- 1School of Surgery, The University of Western Australia, Perth, WA, Australia
- 2Medical Oncology, Royal Perth Hospital, Perth, WA, Australia
- 3Department of Medical Oncology, St Vincent’s Hospital Melbourne, Melbourne, VIC, Australia
- 4Institute of Health and Biomedical Innovation, School of Biomedical Sciences, Queensland University of Technology, Brisbane, QLD, Australia
- 5St. Vincent’s Institute, Melbourne, VIC, Australia
- 6Department of Surgery, St Vincent’s Hospital, University of Melbourne, Melbourne, VIC, Australia
There is increasing interest in circulating tumor cells (CTCs) due to their purported role in breast cancer metastasis, and their potential as a “liquid biopsy” tool in breast cancer diagnosis and management. There are, however, questions with regards to the reliability and consistency of CTC detection and to the relationship between CTCs and prognosis, which is limiting their clinical utility. There is increasing acceptance that the ability of CTCs to alter from an epithelial to mesenchymal phenotype plays an important role in determining the metastatic potential of these cells. This review examines the phenotypic and genetic variation, which has been reported within CTC populations. Importantly, we discuss how the detection and characterization of CTCs provides additional and often differing information from that obtained from the primary tumor, and how this may be utilized in determining prognosis and treatment options. It has been shown for example that hormone receptor status often differs between the primary tumor and CTCs, which may help to explain failure of endocrine treatment. We examine how CTC status may introduce alternative treatment options and also how they may be used to monitor treatment. Finally, we discuss the most interesting current clinical trials involving CTC analysis and note further research that is required before the breast cancer “liquid biopsy” can be realized.
Introduction
Breast cancer is the most common cause of cancer death among women (1). Prognosis for most patients with early breast cancer (EBC) is generally very good, however, a significant proportion (20–30%) of chemotherapy-treated EBC patients relapse with metastatic disease (2). How to identify those breast cancer patients who will relapse in the future and develop metastatic disease remains elusive. Metastatic disease is initiated by circulating tumor cells (CTCs) that originate from the primary tumor and spread the cancer in the body via the blood circulatory system. These CTCs may migrate to, and remain dormant in, sites such as bone marrow as disseminated tumor cells (DTCs). After variable latency periods, DTCs may develop into overt metastases and although this is not seen in all patients, it is seen more frequently in breast cancer patients with persistent DTCs (3). Although considerable research has been conducted to characterize these cells and their role in dissemination, dormancy, and formation of metastasis, many questions remain. For example, why are CTCs not detectable in some patients with metastases, and why is it that some patients with detectable CTCs never develop metastases?
In a rat model, human mammary tumors have been shown to shed 3.2–4.1 × 106 cells per day per gram of tissue (4), most of which (~85%) are destroyed within minutes in the circulation (5) by anoikis, a form of apoptosis driven by loss of cell–cell interactions (6). However, some cells are resistant to anoikis (5). In a mouse model, approximately 2.5% of CTCs formed micrometastases (most of which subsequently disappeared over time) and 0.01% of CTCs progressed to form macrometastases (7). Metastatic potential is not only influenced by CTCs resistance to anoikis, but also the ability of CTCs to change their cellular phenotype from epithelial to mesenchymal – termed epithelial–mesenchymal plasticity (8).
Detection of either CTCs or DTCs is commonly associated with an increased risk of metastases and accompanying poor prognosis (9, 10). Researchers have, however, reported considerable variation in CTC detection rates and correlation with prognosis, even in patients with substantial metastatic disease (11). To date, this has prevented the use of CTCs as a routine prognostic clinical tool (12). We focus our review on CTCs, their role in breast cancer progression, and how CTC molecular variation and epithelial–mesenchymal transition (EMT) may explain discrepancies in CTC detection, therapy response, and relationship to prognosis.
CTC Characteristics
Circulating tumor cells are extremely rare, with a frequency of typically 1 per 106–7 leukocytes (13). Defining characteristics to delineate CTCs from other blood cells is difficult due to the substantial pleomorphism CTCs exhibit (14). Breast cancer CTCs have a mean diameter of 13.1 μm (15), which is only slightly larger than blood leukocytes measured at 10 μm (16). Accepted CTC characteristics include presence of a nucleus, visible cytoplasm, and the expression of cytokeratin and absence of CD45 expression (17).
Clusters of CTCs, also called tumor microemboli, are found in some patients, comprising 4% of CTCs analyzed in one study (14) and have been demonstrated to form prior to entering the circulation, and to be precursors with more malignant potential than their unicellular counterparts (18). Cluster presence, particularly if sustained through treatment, correlates more strongly with poor prognosis than single CTCs do in metastatic breast cancer (MBC) patients (18).
Epithelial–Mesenchymal Transition
Most breast cancers are of epithelial origin (19). Epithelial cells collectively maintain organized tissue architecture through distinct contact between cells facilitated by E-cadherin, a homotypic transmembrane cell–cell adhesion protein (20, 21). A critical step in tumor invasion and metastasis is the phenotypical change known as EMT, normally a highly regulated process involved in embryogenesis and wound healing, and implicated in several disease states including malignancy and fibrosis (22). Physiologically, activation of a range of highly controlled signaling molecules triggers EMT in response to specific stimuli (23). However in cancer cells, activation of this process is dysregulated (22). During EMT, adhesion molecule expression is altered and cells take on mesenchymal characteristics, becoming more elongated, flexible, mobile, and thereby potentially invasive (19). This phenotype also mediates increased resistance to common anti-cancer therapies including taxanes and anthracyclines (24) and is elevated in breast cancer tissues remaining after neoadjuvant therapies (25). Tumor cells surviving in the hostile environment of the blood have undergone demonstrated EMT changes (26, 27), which are considered crucial to the metastatic process (28) and to resistance to anoikis (29). EMT is, for instance, most evident in “triple-negative” tumors [those without estrogen receptors (ER), progesterone receptors (PR), and human epidermal growth factor receptor 2 (HER2)] and HER2 positive (HER2+) tumors, and least frequent in ER positive (ER+) tumors, particularly lobular cancers, mirroring the metastatic potential of these tumor types (27, 30).
Whilst EMT/mesenchymal markers have been demonstrated on CTCs, breast cancer metastases in liver, lung, and brain often express higher levels of E-cadherin and hence are often “more epithelial” than the primary tumor, indicating a reversal of the EMT process (31), termed mesenchymal to epithelial transition (MET). Evidence for the importance of this reverse transition and its role in metastasis is growing rapidly (32–36).
Sub-Populations of CTCs
Circulating tumor cells can exist in intermediate states – sub-populations expressing both epithelial and mesenchymal markers to varying degrees (27, 37–40). This is likely to be considerably more common than complete polarization to either state (41). Sub-populations of tumor cells at any point may also acquire cancer “stem-cell” (CSC) attributes such as quiescence, self-renewal, asymmetric division, drug resistance (38, 42), and resistance to radiation (43), facilitating survival in the circulation and resultant metastasis. Breast CSCs are most commonly identified with a CD44+/CD24− phenotype (44) or by the expression of aldehyde dehydrogenase 1 (ALDH1) (45). These CSC markers have been identified in breast cancer CTCs populations by a number of researchers (46–49).
CTC Isolation Methodologies
Current CTC detection methods rely on CTC physical properties (e.g., size, density, electric charge, and cell deformability) or on the retained expression of surface proteins (predominantly epithelial) or messenger RNA. Although there are currently numerous CTC detection methodologies [comprehensive reviews (13, 50–53), the CellSearch system (Veridex, USA)], an immunomagnetic bead capture system based on epithelial cell adhesion molecule (EpCAM), followed by immunofluorescence analysis predominates, as it is the only current method to achieve Federal Drug Administration approval. As malignant cell transcriptional profiles vary, especially during processes such as EMT and CSC formation, expression of identifying proteins may be lost in CTCs as well as being present in non-CTCs, reducing sensitivity and specificity. Barriere et al. (54) reviewed studies exploring CTC isolation, noting their propensity to co-express epithelial, mesenchymal, and CSC markers, and recommended development of a combined isolation method targeting all three phenotypes to avoid missing clinically relevant CTC sub-populations.
CTCs as Prognostic Tools
Both CTCs and DTCs have been detected in breast cancer patients with disease states ranging from ductal carcinoma in situ to MBC (55–60), and their detection is generally associated with a poor prognosis. Although CTCs are not seen in all MBC, this may be due to the inability of current methods to detect EMT sub-populations (54, 61, 62). Extensive studies in MBC show that CTCs associate with disease progression (57, 63, 64) with a meta-analysis by Zhang et al. (10) confirming CTC presence to be an independent prognostic factor for overall survival (OS) in MBC (HR = 2.33, p < 0.005).
Links with CTC presence and prognosis in EBC are also suggested (65, 66). Confirming this, a defining meta-analysis by Zhang et al. (10) showed the presence of CTCs to be an independent prognostic factor for OS in EBC (HR = 2.78, p < 0.005).
The association of CTCs and prognosis in EBC appears independent of tumor grade, histological type, degree of nodal involvement, lymphovascular invasion, or Ki-67 (proliferation marker) status (67, 68). Mixed results have been seen when considering receptor-defined breast cancer subtypes. Detection of CTCs is prognostic in EBC patients with “triple-negative” tumors or ER negative (ER−) PR negative (PR−) HER2+ primary tumors, but not in patients with ER+ tumors (69). In contrast, Giordano et al. (70) found CTCs to be prognostic in all MBC disease subtypes except HER2+ tumors, whilst Liu et al. (71) found the contrary.
The prognostic importance of CTCs over long-term follow-up has not been established. CTCs have been detected in patients in prolonged remission with 36% of patients in one study having detectable CTCs 8–22 years out from treatment of EBC, despite no clinical evidence of disease (72). What proportion of these patients will go on to develop metastatic disease is not known, nor have the beneficial effects of CTC-guided intervention been established (see Monitoring Treatment – Clinical Utility section below).
Receptor Discordance
Amplification of the HER2/neu gene and subsequent HER2 protein overexpression is associated with significantly decreased disease-free survival (DFS) and OS in the absence of HER2-targeted therapy (73, 74). Similarly, patients with HER2+ CTCs have been reported to have worse progression-free survival (PFS) and OS in comparison with patients with HER2− CTCs or any detectable CTCs (75–77). Heterogeneous amplification of HER2 is, however, known to occur within tumors and this serves to confound HER2 diagnostics and studies of receptor discordance (78). Receptor discordance refers to differences in receptors of primary tumor and metastatic tumors or CTCs. Discordance in HER2 status between primary tumor and CTCs reports are variable, in the order of 15–35% in MBC (75, 79, 80). HER2 discordance has also been reported in EBC patients. Wulfing et al. (77) found that, in EBC patients with detectable CTCs, 12 of 24 (50%) patients with HER2− primary tumors had HER2+ CTCs, and 1 of 3 (33%) patients with HER2+ primary tumors had HER2− CTCs. A few studies have shown that trastuzumab treatment is effective in eliminating HER2+ CTCs, including from patients with HER2− primary tumors and significantly reduced the risk of relapse and prolonged the DFS (81, 82).
Clinical trials are underway testing the utility of CTCs as a therapy decision-making tool in such cases of observed discrepancy in HER2 positivity between the primary tumor and CTCs. The DETECT III trial is randomizing HER2− MBC patients with HER2+ CTCs to standard therapy with or without lapatinib, a HER2-targeted therapy. The TREAT-CTC trial is randomizing HER2− EBC patients with detectable HER2+ CTCs post-neoadjuvant therapy (NT) and surgery to either standard care or additional trastuzumab. These studies may provide a foundation for the use of CTCs in standard clinical practice to identify patients who may benefit from the addition of HER2−directed therapy.
Discordance between the ER and/or PR status of primary and metastatic tumors has long been observed (83–85). Given the role of CTC in progression to metastases, it is not surprising that the hormone receptor status of CTCs may also differ from that of the primary tumor. Interestingly, this discordance appears much greater than that seen between primary and metastatic tissue, implying that receptors may be lost then regained once overt metastases form. Aktas et al. (86) found that discordance rates between primary tumor and CTCs for ER and PR in MBC patients were 59% and 55%, respectively, with most CTCs being ER− and PR− (84% and 92%). Fehm et al. found discordance rates between primary tumors and CTCs in EBC for ER and PR to be 71% and 75%, respectively (87) and HER2 discordance rates in MBC patients to be 36% (76). Although this suggests that hormone receptor loss may often be a transient phenomenon connected with the CTC state, this “sanctuary phenotype” could still contribute to endocrine therapy failure.
As with HER2 discordance; there are also implications for treatment of ER+ CTCs where the primary tumor is ER−. It remains to be seen if estrogen-targeted treatments in ER− primary tumors with ER+ CTCs have a therapeutic effect.
Monitoring Treatment – Clinical Utility
Another important clinical area uses CTCs as an early marker of disease progression or treatment failure – potentially giving an indication of a need for change of therapy before conventional imaging and/or tumor markers demonstrate progression. The lack of a reliable method to monitor the effects of adjuvant systemic therapy in particular is a significant area of need. Multiple studies have shown that in EBC, locally advanced breast cancer, and MBC, detection of CTCs after the completion of treatment is a strong prognostic marker (58, 64, 88–90).
Circulating tumor cell changes in MBC response to treatment can yield important prognostic information. For instance, MBC patients in whom initially high CTC counts reduced to low levels after initial therapy, had identical prognosis to CTC-negative patients (64). Correlations between the changes in CTC numbers and an objective response to therapy as assessed by serial imaging were reported by a study conducted by Nakamura et al. (91). Pachmann et al. (92) showed that patients who had higher CTC numbers that declined following treatment had a better prognosis than those whose CTC count did not change. Utilizing this paradigm, the SWOG SO500 trial evaluated switching therapy in MBC patients after one treatment cycle if certain CTC fall thresholds were not met. This trial did not demonstrate that an early switch improved DFS or OS, but presence of CTCs was an adverse prognostic factor (93). It has been suggested that the reason for the failure of this trial to observe a benefit to switching treatment on the basis of CTC levels is due to the fact that breast cancers with acquired chemo-resistance to one agent rarely exhibit high sensitivity to a randomly chosen alternative chemotherapeutic agent (94).
There are a number of ongoing clinical trials examining the utility of CTCs in breast cancer treatment. Details of some of the interventional studies employing CTC assessments, which are currently being run, are shown in Table 1. Results are eagerly awaited from the CirCe01 trial, which has similar design to the SWOG SO500 trial but evaluates CTCs serially after each cycle, with patients in the intervention arm changing therapy if CTC counts are adverse (see Table 1). Currently, we do not have clinical trial results supporting the use of CTCs to guide clinical decisions. Bardia et al. (94) highlighted the need for future clinical trials to utilize CTC isolation methodologies that are able to isolate CTCs which have undergone EMT, and to genotype CTCs in order to evaluate therapeutic response and guide therapeutic choices.
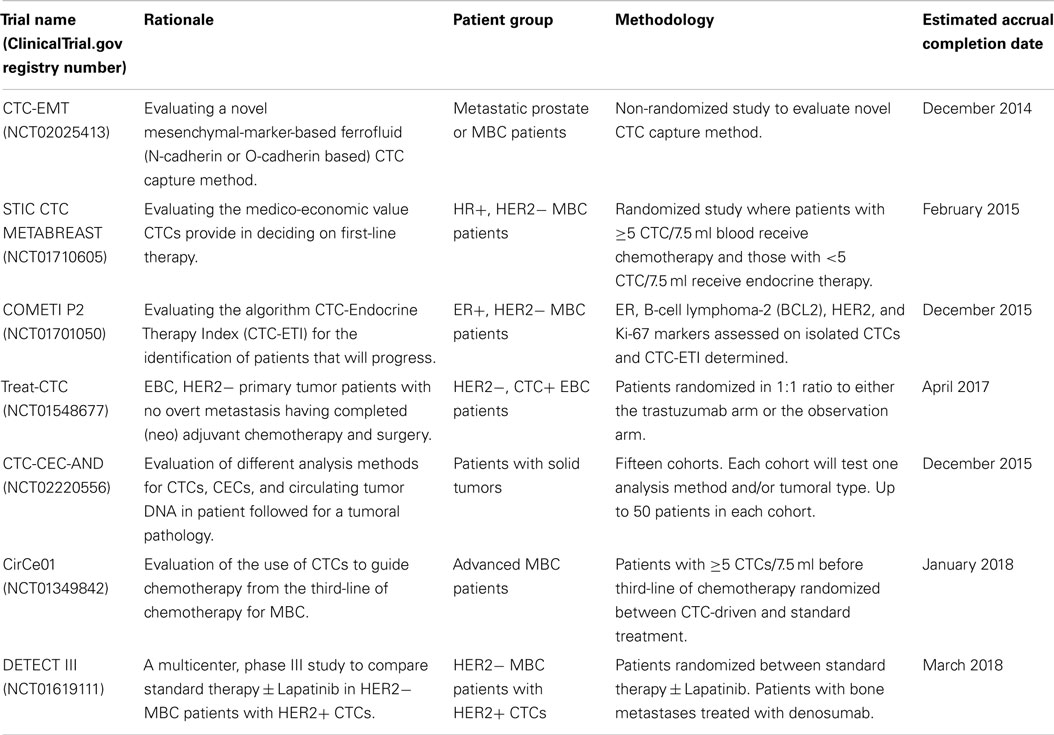
Table 1. A selection of current ongoing clinical trials examining the clinical utility of circulating tumor cells in breast cancer treatment.
Circulating tumor cells have been studied with respect to their potential to inform patient therapy. Pierga et al. (95) found a significant correlation between CTC detection before NT and reduced DFS, but no correlation between the persistence of CTCs post-NT and tumor response. Boutrus et al. (96) also found that CTC presence predicted local and distant relapse, but did not correlate with primary tumor volume reduction. Similarly, Riethdorf et al. (68) showed that CTC detection before NT did not correlate with tumor response to treatment, nor did CTC changes necessarily mirror treatment response. This suggests differential responses to treatment between the primary tumor and CTCs.
A large neoadjuvant chemotherapy study conducted by Rack et al. (60) in EBC patients found separate prognostic importance for the presence of CTCs pre- and post-treatment. Interestingly, the initially CTC-negative patients who subsequently developed CTCs fared better than initially CTC positive patients whose CTCs disappeared post-treatment, suggesting CTC clearance does not predict chemotherapy benefit (60).
To date, few studies have examined drug resistance in CTCs. Gradilone et al. (97) evaluated CTC of 42 MBC patients for expression of multi-drug resistance-related proteins (MRPs) and/or ALDH1, a putative tumor-initiating cell/CSC marker that correlates with resistance to some chemotherapeutics. The expression of MRPs on CTCs was found to be predictive of poor response to chemotherapy and significantly correlated with reduced PFS in MBC patients. Patients with CTCs expressing two or more MRPs had shorter PFS than those with CTCs expressing zero or one MRP (7.1 versus 16.4 months; p = 0.004). Furthermore, the expression of ALDH1 on CTCs was correlated with MRPs (and the number of MRPs expressed (p = 0.000) as well as an increased resistance to chemotherapy. Gazzaniga et al. (98) screened 105 cancer patients (of which 14 had breast cancer) for CTCs and then evaluated the MRP profile of the CTCs, postulating that this could delineate chemotherapy responders from non-responders. Patients were classified as chemotherapy “resistant” or “sensitive” on the basis of their CTC MRP profile, together with the chemotherapy regime the patient had received. This study found that the MRP profiles of patients’ CTCs to be highly predictive of response to chemotherapy, independent of tumor type and stage of disease.
Conclusion
The presence of CTCs is a powerful independent prognostic factor in both MBC and EBC. However, we increasingly understand that CTCs are heterogeneous, even within an individual patient at different times in the disease trajectory (27, 99). This includes in the receptors that they express, in relation to either the primary tumor or any metastatic disease, as well as in their variable expression of epithelial and mesenchymal markers.
Although CTC count changes are predictive of outcome in MBC, this is largely a disease where serial agents are delivered with palliative intent. Hence, early tailoring of therapies may not greatly impact on outcome. To date, clinical trials have shown that absolute CTC count alterations or CTC persistence do not predict strongly for neoadjuvant response, or improved adjuvant or metastatic outcomes, and hence currently do not provide clinically useful information to drive changes in therapies. With the maturation of the current clinical trials and further developments in the molecular characterization of CTCs, this information will hopefully become available.
Further work is needed, looking at CTC sub-populations including the presence and importance of EMT and CSC populations, and their alteration with treatment. There may be potential for targeting of otherwise treatment-resistant CTCs through novel targets on such populations. Additionally, the appearance of established therapeutic targets such as HER2 and ER on CTCs not present on the primary tumor is of considerable clinical importance, and the results of ongoing HER2-targeting trials are awaited with interest.
The promise of a “liquid biopsy” to diagnose, characterize, monitor, and influence treatment of cancer is still some way off. However profiling the presence and molecular characteristics of CTCs is very likely to provide important predictive and prognostic information in both early and MBC, and may prove useful in assessing response to treatment and as an early warning system for disease recurrence.
Conflict of Interest Statement
The authors declare that the research was conducted in the absence of any commercial or financial relationships that could be construed as a potential conflict of interest.
Acknowledgments
The authors gratefully acknowledge the National Breast Cancer Foundation’s National Collaborative Research Program (EMPathy Breast Cancer Network, CG-10-04) and the Victorian Government’s Operational Infrastructure Support Program (St. Vincent’s Institute) for supporting this work.
References
1. Forman D, Bray F, Brewster DH, Gombe Mbalawa C, Kohler B, Piñeros M, et al., editors. Cancer Incidence in Five Continents (CI5). Lyon: IARC Scientific Publications (2013).
2. Albain K, Anderson S, Arriagada R, Barlow W, Bergh J, Bliss J, et al. Comparisons between different polychemotherapy regimens for early breast cancer: meta-analyses of long-term outcome among 100 000 women in 123 randomised trials. Lancet (2012) 379(9814):432–44. doi: 10.1016/S0140-6736(11)61625-5
Pubmed Abstract | Pubmed Full Text | CrossRef Full Text | Google Scholar
3. Tjensvoll K, Oltedal S, Heikkila R, Kvaloy JT, Gilje B, Reuben JM, et al. Persistent tumor cells in bone marrow of non-metastatic breast cancer patients after primary surgery are associated with inferior outcome. BMC Cancer (2012) 12:190. doi:10.1186/1471-2407-12-190
Pubmed Abstract | Pubmed Full Text | CrossRef Full Text | Google Scholar
4. Butler TP, Gullino PM. Quantitation of cell shedding into efferent blood of mammary adenocarcinoma. Cancer Res (1975) 35(3):512–6.
5. Berezovskaya O, Schimmer AD, Glinskii AB, Pinilla C, Hoffman RM, Reed JC, et al. Increased expression of apoptosis inhibitor protein XIAP contributes to anoikis resistance of circulating human prostate cancer metastasis precursor cells. Cancer Res (2005) 65(6):2378–86. doi:10.1158/0008-5472.CAN-04-2649
Pubmed Abstract | Pubmed Full Text | CrossRef Full Text | Google Scholar
6. Frisch SM, Francis H. Disruption of epithelial cell-matrix interactions induces apoptosis. J Cell Biol (1994) 124(4):619–26. doi:10.1083/jcb.124.4.619
Pubmed Abstract | Pubmed Full Text | CrossRef Full Text | Google Scholar
7. Luzzi KJ, MacDonald IC, Schmidt EE, Kerkvliet N, Morris VL, Chambers AF, et al. Multistep nature of metastatic inefficiency: dormancy of solitary cells after successful extravasation and limited survival of early micrometastases. Am J Pathol (1998) 153(3):865–73. doi:10.1016/S0002-9440(10)65628-3
Pubmed Abstract | Pubmed Full Text | CrossRef Full Text | Google Scholar
8. Bonnomet A, Brysse A, Tachsidis A, Waltham M, Thompson EW, Polette M, et al. Epithelial-to-mesenchymal transitions and circulating tumor cells. J Mammary Gland Biol Neoplasia (2010) 15(2):261–73. doi:10.1007/s10911-010-9174-0
Pubmed Abstract | Pubmed Full Text | CrossRef Full Text | Google Scholar
9. Janni W, Vogl FD, Wiedswang G, Synnestvedt M, Fehm T, Juckstock J, et al. Persistence of disseminated tumor cells in the bone marrow of breast cancer patients predicts increased risk for relapse – a European pooled analysis. Clin Cancer Res (2011) 17(9):2967–76. doi:10.1158/1078-0432.CCR-10-2515
Pubmed Abstract | Pubmed Full Text | CrossRef Full Text | Google Scholar
10. Zhang L, Riethdorf S, Wu G, Wang T, Yang K, Peng G, et al. Meta-analysis of the prognostic value of circulating tumor cells in breast cancer. Clin Cancer Res (2012) 18(20):5701–10. doi:10.1158/1078-0432.CCR-12-1587
Pubmed Abstract | Pubmed Full Text | CrossRef Full Text | Google Scholar
11. Van der Auwera I, Peeters D, Benoy IH, Elst HJ, Van Laere SJ, Prove A, et al. Circulating tumour cell detection: a direct comparison between the CellSearch System, the AdnaTest and CK-19/mammaglobin RT-PCR in patients with metastatic breast cancer. Br J Cancer (2010) 102(2):276–84. doi:10.1038/sj.bjc.6605472
Pubmed Abstract | Pubmed Full Text | CrossRef Full Text | Google Scholar
12. Harris L, Fritsche H, Mennel R, Norton L, Ravdin P, Taube S, et al. American Society of Clinical Oncology 2007 update of recommendations for the use of tumor markers in breast cancer. J Clin Oncol (2007) 25(33):5287–312. doi:10.1200/JCO.2007.14.2364
Pubmed Abstract | Pubmed Full Text | CrossRef Full Text | Google Scholar
13. Sun YF, Yang XR, Zhou J, Qiu SJ, Fan J, Xu Y. Circulating tumor cells: advances in detection methods, biological issues, and clinical relevance. J Cancer Res Clin Oncol (2011) 137(8):1151–73. doi:10.1007/s00432-011-0988-y
Pubmed Abstract | Pubmed Full Text | CrossRef Full Text | Google Scholar
14. Marrinucci D, Bethel K, Bruce RH, Curry DN, Hsieh B, Humphrey M, et al. Case study of the morphologic variation of circulating tumor cells. Hum Pathol (2007) 38(3):514–9. doi:10.1016/j.humpath.2006.08.027
Pubmed Abstract | Pubmed Full Text | CrossRef Full Text | Google Scholar
15. Coumans FA, van Dalum G, Beck M, Terstappen LW. Filter characteristics influencing circulating tumor cell enrichment from whole blood. PLoS One (2013) 8(4):e61770. doi:10.1371/journal.pone.0061770
Pubmed Abstract | Pubmed Full Text | CrossRef Full Text | Google Scholar
16. Zheng S, Tai YC, Kasdan H. A micro device for separation of erythrocytes and leukocytes in human blood. Conf Proc IEEE Eng Med Biol Soc (2005) 1:1024–7. doi:10.1109/IEMBS.2005.1616592
Pubmed Abstract | Pubmed Full Text | CrossRef Full Text | Google Scholar
17. Allard WJ, Matera J, Miller MC, Repollet M, Connelly MC, Rao C, et al. Tumor cells circulate in the peripheral blood of all major carcinomas but not in healthy subjects or patients with nonmalignant diseases. Clin Cancer Res (2004) 10(20):6897–904. doi:10.1158/1078-0432.CCR-04-0378
Pubmed Abstract | Pubmed Full Text | CrossRef Full Text | Google Scholar
18. Aceto N, Bardia A, Miyamoto DT, Donaldson MC, Wittner BS, Spencer JA, et al. Circulating tumor cell clusters are oligoclonal precursors of breast cancer metastasis. Cell (2014) 158(5):1110–22. doi:10.1016/j.cell.2014.07.013
Pubmed Abstract | Pubmed Full Text | CrossRef Full Text | Google Scholar
19. Tomaskovic-Crook E, Thompson EW, Thiery JP. Epithelial to mesenchymal transition and breast cancer. Breast Cancer Res (2009) 11(6):213. doi:10.1186/bcr2416
Pubmed Abstract | Pubmed Full Text | CrossRef Full Text | Google Scholar
20. Kokkinos MI, Wafai R, Wong MK, Newgreen DF, Thompson EW, Waltham M. Vimentin and epithelial-mesenchymal transition in human breast cancer observations in vitro and in vivo. Cells Tissues Organs (2007) 185(1–3):191–203. doi:10.1159/000101320
Pubmed Abstract | Pubmed Full Text | CrossRef Full Text | Google Scholar
21. Iwatsuki M, Mimori K, Yokobori T, Ishi H, Beppu T, Nakamori S, et al. Epithelial-mesenchymal transition in cancer development and its clinical significance. Cancer Sci (2010) 101(2):293–9. doi:10.1111/j.1349-7006.2009.01419.x
Pubmed Abstract | Pubmed Full Text | CrossRef Full Text | Google Scholar
22. Thiery JP, Acloque H, Huang RY, Nieto MA. Epithelial-mesenchymal transitions in development and disease. Cell (2009) 139(5):871–90. doi:10.1016/j.cell.2009.11.007
Pubmed Abstract | Pubmed Full Text | CrossRef Full Text | Google Scholar
23. Weber CE, Li NY, Wai PY, Kuo PC. Epithelial-mesenchymal transition, TGF-beta, and osteopontin in wound healing and tissue remodeling after injury. J Burn Care Res (2012) 33(3):311–8. doi:10.1097/BCR.0b013e318240541e
Pubmed Abstract | Pubmed Full Text | CrossRef Full Text | Google Scholar
24. Gupta PB, Onder TT, Jiang G, Tao K, Kuperwasser C, Weinberg RA, et al. Identification of selective inhibitors of cancer stem cells by high-throughput screening. Cell (2009) 138(4):645–59. doi:10.1016/j.cell.2009.06.034
Pubmed Abstract | Pubmed Full Text | CrossRef Full Text | Google Scholar
25. Creighton CJ, Chang JC, Rosen JM. Epithelial-mesenchymal transition (EMT) in tumor-initiating cells and its clinical implications in breast cancer. J Mammary Gland Biol Neoplasia (2010) 15(2):253–60. doi:10.1007/s10911-010-9173-1
Pubmed Abstract | Pubmed Full Text | CrossRef Full Text | Google Scholar
26. Bednarz-Knoll N, Alix-Panabieres C, Pantel K. Plasticity of disseminating cancer cells in patients with epithelial malignancies. Cancer Metastasis Rev (2012) 31(3–4):673–87. doi:10.1007/s10555-012-9370-z
Pubmed Abstract | Pubmed Full Text | CrossRef Full Text | Google Scholar
27. Yu M, Bardia A, Wittner BS, Stott SL, Smas ME, Ting DT, et al. Circulating breast tumor cells exhibit dynamic changes in epithelial and mesenchymal composition. Science (2013) 339(6119):580–4. doi:10.1126/science.1228522
Pubmed Abstract | Pubmed Full Text | CrossRef Full Text | Google Scholar
28. Samatov TR, Tonevitsky AG, Schumacher U. Epithelial-mesenchymal transition: focus on metastatic cascade, alternative splicing, non-coding RNAs and modulating compounds. Mol Cancer (2013) 12(1):107. doi:10.1186/1476-4598-12-107
Pubmed Abstract | Pubmed Full Text | CrossRef Full Text | Google Scholar
29. Frisch SM, Schaller M, Cieply B. Mechanisms that link the oncogenic epithelial-mesenchymal transition to suppression of anoikis. J Cell Sci (2013) 126(1):21–9. doi:10.1242/jcs.120907
Pubmed Abstract | Pubmed Full Text | CrossRef Full Text | Google Scholar
30. Sarrio D, Rodriguez-Pinilla SM, Hardisson D, Cano A, Moreno-Bueno G, Palacios J. Epithelial-mesenchymal transition in breast cancer relates to the basal-like phenotype. Cancer Res (2008) 68(4):989–97. doi:10.1158/0008-5472.CAN-07-2017
Pubmed Abstract | Pubmed Full Text | CrossRef Full Text | Google Scholar
31. Chao YL, Shepard CR, Wells A. Breast carcinoma cells re-express E-cadherin during mesenchymal to epithelial reverting transition. Mol Cancer (2010) 9:179. doi:10.1186/1476-4598-9-179
Pubmed Abstract | Pubmed Full Text | CrossRef Full Text | Google Scholar
32. van Denderen BJ, Thompson EW. Cancer: the to and fro of tumour spread. Nature (2013) 493(7433):487–8. doi:10.1038/493487a
Pubmed Abstract | Pubmed Full Text | CrossRef Full Text | Google Scholar
33. Celia-Terrassa T, Meca-Cortes O, Mateo F, de Paz AM, Rubio N, Arnal-Estape A, et al. Epithelial-mesenchymal transition can suppress major attributes of human epithelial tumor-initiating cells. J Clin Invest (2012) 122(5):1849–68. doi:10.1172/JCI59218
Pubmed Abstract | Pubmed Full Text | CrossRef Full Text | Google Scholar
34. Korpal M, Ell BJ, Buffa FM, Ibrahim T, Blanco MA, Celia-Terrassa T, et al. Direct targeting of Sec23a by miR-200s influences cancer cell secretome and promotes metastatic colonization. Nat Med (2011) 17(9):1101–8. doi:10.1038/nm.2401
Pubmed Abstract | Pubmed Full Text | CrossRef Full Text | Google Scholar
35. Ocana OH, Corcoles R, Fabra A, Moreno-Bueno G, Acloque H, Vega S, et al. Metastatic colonization requires the repression of the epithelial-mesenchymal transition inducer Prrx1. Cancer Cell (2012) 22(6):709–24. doi:10.1016/j.ccr.2012.10.012
Pubmed Abstract | Pubmed Full Text | CrossRef Full Text | Google Scholar
36. Tsai JH, Donaher JL, Murphy DA, Chau S, Yang J. Spatiotemporal regulation of epithelial-mesenchymal transition is essential for squamous cell carcinoma metastasis. Cancer Cell (2012) 22(6):725–36. doi:10.1016/j.ccr.2012.09.022
Pubmed Abstract | Pubmed Full Text | CrossRef Full Text | Google Scholar
37. Bonnomet A, Syne L, Brysse A, Feyereisen E, Thompson EW, Noel A, et al. A dynamic in vivo model of epithelial-to-mesenchymal transitions in circulating tumor cells and metastases of breast cancer. Oncogene (2012) 31(33):3741–53. doi:10.1038/onc.2011.540
Pubmed Abstract | Pubmed Full Text | CrossRef Full Text | Google Scholar
38. Raimondi C, Gradilone A, Naso G, Vincenzi B, Petracca A, Nicolazzo C, et al. Epithelial-mesenchymal transition and stemness features in circulating tumor cells from breast cancer patients. Breast Cancer Res Treat (2011) 130(2):449–55. doi:10.1007/s10549-011-1373-x
Pubmed Abstract | Pubmed Full Text | CrossRef Full Text | Google Scholar
39. Paterlini-Brechot P, Benali NL. Circulating tumor cells (CTC) detection: clinical impact and future directions. Cancer Lett (2007) 253(2):180–204. doi:10.1016/j.canlet.2006.12.014
Pubmed Abstract | Pubmed Full Text | CrossRef Full Text | Google Scholar
40. Lecharpentier A, Vielh P, Perez-Moreno P, Planchard D, Soria JC, Farace F. Detection of circulating tumour cells with a hybrid (epithelial/mesenchymal) phenotype in patients with metastatic non-small cell lung cancer. Br J Cancer (2011) 105(9):1338–41. doi:10.1038/bjc.2011.405
Pubmed Abstract | Pubmed Full Text | CrossRef Full Text | Google Scholar
41. Armstrong AJ, Marengo MS, Oltean S, Kemeny G, Bitting R, Turnbull J, et al. Circulating tumor cells from patients with advanced prostate and breast cancer display both epithelial and mesenchymal markers. Mol Cancer Res (2011) 9(8):997–1007. doi:10.1158/1541-7786.MCR-10-0490
Pubmed Abstract | Pubmed Full Text | CrossRef Full Text | Google Scholar
42. Morel AP, Lievre M, Thomas C, Hinkal G, Ansieau S, Puisieux A. Generation of breast cancer stem cells through epithelial-mesenchymal transition. PLoS One (2008) 3(8):e2888. doi:10.1371/journal.pone.0002888
Pubmed Abstract | Pubmed Full Text | CrossRef Full Text | Google Scholar
43. Rich JN. Cancer stem cells in radiation resistance. Cancer Res (2007) 67(19):8980–4. doi:10.1158/0008-5472.CAN-07-0895
Pubmed Abstract | Pubmed Full Text | CrossRef Full Text | Google Scholar
44. Al-Hajj M, Wicha MS, Benito-Hernandez A, Morrison SJ, Clarke MF. Prospective identification of tumorigenic breast cancer cells. Proc Natl Acad Sci U S A (2003) 100(7):3983–8. doi:10.1073/pnas.0530291100
Pubmed Abstract | Pubmed Full Text | CrossRef Full Text | Google Scholar
45. Ginestier C, Hur MH, Charafe-Jauffret E, Monville F, Dutcher J, Brown M, et al. ALDH1 is a marker of normal and malignant human mammary stem cells and a predictor of poor clinical outcome. Cell Stem Cell (2007) 1(5):555–67. doi:10.1016/j.stem.2007.08.014
Pubmed Abstract | Pubmed Full Text | CrossRef Full Text | Google Scholar
46. Zhang L, Ridgway LD, Wetzel MD, Ngo J, Yin W, Kumar D, et al. The identification and characterization of breast cancer CTCs competent for brain metastasis. Sci Transl Med (2013) 5(180):180ra48. doi:10.1126/scitranslmed.3005109
Pubmed Abstract | Pubmed Full Text | CrossRef Full Text | Google Scholar
47. Theodoropoulos PA, Polioudaki H, Agelaki S, Kallergi G, Saridaki Z, Mavroudis D, et al. Circulating tumor cells with a putative stem cell phenotype in peripheral blood of patients with breast cancer. Cancer Lett (2010) 288(1):99–106. doi:10.1016/j.canlet.2009.06.027
Pubmed Abstract | Pubmed Full Text | CrossRef Full Text | Google Scholar
48. Wang N, Shi L, Li H, Hu Y, Du W, Liu W, et al. Detection of circulating tumor cells and tumor stem cells in patients with breast cancer by using flow cytometry: a valuable tool for diagnosis and prognosis evaluation. Tumour Biol (2012) 33(2):561–9. doi:10.1007/s13277-011-0303-1
Pubmed Abstract | Pubmed Full Text | CrossRef Full Text | Google Scholar
49. Aktas B, Tewes M, Fehm T, Hauch S, Kimmig R, Kasimir-Bauer S. Stem cell and epithelial-mesenchymal transition markers are frequently overexpressed in circulating tumor cells of metastatic breast cancer patients. Breast Cancer Res (2009) 11(4):R46. doi:10.1186/bcr2333
Pubmed Abstract | Pubmed Full Text | CrossRef Full Text | Google Scholar
50. Friedlander TW, Premasekharan G, Paris PL. Looking back, to the future of circulating tumor cells. Pharmacol Ther (2014) 142(3):271–80. doi:10.1016/j.pharmthera.2013.12.011
Pubmed Abstract | Pubmed Full Text | CrossRef Full Text | Google Scholar
51. Liberko M, Kolostova K, Bobek V. Essentials of circulating tumor cells for clinical research and practice. Crit Rev Oncol Hematol (2013) 88(2):338–56. doi:10.1016/j.critrevonc.2013.05.002
Pubmed Abstract | Pubmed Full Text | CrossRef Full Text | Google Scholar
52. Yu M, Stott S, Toner M, Maheswaran S, Haber DA. Circulating tumor cells: approaches to isolation and characterization. J Cell Biol (2011) 192(3):373–82. doi:10.1083/jcb.201010021
Pubmed Abstract | Pubmed Full Text | CrossRef Full Text | Google Scholar
53. Alix-Panabieres C, Pantel K. Circulating tumor cells: liquid biopsy of cancer. Clin Chem (2013) 59(1):110–8. doi:10.1373/clinchem.2012.194258
Pubmed Abstract | Pubmed Full Text | CrossRef Full Text | Google Scholar
54. Barriere G, Tartary M, Rigaud M. Epithelial mesenchymal transition: a new insight into the detection of circulating tumor cells. ISRN Oncol (2012) 2012:382010. doi:10.5402/2012/382010
Pubmed Abstract | Pubmed Full Text | CrossRef Full Text | Google Scholar
55. Ignatiadis M, Rothe F, Chaboteaux C, Durbecq V, Rouas G, Criscitiello C, et al. HER2-positive circulating tumor cells in breast cancer. PLoS One (2011) 6(1):e15624. doi:10.1371/journal.pone.0015624
Pubmed Abstract | Pubmed Full Text | CrossRef Full Text | Google Scholar
56. Sanger N, Effenberger KE, Riethdorf S, Van Haasteren V, Gauwerky J, Wiegratz I, et al. Disseminated tumor cells in the bone marrow of patients with ductal carcinoma in situ. Int J Cancer (2011) 129(10):2522–6. doi:10.1002/ijc.25895
Pubmed Abstract | Pubmed Full Text | CrossRef Full Text | Google Scholar
57. Cristofanilli M, Budd GT, Ellis MJ, Stopeck A, Matera J, Miller MC, et al. Circulating tumor cells, disease progression, and survival in metastatic breast cancer. N Engl J Med (2004) 351(8):781–91. doi:10.1056/NEJMoa040766
Pubmed Abstract | Pubmed Full Text | CrossRef Full Text | Google Scholar
58. Xenidis N, Ignatiadis M, Apostolaki S, Perraki M, Kalbakis K, Agelaki S, et al. Cytokeratin-19 mRNA-positive circulating tumor cells after adjuvant chemotherapy in patients with early breast cancer. J Clin Oncol (2009) 27(13):2177–84. doi:10.1200/JCO.2008.18.0497
Pubmed Abstract | Pubmed Full Text | CrossRef Full Text | Google Scholar
59. Diel IJ, Kaufmann M, Costa SD, Holle R, von Minckwitz G, Solomayer EF, et al. Micrometastatic breast cancer cells in bone marrow at primary surgery: prognostic value in comparison with nodal status. J Natl Cancer Inst (1996) 88(22):1652–8. doi:10.1093/jnci/88.22.1652
Pubmed Abstract | Pubmed Full Text | CrossRef Full Text | Google Scholar
60. Rack B, Schindlbeck C, Juckstock J, Andergassen U, Hepp P, Zwingers T, et al. Circulating tumor cells predict survival in early average-to-high risk breast cancer patients. J Natl Cancer Inst (2014) 106(5). doi:10.1093/jnci/dju066
Pubmed Abstract | Pubmed Full Text | CrossRef Full Text | Google Scholar
61. Pecot CV, Bischoff FZ, Mayer JA, Wong KL, Pham T, Bottsford-Miller J, et al. A novel platform for detection of CK+ and CK− CTCs. Cancer Discov (2011) 1(7):580–6. doi:10.1158/2159-8290.CD-11-0215
Pubmed Abstract | Pubmed Full Text | CrossRef Full Text | Google Scholar
62. Gradilone A, Raimondi C, Nicolazzo C, Petracca A, Gandini O, Vincenzi B, et al. Circulating tumour cells lacking cytokeratin in breast cancer: the importance of being mesenchymal. J Cell Mol Med (2011) 15(5):1066–70. doi:10.1111/j.1582-4934.2011.01285.x
Pubmed Abstract | Pubmed Full Text | CrossRef Full Text | Google Scholar
63. Muller V, Stahmann N, Riethdorf S, Rau T, Zabel T, Goetz A, et al. Circulating tumor cells in breast cancer: correlation to bone marrow micrometastases, heterogeneous response to systemic therapy and low proliferative activity. Clin Cancer Res (2005) 11(10):3678–85. doi:10.1158/1078-0432.CCR-04-2469
Pubmed Abstract | Pubmed Full Text | CrossRef Full Text | Google Scholar
64. Hayes DF, Cristofanilli M, Budd GT, Ellis MJ, Stopeck A, Miller MC, et al. Circulating tumor cells at each follow-up time point during therapy of metastatic breast cancer patients predict progression-free and overall survival. Clin Cancer Res (2006) 12(14 Pt 1):4218–24. doi:10.1158/1078-0432.CCR-05-2821
Pubmed Abstract | Pubmed Full Text | CrossRef Full Text | Google Scholar
65. Molloy TJ, Devriese LA, Helgason HH, Bosma AJ, Hauptmann M, Voest EE, et al. A multimarker QPCR-based platform for the detection of circulating tumour cells in patients with early-stage breast cancer. Br J Cancer (2011) 104(12):1913–9. doi:10.1038/bjc.2011.164
Pubmed Abstract | Pubmed Full Text | CrossRef Full Text | Google Scholar
66. Saloustros E, Perraki M, Apostolaki S, Kallergi G, Xyrafas A, Kalbakis K, et al. Cytokeratin-19 mRNA-positive circulating tumor cells during follow-up of patients with operable breast cancer: prognostic relevance for late relapse. Breast Cancer Res (2011) 13(3):R60. doi:10.1186/bcr2897
Pubmed Abstract | Pubmed Full Text | CrossRef Full Text | Google Scholar
67. Lang JE, Mosalpuria K, Cristofanilli M, Krishnamurthy S, Reuben J, Singh B, et al. HER2 status predicts the presence of circulating tumor cells in patients with operable breast cancer. Breast Cancer Res Treat (2009) 113(3):501–7. doi:10.1007/s10549-008-9951-2
Pubmed Abstract | Pubmed Full Text | CrossRef Full Text | Google Scholar
68. Riethdorf S, Muller V, Zhang L, Rau T, Loibl S, Komor M, et al. Detection and HER2 expression of circulating tumor cells: prospective monitoring in breast cancer patients treated in the neoadjuvant GeparQuattro trial. Clin Cancer Res (2010) 16(9):2634–45. doi:10.1158/1078-0432.CCR-09-2042
Pubmed Abstract | Pubmed Full Text | CrossRef Full Text | Google Scholar
69. Ignatiadis M, Xenidis N, Perraki M, Apostolaki S, Politaki E, Kafousi M, et al. Different prognostic value of cytokeratin-19 mRNA positive circulating tumor cells according to estrogen receptor and HER2 status in early-stage breast cancer. J Clin Oncol (2007) 25(33):5194–202. doi:10.1200/JCO.2007.11.7762
Pubmed Abstract | Pubmed Full Text | CrossRef Full Text | Google Scholar
70. Giordano A, Giuliano M, De Laurentiis M, Arpino G, Jackson S, Handy BC, et al. Circulating tumor cells in immunohistochemical subtypes of metastatic breast cancer: lack of prediction in HER2-positive disease treated with targeted therapy. Ann Oncol (2012) 23(5):1144–50. doi:10.1093/annonc/mdr434
Pubmed Abstract | Pubmed Full Text | CrossRef Full Text | Google Scholar
71. Liu Y, Liu Q, Wang T, Bian L, Zhang S, Hu H, et al. Circulating tumor cells in HER2-positive metastatic breast cancer patients: a valuable prognostic and predictive biomarker. BMC Cancer (2013) 13:202. doi:10.1186/1471-2407-13-202
Pubmed Abstract | Pubmed Full Text | CrossRef Full Text | Google Scholar
72. Meng S, Tripathy D, Frenkel EP, Shete S, Naftalis EZ, Huth JF, et al. Circulating tumor in patients with breast cancer dormancy. Clin Cancer Res (2004) 10(24):8152–62. doi:10.1158/1078-0432.CCR-04-1110
Pubmed Abstract | Pubmed Full Text | CrossRef Full Text | Google Scholar
73. Slamon DJ, Godolphin W, Jones LA, Holt JA, Wong SG, Keith DE, et al. Studies of the HER-2/neu proto-oncogene in human breast and ovarian cancer. Science (1989) 244(4905):707–12. doi:10.1126/science.2470152
Pubmed Abstract | Pubmed Full Text | CrossRef Full Text | Google Scholar
74. Slamon DJ, Clark GM, Wong SG, Levin WJ, Ullrich A, McGuire WL. Human breast cancer: correlation of relapse and survival with amplification of the HER-2/neu oncogene. Science (1987) 235(4785):177–82. doi:10.1126/science.3798106
Pubmed Abstract | Pubmed Full Text | CrossRef Full Text | Google Scholar
75. Munzone E, Nole F, Goldhirsch A, Botteri E, Esposito A, Zorzino L, et al. Changes of HER2 status in circulating tumor cells compared with the primary tumor during treatment for advanced breast cancer. Clin Breast Cancer (2010) 10(5):392–7. doi:10.3816/CBC.2010.n.052
Pubmed Abstract | Pubmed Full Text | CrossRef Full Text | Google Scholar
76. Hayashi N, Nakamura S, Tokuda Y, Shimoda Y, Yagata H, Yoshida A, et al. Prognostic value of HER2-positive circulating tumor cells in patients with metastatic breast cancer. Int J Clin Oncol (2012) 17(2):96–104. doi:10.1007/s10147-011-0260-0
Pubmed Abstract | Pubmed Full Text | CrossRef Full Text | Google Scholar
77. Wulfing P, Borchard J, Buerger H, Heidl S, Zanker KS, Kiesel L, et al. HER2-positive circulating tumor cells indicate poor clinical outcome in stage I to III breast cancer patients. Clin Cancer Res (2006) 12(6):1715–20. doi:10.1158/1078-0432.CCR-05-2087
Pubmed Abstract | Pubmed Full Text | CrossRef Full Text | Google Scholar
78. Bartlett AI, Starcyznski J, Robson T, Maclellan A, Campbell FM, van de Velde CJ, et al. Heterogeneous HER2 gene amplification: impact on patient outcome and a clinically relevant definition. Am J Clin Pathol (2011) 136(2):266–74. doi:10.1309/AJCP0EN6AQMWETZZ
Pubmed Abstract | Pubmed Full Text | CrossRef Full Text | Google Scholar
79. Fehm T, Muller V, Aktas B, Janni W, Schneeweiss A, Stickeler E, et al. HER2 status of circulating tumor cells in patients with metastatic breast cancer: a prospective, multicenter trial. Breast Cancer Res Treat (2010) 124(2):403–12. doi:10.1007/s10549-010-1163-x
Pubmed Abstract | Pubmed Full Text | CrossRef Full Text | Google Scholar
80. Flores LM, Kindelberger DW, Ligon AH, Capelletti M, Fiorentino M, Loda M, et al. Improving the yield of circulating tumour cells facilitates molecular characterisation and recognition of discordant HER2 amplification in breast cancer. Br J Cancer (2010) 102(10):1495–502. doi:10.1038/sj.bjc.6605676
Pubmed Abstract | Pubmed Full Text | CrossRef Full Text | Google Scholar
81. Georgoulias V, Bozionelou V, Agelaki S, Perraki M, Apostolaki S, Kallergi G, et al. Trastuzumab decreases the incidence of clinical relapses in patients with early breast cancer presenting chemotherapy-resistant CK19 mRNA-positive circulating tumor cells: results of a randomized phase II study. Ann Oncol (2012) 23(7):1744–50. doi:10.1093/annonc/mds020
Pubmed Abstract | Pubmed Full Text | CrossRef Full Text | Google Scholar
82. Meng S, Tripathy D, Shete S, Ashfaq R, Haley B, Perkins S, et al. HER-2 gene amplification can be acquired as breast cancer progresses. Proc Natl Acad Sci U S A (2004) 101(25):9393–8. doi:10.1073/pnas.0402993101
Pubmed Abstract | Pubmed Full Text | CrossRef Full Text | Google Scholar
83. Brennan MJ, Donegan WL, Appleby DE. The variability of estrogen receptors in metastatic breast cancer. Am J Surg (1979) 137(2):260–2. doi:10.1016/0002-9610(79)90159-4
Pubmed Abstract | Pubmed Full Text | CrossRef Full Text | Google Scholar
84. Holdaway IM, Bowditch JV. Variation in receptor status between primary and metastatic breast cancer. Cancer (1983) 52(3):479–85. doi:10.1002/1097-0142(19830801)52:3<479::AID-CNCR2820520317>3.0.CO;2-O
Pubmed Abstract | Pubmed Full Text | CrossRef Full Text | Google Scholar
85. Li BD, Byskosh A, Molteni A, Duda RB. Estrogen and progesterone receptor concordance between primary and recurrent breast cancer. J Surg Oncol (1994) 57(2):71–7. doi:10.1002/jso.2930570202
Pubmed Abstract | Pubmed Full Text | CrossRef Full Text | Google Scholar
86. Aktas B, Muller V, Tewes M, Zeitz J, Kasimir-Bauer S, Loehberg CR, et al. Comparison of estrogen and progesterone receptor status of circulating tumor cells and the primary tumor in metastatic breast cancer patients. Gynecol Oncol (2011) 122(2):356–60. doi:10.1016/j.ygyno.2011.04.039
Pubmed Abstract | Pubmed Full Text | CrossRef Full Text | Google Scholar
87. Fehm T, Hoffmann O, Aktas B, Becker S, Solomayer EF, Wallwiener D, et al. Detection and characterization of circulating tumor cells in blood of primary breast cancer patients by RT-PCR and comparison to status of bone marrow disseminated cells. Breast Cancer Res (2009) 11(4):R59. doi:10.1186/bcr2349
Pubmed Abstract | Pubmed Full Text | CrossRef Full Text | Google Scholar
88. Mathiesen RR, Borgen E, Renolen A, Lokkevik E, Nesland JM, Anker G, et al. Persistence of disseminated tumor cells after neoadjuvant treatment for locally advanced breast cancer predicts poor survival. Breast Cancer Res (2012) 14(4):R117. doi:10.1186/bcr3242
Pubmed Abstract | Pubmed Full Text | CrossRef Full Text | Google Scholar
89. Slade MJ, Payne R, Riethdorf S, Ward B, Zaidi SA, Stebbing J, et al. Comparison of bone marrow, disseminated tumour cells and blood-circulating tumour cells in breast cancer patients after primary treatment. Br J Cancer (2009) 100(1):160–6. doi:10.1038/sj.bjc.6604773
Pubmed Abstract | Pubmed Full Text | CrossRef Full Text | Google Scholar
90. Bidard FC, Mathiot C, Delaloge S, Brain E, Giachetti S, de Cremoux P, et al. Single circulating tumor cell detection and overall survival in nonmetastatic breast cancer. Ann Oncol (2010) 21(4):729–33. doi:10.1093/annonc/mdp391
Pubmed Abstract | Pubmed Full Text | CrossRef Full Text | Google Scholar
91. Nakamura S, Yagata H, Ohno S, Yamaguchi H, Iwata H, Tsunoda N, et al. Multi-center study evaluating circulating tumor cells as a surrogate for response to treatment and overall survival in metastatic breast cancer. Breast Cancer (2010) 17(3):199–204. doi:10.1007/s12282-009-0139-3
Pubmed Abstract | Pubmed Full Text | CrossRef Full Text | Google Scholar
92. Pachmann K, Camara O, Kavallaris A, Krauspe S, Malarski N, Gajda M, et al. Monitoring the response of circulating epithelial tumor cells to adjuvant chemotherapy in breast cancer allows detection of patients at risk of early relapse. J Clin Oncol (2008) 26(8):1208–15. doi:10.1200/JCO.2007.13.6523
Pubmed Abstract | Pubmed Full Text | CrossRef Full Text | Google Scholar
93. Smerage JB, Barlow WE, Hortobagyi GN, Winer EP, Leyland-Jones B, Srkalovic G, et al. Circulating tumor cells and response to chemotherapy in metastatic breast cancer: SWOG S0500. J Clin Oncol (2014) 32(31):3483–9. doi:10.1200/JCO.2014.56.2561
Pubmed Abstract | Pubmed Full Text | CrossRef Full Text | Google Scholar
94. Bardia A, Haber DA. Solidifying liquid biopsies: can circulating tumor cell monitoring guide treatment selection in breast cancer? J Clin Oncol (2014) 32(31):3470–1. doi:10.1200/JCO.2014.57.1505
Pubmed Abstract | Pubmed Full Text | CrossRef Full Text | Google Scholar
95. Pierga JY, Bidard FC, Mathiot C, Brain E, Delaloge S, Giachetti S, et al. Circulating tumor cell detection predicts early metastatic relapse after neoadjuvant chemotherapy in large operable and locally advanced breast cancer in a phase II randomized trial. Clin Cancer Res (2008) 14(21):7004–10. doi:10.1158/1078-0432.CCR-08-0030
Pubmed Abstract | Pubmed Full Text | CrossRef Full Text | Google Scholar
96. Boutrus RR, Abi Raad RF, Kuter I, Ancukiewicz M, Roberts L, Solomon N, et al. Circulating tumor cells as predictors of response and failure in breast cancer patients treated with preoperative chemotherapy. Int J Biol Markers (2012) 28(1):17–23. doi:10.5301/JBM.2012.9580
Pubmed Abstract | Pubmed Full Text | CrossRef Full Text | Google Scholar
97. Gradilone A, Naso G, Raimondi C, Cortesi E, Gandini O, Vincenzi B, et al. Circulating tumor cells (CTCs) in metastatic breast cancer (MBC): prognosis, drug resistance and phenotypic characterization. Ann Oncol (2011) 22(1):86–92. doi:10.1093/annonc/mdq323
Pubmed Abstract | Pubmed Full Text | CrossRef Full Text | Google Scholar
98. Gazzaniga P, Naso G, Gradilone A, Cortesi E, Gandini O, Gianni W, et al. Chemosensitivity profile assay of circulating cancer cells: prognostic and predictive value in epithelial tumors. Int J Cancer (2010) 126(10):2437–47. doi:10.1002/ijc.24953
Pubmed Abstract | Pubmed Full Text | CrossRef Full Text | Google Scholar
99. Powell AA, Talasaz AH, Zhang H, Coram MA, Reddy A, Deng G, et al. Single cell profiling of circulating tumor cells: transcriptional heterogeneity and diversity from breast cancer cell lines. PLoS One (2012) 7(5):e33788. doi:10.1371/journal.pone.0033788
Pubmed Abstract | Pubmed Full Text | CrossRef Full Text | Google Scholar
Keywords: circulating tumor cells, epithelial–mesenchymal transition, breast cancer, clinical application, metastasis
Citation: McInnes LM, Jacobson N, Redfern A, Dowling A, Thompson EW and Saunders CM (2015) Clinical implications of circulating tumor cells of breast cancer patients: role of epithelial–mesenchymal plasticity. Front. Oncol. 5:42. doi: 10.3389/fonc.2015.00042
Received: 08 October 2014; Accepted: 05 February 2015;
Published online: 26 February 2015.
Edited by:
Robin Anderson, Peter MacCallum Cancer Centre, AustraliaCopyright: © 2015 McInnes, Jacobson, Redfern, Dowling, Thompson and Saunders. This is an open-access article distributed under the terms of the Creative Commons Attribution License (CC BY). The use, distribution or reproduction in other forums is permitted, provided the original author(s) or licensor are credited and that the original publication in this journal is cited, in accordance with accepted academic practice. No use, distribution or reproduction is permitted which does not comply with these terms.
*Correspondence: Christobel M. Saunders, School of Surgery, The University of Western Australia, Mailbox M581, 35 Stirling Highway, Crawley, WA 6009, Australia e-mail:Y2hyaXN0b2JlbC5zYXVuZGVyc0B1d2EuZWR1LmF1
†Joint first authors.
‡Joint last authors.