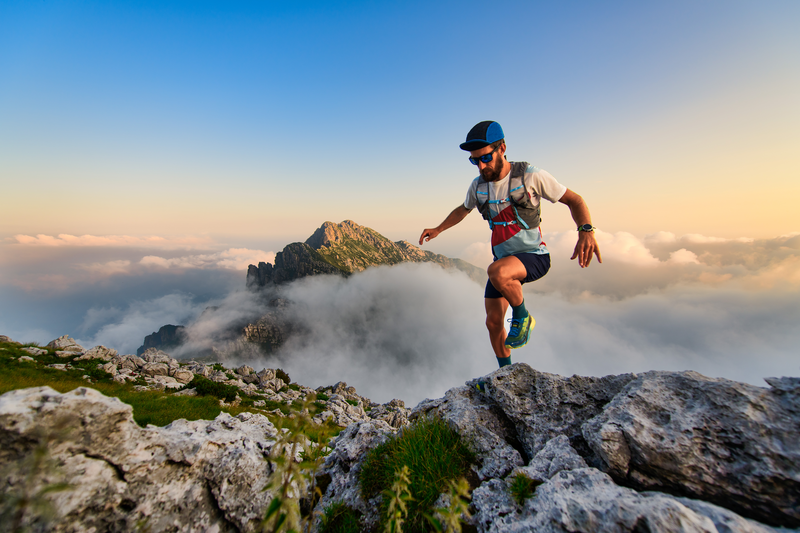
94% of researchers rate our articles as excellent or good
Learn more about the work of our research integrity team to safeguard the quality of each article we publish.
Find out more
CASE REPORT article
Front. Oncol. , 07 October 2016
Sec. Pharmacology of Anti-Cancer Drugs
Volume 6 - 2016 | https://doi.org/10.3389/fonc.2016.00204
This article is part of the Research Topic Adverse Effects of Cancer Chemotherapy: Anything New to Improve Tolerance and Reduce Sequelae? View all 20 articles
A 52-year-old male patient was treated with standard radiochemotherapy with temozolomide for glioblastoma multiforme (GBM). After worsening of his clinical condition, further tumor-specific treatment was unlikely to be successful, and the patient seeked help from an alternative practitioner, who administered a combination of dichloroacetate (DCA) and artesunate (ART). A few days later, the patient showed clinical and laboratory signs of liver damage and bone marrow toxicity (leukopenia, thrombocytopenia). Despite successful restoration of laboratory parameters upon symptomatic treatment, the patient died 10 days after the infusion. DCA bears a well-documented hepatotoxic risk, while ART can be considered as safe concerning hepatotoxicity. Bone marrow toxicity can appear upon ART application as reduced reticulocyte counts and disturbed erythropoiesis. It can be assumed that the simultaneous use of both drugs caused liver injury and bone marrow toxicity. The compassionate use of DCA/ART combination therapy outside of clinical trials cannot be recommended for GBM treatment.
Glioblastoma multiforme (GBM) is an aggressive brain tumor that is currently treated with a combination of radiotherapy and temozolomide (TMZ) chemotherapy. The prognosis is unfavorable with an average survival of 15 months (1–3). In this desperate situation, it is not uncommon for patients to seek help outside standard medicine from alternative practitioners and healers. Often, non-approved remedies or unproven combination of drugs are prescribed, which occasionally may lead to undesired side effects or even life-threatening toxicities.
Dichloroacetate (DCA) is generated as by-product of chlorination of drinking water and by metabolitzation of drugs and chemicals (4). DCA accumulation in groundwater is considered as potential health hazard. In vitro and in vivo investigations showed that DCA inhibits tumor growth by redirecting glycolysis to oxidative phosphorylation and oxidative removal of lactate via pyruvate (5). Although five GBM patients have been previously treated with DCA (6), there is only limited knowledge about the efficacy or toxicity of DCA in cancer therapy.
In addition to their antimalarial activity, the artemisinin (ARS) derivatives [artesunate (ART), artemether, dehydroartemisinin] also exert anticancer activity in vitro and in vivo (7–13), including some brain tumor models (14–18). Compassionate use of ARS-type drugs encouraged the initiation of phase I/II trials in cancer patients (19–27). Most of these studies report are case reports or consist of only small numbers of patients. Therefore, there is still limited evidence regarding the safe use of ARS in cancer patients.
In the present case report, we describe a patient, who died with severe liver and bone marrow toxicity after intake of combined DCA and ART.
A 52-year-old male patient was diagnosed with GBM after suffering for several weeks from cognitive decline, headaches, gait ataxia, and a series of epileptic seizures. The initiation of adjuvant therapy was delayed by complicated wound healing, but finally – 53 days after surgery – radiotherapy up to 60 Gy of the tumor region was initiated with simultaneous TMZ chemotherapy (75 mg/m2) according to local guidelines (28).
The general state of health was unfavorable (Karnofsky score: 50). The patient suffered from right-side hemiparesis and required considerable help and medical assistance. Therefore, adjuvant TMZ chemotherapy was ruled out, and rehabilitation actions were initiated. Rehabilitation had to be discontinued 128 days after surgery, because of another series of epileptic seizures. Antiepileptic treatment was escalated to 1800 mg valproic acid (VA), 3000 mg levetiracetam, 200 mg lacosamide, and 20 mg clobazam. Progressive intracranial tumor burden by CT and Fet-PET scan diagnosis was considered as non-suitable for tumor-specific treatment, and steroid medication was escalated.
At that point, the patient and his family were seeking help from an alternative practitioner. An unknown amount of DCA was administered and ART (2.5 mg/kg bodyweight) was intravenously infused 148 days after surgery. At that time, the patient had a stable/unchanged concomitant medication. The patient’s cognitive condition declined during the following days with adynamia, severe headaches, and psychomotoric retardation in rapid change with signs of delusions. After admission to the hospital, epileptic activity was not found by EEG and CT scanning did not show relevant changes concerning mass effect or edema. However, blood examinations showed signs of exsiccosis, pancytopenia, and markedly increased hepatic enzyme activities (Figure 1). Upon fluid substitution, laboratory parameter stabilized. However, two days after hospitalization, the state of the patient suddenly deteriorated with hypotension, systemic signs of infection, and a series of epileptic seizures. Discussing the need for intensified medical intervention and possible mechanical ventilation, the family did not wish these the actions to be undertaken according to the patient’s provision. The patient died during the course of the following night and 157 days after surgery.
Figure 1. Time course of leukocyte (A), thrombocyte (B) count, serum levels of ALAT (C), ASAT (D), g-GT (E), and CRP (F). Radiotherapy with Temozolomide as indicated between 53 and 92 days after surgery. Infusion with ART and DCA is labeled 148 days after surgery.
The timing of events can be summarized as follows:
• Surgery at day 0
• Start of radiotherapy 53 days after surgery
• End of radiotherapy 92 days after surgery
• Infusion of ART and DCA 148 days after surgery
• First signs of toxicity 154 days after surgery (elevated liver enzymes and hematotoxicity)
• Death of the patient 157 days after surgery
A valuable measure for the causality of adverse reactions of drugs in patients with liver injury is the Roussel Uclaf Causality Assessment Method (RUCAM) (29, 30). RUCAM considers all relevant criteria for liver injury by drugs. We applied the RUCAM scoring system to the patient presented here and found an overall quantitative grading of causality of 6, which indicates reasonable probability that the combinational administration of DCA and ART caused liver injury (Table 1).
Table 1. Causality assessment of adverse reactions to the DCA/ART combination treatment according to RUCAM (29, 30).
The severity and outcome of this case of compassionate use of alternative medication is remarkable. While the hepatotoxic potential of DCA is well documented, ART is actually considered a rather safe antimalarial drug. It can be speculated that the specific combination of both drugs provoked fatal liver and bone marrow toxicity in the patient.
At the day of hospitalization, prior alternative medication had not been declared by the patient. Therefore, liver toxicity by VA or TMZ has been suspected. In the past, severe and even fatal toxicity were reported for both for VA (31–36) and for TMZ (37–40). Taking into account the additional sudden decline in leukocyte and thrombocyte counts during the next days and considering the prior normal values made this possibility, however, rather unlikely. The dynamics of TMZ- or VA-caused liver damage usually represent more continuous processes. The nadir of TMZ is expected after 21 days. Even delayed forms of bone marrow toxicity are not comparable to the dramatic decline observed here.
The cause of death remains speculative, since an autopsy was not performed in accordance to the patient’s provision and family wishes. We consider aspiration pneumonia or spontaneous internal bleeding as possible causes for the sudden decline of blood pressure.
As shown in Table 2, DCA administration in animal experiments induced hepatotoxicity and hepatocarcinogenesis. DCA increased hepatic oxidative stress and disturbed liver metabolism. Although treatment of five GBM patients with DCA did not reveal hepatotoxicity (6), there is evidence from preclinical in vivo experiments that DCA affects the liver (Table 2) (4, 41). However, a straightforward conclusion to the observed hepatotoxicity in the present case is difficult, because the dose of applied DCA to the patient was not disclosed by the alternative practitioner.
The clinical safety of ART is well documented. Large clinical trials and meta-analyses of clinical trials dealing with many thousands of malaria patients did not unravel serious adverse effects (59, 60). Preclinical toxicity studies gave some hints for neurotoxicity, embryotoxicity, genotoxicity, hematotoxicity, cardiotoxicity, nephrotoxicity, and allergic reaction (61). Long-term application of low ARS concentrations may be more toxic than short-term application of high doses. This may explain, why toxicities can be observed in animal experiments, but not in human studies. A large meta-analysis with 5000 malaria patients revealed that hepatotoxicity was a rare event, and elevated liver enzymes have been found in 0.9% of all cases (59). Although most papers on clinical safety were published in the context of malaria treatment, there are also some reports on the use of ARS-derivatives in cancer patients. Case reports on the compassionate use of ART or artemether in patients, with laryngeal squamous cell carcinoma, uveal melanoma, pituitary macroadenoma, and prostate carcinoma, reported that the ARSs were well tolerated with no additional side effects in addition to those caused by standard chemotherapy. A randomized controlled trial with 120 advanced non-small cell lung cancer patients on vinorelbine alone versus vinorelbine plus ART did not find significant differences in toxicity between the two treatment groups (23). In a pilot phase I/II trial in 10 patients suffering from cervical carcinoma, artenimol reduced clinical symptoms, vaginal discharge, and pain, and no adverse events of grade 3 and 4 were observed (24). Another phase I/II pilot study in veterinary cancers was conducted in 23 dogs with non-resectable tumors. No neurological or cardiac toxicity was observed, and seven dogs exhibited no adverse effects at all. Fever and hematological or gastrointestinal toxicity, mostly transient, occurred in 16 dogs. One dog died from treatment-unrelated pneumonia (25). As reported from a randomized, double-blind placebo-controlled pilot study in 23 colorectal cancer patients, oral ART therapy was well tolerated without signs of hepatotoxicity (26). Another recent phase I trial on 23 metastasized breast cancer patients reported that four patients had adverse events of the auditory system possibly related to the intake of ART. However, none of these side effects were severe adverse events. Four patients had adverse events concerning the vestibular system, one of which was severe, but fully reversible after discontinuation of ART treatment (27). In summary, hepatotoxicity has not been found in any of these patients.
Hematotoxicity is worth mentioning in this context, because the patient suffered from reduced leukocyte and thrombocyte counts. The toxicity of ARS-type drugs on leukopoiesis is controversially discussed, and both enhanced and inhibited leukocyte functions have been observed (61). Dihydroartemisinin ameliorated inflammatory disease (62). However, ARS-derivatives exhibited higher cytotoxicity in vitro toward hematopoietic progenitor cells of the granulocyte-monocyte lineage (CFU-GM) than toward cancer cells (63), indicating that myelosuppression might be an issue in cancer therapy. While thrombocytopenia was apparently not relevant, damage of erythrocytes occurred in animal experiments (61). A sensitive measure for erythropoiesis is the blood count of reticulocytes in peripheral blood. Reduced reticulocyte counts (as erythrocyte precursors) have not only been observed in vitro and in animals, but also in human patients upon treatment with ARS-type drugs (59, 61, 64, 65).
In conclusion, the presented case illustrates the possible consequences of compassionate use of non-approved drugs or unproven drug combinations. Drug therapy should always be in accordance to the guidelines of good clinical practice.
MU and SS: treated the patient. TE: wrote the paper.
The authors declare that the research was conducted in the absence of any commercial or financial relationships that could be construed as a potential conflict of interest.
ARS, artemisinin; ART, artesunate; DCA, dichloroacetate; GBM, glioblastoma multiforme; TMZ, temozolomide; VA, valproic acid.
1. Stupp R, Hegi ME, Mason WP, van den Bent MJ, Taphoorn MJ, Janzer RC, et al. Effects of radiotherapy with concomitant and adjuvant temozolomide versus radiotherapy alone on survival in glioblastoma in a randomised phase III study: 5-year analysis of the EORTC-NCIC trial. Lancet Oncol (2009) 10(5):459–66. doi:10.1016/S1470-2045(09)70025-7
2. Woehrer A, Bauchet L, Barnholtz-Sloan JS. Glioblastoma survival: has it improved? Evidence from population-based studies. Curr Opin Neurol (2014) 27(6):666–74. doi:10.1097/WCO.0000000000000144
3. Yang LJ, Zhou CF, Lin ZX. Temozolomide and radiotherapy for newly diagnosed glioblastoma multiforme: a systematic review. Cancer Invest (2014) 32(2):31–6. doi:10.3109/07357907.2013.861474
4. Stacpoole PW, Henderson GN, Yan Z, James MO. Clinical pharmacology and toxicology of dichloroacetate. Environ Health Perspect (1998) 106(Suppl 4):989–94. doi:10.1289/ehp.98106s4989
5. Kankotia S, Stacpoole PW. Dichloroacetate and cancer: new home for an orphan drug? Biochim Biophys Acta (2014) 1846(2):617–29. doi:10.1016/j.bbcan.2014.08.005
6. Michelakis ED, Sutendra G, Dromparis P, Webster L, Haromy A, Niven E, et al. Metabolic modulation of glioblastoma with dichloroacetate. Sci Transl Med (2010) 2(31):31ra34. doi:10.1126/scitranslmed.3000677
7. Moore JC, Lai H, Li JR, Ren RL, McDougall JA, Singh NP, et al. Oral administration of dihydroartemisinin and ferrous sulfate retarded implanted fibrosarcoma growth in the rat. Cancer Lett (1995) 98(1):83–7. doi:10.1016/0304-3835(95)03999-D
8. Efferth T, Rücker G, Falkenberg M, Manns D, Olbrich A, Fabry U, et al. Detection of apoptosis in KG-1a leukemic cells treated with investigational drugs. Arzneimittelforschung (1996) 46(2):196–200.
9. Efferth T, Dunstan H, Sauerbrey A, Miyachi H, Chitambar CR. The anti-malarial artesunate is also active against cancer. Int J Oncol (2001) 18(4):767–73.
10. Efferth T, Olbrich A, Bauer R. mRNA expression profiles for the response of human tumor cell lines to the antimalarial drugs artesunate, arteether, and artemether. Biochem Pharmacol (2002) 64(4):617–23. doi:10.1016/S0006-2952(02)01221-2
11. Efferth T, Sauerbrey A, Olbrich A, Gebhart E, Rauch P, Weber HO, et al. Molecular modes of action of artesunate in tumor cell lines. Mol Pharmacol (2003) 64(2):382–94. doi:10.1124/mol.64.2.382
12. Dell’Eva R, Pfeffer U, Vene R, Anfosso L, Forlani A, Albini A, et al. Inhibition of angiogenesis in vivo and growth of Kaposi’s sarcoma xenograft tumors by the anti-malarial artesunate. Biochem Pharmacol (2004) 68(12):2359–66. doi:10.1016/j.bcp.2004.08.021
13. Disbrow GL, Baege AC, Kierpiec KA, Yuan H, Centeno JA, Thibodeaux CA, et al. Dihydroartemisinin is cytotoxic to papillomavirus-expressing epithelial cells in vitro and in vivo. Cancer Res (2005) 65(23):10854–61. doi:10.1158/0008-5472.CAN-05-1216
14. Efferth T, Ramirez T, Gebhart E, Halatsch ME. Combination treatment of glioblastoma multiforme cell lines with the anti-malarial artesunate and the epidermal growth factor receptor tyrosine kinase inhibitor OSI-774. Biochem Pharmacol (2004) 67(9):1689–700. doi:10.1016/j.bcp.2003.12.035
15. Huang XJ, Ma ZQ, Zhang WP, Lu YB, Wei EQ. Dihydroartemisinin exerts cytotoxic effects and inhibits hypoxia inducible factor-1alpha activation in C6 glioma cells. J Pharm Pharmacol (2007) 59(6):849–56. doi:10.1211/jpp.59.6.0011
16. Wu ZP, Gao CW, Wu YG, Zhu QS, Yan C, Xin L, et al. Inhibitive effect of artemether on tumor growth and angiogenesis in the rat C6 orthotopic brain gliomas model. Integr Cancer Ther (2009) 8(1):88–92. doi:10.1177/1534735408330714
17. Berdelle N, Nikolova T, Quiros S, Efferth T, Kaina B. Artesunate induces oxidative DNA damage, sustained DNA double-strand breaks, and the ATM/ATR damage response in cancer cells. Mol Cancer Ther (2011) 10(12):2224–33. doi:10.1158/1535-7163.MCT-11-0534
18. Chen J, Chen X, Wang F, Gao H, Hu W. Dihydroartemisinin suppresses glioma proliferation and invasion via inhibition of the ADAM17 pathway. Neurol Sci (2015) 36(3):435–40. doi:10.1007/s10072-014-1963-6
19. Singh NP, Verma KB. Case report of a laryngeal squamous cell carcinoma treated with artesunate. Arch Oncol (2002) 10:279–80. doi:10.2298/AOO0204279S
20. Berger TG, Dieckmann D, Efferth T, Schultz ES, Funk JO, Baur A, et al. Artesunate in the treatment of metastatic uveal melanoma – first experiences. Oncol Rep (2005) 14(6):1599–603.
21. Singh NP, Panwar VK. Case report of a pituitary macroadenoma treated with artemether. Integr Cancer Ther (2006) 5:391–4. doi:10.1177/1534735406295311
22. Michaelsen FW, Saeed ME, Schwarzkopf J, Efferth T. Activity of Artemisia annua and artemisinin derivatives, in prostate carcinoma. Phytomedicine (2015) 22(14):1223–31. doi:10.1016/j.phymed.2015.11.001
23. Zhang ZY, Yu SQ, Miao LY, Huang XY, Zhang XP, Zhu YP, et al. [Artesunate combined with vinorelbine plus cisplatin in treatment of advanced non-small cell lung cancer: a randomized controlled trial]. Zhong Xi Yi Jie He Xue Bao (2008) 6(2):134–8. doi:10.3736/jcim20080206
24. Jansen FH, Adoubi I, Kouassi JC, DE C, Jansen N, Tschulakow A, et al. First study of oral Artenimol-R in advanced cervical cancer: clinical benefit, tolerability and tumor markers. Anticancer Res (2011) 31(12):4417–22.
25. Rutteman GR, Erich SA, Mol JA, Spee B, Grinwis GC, Fleckenstein L, et al. Safety and efficacy field study of artesunate for dogs with non-resectable tumours. Anticancer Res (2013) 33(5):1819–27.
26. Krishna S, Ganapathi S, Ster IC, Saeed ME, Cowan M, Finlayson C, et al. A randomised, double blind, placebo-controlled pilot study of oral artesunate therapy for colorectal cancer. EBioMedicine (2015) 2(1):82–90. doi:10.1016/j.ebiom.2014.11.010
27. Konig M, von Hagens C, Hoth S, Baumann I, Walter-Sack I, Edler L, et al. Investigation of ototoxicity of artesunate as add-on therapy in patients with metastatic or locally advanced breast cancer: new audiological results from a prospective, open, uncontrolled, monocentric phase I study. Cancer Chemother Pharmacol (2016) 77(2):413–27. doi:10.1007/s00280-016-2960-7
28. Weller M, van den Bent M, Hopkins K, Tonn JC, Stupp R, Falini A, et al. EANO guideline for the diagnosis and treatment of anaplastic gliomas and glioblastoma. Lancet Oncol (2014) 15(9):e395–403. doi:10.1016/S1470-2045(14)70011-7
29. Danan G, Benichou C. Causality assessment of adverse reactions to drugs – I. A novel method based on the conclusions of international consensus meetings: application to drug-induced liver injuries. J Clin Epidemiol (1993) 46(11):1323–30. doi:10.1016/0895-4356(93)90101-6
30. Teschke RLD, Melchart D, Danan G. Traditional Chinese Medicine (TCM) and herbal hepatotoxicity: RUCAM and the role of novel diagnostic biomarkers such as MicroRNAs. Medicines (2016) 3(3):18. doi:10.3390/medicines3030018
31. Hjelm M, de Silva LV, Seakins JW, Oberholzer VG, Rolles CJ. Evidence of inherited urea cycle defect in a case of fatal valproate toxicity. Br Med J (Clin Res Ed) (1986) 292(6512):23–4. doi:10.1136/bmj.292.6512.23
32. Evans RJ, Miranda RN, Jordan J, Krolikowski FJ. Fatal acute pancreatitis caused by valproic acid. Am J Forensic Med Pathol (1995) 16(1):62–5. doi:10.1097/00000433-199503000-00014
33. Pinkston R, Walker LA. Multiorgan system failure caused by valproic acid toxicity. Am J Emerg Med (1997) 15(5):504–6. doi:10.1016/S0735-6757(97)90195-9
34. Acharya S, Bussel JB. Hematologic toxicity of sodium valproate. J Pediatr Hematol Oncol (2000) 22(1):62–5. doi:10.1097/00043426-200001000-00012
35. Pronicka E, Weglewska-Jurkiewicz A, Pronicki M, Sykut-Cegielska J, Kowalski P, Pajdowska M, et al. Drug-resistant epilepsia and fulminant valproate liver toxicity. Alpers-Huttenlocher syndrome in two children confirmed post mortem by identification of p.W748S mutation in POLG gene. Med Sci Monit (2011) 17(4):CR203–9. doi:10.12659/MSM.881716
36. Star K, Edwards IR, Choonara I. Valproic acid and fatalities in children: a review of individual case safety reports in VigiBase. PLoS One (2014) 9(10):e108970. doi:10.1371/journal.pone.0108970
37. Su YW, Chang MC, Chiang MF, Hsieh RK. Treatment-related myelodysplastic syndrome after temozolomide for recurrent high-grade glioma. J Neurooncol (2005) 71(3):315–8. doi:10.1007/s11060-004-2028-0
38. George BJ, Eichinger JB, Richard TJ. A rare case of aplastic anemia caused by temozolomide. South Med J (2009) 102(9):974–6. doi:10.1097/SMJ.0b013e3181b1d2fa
39. Letarte N, Gabay MP, Bressler LR, Long KE, Stachnik JM, Villano JL. Analyzing temozolomide medication errors: potentially fatal. J Neurooncol (2014) 120(1):111–5. doi:10.1007/s11060-014-1523-1
40. Grieco A, Tafuri MA, Biolato M, Diletto B, Di Napoli N, Balducci N, et al. Severe cholestatic hepatitis due to temozolomide: an adverse drug effect to keep in mind. Case report and review of literature. Medicine (Baltimore) (2015) 94(12):e476. doi:10.1097/MD.0000000000000476
41. Bull RJ. Mode of action of liver tumor induction by trichloroethylene and its metabolites, trichloroacetate and dichloroacetate. Environ Health Perspect (2000) 108(Suppl 2):241–59. doi:10.1289/ehp.00108s2241
42. Graf H, Leach W, Arieff AI. Effects of dichloroacetate in the treatment of hypoxic lactic acidosis in dogs. J Clin Invest (1985) 76(3):919–23. doi:10.1172/JCI112090
43. Bull RJ, Sanchez IM, Nelson MA, Larson JL, Lansing AJ. Liver tumor induction in B6C3F1 mice by dichloroacetate and trichloroacetate. Toxicology (1990) 63(3):341–59. doi:10.1016/0300-483X(90)90195-M
44. Sanchez IM, Bull RJ. Early induction of reparative hyperplasia in the liver of B6C3F1 mice treated with dichloroacetate and trichloroacetate. Toxicology (1990) 64(1):33–46. doi:10.1016/0300-483X(90)90097-Z
45. Bull RJ, Templin M, Larson JL, Stevens DK. The role of dichloroacetate in the hepatocarcinogenicity of trichloroethylene. Toxicol Lett (1993) 68(1–2):203–11. doi:10.1016/0378-4274(93)90131-G
46. Stauber AJ, Bull RJ. Differences in phenotype and cell replicative behavior of hepatic tumors induced by dichloroacetate (DCA) and trichloroacetate (TCA). Toxicol Appl Pharmacol (1997) 144(2):235–46. doi:10.1006/taap.1997.8159
47. Stauber AJ, Bull RJ, Thrall BD. Dichloroacetate and trichloroacetate promote clonal expansion of anchorage-independent hepatocytes in vivo and in vitro. Toxicol Appl Pharmacol (1998) 150(2):287–94. doi:10.1006/taap.1998.8417
48. Miller JH, Minard K, Wind RA, Orner GA, Sasser LB, Bull RJ. In vivo MRI measurements of tumor growth induced by dichloroacetate: implications for mode of action. Toxicology (2000) 145(2–3):115–25. doi:10.1016/S0300-483X(00)00148-7
49. Kato-Weinstein J, Stauber AJ, Orner GA, Thrall BD, Bull RJ. Differential effects of dihalogenated and trihalogenated acetates in the liver of B6C3F1 mice. J Appl Toxicol (2001) 21(2):81–9. doi:10.1002/jat.717
50. Lingohr MK, Thrall BD, Bull RJ. Effects of dichloroacetate (DCA) on serum insulin levels and insulin-controlled signaling proteins in livers of male B6C3F1 mice. Toxicol Sci (2001) 59(1):178–84. doi:10.1093/toxsci/59.1.178
51. Bull RJ, Orner GA, Cheng RS, Stillwell L, Stauber AJ, Sasser LB, et al. Contribution of dichloroacetate and trichloroacetate to liver tumor induction in mice by trichloroethylene. Toxicol Appl Pharmacol (2002) 182(1):55–65. doi:10.1006/taap.2002.9427
52. Saghir SA, Schultz IR. Low-dose pharmacokinetics and oral bioavailability of dichloroacetate in naive and GST-zeta-depleted rats. Environ Health Perspect (2002) 110(8):757–63. doi:10.1289/ehp.02110757
53. Guo X, Dixit V, Liu H, Shroads AL, Henderson GN, James MO, et al. Inhibition and recovery of rat hepatic glutathione S-transferase zeta and alteration of tyrosine metabolism following dichloroacetate exposure and withdrawal. Drug Metab Dispos (2006) 34(1):36–42. doi:10.1124/dmd.105.003996
54. Hassoun EA, Dey S. Dichloroacetate- and trichloroacetate-induced phagocytic activation and production of oxidative stress in the hepatic tissues of mice after acute exposure. J Biochem Mol Toxicol (2008) 22(1):27–34. doi:10.1002/jbt.20210
55. Hassoun EA, Cearfoss J, Spildener J. Dichloroacetate- and trichloroacetate-induced oxidative stress in the hepatic tissues of mice after long-term exposure. J Appl Toxicol (2010) 30(5):450–6. doi:10.1002/jat.1516
56. Li W, James MO, McKenzie SC, Calcutt NA, Liu C, Stacpoole PW. Mitochondrion as a novel site of dichloroacetate biotransformation by glutathione transferase zeta 1. J Pharmacol Exp Ther (2011) 336(1):87–94. doi:10.1124/jpet.110.173195
57. Gattone VH II, Bacallao RL. Dichloroacetate treatment accelerates the development of pathology in rodent autosomal recessive polycystic kidney disease. Am J Physiol Renal Physiol (2014) 307(10):F1144–8. doi:10.1152/ajprenal.00009.2014
58. Hassoun E, Cearfoss J, Mamada S, Al-Hassan N, Brown M, Heimberger K, et al. The effects of mixtures of dichloroacetate and trichloroacetate on induction of oxidative stress in livers of mice after subchronic exposure. J Toxicol Environ Health A (2014) 77(6):313–23. doi:10.1080/15287394.2013.864576
59. Ribeiro IR, Olliaro P. Safety of artemisinin and its derivatives. A review of published and unpublished clinical trials. Med Trop (Mars) (1998) 58(3 Suppl):50–3.
60. Adjuik M, Babiker A, Garner P, Olliaro P, Taylor W, White N. Artesunate combinations for treatment of malaria: meta-analysis. Lancet (2004) 363(9402):9–17. doi:10.1016/S0140-6736(03)15162-8
61. Efferth T, Kaina B. Toxicity of the antimalarial artemisinin and its dervatives. Crit Rev Toxicol (2010) 40(5):405–21. doi:10.3109/10408441003610571
62. Zhao YG, Wang Y, Guo Z, Gu AD, Dan HC, Baldwin AS, et al. Dihydroartemisinin ameliorates inflammatory disease by its reciprocal effects on Th and regulatory T cell function via modulating the mammalian target of rapamycin pathway. J Immunol (2012) 189(9):4417–25. doi:10.4049/jimmunol.1200919
63. Beekman AC, Wierenga PK, Woerdenbag HJ, Van Uden W, Pras N, Konings AW, et al. Artemisinin-derived sesquiterpene lactones as potential antitumour compounds: cytotoxic action against bone marrow and tumour cells. Planta Med (1998) 64(7):615–9. doi:10.1055/s-2006-957533
64. Wootton DG, Opara H, Biagini GA, Kanjala MK, Duparc S, Kirby PL, et al. Open-label comparative clinical study of chlorproguanil-dapsone fixed dose combination (Lapdap) alone or with three different doses of artesunate for uncomplicated Plasmodium falciparum malaria. PLoS One (2008) 3(3):e1779. doi:10.1371/journal.pone.0001779
Keywords: adverse side effects, cancer, chemotherapy, toxicology
Citation: Uhl M, Schwab S and Efferth T (2016) Fatal Liver and Bone Marrow Toxicity by Combination Treatment of Dichloroacetate and Artesunate in a Glioblastoma Multiforme Patient: Case Report and Review of the Literature. Front. Oncol. 6:204. doi: 10.3389/fonc.2016.00204
Received: 17 April 2016; Accepted: 09 September 2016;
Published: 07 October 2016
Edited by:
Raquel Abalo, King Juan Carlos University, SpainReviewed by:
Janet K. Coller, University of Adelaide, AustraliaCopyright: © 2016 Uhl, Schwab and Efferth. This is an open-access article distributed under the terms of the Creative Commons Attribution License (CC BY). The use, distribution or reproduction in other forums is permitted, provided the original author(s) or licensor are credited and that the original publication in this journal is cited, in accordance with accepted academic practice. No use, distribution or reproduction is permitted which does not comply with these terms.
*Correspondence: Thomas Efferth, ZWZmZXJ0aEB1bmktbWFpbnouZGU=
Disclaimer: All claims expressed in this article are solely those of the authors and do not necessarily represent those of their affiliated organizations, or those of the publisher, the editors and the reviewers. Any product that may be evaluated in this article or claim that may be made by its manufacturer is not guaranteed or endorsed by the publisher.
Research integrity at Frontiers
Learn more about the work of our research integrity team to safeguard the quality of each article we publish.