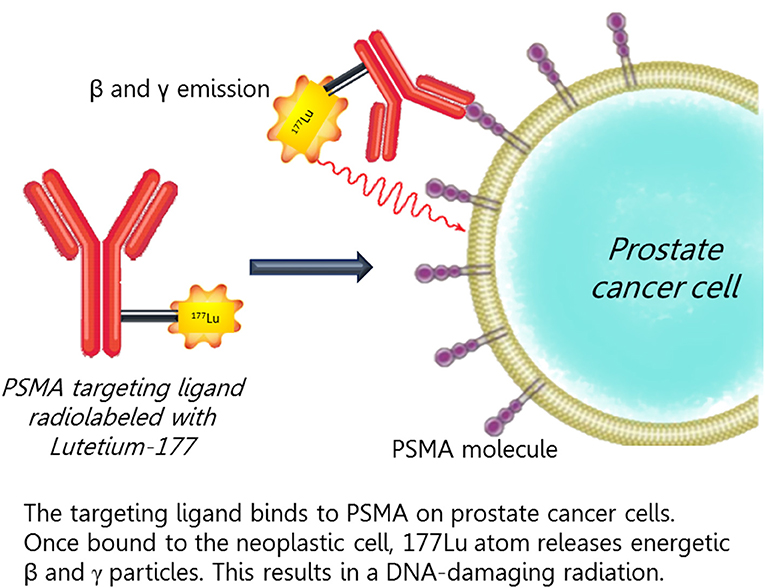
Figure 3. The targeting ligand binds to PSMA on prostate cancer cells. Once bound to the neoplastic cell, 177Lu atom releases an energetic beta and gamma particles that results in a DNA-damaging radiation.
J591 was the first humanized monoclonal antibody directed against the extracellular domain of PSMA (76). The PSMA antibody–based radiotherapeutic Lutetium-177 J591 (177Lu-J591) showed acceptable toxicity with evident targeting of known metastatic sites in a phase 1 trial. In phase II, almost 60% of men showed a decline in serum PSA levels, 10.6% of them experiencing a >50% decline in PSA (77). Myelosuppression associated with treatment was reversible. “Fractioned dosing allowed for higher cumulative doses with less toxicity” (78). However, there are limitations to the use of PSMA antibody—based radiotherapeutic. Lutetium-177 J591, i.e., slow diffusion of the antibodies into solid tumor lesions and hematotoxicity caused by a long circulation time in the blood (79). Retrospective studies have caveats such as treatments that have been done outside of clinical trials, unrecognized and unmeasured covariates that might influence final results.
A recent systematic review showed that 43% of the men showed a maximum decline of serum PSA of ≥50% following treatment with 177Lu-PSMA radioligand therapy (RLT). In particular, 177Lu-PSMA RLT gave objective remission and decline of PSA of ≥50%, more often than with third-line treatments (enzalutamide and cabazitaxel). Median survival was longer after 177Lu-PSMA RLT than after third-line treatment, the difference being not significant from statistically point of view (80).
A promising available option for patients with mCRPC is the recently investigated compound PSMA–DKFZ-617, a small molecule peptide, rather than an antibody, chemically conjugated with 177Lutetium that binds with high affinity to PSMA. Unlike antibodies such as J591, it shows more rapid plasma clearance, higher affinity binding to PSMA and lower toxicity. Interesting outcomes came up from a single-arm, single-center, phase 2 trial (ACTRN12615000912583) recently published by Hofman et al. (81, 82). Patients with mCRPC and progressive disease after standard treatments underwent screening PSMA and FDG-PET/CT to confirm high PSMA-expression. After four cycles of intravenous [Lu]-PSMA-617, 17 (57%) of 30 patients (95% CI 37–75) obtained a PSA decrease of 50% or more, objective response in nodal or visceral disease in 14 (82%) of 17 patients with measurable disease and reported minor toxic effects and improvement in pain severity. Phase 3 trial of 177Lu-PSMA-617 (NCT03511664) is currently recruiting. A multicenter randomized trial comparing LuPSMA with cabazitaxel chemotherapy (NCT03392428) is ongoing.
Table 2 summarizes a selection of active and completed trials on PSMA and therapy. For a full list of trials please visit: https://clinicaltrials.gov.
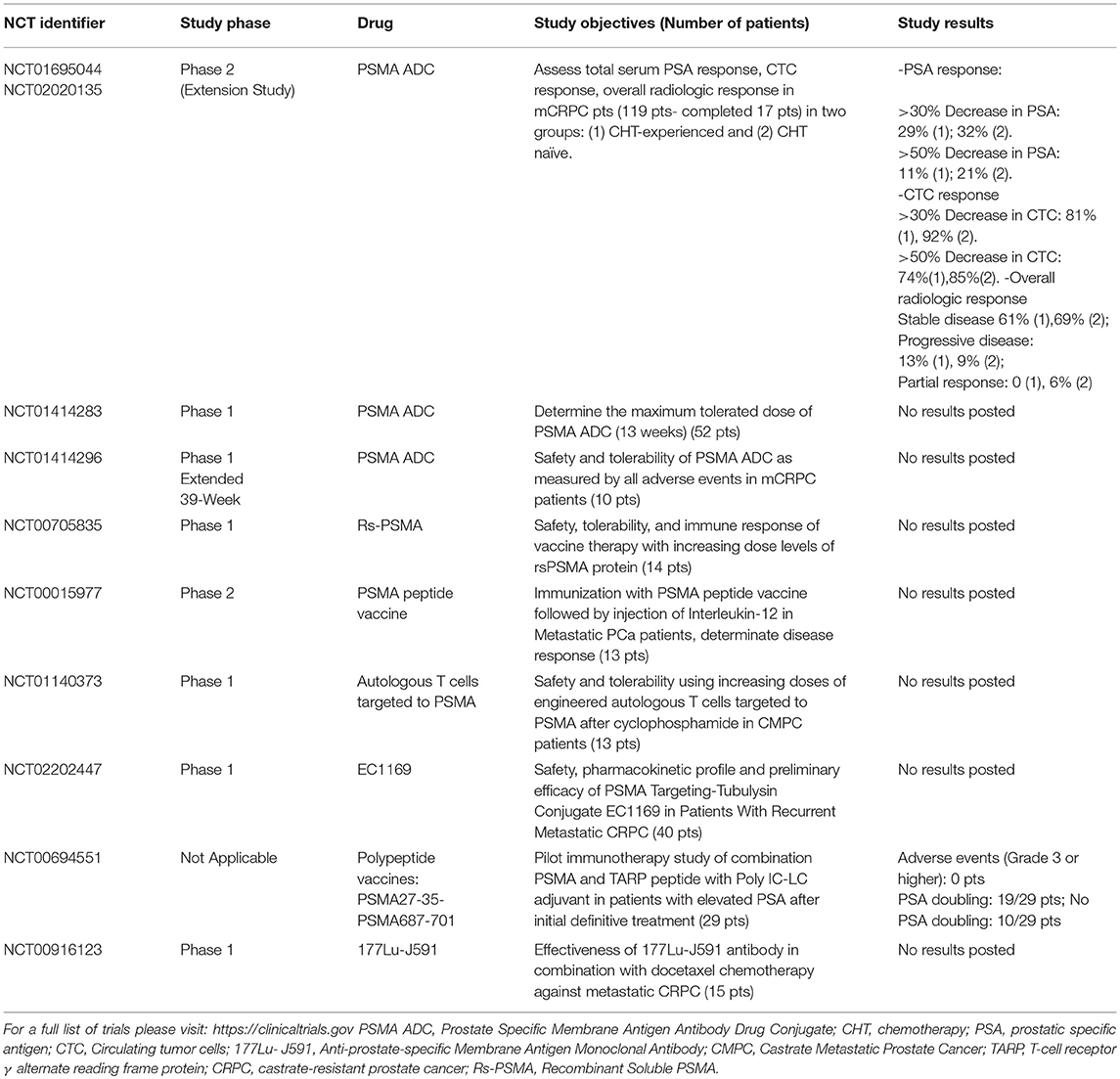
Table 2. Selection of trials of PSMA-based therapy (Selection based on active and completed trials).
Conclusion
PSMA represents an attractive target for the detection and treatment of patients with PCa. PSMA immunohistochemical evaluation should be further investigated as a predictive marker in men with metastatic PCa, to guide clinicians in the selection of the most appropriate imaging technique and therapy in individual patients. The choice of emerging PSMA-targeted tracers and therapeutic agents requires further investigation in order to identify the most specific compound for the distinct sites and phases of the disease (83–85). As our understanding of the role of PSMA in prostate carcinogenesis advances and molecular techniques become more refined, PSMA-based strategies will have a crucial role in the evolving diagnostic and therapeutic landscape of patients with PCa.
Author Contributions
RM and MSc conception and design. AC and MSa drafting the manuscript. MC, FM, and AG review of the literature. NB and AL-B critical revision of the manuscript.
Conflict of Interest Statement
The authors declare that the research was conducted in the absence of any commercial or financial relationships that could be construed as a potential conflict of interest.
References
1. Pinto JT, Suffoletto BP, Berzin TM, Qiao CH, Lin S, Tong WP, et al. Prostate-specific membrane antigen: a novel folate hydrolase in human prostatic carcinoma cells. Clin Cancer Res. (1996) 2:1445–51.
2. Carter RE, Feldman AR, Coyle JT. Prostate-specific membrane antigen is a hydrolase with substrate and pharmacologic characteristics of a neuropeptidase. Proc Natl Acad Sci USA. (1996) 93:749–53. doi: 10.1073/pnas.93.2.749
3. Ghosh A, Heston WD. Tumor target prostate specific membrane antigen (PSMA) and its regulation in prostate cancer. J Cell Biochem. (2004) 91:528–39. doi: 10.1002/jcb.10661
4. Horoszewicz JS, Kawinski E, Murphy GP. Monoclonal antibodies to a new antigenic marker in epithelial prostatic cells and serum of prostatic cancer patients. Anticancer Res. (1987) 7:927–35.
5. Kasperzyk JL, Finn SP, Flavin R, Fiorentino M, Lis R, Hendrickson WK, et al. Prostate-specific membrane antigen protein expression in tumor tissue and risk of lethal prostate cancer. Cancer Epidemiol Biomarkers Prev. (2013) 22:2354–63. doi: 10.1158/1055-9965.EPI-13-0668
6. Wright GL Jr, Grob BM, Haley C, Grossman K, Newhall K, Petrylak D, et al. Upregulation of prostate-specific membrane antigen after androgen-deprivation therapy. Urology (1996) 48:326–34.
7. Noss KR, Wolfe SA, Grimes SR. Upregulation of prostate specific membrane antigen/folate hydrolase transcription by an enhancer. Gene (2002) 285:247–56. doi: 10.1016/S0378-1119(02)00397-9
8. Chang SS, Gaudin PB, Reuter VE, Heston WD. Prostate-specific membrane antigen: present and future applications. Urology (2000) 55:622–9. doi: 10.1016/S0090-4295(99)00600-7
9. Yao V, Bacich DJ. Prostate specific membrane antigen (PSMA) expression gives prostate cancer cells a growth advantage in a physiologically relevant folate environment in vitro. Prostate (2006) 66:867–75. doi: 10.1002/pros.20361
10. Yao V, Berkman CE, Choi JK, O'Keefe DS, Bacich DJ. Expression of prostate-specific membrane antigen (PSMA), increases cell folate uptake and proliferation and suggests a novel role for PSMA in the uptake of the non-polyglutamated folate, folic acid. Prostate (2010) 70:305–16. doi: 10.1002/pros.21065
11. Zhang Y, Guo Z, Du T, Chen J, Wang W, Xu K, et al. Prostate specific membrane antigen (PSMA): a novel modulator of p38 for proliferation, migration, and survival in prostate cancer cells. Prostate (2013) 73:835–41. doi: 10.1002/pros.22627
12. Guo Z, Lai Y, Du T, Zhang Y, Chen J, Bi L, et al. Prostate specific membrane antigen knockdown impairs the tumorigenicity of LNCaP prostate cancer cells by inhibiting the phosphatidylinositol 3-kinase/Akt signaling pathway. Chin Med J. (2014) 127:929–36.
13. Xu L, Wang Z, Li XF, He X, Guan LL, Tuo JL, et al. Screening and identification of significant genes related to tumor metastasis and PSMA in prostate cancer using microarray analysis. Oncol Rep. (2013) 30:1920–8. doi: 10.3892/or.2013.2656
14. Miyahira AK, Pienta KJ, Morris MJ, Bander NH, Baum RP, Fendler WP, et al. Meeting report from the Prostate Cancer Foundation PSMA-directed radionuclide scientific working group. Prostate (2018) 78:775–89. doi: 10.1002/pros.23642
15. Fanti S, Minozzi S, Antoch G, Banks I, Briganti A, Carrio I, et al. Consensus on molecular imaging and theranostics in prostate cancer. Lancet Oncol. (2018) 19:e696–708. doi: 10.1016/S1470-2045(18)30604-1
16. Bermejo CE, Coursey J, Basler J, Austenfeld M, Thompson I. Histologic confirmation of lesions identified by Prostascint scan following definitive treatment. Urol Oncol. (2003) 21:349–52. doi: 10.1016/S1078-1439(02)00253-3
17. Lau HY, Kindrachuk G, Carter M, Prestage K, Webber D, Stauffer E, et al. Surgical confirmation of ProstaScint abnormalities in two patients with high risk prostate cancer. Can J Urol. (2001) 8:1199–202.
18. Hinkle GH, Burgers JK, Olsen JO, Williams BS, Lamatrice RA, Barth RF, et al. Prostate cancer abdominal metastases detected with indium-111 capromab pendetide. J Nucl Med. (1998) 39:650–2.
19. Pandit-Taskar N, O'Donoghue JA, Morris MJ, Wills EA, Schwartz LH, Gonen M, et al. Antibody mass escalation study in patients with castration-resistant prostate cancer using 111In-J591: lesion detectability and dosimetric projections for 90Y radioimmunotherapy. J Nucl Med. (2008) 49:1066–74. doi: 10.2967/jnumed.107.049502
20. Foss CA, Mease RC, Fan H, Wang Y, Ravert HT, Dannals RF, et al. Radiolabeled small-molecule ligands for prostate-specific membrane antigen: in vivo imaging in experimental models of prostate cancer. Clin Cancer Res. (2005) 11:4022–8. doi: 10.1158/1078-0432.CCR-04-2690
21. Lu G, Maresca KP, Hillier SM, Zimmerman CN, Eckelman WC, Joyal JL, et al. Synthesis and SAR of (99m)Tc/Re-labeled small molecule prostate specific membrane antigen inhibitors with novel polar chelates. Bioorg Med Chem Lett. (2013) 23:1557–63. doi: 10.1016/j.bmcl.2012.09.014
22. Pandit-Taskar N, O'Donoghue JA, Beylergil V, Lyashchenko S, Ruan S, Solomon SB, et al. (89)Zr-huJ591 immuno-PET imaging in patients with advanced metastatic prostate cancer. Eur J Nucl Med Mol Imaging (2014) 4:2093–105. doi: 10.1007/s00259-014-2830-7
23. Evazalipour M, D'Huyvetter M, Tehrani BS, Abolhassani M, Omidfar K, Abdoli S, et al. Generation and characterization of nanobodies targeting PSMA for molecular imaging of prostate cancer. Contrast Media Mol Imaging (2014) 9:211–20. doi: 10.1002/cmmi.1558
24. Pandit-Taskar N, O'Donoghue JA, Ruan S, Lyashchenko SK, Carrasquillo JA, Heller G, et al. First-in-human imaging with 89Zr-Df-IAB2M anti-PSMA minibody in patients with metastatic prostate cancer: pharmacokinetics, biodistribution, dosimetry, and lesion uptake. J Nucl Med. (2016) 57:1858–64. doi: 10.2967/jnumed.116.176206
25. Tiffany CW, Lapidus RG, Merion A, Calvin DC, Slusher BS. Characterization of the enzymatic activity of PSM: comparison with brain NAALADase. Prostate (1999) 39:28–35. doi: 10.1002/(SICI)1097-0045(19990401)39:1<28::AID-PROS5>3.0.CO;2-A
26. Luthi-Carter R, Barczak AK, Speno H, Coyle JT. Molecular characterization of human brain N-acetylated alpha-linked acidic dipeptidase (NAALADase). J Pharmacol Exp Ther. (1998) 286:1020–5.
27. Jackson PF, Cole DC, Slusher BS, Stetz SL, Ross LE, Donzanti BA, et al. Design, synthesis, and biological activity of a potent inhibitor of the neuropeptidase N-acetylated alpha-linked acidic dipeptidase. J Med Chem. (1996) 39:619–22. doi: 10.1021/jm950801q
28. Jackson PF, Slusher BS. Design of NAALADase inhibitors: a novel neuroprotective strategy. Curr Med Chem. (2001) 8:949–57. doi: 10.2174/0929867013372797
29. Maresca KP, Hillier SM, Femia FJ, Keith D, Barone C, Joyal JL, et al. A series of halogenated heterodimeric inhibitors of prostate specific membrane antigen (PSMA) as radiolabeled probes for targeting prostate cancer. J Med Chem. (2009) 52:347–57. doi: 10.1021/jm800994j
30. Hillier SM, Maresca KP, Femia FJ, Marquis JC, Foss CA, Nguyen N, et al. Preclinical evaluation of novel glutamate-urea-lysine analogues that target prostate-specific membrane antigen as molecular imaging pharmaceuticals for prostate cancer. Cancer Res. (2009) 69:6932–40. doi: 10.1158/0008-5472.CAN-09-1682
31. Kularatne SA, Zhou Z, Yang J, Post CB, Low PS. Design, synthesis, and preclinical evaluation of prostate-specific membrane antigen targeted (99m)Tc-radioimaging agents. Mol Pharm. (2009) 6:790–800. doi: 10.1021/mp9000712
32. Chen Y, Pullambhatla M, Byun Y, Foss CA, Nimmagadda S, Senthamizhchelvan S, et al. 2-(3-{1-Carboxy-5-((6-(18F)fluoropyridine- 3-carbonyl)-amino)-pentyl}-ureido)-pentanedioic acid, (18F)DCFPyL, a PSMA-based PET imaging agent for prostate cancer. Clin Cancer Res. (2011) 17:7645–53. doi: 10.1158/1078-0432.CCR-11-1357
33. Banerjee SR, Pullambhatla M, Shallal H, Lisok A, Mease RC, Pomper MG. A modular strategy to prepare multivalent inhibitors of prostate-specific membrane antigen (PSMA). Oncotarget (2011) 2:1244–53. doi: 10.18632/oncotarget.415
34. Afshar-Oromieh A, Haberkorn U, Schlemmer HP, Fenchel M, Eder M, Eisenhut M, et al. Comparison of PET/CT and PET/MRI hybrid systems using a 68Ga-labelled PSMA ligand for the diagnosis of recurrent prostate cancer: initial experience. Eur J Nucl Med Mol Imaging (2014) 4:887–97. doi: 10.1007/s00259-013-2660-z
35. Barrett JA, Coleman RE, Goldsmith SJ, Vallabhajosula S, Petry NA, Cho S, et al. First-in-man evaluation of two high-affinity PSMA-avid small molecules for imaging prostate cancer. J Nucl Med. (2013) 54:380–7. doi: 10.2967/jnumed.112.111203
36. Mease RC, Foss CA, Pomper MG. PET imaging in prostate cancer: focus on prostate-specific membrane antigen. Curr Top Med Chem. (2013) 13:951–62. doi: 10.2174/1568026611313080008
37. Mease RC, Dusich CL, Foss CA, Ravert HT, Dannals RF, Seidel J, et al. N-(N-(S)-1,3-Dicarboxypropyl)carbamoyl)-4- (18F)fluorobenzyl-L-cysteine, (18F)DCFBC: a new imaging probe for prostate cancer. Clin Cancer Res. (2008) 14:3036–43. doi: 10.1158/1078-0432.CCR-07-1517
38. Cho SY, Gage KL, Mease RC, Senthamizhchelvan S, Holt DP, Kwanisai-Jeffrey A, et al. Biodistribution, tumor detection and Radiation dosimetry of 18F-DCFBC, a low molecular weight inhibitor of PSMA, in patients with metastatic prostate cancer. J Nucl Med. (2012) 53:1883–91. doi: 10.2967/jnumed.112.104661
39. Giovacchini G, Picchio M, Coradeschi E, Bettinardi V, Gianolli L, Scattoni V, et al. Predictive factors of ((11)C)choline PET/CT in patients with biochemical failure after radical prostatectomy. Eur J Nucl Med Mol Imaging (2010) 37:301–9. doi: 10.1007/s00259-009-1253-3
40. Afshar-Oromieh A, Haberkorn U, Eder M, Eisenhut M, Zechmann C. ((68)Ga)Gallium-labelled PSMA ligand as superior PET tracer for the diagnosis of prostate cancer: comparison with (18)F-FECH. Eur J Nucl Med Mol Imaging (2012) 39:1085–6. doi: 10.1007/s00259-012-2069-0
41. Afshar-Oromieh A, Malcher A, Eder M, Eisenhut M, Linhart HG, Hadaschik BA, et al. PET imaging with a ((68)Ga)gallium-labelled PSMA ligand for the diagnosis of prostate cancer: biodistribution in humans and first evaluation of tumour lesions. Eur J Nucl Med Mol Imaging (2013) 40:486–95. doi: 10.1007/s00259-012-2298-2
42. Eder M, Schafer M, Bauder-Wust U, Hull WE, Wangler C, Mier W, et al. (68)Ga-Complex lipophilicity and the targeting property of a urea-based PSMA inhibitor for PET imaging. Bioconjug Chem. (2012) 23:688–97. doi: 10.1021/bc200279b
43. Eder M, Eisenhut M, Babich J, Haberkorn U. PSMA as a target for radiolabelled small molecules. Eur J Nucl Med Mol Imaging (2013) 40:819–23. doi: 10.1007/s00259-013-2374-2
44. Graham K, Kettschau G, Gromov A, Friebe M, Gekeler V, Dinkelborg L. (18F)-Labeled PET Tracer BAY1075553, Small Molecule Inhibitor of PSMA for Molecular Imaging of Prostate Cancer. World Molecular Imaging Conference; September 7–10; San Diego, CA (2011) p. P235
45. Langsteger W, Kunit T, Haim S, Nader M, Valencia R, Lesche R, et al. BAY 1075553 PET/CT in the assessment of prostate cancer: safety, tolerability and biodistribution-Phase I first in human study results. J Nucl Med. (2012) 53:1125.
46. Fendler WP, Schmidt DF, Wenter V, Thierfelder KM, Zach C, Stief C, et al. 68GaPSMA PET/CT detects the location and extent of primary prostate cancer. J Nucl Med. (2016) 57:1720–5. doi: 10.2967/jnumed.116.172627
47. Woythal N, Arsenic R, Kempkensteffen C, Miller K, Janssen JC, Huang K, et al. Immunohistochemical validation of PSMA expression measured by 68Ga-PSMA PET/CT in primary prostate cancer. J Nucl Med. (2018) 59:238–43. doi: 10.2967/jnumed.117.195172
48. Uprimny C, Kroiss AS, Decristoforo C, Fritz J, von Guggenberg E, Kendler D, et al. 68Ga-PSMA-11 PET/CT in primary staging of prostate cancer: PSA and Gleason score predict the intensity of tracer accumulation in the primary tumour. Eur J Nucl Med Mol Imaging (2017) 44:941–9. doi: 10.1007/s00259-017-3631-6
49. Koerber SA, Utzinger MT, Kratochwil C, Kesch C, Haefner MF, Katayama S, et al. 68Ga-PSMA-11 PET/CT in newly diagnosed carcinoma of the prostate: correlation of intraprostatic PSMA uptake with several clinical parameters. J Nucl Med. (2017) 58:1943–8. doi: 10.2967/jnumed.117.190314
50. Mottet N, Bellmunt J, Bolla M, Briers E, Cumberbatch MG, De Santis M, et al. EAU-ESTROSIOG guidelines on prostate cancer. Part 1: screening, diagnosis, and local treatment with curative intent. Eur Urol. (2017) 71:618–29. doi: 10.1016/j.eururo.2016.08.003
51. van Leeuwen PJ, Emmett L, Ho B, Delprado W, Ting F, Nguyen Q, et al. Prospective evaluation of 68Gallium-prostate-specific membrane antigen positron emission tomography/computed tomography for preoperative lymph node staging in prostate cancer. BJU Int. (2017) 119:209–15. doi: 10.1111/bju.13540
52. Gorin MA, Rowe SP, Patel HD, Vidal I, Mana-Ay M, Javadi MS, et al. Prostate specific membrane antigen targeted 18F-DCFPyL positron emission tomography/computerized tomography for the preoperative staging of high risk prostate cancer: results of a prospective, phase ii, single center study. J Urol. (2018) 199:126–32. doi: 10.1016/j.juro.2017.07.070
53. Maurer T, Gschwend JE, Rauscher I, Souvatzoglou M, Haller B, Weirich G, et al. Diagnostic efficacy of (68)gallium-PSMA positron emission tomography compared to conventional imaging for lymph node staging of 130 consecutive patients with intermediate to high risk prostate cancer. J Urol. (2016) 195:1436–43. doi: 10.1016/j.juro.2015.12.025
54. Meredith G, Wong D, Yaxley J, Coughlin G, Thompson L, Kua B, Gianduzzo T. The use of 68 Ga-PSMA PET CT in men with biochemical recurrence after definitive treatment of acinar prostate cancer. BJU Int. (2016) 118(Suppl 3):49–55. doi: 10.1111/bju.13616
55. Perera M, Papa N, Christidis D, Wetherell D, Hofman MS, Murphy DG, et al. Sensitivity, specificity, and predictors of positive 68Ga-prostate-specific membrane antigen positron emission tomography in advanced prostate cancer: a systematic review and meta-analysis. Eur Urol. (2016) 70:926–37. doi: 10.1016/j.eururo.2016.06.021
56. Rauscher I, Düwel C, Haller B, Rischpler C, Heck MM, Gschwend JE, et al. Efficacy, predictive factors, and prediction nomograms for Ga-labeled prostate-specific membrane antigen–ligand positron-emission tomography/computed tomography in early biochemical recurrent prostate cancer after radical prostatectomy. Eur Urol. (2018) 73:656–61. doi: 10.1016/j.eururo.2018.01.006
57. Rauscher I, Maurer T, Beer AJ, Graner FP, Haller B, Weirich G, et al. Value of 68GaPSMA HBED-CC PET for the assessment of lymph node metastases in prostate cancer patients with biochemical recurrence: comparison with histopathology after salvage lymphadenectomy. J Nucl Med. (2016) 57:1713–9. doi: 10.2967/jnumed.116.173492
58. Cimadamore A, Scarpelli M, Cheng L, Lopez-Beltran A, Montorsi F, Montironi R. Re: Isabel Rauscher, Charlotte Düwel, Bernhard Haller, et al. Efficacy, predictive factors, and prediction nomograms for 68Ga-labeled prostate-specific membrane antigen-ligand positron-emission tomography/computed tomography in early biochemical recurrent prostate cancer after radical prostatectomy. Eur Urol 2018;73:656-61: Clinical Significance of Prostate-specific Membrane Antigen Immunohistochemistry and Role of the Uropathologists. Eur Urol. (2018) 74:e141–4. doi: 10.1016/j.eururo.2018.07.034
59. Parent EE, Schuster DM. Update on 18F-Fluciclovine PET for prostate cancer imaging. J Nucl Med. (2018) 59:733–9. doi: 10.2967/jnumed.117.204032
60. Akin-Akintayo OO, Jani AB, Odewole O, Tade FI, Nieh PT, Master VA, et al. Change in salvage radiotherapy management based on guidance with FACBC (fluciclovine) PET-CT in postprostatectomy recurrent prostate cancer. Clin Nucl Med. (2017) 42:e22–8. doi: 10.1097/RLU.0000000000001379
61. Odewole OA, Tade FI, Nieh PT, Savir-Baruch B, Jani AB, Master VA, et al. Recurrent prostate cancer detection with anti-3-[18F]FACBC PET-CT: comparison with CT. Eur J Nucl Med Mol Imaging (2016) 43:1773–83. doi: 10.1007/s00259-016-3383-8
62. Han S, Woo S, Kim YJ, Suh CH. Impact of 68Ga-PSMA PET on the management of patients with prostate cancer: a systematic review and meta-analysis. Eur Urol. (2018) 74:179–90. doi: 10.1016/j.eururo.2018.03.030
63. Nanni C, Schiavina R, Brunocilla E, Borghesi M, Ambrosini V, Zanoni L, et al. 18F-FACBC compared with 11C-choline PET/CT in patients with biochemical relapse after radical prostatectomy: a prospective study in 28 patients. Clin Genitourin Cancer (2014) 12:106–10. doi: 10.1016/j.clgc.2013.08.002
64. Ireson CR, Kelland LR. Discovery and development of anticancer aptamers. Mol Cancer Ther. (2006) 5:2957–62. doi: 10.1158/1535-7163.MCT-06-0172
65. Lupold SE, Hicke BJ, Lin Y, Coffey DS. Identification and characterization of nuclease-stabilized RNA molecules that bind human prostate cancer cells via the prostate-specific membrane antigen. (2002) Cancer Res. 62:4029–33.
66. Xu W, Siddiqui IA, Nihal M, Pilla S, Rosenthal K, Mukhtar H, et al. Aptamer-conjugated and doxorubicin-loaded unimolecular micelles for targeted therapy of prostate cancer. Biomaterials (2013) 34:5244–53. doi: 10.1016/j.biomaterials.2013.03.006
67. Wang X, Ma D, Olson WC, Heston WD. In vitro and in vivo responses of advanced prostate tumors to PSMA ADC, an auristatin-conjugated antibody to prostate-specific membrane antigen. Mol Cancer Ther. (2011) 10:1728–39. doi: 10.1158/1535-7163.MCT-11-0191
68. Petrylak DP, Smith DC, Appleman LJ, Fleming MT, Hussain A, Dreicer R, et al. A Phase 2 Trial of Prostate-Specific Membrane Antigen Antibody Drug Conjugate (PSMA ADC) in Taxane-Refractory Metastatic Castration-Resistant Prostate Cancer (mCRPC). 2014 ASCO Annual Meeting; abstract 5023.
69. Denmeade SR, Mhaka AM, Rosen DM, Brennen WN, Dalrymple S, Dach I, et al. Engineering a prostate-specific membrane antigen-activated tumor endothelial cell prodrug for cancer therapy. Sci Transl Med. (2012) 4:140ra86. doi: 10.1126/scitranslmed.3003886
70. Ma Q, Safar M, Holmes E, Wang Y, Boynton AL, Junghans RP. Anti-prostate specific membrane antigen designer T cells for prostate cancer therapy. Prostate (2004) 61:12–25. doi: 10.1002/pros.20073
71. Sugimoto Y, Hirota M, Yoshikawa K, Sumitomo M, Nakamura K, Ueda R, et al. The therapeutic potential of a novel PSMA antibody and its IL-2 conjugate in prostate cancer. Anticancer Res. (2014) 34:89–97.
72. Yeku O, Slovin SF. Immune therapy for prostate cancer. Cancer J. (2016) 22:334–41. doi: 10.1097/PPO.0000000000000223
73. Bandekar A, Zhu C, Jindal R, Bruchertseifer F, Morgenstern A, Sofou S. Anti-prostate-specific membrane antigen liposomes loaded with 225Ac for potential targeted antivascular α-particle therapy of cancer. J Nucl Med. (2014) 55:107–14. doi: 10.2967/jnumed.113.125476
74. Deb N, Goris M, Trisler K, Fowler S, Saal J, Ning S, et al. Treatment of hormone-refractory prostate cancer with 90Y-CYT-356monoclonal antibody. Clin Cancer Res. (1996) 2:1289–97.
75. Kahn D, Austin JC, Maguire RT, Miller SJ, Gerstbrein J, Williams RD. A phase II study of [90Y]yttrium-capromabpendetideinthe treatment of men with prostate cancer recurrence following radical prostatectomy. Cancer Biother Radiopharm. (1999) 14:99–111. doi: 10.1089/cbr.1999.14.99
76. Bander NH, Trabulsi EJ, Kostakoglu L, Yao D, Vallabhajosula S, Smith-Jones P, et al. Targeting metastatic prostate cancer with radiolabeled monoclonal antibody J591 to the extracellular domain of prostate specific membrane antigen. J Urol. (2003) 170:1717–21. doi: 10.1097/01.ju.0000091655.77601.0c
77. Tagawa ST, Milowski MI, Morris M. PhaseII trial of 177Lutetium radiolabeled anti-prostate specific membrane antigen (PSMA) mono-clonal antibody J591(177Lu-J591) in patients (pts) with metastatic castrate resistant prostate cancer (metCRPC). J Clin Oncol. (2008) 28 (Suppl. 15):5140. doi: 10.1200/jco.2008.26.15_suppl.5140
78. Tagawa ST, Vallabhajosula S, Osborne J, Goldsmith K, Petrillo L, Tyrell GS, et al. Phase I trial of fractionated-dose 177lutetium radio labeled anti-prostate specific membrane antigen (PSMA)monocloncal antibody J591(177Lu- J591) in patients with metastatic castration-resistant prostate cancer. J Clin Oncol. (2010) 28:4667. doi: 10.1200/jco.2010.28.15_suppl.4667
79. Tagawa ST, Milowsky MI, Morris M, Vallabhajosula S, Christos P, Akhtar NH, et al. Phase II study of Lutetium-177-labeled anti-prostate-specific membrane antigen monoclonal antibody J591 for metastatic castration-resistant prostate cancer. Clin Cancer Res. (2013) 19:5182–91. doi: 10.1158/1078-0432.CCR-13-0231
80. von Eyben FE, Roviello G, Kiljunen T, Uprimny C, Virgolini I, Kairemo K, Joensuu T. Third-line treatment and 177Lu-PSMA radioligand therapy of metastatic castration-resistant prostate cancer: a systematic review. Eur J Nucl Med Mol Imaging (2018) 45:496–508. doi: 10.1007/s00259-017-3895-x
81. Hofman MS, Violet J, Hicks RJ, Ferdinandus J, Thang SP, Akhurst T, et al. [177Lu]-PSMA-617 radionuclide treatment in patients with metastatic castration-resistant prostate cancer (LuPSMA trial): a single-centre, single-arm, phase 2 study. Lancet Oncol. (2018) 19:825–33. doi: 10.1016/S1470-2045(18)30198-0
82. Bradley CA. [177Lu]PSMA-617 radionuclide therapy shows promise. Nat Rev Urol. (2018) 15:468. doi: 10.1038/s41585-018-0029-6
83. Cimadamore A, Gasparrini S, Scarpelli M, Doria A, Mazzucchelli R, Massari F, et al. Epigenetic modifications and modulators in prostate cancer. Crit Rev Oncog. (2017) 22:439–50. doi: 10.1615/CritRevOncog.2017020964
84. Gasparrini S, Cimadamore A, Mazzucchelli R, Scarpelli M, Massari F, Raspollini MR, et al. Pathology and molecular updates in tumors of the prostate: towards a personalized approach. Expert Rev Mol Diagn. (2017) 17:781–9. doi: 10.1080/14737159.2017.1341314
Keywords: prostate cancer, prostate-specific membrane antigen, PSMA, small molecule inhibitors, RNA aptamer conjugates, PSMA-based immunotherapy, PSMA-targeted prodrug therapy, positron emission tomography
Citation: Cimadamore A, Cheng M, Santoni M, Lopez-Beltran A, Battelli N, Massari F, Galosi AB, Scarpelli M and Montironi R (2018) New Prostate Cancer Targets for Diagnosis, Imaging, and Therapy: Focus on Prostate-Specific Membrane Antigen. Front. Oncol. 8:653. doi: 10.3389/fonc.2018.00653
Received: 02 July 2018; Accepted: 10 December 2018;
Published: 21 December 2018.
Edited by:
Scott T. Tagawa, Cornell University, United StatesReviewed by:
Jaspreet Singh Batra, Johns Hopkins Medicine, United StatesDaniele Baiz, Plymouth University, United Kingdom
Copyright © 2018 Cimadamore, Cheng, Santoni, Lopez-Beltran, Battelli, Massari, Galosi, Scarpelli and Montironi. This is an open-access article distributed under the terms of the Creative Commons Attribution License (CC BY). The use, distribution or reproduction in other forums is permitted, provided the original author(s) and the copyright owner(s) are credited and that the original publication in this journal is cited, in accordance with accepted academic practice. No use, distribution or reproduction is permitted which does not comply with these terms.
*Correspondence: Alessia Cimadamore, YWxlc3NpYWNpbWFkYW1vcmVAZ21haWwuY29t
Rodolfo Montironi, ci5tb250aXJvbmlAdW5pdnBtLml0