- Department of Radiation Oncology, University Hospital Zurich, Zurich, Switzerland
Purpose: Stereotactic radiosurgery (SRS) is the preferred primary treatment option for patients with a limited number of asymptomatic brain metastases. In case of relapse after initial SRS the optimal salvage treatment is not well defined. Within this retrospective analysis, we investigated the feasibility of repeated courses of SRS to defer Whole-Brain Radiation Therapy (WBRT) and aimed to derive prognostic factors for patient selection.
Materials and Methods: From 2014 until 2017, 42 patients with 197 brain metastases have been treated with multiple courses of SRS at our institution. Treatment was delivered as single fraction (18 or 20 Gy) or hypo-fractionated (6 fractions with 5 Gy) radiosurgery. Regular follow-up included clinical examination and contrast-enhanced cMRI at 3–4 months' intervals. Besides clinical and treatment related factors, brain metastasis velocity (BMV) as a newly described clinical prognostic metric was included and calculated between first and second treatment.
Results: A median number of 1 lesion (range: 1–13) per course and a median of 2 courses (range: 2–6) per patient were administered resulting in a median of 4 (range: 2–14) metastases treated over time per patient. The median interval between SRS courses was 5.8 months (range: 0.9–35 months). With a median follow-up of 17.4 months (range: 4.6–45.5 months) after the first course of treatment, a local control rate of 84% was observed after 1 year and 67% after 2 years. Median time to out-of-field-brain-failure (OOFBF) was 7 months (95%CI 4–8 months). WBRT as a salvage treatment was eventually required in 7 patients (16.6%). Median overall survival (OS) has not been reached. Grouped by ds-GPA (≤ 2 vs. >2) the survival curves showed a significant split (p = 0.039). OS differed also significantly between BMV-risk groups when grouped into low vs. intermediate/high risk groups (p = 0.025). No grade 4 or 5 acute or late toxicity was observed.
Conclusion: In selected patients with relapse after SRS for brain metastases, repeat courses of SRS were safe and minimized the need for rescue WBRT. The innovative, yet easy to calculate metric BMV may facilitate treatment decisions as a prognostic factor for OS.
Introduction
Treatment of brain metastases is still a challenge since different treatment goals need to be weighed: for one thing preventing progression of metastases with its associated neurological deterioration then again ensuring quality of life and limiting treatment-associated morbidity (1). In the past, whole-brain radiation therapy (WBRT) was standard of care for symptom control and presumably prolonged survival. Still, with its risk of neurotoxicity and early functional impairment (2–5), its inferior rate of local control (6) and its questionable impact on overall survival (7) the role of WBRT in the treatment of limited numbers of brain metastases (≤4) has been challenged and transformed over the past 10 years.
Brain metastases serve as the ideal target for stereotactic radiosurgery (SRS) (1, 8) and the efficacy of this treatment has been proven in several prospective phase III trials (2, 4, 6, 9). Consequently, SRS alone has been recommended within national guidelines as the preferred radiation treatment option for up to four asymptomatic brain metastases (1, 10, 11) to defer WBRT. For patients with more than 4 metastases SRS without WBRT has equally shown encouraging results regarding overall survival and toxicity (12).
Despite the excellent local control rates of SRS only, up to 50% of patients will present with new brain metastases within a year in terms of distant brain failure (4, 6, 9, 12). The optimal salvage treatment in this situation has not yet been defined. WBRT is still administered, but challenges to WBRT remain the same as in the primary situation. Furthermore, the concept of salvage WBRT has been investigated particularly as a subset of patients with long term WBRT-free survival can be identified by predictive parameters (13). Therefore, repeat radiosurgery for new brain metastases to defer or even avoid WBRT represents an attractive concept, although data considering efficacy, safety or optimal patient selection is still missing. Only few groups have recently reported retrospective analyses of their experience with repeated courses of radiosurgery (14–18) and hence optimal patient selection appears crucial.
The graded prognostic assessment (GPA) predicts survival upon the initial diagnosis of brain metastases (19–22) and potentially remains prognostic for new lesions treated with repeated courses of SRS (23). Recently, Farris et al. have introduced a novel metric, prognostic of overall survival: the clinical metric brain metastasis velocity (BMV) serves as an estimate for development of new metastases over time and is not only associated with overall survival, but also predicts need of salvage therapy in case of distant brain failure or risk of neurological death (24).
Within this retrospective study, we present our results of the feasibility, toxicity, and outcome of repeated courses of radiosurgery for the treatment of new brain metastases and examine the value of the metric BMV.
Patients and Methods
Patient Eligibility
From February 2014 until August 2017, 42 patients with 197 brain metastases were treated with at least 2 courses of SRS for either intact brain lesions or resection cavities at the University Hospital of Zurich. According to institutional standards, repeat SRS was given, if all new lesions (≤ 9 lesions at a time) were deemed amenable for SRS (criteria defined in treatment section) and the patient was assessed in good performance status by the treating physician. A multi-disciplinary tumor board approved all indications.
All patients received a physical examination and an oncological re-staging by either computed tomography (CT) imaging or combined whole body positron emission tomography (PET) with CT imaging. Cerebral metastases were assessed by contrast enhanced high-resolution cranial magnetic resonance imaging (MRI).
Treatment
According to institutional guidelines following international recommendations (1, 9, 12, 25, 26) treatment was delivered as follows:
All patients were immobilized using a dedicated frame-less stereotactic mask system (CIVCO Medical Solutions, Coralville, IA, USA). Planning-CT was done in treatment position using a 0.75–1 mm slice thickness and intravenous (IV) contrast agent. A dedicated planning MRI with IV contrast agent including a volumetric T1 sequence and a slice thickness of 0.6 mm was acquired. Both image modalities were fused using rigid image registration for target delineation. For contouring, the MRI-visible gross tumor volume (GTV) was delineated without additional margin as clinical target volume (CTV). The planning target volume (PTV) was derived by adding an isotropic margin of 1 mm. In contrast, for resected brain metastases the cavities were delineated as CTV. In this case, the PTV was derived by adding an isotropic margin of 2 mm. Patients were grouped for dose prescription: For 1–4 small metastases with diameter of ≤ 2.5 cm each, a single fraction scheme was used (1 × 18–20 Gy). If metastases measured > 2.5 cm, 6 fractions of 5 Gy were prescribed. For patients with 5–9 metastases, the threshold was a diameter of 1 cm for either single doses SRS or, if larger in size, 6 fractions of 5 Gy was used. If >9 metastases were treated, the regimen with 6 fractions was also favored. Postsurgical treatments were delivered in 6 × 5 Gy. All doses were prescribed to the PTV encompassing the 80%-isodose line and delivered as Volumetric Modulated Arc Therapy (VMAT) using True Beam or Edge linear accelerators (LINACs) (Varian Medical Systems, Inc., Palo Alto, CA, USA).
Toxicity and Endpoint Definitions
We followed patients from time of initial SRS until death or closure of data-entry. For the first year, cMRI and clinical examination were appointed every 3 months and thereafter at 4 months' intervals. Toxicity was graded according to National Cancer Institute Common Terminology Criteria for Adverse Events (CTCAE v4.0) and classified as acute (up to 3 months after respective course of SRS) or late toxicity.
Treatment response and radiation necrosis (RN) as an adverse event were investigated following recommendations of the response assessment in neuro- oncology (RANO)-group (27). Gadolinium-enhanced MRI served as a basis for response assessment. Local control (LC) of a treated lesion was defined as either response or stable disease in the last follow-up cMRI scans. Newly diagnosed out-of-field lesions were regarded as out of field brain failure (OOFBF). In order to distinguish between true progression and pseudo-progression, time course over all follow-up MRI-scans was taken in account (28). The diagnosis of RN followed the RANO and was derived by suggested multimodal diagnostics (29): if RN was suspected in standard cMRI, susceptibility-weighted contrast enhanced (DSC) perfusion MRI or 18F-Fluorethyltyrosin (18FLT)-PET were performed. A RN was called symptomatic RN if a patient presented with clinical symptoms as nausea, headache or fatigue.
Dying with increased or new neurological dysfunction or progressive metastases was considered neurological death, whereas the status of stable metastases was not (30).
Statistics
Actuarial survival time and tumor control as freedom from local failure (LF) or OOFBF were calculated according to the Kaplan-Meier method. OOFBF was assessed patient-specifically after the individual courses. In contrast, freedom from LF was determined as lesion-specific local control from time of corresponding course to date of the event. Overall survival was calculated from each course of SRS to the time of death.
To analyze prognostic factors for OS a Cox proportional hazard model (P < 0.05) was trained. Primarily a univariate analysis was performed, followed by a multivariate analysis of factors that were prognostic on univariate level. Histology (NSCLC vs. others) and diagnostic-specific GPA (0–2 vs. 2.5–4) were analyzed as categorical variables.
BMV was calculated as the cumulative number of new brain metastases that developed since initial SRS over time. Within our cohort it was determined at time of first DBF after the first course of SRS treatment. According to proposed risk profile groups (24), patients were classified as follows:
• low risk: < 4 new metastases/year.
• intermediate risk: 4–13 new metastases/year.
• high risk: >13 new metastases/year.
Results
Patient, Tumor, and Treatment Characteristics
We identified 42 consecutive patients who received repeated courses of SRS from our institutional database at the University-Hospital Zurich. At time of first treatment most presented with good Karnofsky performance status (KPS) of median of 80% (range: 50–100%) and a median age of 56 years (range: 35–83 years). Ds-GPA was calculated individually with a median of 2.5 (range: 1–4). 12 of 23 patients (52%) presented with non-small cellular lung carcinoma as primary tumor diagnosis; 9 of these with adenocarcinoma. Further patient characteristics are summarized in Table 1.
Within the observation period, a total number of 197 metastases from 100 SRS courses was treated. The median size of the GTV was 0.3 cm3 (range: 0.1–39.4 cm3) whereas the median size of the PTV was 0.7 cm3 (range: 0.1–55.4 cm3). The sum of all GTVs for a single patient at one treatment period was defined as aggregated volume (AV). The median AV per course was 1.2 cm3 (range: 0.1–48.4 cm3). Hundred and Twenty-One (61%) of the lesions were treated with a single dose SRS of 18 or 20 Gy. The remaining 76 lesions received fractionated treatments of 6 fractions with 5 Gy single dose (see treatment characteristics in Table 2).
Ds-GPA Score
As Figure 1 demonstrates the median ds-GPA remained stable during the entire treatment time. However, a shift of the quartiles and the range toward lower ds-GPA values was obvious within subsequent treatment courses.
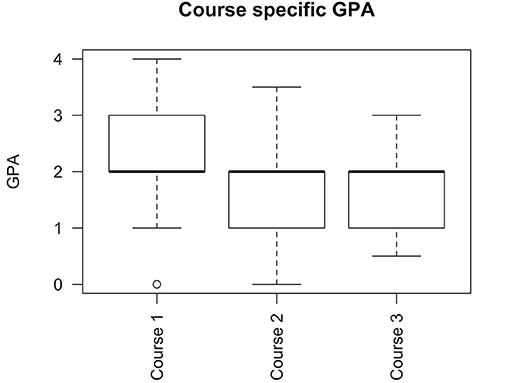
Figure 1. Course specific GPA. Boxplot showing median, 1st and 3rd quartile and 95% CI. A median GPA of 2 for all courses was observed.
Brain Metastasis Velocity
Median 3.5 new metastases / year (range: 0.3–22.1) were observed at time of first distant failure. Twenty-Three of Forty-Two patients (55%) could be grouped within low-risk (< 4 new metastases/year), Twelve (28%) had an intermediate-risk (4–13 new metastases/year), and Seven of Forty-patients (17%) were in the high-risk group (>13 new metastases/year).
Brain Tumor Control
With a median follow-up of 17.4 months (range: 4.6–45.5 months) after the first course of treatment a local control rate of 84% was observed at 1 year and 67% at 2 years independently of treatment course. Figure 2 shows Kaplan-Meier-Plot for local control. There was no statistically significant difference to be found between lesions treated with SRS vs. fSRT (p = 0.6).
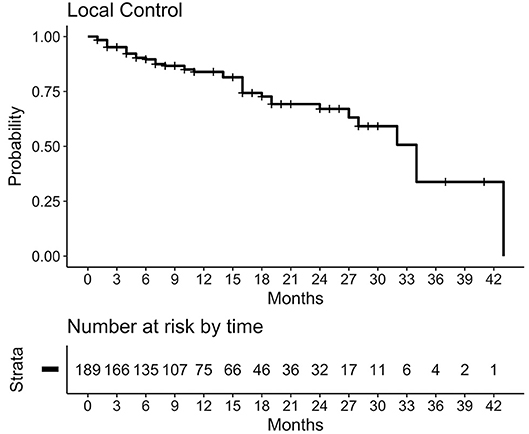
Figure 2. Kaplan-Meier-Plot for local control. For 189 lesions (with at least one follow-up with cMRI) a 1-year local control rate of 84% was observed, calculated from time of corresponding course to date of the event. Censored in case no event occurred or if WBRT had to be administered.
Considering overall brain control, median time to out-of-field-brain-failure (OOFBF) was 7 months (95%CI 4–8 months) after the first treatment and 6 months (95%CI 3–17 months) after the second. One-year and Two-year cumulative incidence of OOFBF after first course was 83 and 95%, respectively. Figure 3 shows Kaplan-Meier-Plot for OOFBF.
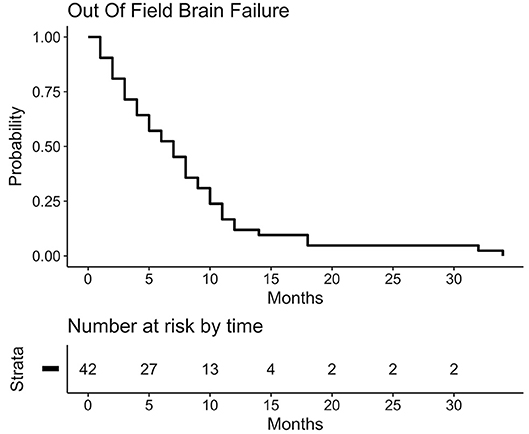
Figure 3. Kaplan-Meier-Plot for out-of-field brain control after 1st treatment course. Censored in case no event occurred or if WBRT had to be administered.
Salvage WBRT
Seven of Forty-Two (16.6%) patients were not amenable to another course of repeat SRS at time of second recurrence and had received WBRT within a median interval of 6 months (range: 4–13 months) to the first SRS course.
Patient Survival: Univariate and Multivariate Analysis
From date of first diagnosis of brain metastases a median OS has not been reached at time of final analysis. As Figure 4 demonstrates, the survival curves showed a significant split (p = 0.039), when grouped by ds-GPA (≤ 2 vs. >2). The median OS for patients with a ds-GPA >2 after diagnosis of first brain metastasis has not been achieved. A median OS of 21 months (95% CI: 17-NA) for patients with ds-GPA ≤ 2 was observed.
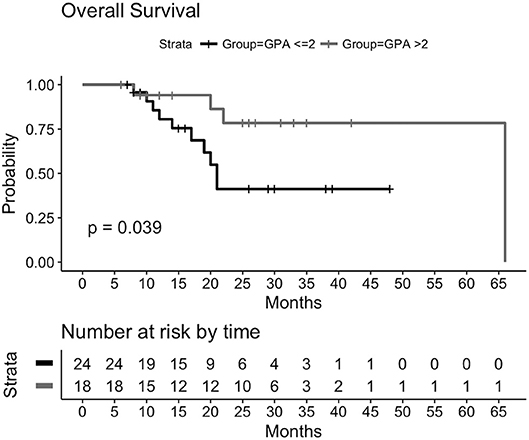
Figure 4. Kaplan-Meier-Plot for overall survival grouped by ds-GPA ≤ 2 vs. >2 after time of first diagnosis of brain metastases. Median overall survival for ds-GPA >2 after diagnosis of first brain metastasis has not been reached. For ds-GPA ≤ 2: median OS of 21 months (95% CI: 17-NA).
BMV proved to be a prognostic metric for OS calculated from time of first distant-brain-failure. Figure 5 displays how survival curves differ significantly for low-risk (23 patients, 55%) vs. intermediate- and high-risk patients (19 patients, 45%) (p = 0.025). Median OS from time of first DBF for the low-risk group has not been reached, whereas the median OS for the combined group was 10 months (95% CI: 7-NA).
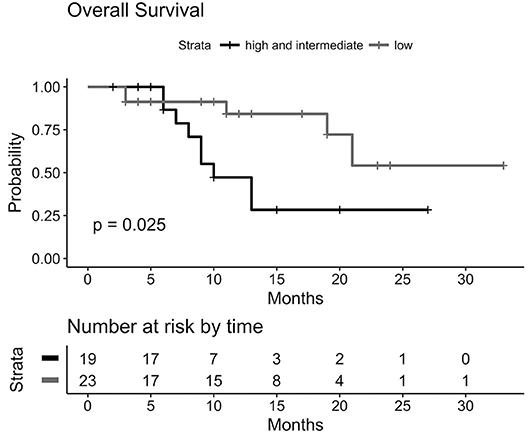
Figure 5. Kaplan-Meier-Plot for Overall Survival grouped by BMV risk group. For low-risk group median OS has not been reached after first DBF. Combined intermediate /high-risk group: median OS of 10 months (95%CI: 7-NA).
At time of last follow-up 28 of 42 (67%) patients were still alive. Eight of Fourteen (57%) deaths can be attributed to neurological death according to the definition in the methods section.
Results of univariate analysis are shown in Table 3. On univariate analysis, local control of the primary tumor at time of second course and the calculated BMV after the first course correlated significantly with OS. For multivariate analysis we looked at the influence of ds-GPA, which already includes various clinical parameters, and additional non-included clinical parameters like aggregated treated tumor and brain metastases velocity. Results are shown in Table 4. BMV remained significantly correlating with OS [p = 0.04; HR: 1.10 (1.00–1.21)].
Toxicity
Within 197 treated lesions 14 sites of RN were observed in 10 (23.8%) patients. None of the patients suffered from severe symptoms. Treatment was only needed for symptomatic RN (n = 5, 35.7%). All symptoms resolved after a short course of dexamethasone as temporary treatment and no resection was needed in any of our patients.
Eighteen of Forty-One (one patient did not have a FU within 90 days) patients (43.9%) developed acute toxicities, with grade 3 in 2 (4.8%), grade 2 in 11 (26.8%), and grade 1 in 6 (14.6%). Eighteen of all Forty-Two patients suffered from low-grade late toxicities: 2 (4.8%) with grade 3, 14 (33.3%) with grade 2, and 2 (4.8%) with grade 1. No grade 4 or 5 toxicity occurred. Figure 6 shows distribution of observed toxicity. Considering presenting symptoms, we observed edema as the most common acute and chronic side effect, provided that edema was diagnosed on regular follow-up MRI scans. It was followed by headache and vertigo, which were defined clinically. Detailed symptoms and grades of acute and late toxicity are reported for patients individually in tables in the Supplementary Material.
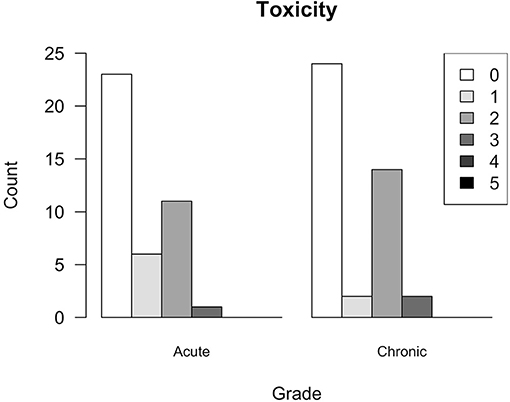
Figure 6. Toxicity. Acute toxicity graded according to Criteria for Adverse Events (CTCAE v4.0) during and ≤ 90 days after respective course of SRS; chronic toxicity occurring >90 days after respective course of SRS. Graph shows highest grade of toxicity observed for individual patients during any course of treatment.
Discussion
Administering SRS only has become the accepted standard of care in treatment of limited number of newly diagnosed brain metastases (2, 4, 6, 9, 12). Compared to WBRT, this approach significantly reduces the risk for neurocognitive impairment (3–5), while at the same time accepting higher rates of distant brain relapse of up to 60% (4, 6, 9, 12). For the frequent scenario of newly occurring brain metastases after initial SRS treatment, a valid standard of care has not been established by prospective trials. WBRT is still the predominantly used treatment option whereas the application of repeated courses of SRS represents an emerging concept, but still provokes safety concerns, since only little data exists.
Recently, a retrospective analysis of 95 patients with 652 metastases treated with repeated SRS only showed that newly diagnosed metastases could be treated safely and effectively with additional courses of SRS (13). Notwithstanding the well-known high rate of distant intracranial progression, only 20% of patients within this study eventually received WBRT as salvage treatment. The authors concluded that WBRT might even be avoided completely for patients with brain metastases. Our findings support this conclusion with a comparable rate of administered WBRT in 17% of our patients.
Although all existing data is limited by the recognized biases of retrospective analyses, patients treated with multiple courses of SRS have acceptable rates of toxicity and no sign of increased neurological death (12, 14, 16–18, 31). Overall, only 14 sites of RN were observed in 197 treated lesions (7%) within our cohort, which is reasonable and comparable to incidences reported in previous studies (14, 16, 30, 32–34). Still, no recommendation exists to what extent repeat radiosurgery is truly safe and what parameters regarding dose overlap should be used to judge about safety. It seems prudent to apply the same general constraints as in first course SRS and to avoid significant dose overlap (e.g., >50% IDL overlap). Also, overall survival is very favorable in our cohort and—although it rather reflects careful patient selection—it is reassuring that deferring WBRT in such patients appears to be a reasonable approach.
It remains controversial which subpopulation of BM patients benefits most from local treatment including repeat SRS (35–37). Determination of predictive factors and development of prognostic indices is crucial in deriving a solid basis for decision-making. The GPA, developed to guide these decisions, has been validated in WBRT as well as SRS treated brain metastases patients (19, 21). Recently, it has been expanded and validated to a disease specific prognostic score (20, 38), even incorporating information of tumor specific mutations (39). Interestingly, as reported by Shultz et al. and Yamamoto et al., GPA remained valid as a prognostic tool for repeat SRS (14, 23). Within our cohort the ds-GPA on univariate and multivariate analysis did not prove to be predictive for overall survival. However, the survival curves split significantly. Interestingly, ds-GPA did not change significantly over time.
Various models assisting clinicians in deciding between administration of WBRT or SRS as initial treatment have been developed. All of them intend to enable estimation of risk of Rodrigues et al. (40) and time to Ayala-Peacock et al. and Press et al. (41, 42) DBF. All of these studies included the number of primarily diagnosed metastases in their scoring system, as it was significantly predictive for early DBF after initial treatment. However, the effect of the estimated number of future metastases or of the dynamics of relapse on overall survival has so far not been investigated. Therefore, an innovative and promising new metric BMV has been developed (24). BMV was established by analysis of 737 patients treated with upfront SRS only for new brain metastases and represents the rate of new metastases that develop over time. The value can be re-calculated over a patient's disease course multiple times and stays prognostic. A multi-institutional study with almost 3,000 has recently validated the BMV as a dominant predictor for OS but the full results have not been published yet (43). BMV has also been included into an announced web-based predictive tool which is not online yet (44). With a median BMV of 3.5 new metastases/year (range: 0.3–22.1) our cohort compared favorably to the reported median BMV of 5.5 (range: 0.2–156.4) by Farris et al. BMV correlated significantly with OS when analyzed as a continuous variable and when factored in the suggested risk stratified groups (BMV < 4, 4–13, >13). Higher BMV predicted for shorter OS, especially when low and high-risk groups were directly compared [P = 0.0006, HR (95%CI) = 23.78 (3.88–145.74)] and BMV remained the only significant factor for OS in the multivariable model. Our findings with a median OS of 10 months for the combined intermediate and high-risk group exceed values previously reported by the authors (8.2 and 4.3 months), which might be a combined effect of small sample size and favorable patient selection. BMV seems to be a powerful and promising prognostic metric, even in a small cohort such as ours.
The results of the present study are prone to bias inherent to retrospective studies, particularly selection bias. Furthermore, the ability to analyze patient-specific variables with regard to predictive character is limited due to the small cohort of 42 patients and statistics beyond univariate analysis should be viewed with caution.
Conclusion
Considering the excellent local control rate, the low toxicity profile and the long OS observed within this study, distant intracranial relapse should not preclude administering SRS: In selected patients with various relapses of brain metastases (DBF) amenable to SRS, repeat courses of SRS can safely be administered to defer or even avoid WBRT. The innovative metric BMV also proved to be prognostic in our cohort and should be further evaluated as a decision-guiding metric.
Data Availability Statement
The raw data supporting the conclusions of this manuscript will be made available by the authors, without undue reservation, to any qualified researcher.
Ethics Statement
This study was carried out in accordance with the recommendations of the Humanforschungsgesetz (HFG) and the Kantonale Ethikkommission Zürich with written informed consent from all subjects. All subjects gave written informed consent in accordance with the Declaration of Helsinki. The protocol was approved by the Kantonale Ethikkommission Zürich.
Author Contributions
CF and NA designed and directed the analysis. CF, KB, and SK performed data collection in a database generated by LS, ST-L, and JK. Statistical analysis was performed by CF and KB. CF contributed to the analysis of the results and to the writing of the manuscript. NA and MG supervised the project.
Conflict of Interest Statement
The authors declare that the research was conducted in the absence of any commercial or financial relationships that could be construed as a potential conflict of interest.
Supplementary Material
The Supplementary Material for this article can be found online at: https://www.frontiersin.org/articles/10.3389/fonc.2018.00551/full#supplementary-material
References
1. Kocher M, Wittig A, Piroth DM, Treuer H, Seegenschmiedt H, Ruge M, et al. Stereotactic radiosurgery for treatment of brain metastases. Strahlentherapie und Onkol. (2014) 190:521–32. doi: 10.1007/s00066-014-0648-7
2. Brown PD, Jaeckle K, Ballman KV, Farace E, Cerhan JH, Anderson SK, et al. Effect of radiosurgery alone vs radiosurgery with whole brain radiation therapy on cognitive function in patients with 1 to 3 brain metastases: a randomized clinical trial. JAMA (2016) 316:401–9. doi: 10.1001/jama.2016.9839
3. Aoyama H, Tago M, Kato N, Toyoda T, Kenjyo M, Hirota S, et al. Neurocognitive function of patients with brain metastasis who received either whole brain radiotherapy plus stereotactic radiosurgery or radiosurgery alone. Int J Radiat Oncol. (2007) 68:1388–95. doi: 10.1016/j.ijrobp.2007.03.048
4. Chang EL, Wefel JS, Hess KR, Allen PK, Lang FF, Kornguth DG. Neurocognition in patients with brain metastases treated with radiosurgery or radiosurgery plus whole-brain irradiation: a randomised controlled trial. Lancet Oncol. (2009) 10:1037–44. doi: 10.1016/s1470-2045(09)70263-3
5. Gondi V, Paulus R, Bruner DW, Meyers CA, Gore EM, Wolfson A, et al. Decline in tested and self-reported cognitive functioning following prophylactic cranial irradiation for lung cancer: pooled secondary analysis of RTOG randomized trials 0212 and 0214. Int J Radiat Oncol Biol Phys. (2013) 86:656–64. doi: 10.1016/j.ijrobp.2013.02.033
6. Aoyama H, Shirato H, Tago M, Nakagawa K, Toyoda T, Hatano K. Stereotactic radiosurgery plus whole-brain radiation therapy vs stereotactic radiosurgery alone for treatment of brain metastases: a randomized controlled trial. JAMA (2006) 295:2483–91. doi: 10.1001/jama.295.21.2483
7. Mulvenna P, Nankivell M, Barton R, Faivre-Finn C, Wilson P, McColl E, et al. Dexamethasone and supportive care with or without whole brain radiotherapy in treating patients with non-small cell lung cancer with brain metastases unsuitable for resection or stereotactic radiotherapy (QUARTZ): results from a phase 3, non-inferiority. Lancet (2016) 388:2004–14. doi: 10.1016/S0140-6736(16)30825-X
8. Sturm V, Kober B, Hover K-H, Schlegel W, Boesecke R, Pastyr O, et al. Stereotactic percutaneous single dose irradiation of brain metastases with a linear accelerator. Int J Radiat Oncol Biol Phys. (1987) 13:279–82. doi: 10.1016/0360-3016(87)90140-4
9. Kocher M, Soffietti R, Abacioglu U, Villà S, Fauchon F, Baumert BG, et al. Adjuvant whole-brain radiotherapy versus observation after radiosurgery or surgical resection of one to three cerebral metastases: results of the EORTC 22952-26001 study. J Clin Oncol. (2011) 29:134–41. doi: 10.1200/JCO.2010.30.1655
10. Tsao MN, Rades D, Wirth A, Lo SS, Danielson BL, Gaspar LE, et al. Radiotherapeutic and surgical management for newly diagnosed brain metastasis(es): an American Society for radiation oncology evidence-based guideline. Pract Radiat Oncol. (2012) 2:210–25. doi: 10.1016/j.prro.2011.12.004
11. American, Society for Radiation Oncology,. Choosing Wisely. Available online at: http://www.choosingwisely.org/societies/american-society-for-radiation-oncology/ (accessed June 5, 2018).
12. Yamamoto M, Serizawa T, Shuto T, Akabane A, Higuchi Y, Kawagishi J, et al. Stereotactic radiosurgery for patients with multiple brain metastases (JLGK0901): a multi-institutional prospective observational study. Lancet Oncol. (2014) 15:387–95. doi: 10.1016/S1470-2045(14)70061-0
13. Gorovets D, Rava P, Ebner DK, Tybor DJ, Cielo D, Puthawala Y, et al. Predictors for long-term survival free from whole brain radiation therapy in patients treated with radiosurgery for limited brain metastases. Front Oncol. (2015) 5:110. doi: 10.3389/fonc.2015.00110
14. Shultz DB, Modlin LA, Jayachandran P, Von Eyben R, Gibbs IC, Choi CYH, et al. Repeat Courses of Stereotactic Radiosurgery (SRS), deferring whole-brain irradiation, for new brain metastases after initial SRS. Int J Radiat Oncol. (2015) 92:993–9. doi: 10.1016/j.ijrobp.2015.04.036
15. Minniti G, Scaringi C, Paolini S, Clarke E, Cicone F, Esposito V, et al. Repeated stereotactic radiosurgery for patients with progressive brain metastases. J Neurooncol. (2016) 126:91–7. doi: 10.1007/s11060-015-1937-4
16. Kim DH, Schultheiss TE, Radany EH, Badie B, Pezner RD. Clinical outcomes of patients treated with a second course of stereotactic radiosurgery for locally or regionally recurrent brain metastases after prior stereotactic radiosurgery. J Neurooncol. (2013) 115:37–43. doi: 10.1007/s11060-013-1191-6
17. Shen CJ, Rigamonti D, Redmond KJ, Kummerlowe MN, Lim M, Kleinberg LR. The strategy of repeat stereotactic radiosurgery without whole brain radiation treatment for new brain metastases: outcomes and implications for follow-up monitoring. Pract Radiat Oncol. (2016) 6:409–16. doi: 10.1016/j.prro.2016.04.004
18. Koiso T, Yamamoto M, Kawabe T, Watanabe S, Sato Y, Higuchi Y, et al. Follow-up results of brain metastasis patients undergoing repeat Gamma Knife radiosurgery. Spec Suppl. (2016) 125:2–10. doi: 10.3171/2016.6.gks161404
19. Sperduto PW, Berkey B, Gaspar LE, Mehta M, Curran W. A new prognostic index and comparison to three other indices for patients with brain metastases: an analysis of 1,960 patients in the RTOG database. Int J Radiat Oncol. (2008) 70:510–4. doi: 10.1016/j.ijrobp.2007.06.074
20. Likhacheva A, Pinnix CC, Parikh N, Allen PK, Guha-Thakurta N, McAleer M, et al. Validation of recursive partitioning analysis and diagnosis-specific graded prognostic assessment in patients treated initially with radiosurgery alone. J Neurosurg. (2012) 117:38–44. doi: 10.3171/2012.3.GKS1289
21. Nieder C, Bremnes RM, Andratschke NH. Prognostic scores in patients with brain metastases from non-small cell lung cancer. J Thorac Oncol. (2009) 4:1337–41. doi: 10.1097/JTO.0b013e3181b6b6f4
22. Nieder C, Andratschke NH, Geinitz H, Grosu AL. Use of the graded prognostic assessment (GPA) score in patients with brain metastases from primary tumours not represented in the diagnosis-specific GPA studies. Strahlentherapie und Onkol. (2012) 188:692–5. doi: 10.1007/s00066-012-0107-2
23. Yamamoto M, Kawabe T, Higuchi Y, Sato Y, Nariai T, Watanabe S, et al. Validity of prognostic grading indices for brain metastasis patients undergoing repeat radiosurgery. World Neurosurg. (2014) 82:1242–9. doi: 10.1016/j.wneu.2014.08.008
24. Farris M, McTyre ER, Cramer CK, Hughes R, Randolph DM, Ayala-Peacock DN, et al. Brain metastasis velocity: a novel prognostic metric predictive of overall survival and freedom from whole-brain radiation therapy after distant brain failure following upfront radiosurgery alone. Int J Radiat Oncol Biol Phys. (2017) 98:131–41. doi: 10.1016/j.ijrobp.2017.01.201
25. Yamamoto M, Kawabe T, Sato Y, Higuchi Y, Nariai T, Barfod BE, et al. A case-matched study of stereotactic radiosurgery for patients with multiple brain metastases: comparing treatment results for 1–4 vs ≥ 5 tumors: clinical article. J Neurosurg. (2013) 118:1258–68. doi: 10.3171/2013.3.JNS121900
26. Yamamoto M, Kawabe T, Sato Y, Higuchi Y, Nariai T, Watanabe S, et al. Stereotactic radiosurgery for patients with multiple brain metastases: a case-matched study comparing treatment results for patients with 2–9 versus 10 or more tumors: clinical article. J Neurosurg. (2014) 121:16–25. doi: 10.3171/2014.8.GKS141421
27. Lin NU, Lee EQ, Aoyama H, Barani IJ, Barboriak DP, Baumert BG, et al. Response assessment criteria for brain metastases: proposal from the RANO group. Lancet Oncol. (2015) 16:e270–8. doi: 10.1016/S1470-2045(15)70057-4
28. Patel TR, McHugh BJ, Bi WL, Minja FJ, Knisely JPS, Chiang VL. A comprehensive review of MR imaging changes following radiosurgery to 500 brain metastases. Am J Neuroradiol. (2011) 32:1885–92. doi: 10.3174/ajnr.A2668
29. Shah R, Vattoth S, Jacob R, Manzil FFP, O'Malley JP, Borghei P, et al. Radiation necrosis in the brain: imaging features and differentiation from tumor recurrence. RadioGraphics (2012) 32:1343–59. doi: 10.1148/rg.325125002
30. Patchell RA, Tibbs PA, Regine WF, Dempsey RJ, Mohiuddin M, Kryscio RJ, et al. Postoperative radiotherapy in the treatment of single metastases to the brain: a randomized trial. JAMA (1998) 280:1485–9. doi: 10.1001/jama.280.17.1485
31. Mariya Y, Sekizawa G, Matsuoka Y, Seki H, Sugawara T, Sasaki Y. Repeat stereotactic radiosurgery in the management of brain metastases from non-small cell lung cancer. Tohoku J Exp Med. (2011) 223:125–31. doi: 10.1620/tjem.223.125
32. Chen JCT, Petrovich Z, Giannotta SL, Yu C, Apuzzo MLJ. Radiosurgical salvage therapy for patients presenting with recurrence of metastatic disease to the brain. Neurosurgery (2000) 46:860–7; discussion: 866–7. doi: 10.1097/00006123-200004000-00017
33. Hillard VH, Shih LL, Chin S, Moorthy CR, Benzil DL. Safety of multiple stereotactic radiosurgery treatments for multiple brain lesions. J Neurooncol. (2003) 63:271–8. doi: 10.1023/a:1024251721818
34. McKay WH, McTyre ER, Okoukoni C, Alphonse-Sullivan NK, Ruiz J, Munley MT, et al. Repeat stereotactic radiosurgery as salvage therapy for locally recurrent brain metastases previously treated with radiosurgery. J Neurosurg. (2016) 127:148–56. doi: 10.3171/2016.5.jns153051
35. Nieder C, Spanne O, Mehta MP, Grosu AL, Geinitz H. Presentation, patterns of care, and survival in patients with brain metastases. Cancer (2011) 117:2505–12. doi: 10.1002/cncr.25707
36. Mehta MP, Aoyama H, Gondi V. The changing role of whole-brain radiotherapy: demise or time for selective usage? JAMA Oncol. (2017) 3:1021–2. doi: 10.1001/jamaoncol.2016.5414
37. Li J, Brown PD. The diminishing role of whole-brain radiation therapy in the treatment of brain metastases. JAMA Oncol. (2017) 1023–4. doi: 10.1001/jamaoncol.2016.5411
38. Sperduto PW, Chao ST, Sneed PK, Luo X, Suh J, Roberge D. Diagnosis-specific prognostic factors, indexes, and treatment outcomes for patients with newly diagnosed brain metastases: a multi-institutional analysis of 4,259 patients. Int J Radiat Oncol Biol Phys. (2010) 77:655–1. doi: 10.1016/j.ijrobp.2009.08.025
39. Sperduto PW, Yang TJ, Beal K, Pan H, Brown PD, Bangdiwala A, et al. Estimating survival in patients with lung cancer and brain metastases: an update of the graded prognostic assessment for lung cancer using molecular markers (Lung-molGPA). JAMA Oncol. (2016) 3:827–31. doi: 10.1001/jamaoncol.2016.3834
40. Rodrigues G, Warner A, Zindler J, Slotman B, Lagerwaard F. A clinical nomogram and recursive partitioning analysis to determine the risk of regional failure after radiosurgery alone for brain metastases. Radiother Oncol. (2014) 111:52–8. doi: 10.1016/j.radonc.2013.11.015
41. Ayala-Peacock DN, Peiffer AM, Lucas JT, Isom S, Kuremsky JG, Urbanic JJ, et al. A nomogram for predicting distant brain failure in patients treated with gamma knife stereotactic radiosurgery without whole brain radiotherapy. Neuro Oncol. (2014) 16:1283–8. doi: 10.1093/neuonc/nou018
42. Press RH, Prabhu RS, Nickleach DC, Liu Y, Shu HG, Kandula S, et al. Novel risk stratification score for predicting early distant brain failure and salvage whole-brain radiotherapy after stereotactic radiosurgery for brain metastases. Cancer (2015) 121:3836–43. doi: 10.1002/cncr.29590
43. McTyre E, Farris M, Ayala-Peacock DN, Page BR, Shen C, Kleinberg LR, et al. Multi-institutional validation of brain metastasis velocity, a recently defined predictor of outcomes following stereotactic radiosurgery. Int J Radiat Oncol Biol Phys. (2017) 99:E93. doi: 10.1016/j.ijrobp.2017.06.813
44. Henson A, Ayala-Peacock DN, Chung C, Hepel JT, Chao ST, Contessa JN, et al. DBF 2.0: a web-based predictive model for distant brain failure, brain metastasis velocity, and early death after radiosurgery for brain metastases. Int J Radiat Oncol Biol Phys. (2017) 99:E78–9. doi: 10.1016/j.ijrobp.2017.06.778
Keywords: brain metastases (BM), stereotactic radiosurgery, repeat radiosurgery, brain metastasis velocity, whole brain radiation therapy (WBRT), salvage radiation therapy (SRT)
Citation: Fritz C, Borsky K, Stark LS, Tanadini-Lang S, Kroeze SGC, Krayenbühl J, Guckenberger M and Andratschke N (2018) Repeated Courses of Radiosurgery for New Brain Metastases to Defer Whole Brain Radiotherapy: Feasibility and Outcome With Validation of the New Prognostic Metric Brain Metastasis Velocity. Front. Oncol. 8:551. doi: 10.3389/fonc.2018.00551
Received: 12 September 2018; Accepted: 07 November 2018;
Published: 22 November 2018.
Edited by:
Sean P. Collins, Georgetown University, United StatesReviewed by:
Ima Paydar, Hospital of the University of Pennsylvania, United StatesMichael Charles Repka, Winthrop University Hospital, United States
Copyright © 2018 Fritz, Borsky, Stark, Tanadini-Lang, Kroeze, Krayenbühl, Guckenberger and Andratschke. This is an open-access article distributed under the terms of the Creative Commons Attribution License (CC BY). The use, distribution or reproduction in other forums is permitted, provided the original author(s) and the copyright owner(s) are credited and that the original publication in this journal is cited, in accordance with accepted academic practice. No use, distribution or reproduction is permitted which does not comply with these terms.
*Correspondence: Nicolaus Andratschke, nicolaus.andratschke@usz.ch