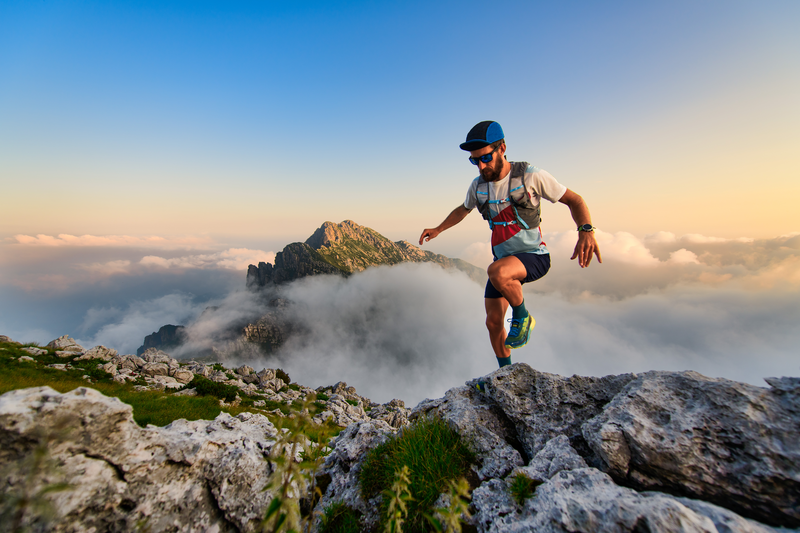
94% of researchers rate our articles as excellent or good
Learn more about the work of our research integrity team to safeguard the quality of each article we publish.
Find out more
HYPOTHESIS AND THEORY article
Front. Oncol. , 27 July 2018
Sec. Hematologic Malignancies
Volume 8 - 2018 | https://doi.org/10.3389/fonc.2018.00280
Myelodysplasia refers to a group of clonal hematopoietic neoplasms characterized by genetic heterogeneity, different clinical behaviors and prognoses. Some of this group of bone marrow failure conditions have known external causes, some are of unknown origin. Within marrow, intracellular, and extracellular elements of the innate immune system are activated and contribute to creation of multiple cytogenetic abnormalities and are central to the mode of hematopoietic cell failure. Basiorka et al. showed that NLRP3 inflammasome activity is essential to the innate immune system's destruction of marrow hematopoietic cells commonly in myelodysplasia. In April 2018 Hao et al. reported that methylene blue inhibits rat NLRP3 inflammasome function. Methylene blue has been in continuous use in humans for over a century. It is associated with an eminently benign side effect profile in human use. If as in rodents, methylene blue also inhibits NLRP3 inflammasome function in human myelodysplasia a trial of adjunctive methylene blue treatment in transfusion dependent, low risk myelodysplasia where marrow inflammation and apoptosis predominates, would be worth trying.
HIGHLIGHTS
- Cytogenetic abnormalities and innate immune activation are seen in myelodysplasia
- The NLRP3 inflammasome is a core element generating marrow failure of myelodysplasia
- In April 2018 methylene blue was reported to potently inhibit NLRP3 inflammasome function
- Methylene blue has benign side effects and has been in human use for a century
- Study of methylene blue treatment of myelodysplasia would be a low-risk intervention
Myelodysplasia (MDS) refers to a group of clonal hematopoietic neoplasms characterized by genetic heterogeneity, differences in clinical behavior and prognosis, the incidence of which rises sharply with age (1). The clinical picture is variable, encompassing hematopoietic failure syndromes with pancytopenia, hypocellular dysplastic marrow, ineffective hematopoiesis, marrow stem cell macrocytosis, production of aberrant clones, a high marrow cell apoptosis rate (particularly in early stage disease), and multiple gross cytogenetic abnormalities (2, 3). A large number of different mutations are seen even within histologically homogeneous MDS patients, particularly in genes. related to RNA splicing machinery (4). Immunophenotyping with flow cytometry can provide indicators of low risk MDS (5) and is an important aspect to MDS subclassification (6).
Clinical heterogeneity need not be reflected by mutational heterogeneity (7). Seventeen percentage of people presenting with pancytopenia will be found to have MDS on marrow exam (8). Although MDS can run a smoldering course, an accelerated stage can supervene at some point. Differentiating therapies for (a) low risk MDS with repeated erythropoietin, lenalidomide, or various drugs to diminish immune mediated or inflammation related hematopoietic cell apoptosis, and (b) drugs for high risk MDS like azacitidine, decitabine, dose cytarabine, or marrow cytotoxic chemotherapy (7, 9, 10) leave room for improvement since across all MDS subtypes overall mortality at 5 years remains around 50% (1).
Bone marrow transplantation can be curative but carries its own morbidity and mortality and many MDS patients aren't eligible for transplantation (7). This paper presents the rationale for a currently available low-risk adjunct compatible with current treatment options to hopefully improve MDS prognosis.
Association of inflammation and chronic immune stimulation has long been associated with MDS, with destructive inflammation feedback amplification loops active in disease progression. Difficulties in modeling bone marrow niche in which MDS develops are well-known, limiting drug screening applicable to MDS. Below I show how a recent discovery about an old medicine might be able to clinically inhibit a key link in MDS pathophysiology, thereby retarding MDS progression.
As part of innate immunity, a wide array (dozens) of intracellular sensors are built-in to mammalian cells detect intracellular pathogens or bacterial products (11). The 10 human Toll-like receptors (TLR) tend to be on cell outer surface. Retinoid acid-inducible gene-I (RIG-I)-like receptors, and nucleotide oligomerization domain (NOD) -like receptors (NLR) are classes of such detectors that tend to be intracellular. NLR form an essential element of epithelial integrity protection, particularly along the gut.
Pathogen-associated molecular patterns (PAMPS) are commonly encountered lipids, sugars, or peptides found in pathogens that would not otherwise be present. Mammals have pre-existing receptors that when stimulated by their cognate molecular pattern—a PAMP—set in motion various defensive physiology changes. Receptors for PAMPS constitute important elements of the non-adaptive, innate immune system.
PAMPS' presence triggers NLRs to associate in multiprotein complexes called inflammasomes that mediate caspase-1 activation and cytokine secretion (12, 13). Caspase-1 converts pro-IL-1 beta to active 17.5 kDa. IL-1 beta and can lead to inflammation generation or to a specialized form of cell death or sequentially to both.
Nucleotide Binding Domain Leucine-Rich Repeat-Containing Receptor, Pyrin Domain-Containing-3 (NLRP3) Inflammasome is one such innate receptor complex (13).
NLRP3 itself acts both as an innate immune system sensor for a variety of intracellular PAMPS as well as an upstream link in triggering an inflammation response (13), the multiprotein macromolecular inflammasome forming in response to intracytoplasmic PAMPS' recognition.
But caspase-1 activation by NLRP3 inflammasome formation can, and in MDS does, lead to hematopoietic stem cell death (14–16). Caspase-1 is 200 times higher in MDS hematopoietic stem cells compared to normal (14).
The NLRP3 is one of dozens of NLRs. The NLRP3 macromolecular complex forms a critical convergence point in generating the swelling, DNA fragmentation, and caspase 1 activation characteristic of a subgroup of MDS marrows (15, 16). Cytosolic NLRP3 activation and the flood of cytokines consequent to its activation play a major role in death of in hematopoietic stem/progenitor cells seen in MDS.
Although the full pathophysiology of MDS remains today unexplained, Sallman et al showed that inhibition of the NLRP3 inflammasome could restore effective hematopoiesis in (16) in MDS. Below I show how this might be achieved today with an FDA, EMA, and Health Canada approved drug—methylene blue (MB, same as methylthioninium chloride USP, available today as Proveblue™ or from Star Pharmaceuticals (http://www.starpharm.com/).
Beginning with Paul Ehrlich in the late 1800's (17) continuing to today, as of 2018 (18–20), MB has been used to treat malaria. Today's clinical use of MB however is mainly (i) for treating methemoglobinemia (21), (ii) as a marker in finding compartment leaks and fistulae, (iii) in treating ifosfamide encephalopathy, and (iii) in reversal of perioperative vasoplegic after or during coronary artery bypass surgery (22, 23). Side effects from MB are few, transient, and mild (20, 24).
MB can enhance mitochondrial function. Under some circumstances MB circumvents mitochondrial complexes 2 and 3 by accepting an electron from complex 1, then delivering it to cytochrome C (20, 25).
Of central importance to MDS, three recent independent studies showed that MB inhibits NLRP3 inflammasome function. Ahn et al. showed that MB inhibited LPS stimulated increase in murine NLRP3 function (26). Lin et al. showed that MB inhibited murine cord transection initiated NLRP3 activation (27). Hao et al. showed that MB inhibited NLRP3 assembly and function in streptozocin murine model of diabetic retinopathy (28).
We have only indirect evidence MB indeed inhibits NLRP3 activity in vivo. MB is clearly effective at stopping cerebral malaria in a murine model where NLRP3 is known to play a pivotal pathogenic role (29, 30). Of note this effect is independent of MB's anti-plasmodia effects (29, 30) with a parallel anti-inflammation action in minocycline's effect in cerebral malaria as predicted in 2008 and experimentally affirmed in a murine model in 2017 (31, 32).
Post-traumatic stress, when severe is associated with activated inflammation pathways and documented downstream cytokines known to be related to NLRP3 activation (33–35). As a consequence of these associations, a clinical trial of oral MB during standard exposure treatment of post-traumatic stress disorder showed benefit beyond that of exposure treatment plus placebo (35).
This short note combines, (A) the 2015 and 2016 observations of Basiorka et al. (14, 15) and 2016 work of Sallman (16)—all three reporting that NLRP3 inflammasome constitutes a final common effector node driving a core element of the pathophysiology of MDS with, (B) the 2017 data sets of Ahn et al. (26) and of Lin et al. (27) plus the 2018 report of Hao et al. (28)—all three reporting that the NLRP3 inflammasome can be inhibited by the century old drug MB.
Adjunctive treatment of MDS might best be explored in early phase, low risk disease where apoptosis predominates the marrow pathology picture (36).
Thus a cheap, low risk adjuvant treatment option, MB, is potentially available now that might retard MDS progression.
The author confirms being the sole contributor of this work and approved it for publication.
The author declares that the research was conducted in the absence of any commercial or financial relationships that could be construed as a potential conflict of interest.
MDS, myelodysplasic syndrome; NLR, nucleotide oligomerization domain (NOD) -like receptors; NLRP3, Nucleotide BindingDomain Leucine-Rich Repeat-Containing Receptor, Pyrin Domain-Containing-3; PAMPS, Pathogenassociated molecular pattern; TLR, Toll-like receptors.
1. Bonadies N, Feller A, Rovo A, Ruefer A, Blum S, Gerber B, et al. Trends of classification, incidence, mortality, and survival of MDS patients in Switzerland between 2001 and 2012. Cancer Epidemiol. (2017) 46:85–92. doi: 10.1016/j.canep.2016.12.005
2. Lindsley RC. Mutational complexity in myelodysplasia. Best Pract Res Clin Haematol. (2017) 30:290–4. doi: 10.1016/j.beha.2017.10.003
3. Zahid MF, Malik UA, Sohail M, Hassan IN, Ali S, Shaukat MHS. Cytogenetic abnormalities in myelodysplastic syndromes: an overview. Int J Hematol Oncol Stem Cell Res. (2017) 11:231–9.
4. Malcovati L, Ambaglio I, Elena C. The genomic landscape of myeloid neoplasms with myelodysplasia and its clinical implications. Curr Opin Oncol. (2015) 27:551–9. doi: 10.1097/CCO.0000000000000229
5. Aires A, Teixeira MDA, Lau C, Moreira C, Spínola A, Mota A, et al. A pilot study on the usefulness of peripheral blood flow cytometry for the diagnosis of lower risk myelodysplastic syndromes: the “MDS thermometer”. BMC Hematol. (2018)18:6. doi: 10.1186/s12878-018-0101-8
6. Porwit A, van de Loosdrecht AA, Bettelheim P, Brodersen LE, Burbury K, Cremers E, et al. Revisiting guidelines for integration of flow cytometry results in the WHO classification of myelodysplastic syndromes-proposal from the International/European LeukemiaNet Working Group for Flow Cytometry in MDS. Leukemia (2014) 28:1793–8. doi: 10.1038/leu.2014.191
7. Fenaux P, Seymour JF, Santini V, Silverman L, Gore S, List A, et al. Challenges of phase III trial design for novel treatments in diseases with no standard treatment: the AZA-001 myelodysplasia study model. Leuk Res. (2014) 38:258–62. doi: 10.1016/j.leukres.2013.10.014
8. Devitt KA, Lunde JH, Lewis MR. New onset pancytopenia in adults: a review of underlying pathologies and their associated clinical and laboratory findings. Leuk Lymphoma (2014) 55:1099–105. doi: 10.3109/10428194.2013.821703
9. List A, Ebert BL, Fenaux P. A decade of progress in myelodysplastic syndrome with chromosome 5q deletion. Leukemia (2018) 32:1493–9. doi: 10.1038/s41375-018-0029-9
10. List AF, Bennett JM, Sekeres MA, Skikne B, Fu T, Shammo JM, et al. Extended survival and reduced risk of AML progression in erythroid-responsive lenalidomide-treated patients with lower-risk del(5q) MDS. Leukemia (2014) 28:1033–40. doi: 10.1038/leu.2013.305
11. Franchi L, Warner N, Viani K, Nuñez G. Function of Nod-like receptors in microbial recognition and host defense. Immunol Rev. (2009) 227:106–28. doi: 10.1111/j.1600-065X.2008.00734.x
12. Awad F, Assrawi E, Louvrier C, Jumeau C, Georgin-Lavialle S, Grateau G, et al. Inflammasome biology, molecular pathology and therapeutic implications. Pharmacol Ther. (2018) 187:133–49. doi: 10.1016/j.pharmthera.2018.02.011
13. Hafner-Bratkovič I, Pelegrín P. Ion homeostasis and ion channels in NLRP3 inflammasome activation and regulation. Curr Opin Immunol. (2018) 52:8–17. doi: 10.1016/j.coi.2018.03.010
14. Basiorka A, McGraw K, Eksioglu E, Chen X, Johnson J, Padron E, et al. 32 Activation of redox-sensitive inflammasomes underlies the biological phenotype of myelodysplastic syndromes. Leuk Res. (2015) 39:S14. doi: 10.1016/S0145-2126(15)30033-3
15. Basiorka AA, McGraw KL, Eksioglu EA, Chen X, Johnson J, Zhang L, et al. The NLRP3 inflammasome functions as a driver of the myelodysplastic syndrome phenotype. Blood (2016) 128:2960–75. doi: 10.1182/blood-2016-07-730556
16. Sallman DA, Cluzeau T, Basiorka AA, List A. Unraveling the Pathogenesis of MDS: the NLRP3 inflammasome and pyroptosis drive the MDS phenotype. Front Oncol. (2016) 6:151. doi: 10.3389/fonc.2016.00151
17. Marshall DG, Gee FW. On the use of methylene blue in malarial fevers. Indian Med Gazette (1893) 28:409–10.
18. Fall B, Madamet M, Diawara S, Briolant S, Wade KA, Lo G, et al. Ex vivo activity of Proveblue, a methylene blue, against field isolates of Plasmodium falciparum in Dakar, Senegal from 2013-2015. Int J Antimicrob Agents (2017) 50:155–58. doi: 10.1016/j.ijantimicag.2017.03.019
19. Dicko A, Roh ME, Diawara H, Mahamar A, Soumare HM, Lanke K, et al. Efficacy and safety of primaquine and methylene blue for prevention of Plasmodium falciparum transmission in Mali: a phase 2, single-blind, randomised controlled trial. Lancet Infect Dis. (2018) 18:627–39. doi: 10.1016/S1473-3099(18)30044-6
20. Tucker D, Lu Y, Zhang Q. From Mitochondrial Function to Neuroprotection-an Emerging Role for Methylene Blue. Mol Neurobiol. (2018) 55:5137–53. doi: 10.1007/s12035-017-0712-2
21. Grauman Neander N, Loner CA, Rotoli JM. The acute treatment of methemoglobinemia in pregnancy. J Emerg Med. (2018) 54:685–9. doi: 10.1016/j.jemermed.2018.01.038
22. McCartney SL, Duce L, Ghadimi K. Intraoperative vasoplegia: methylene blue to the rescue! Curr Opin Anaesthesiol. (2018) 31:43–9. doi: 10.1097/ACO.0000000000000548
23. Liu H, Yu L, Yang L, Green MS. Vasoplegic syndrome: an update on perioperative considerations. J Clin Anesth. (2017) 40:63–71. doi: 10.1016/j.jclinane.2017.04.017
24. Alda M, McKinnon M, Blagdon R, Garnham J, MacLellan S, O'Donovan C, et al. Methylene blue treatment for residual symptoms of bipolar disorder: randomised crossover study. Br J Psychiatry (2017) 210:54–60. doi: 10.1192/bjp.bp.115.173930
25. Yang SH, Li W, Sumien N, Forster M, Simpkins JW, Liu R. Alternative mitochondrial electron transfer for the treatment of neurodegenerative diseases and cancers: methylene blue connects the dots. Prog Neurobiol. (2017) 157:273–91. doi: 10.1016/j.pneurobio.2015.10.005
26. Ahn H, Kang SG, Yoon SI, Ko HJ, Kim PH, Hong EJ, et al. Methylene blue inhibits NLRP3, NLRC4, AIM2, and non-canonical inflammasome activation. Sci Rep. (2017) 7:12409. doi: 10.1038/s41598-017-12635-6
27. Lin ZH, Wang SY, Chen LL, Zhuang JY, Ke QF, Xiao DR, et al. Methylene blue mitigates acute neuroinflammation after spinal cord injury through inhibiting NLRP3 inflammasome activation in microglia. Front Cell Neurosci. (2017) 11:391. doi: 10.3389/fncel.2017.00391
28. Hao J, Zhang H, Yu J, Chen X, Yang L. Methylene blue attenuates diabetic retinopathy by inhibiting NLRP3 inflammasome activation in STZ-induced diabetic rats. Ocul Immunol Inflamm. (2018). doi: 10.1080/09273948.2018.1450516 [Epub ahead of print].
29. Dormoi J, Briolant S, Desgrouas C, Pradines B. Efficacy of proveblue (methylene blue) in an experimental cerebral malaria murine model. Antimicrob Agents Chemother. (2013) 57:3412–4. doi: 10.1128/AAC.02381-12
30. Dormoi J, Pradines B. Dose responses of proveblue methylene blue in an experimental murine cerebral malaria model. Antimicrob Agents Chemother. (2013) 57:4080–1. doi: 10.1128/AAC.00634-13
31. Kast RE. Minocycline in cerebral malaria. J Neurosci Res. (2008) 86:3257. doi: 10.1002/jnr.21791
32. Apoorv TS, Babu PP. Minocycline prevents cerebral malaria, confers neuroprotection and increases survivability of mice during Plasmodium berghei ANKA infection. Cytokine (2017) 90:113–23. doi: 10.1016/j.cyto.2016.11.001
33. Speer K, Upton D, Semple S, McKune A. Systemic low-grade inflammation in post-traumatic stress disorder: a systematic review. J Inflamm Res. (2018) 11:111–21. doi: 10.2147/JIR.S155903
34. Preston G, Kirdar F, Kozicz T. The role of suboptimal mitochondrial function in vulnerability to post-traumatic stress disorder. J Inherit Metab Dis. (2018) 41:585–96. doi: 10.1007/s10545-018-0168-1
35. Zoellner LA, Telch M, Foa EB, Farach FJ, McLean CP, Gallop R, et al. Enhancing extinction learning in posttraumatic stress disorder with brief daily imaginal exposure and methylene blue: a randomized controlled trial. J Clin Psychiatry (2017) 78:e782–9. doi: 10.4088/JCP.16m10936
Keywords: bone marrow, inflammasome, innate immune response, methylene blue, myelodysplasia, pyroptosis
Citation: Kast RE (2018) Inhibiting the NLRP3 Inflammasome With Methylene Blue as Treatment Adjunct in Myelodysplasia. Front. Oncol. 8:280. doi: 10.3389/fonc.2018.00280
Received: 22 April 2018; Accepted: 04 July 2018;
Published: 27 July 2018.
Edited by:
Sara Galimberti, Università degli Studi di Pisa, ItalyReviewed by:
Luca Maurillo, Università degli Studi di Roma Tor Vergata, ItalyCopyright © 2018 Kast. This is an open-access article distributed under the terms of the Creative Commons Attribution License (CC BY). The use, distribution or reproduction in other forums is permitted, provided the original author(s) and the copyright owner(s) are credited and that the original publication in this journal is cited, in accordance with accepted academic practice. No use, distribution or reproduction is permitted which does not comply with these terms.
*Correspondence: Richard E. Kast, cmljaGFyZGVyaWNrYXN0QGdtYWlsLmNvbQ==
Disclaimer: All claims expressed in this article are solely those of the authors and do not necessarily represent those of their affiliated organizations, or those of the publisher, the editors and the reviewers. Any product that may be evaluated in this article or claim that may be made by its manufacturer is not guaranteed or endorsed by the publisher.
Research integrity at Frontiers
Learn more about the work of our research integrity team to safeguard the quality of each article we publish.