- 1Division of Cardiology, Department of Internal Medicine, Faculty of Health Sciences, University of the Witwatersrand, Johannesburg, South Africa
- 2Department of Nuclear Medicine, University of Pretoria, Pretoria, Gauteng, South Africa
- 3Nuclear Medicine Research Infrastructure, Steve Biko Academic Hospital, Pretoria, South Africa
- 4Netcare Sunninghill, Sunward Park Hospitals, Johannesburg, South Africa
- 5Division of Nuclear Medicine, Department of Radiation Medicine, Faculty of Health Sciences, University of Cape Town, Cape Town, South Africa
- 6Department of Nuclear Medicine, College of Health Sciences, University of KwaZulu Natal, Durban, South Africa
- 7Department of Nuclear Medicine, Inkosi Albert Luthuli Central Hospital, Durban, South Africa
Gallium-68 fibroblast activation protein inhibitor [(68Ga)Ga-FAPI] is a new radiopharmaceutical positioning itself as the preferred agent in patients with malignant tumours, competing with 2-Deoxy-2-[18F]fluoro-d-glucose [2-(18F)FDG] using positron emission tomography (PET). While imaging oncology patients with [68Ga]Ga-FAPI PET, incidental uptake of [68Ga]Ga-FAPI has been detected in the myocardium. This review summarises original research studies associating the visualisation of FAPI-based tracers in the myocardium with underlying active cardiovascular disease.
1. Introduction
Gallium-68 fibroblast activation protein inhibitor [68Ga]Ga-FAPI is a new radiopharmaceutical widely used when imaging patients with various malignancies, inflammatory and pre-fibrotic conditions. The tumour environment predominantly consists of cancer-associated fibroblasts (CAF) and non-malignant cells that play a role in cancer metabolism and regulate tumour growth and aggressiveness (1). These CAF overexpress the fibroblast activation protein, a binding site for [68Ga]Ga-FAPI. Similarly, increased fibroblast activation protein (FAP) expression in patients with cardiac disease has been identified at day seven post myocardial infarction (2). When a myocardial injury occurs, fibroblasts differentiate into myofibroblasts which produce components of the extracellular matrix (ECM), predominantly collagen, to allow healing and maintaining the structural integrity of the heart.
2. Gallium-68 fibroblast activation protein inhibitor positron emission tomography
Fibroblasts are elongated spindle-shaped cells with a basophilic cytoplasm, an oval nucleus, a well-developed Golgi apparatus, and an abundantly rough endoplasmic reticulum (Figure 1). Fibroblasts originate from mesenchymal cells derived from stem cells. They are the most abundant cell type in the connective tissue of various organs. The cellular membrane of activated fibroblasts expresses FAP, a transmembrane serine protease composed of amino acids with an intracellular domain of six amino acids, and a transmembrane domain of 20 amino acids (3).
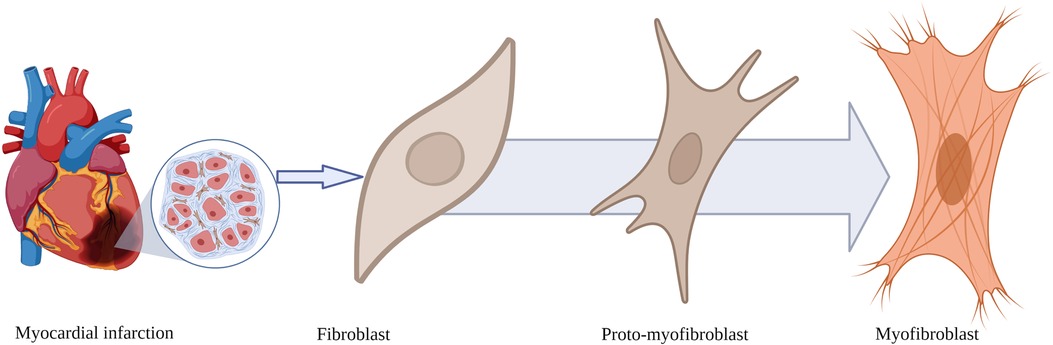
Figure 1. Morphological transformation of fibroblasts after myocardial infarction. Fibroblasts transform from a resting state into proto-myofibroblasts and myofibroblasts expressing fibroblast activation protein, the binding site for fibroblast activation protein inhibitor-based imaging agents.
Gallium-68 fibroblast activation protein inhibitor positron emission tomography (PET) has positioned itself as the preferred imaging modality for the staging and restaging of various oncological malignancies such as head, neck, and abdominal tumours, almost overthrowing 2-Deoxy-2-[18F]fluoro-d-glucose [2-(18F)FDG] PET (4). The ease of onsite preparation and the high tissue-to-background contrast ratio of gallium-labelled ligands render them ideal imaging agents over [2-(18F)FDG]. In some studies, [68Ga]Ga-FAPI PET has been used for radiation treatment planning and evaluation of biodistribution kinetics (5).
The synthesis of FAPI precursors for tumour binding and potential therapy, FAPI-02 up to FAPI-15, has been reported by Linder et al. (6). In their study, FAPI-04 had a favourable tumour-to-blood volume in patients with metastatic breast cancer. Also, FAPI-04 was more stable in human serum (6). Other FAPI precursors include FAP-34, which has been labelled with technetium-99m, FAPI-74, and FAPI-46 (7). Novel techniques for synthesising [68Ga]Ga-FAPI-46 involve mixing a buffer solution with ascorbic acid and 50 micrograms (µ) of FAPI-46 and transferring the mixture into the reactor vial. Gallium-68 is eluted from a Germanium-68/Gallium-68 generator, typically with 5 millilitres of 0.1 M hydrochloric acid, and then preheated. The [68Ga]GaCl3 solution eluted from the generator is mixed with 50 µg of the FAPI-46 precursor and heated at 90° C for 4 min (8).
Once the radiopharmaceutical has been administered intravenously, it travels in the bloodstream and enters the myocardium. Eventually, the [68Ga]Ga-FAPI complex binds to the fibroblast activation protein expressed on the cell membrane of activated fibroblasts. On the PET images of the myocardium, the localisation of the radiopharmaceutical in actively healing tissue will manifest as focal and sometimes diffuse increased uptake of [68Ga]Ga-FAPI as seen in cardiac amyloidosis. This has been demonstrated in case reports and retrospective studies conducted on patients with malignant or inflammatory diseases (9–11).
3. Preclinical studies supporting the use of a Gallium-68 fibroblast activation protein inhibitor post myocardial infarction
Preclinical studies have shown promising results on the role of [68Ga]Ga-FAPI in studying cardiac remodelling after myocardial infarction (MI) and in heart failure. Varasteh et al. induced MI in 20 rats by ligating the left anterior descending coronary artery, and a sham procedure was performed in four rats (2). A series of [68Ga]Ga-FAPI-04 positron emission tomography/computerised tomography (PET/CT) images were acquired on days 1, 3, 6, 14, 23, and 30 after MI, and the [68Ga]Ga-FAPI uptake peaked on day 6 and decreased rapidly by day 14. Immunofluorescence staining analyses on infarcted hearts on day 7 showed selective accumulation of FAP-positive cells in the peri-infarct zone (2).
Also, Qiao et al. noninvasively monitored reparative fibrosis in rats using [68Ga]Ga-FAPI PET/CT. In their study, they induced myocardial infarction in 16 rats by performing a thoracotomy and ligating the left anterior descending coronary artery (12). A thoracotomy was done on another 17 rats without ligating any coronary arteries (sham procedure). Serial imaging with [68Ga]Ga-FAPI PET/CT was performed in rats at different time points, from day 1 to day 35 post-surgery. Also, the excised cardiac tissue specimens from two rats with induced myocardial infarction and two sham-operated rats were subjected to histological examination, autoradiography, and immunofluorescence staining.
In the rats with MI, the excised cardiac tissue revealed an infiltrate of inflammatory cells, dissolved and broken muscle fibres, necrosis, and features of replacement fibrosis. Autoradiography showed an accumulation of FAPI in the infarcted myocardium and infarct border zone (12). Similarly, FAP-positive cells were identified in the infarcted area, specifically on days 3, 6, and 15 after the infarction. The myocardium of sham-operated rats exhibited normal morphology with neatly arranged myocardial fibres (12). Both of these studies demonstrated the feasibility of monitoring ventricular remodelling after MI.
Gallium-68 FAPI PET/CT findings have also been correlated with histopathological changes in rats with heart failure. Serial imaging was performed on the experimental and control groups of mice on day 0 before inducing heart failure with isoproterenol hydrochloride and 7, 14, 21, and 28 days thereafter (13). In rats with heart failure, [68Ga]Ga-FAPI uptake increased on days 7 and 14 and declined on days 21 and 28. Histological evaluation of heart tissue specimens showed fibrotic activity, which increased from day 0 to day 28 in rats with heart failure. No tracer uptake was seen on day 0, and a peak uptake was observed on day 7. Serial echocardiography findings showed a decline in systolic function on day 7. After day 7, ventricular chamber enlargement, ventricular wall thinning, and a reduction in myocardial contractility were observed. Also, the heart-to-muscle uptake ratio was the highest on day 7, gradually decreasing over time (13).
4. Clinical studies demonstrating the use of a Gallium-68 fibroblast activation protein inhibitor positron emission tomography in patients with various cardiovascular diseases
We conducted a systematic literature search on PubMed and Web of Science to identify original research studies and case reports demonstrating the use of FAPI on subjects known or suspected to have underlying cardiovascular diseases (CVD). The following search terms and associated Medical Subject Headings (MeSH) were used: “Gallium-68 AND Fibroblast activation protein” OR “Flourine-18 AND Fibroblast activation protein.” We retrieved 15 studies focused on the clinical utility of FAPI-based PET tracers labelled with either Gallium-68 or Flourine-18 on conditions such as coronary artery diseases, cardiomyopathies, infiltrative heart diseases, immune checkpoint inhibitor-related myocarditis, systemic sclerosis, and other pathologies such as pulmonary arterial hypertension, which may lead to fibrotic changes in the right ventricle (Table 1). Most of the evidence supporting the potential role of FAPI-based tracers in identifying active cardiac disease and managing CVD originates from original research studies with small sample sizes, case reports, or a retrospective review of FAPI PET images in patients with malignancies referred for PET imaging. In studies reporting incidental visualisation of cardiac uptake of FAPI in patients with underlying malignancies, logistic regression analysis reporting odds ratios and correlation studies reporting correlation coefficients were used to associate the visualisation of FAPI with CVD or its risk factors.
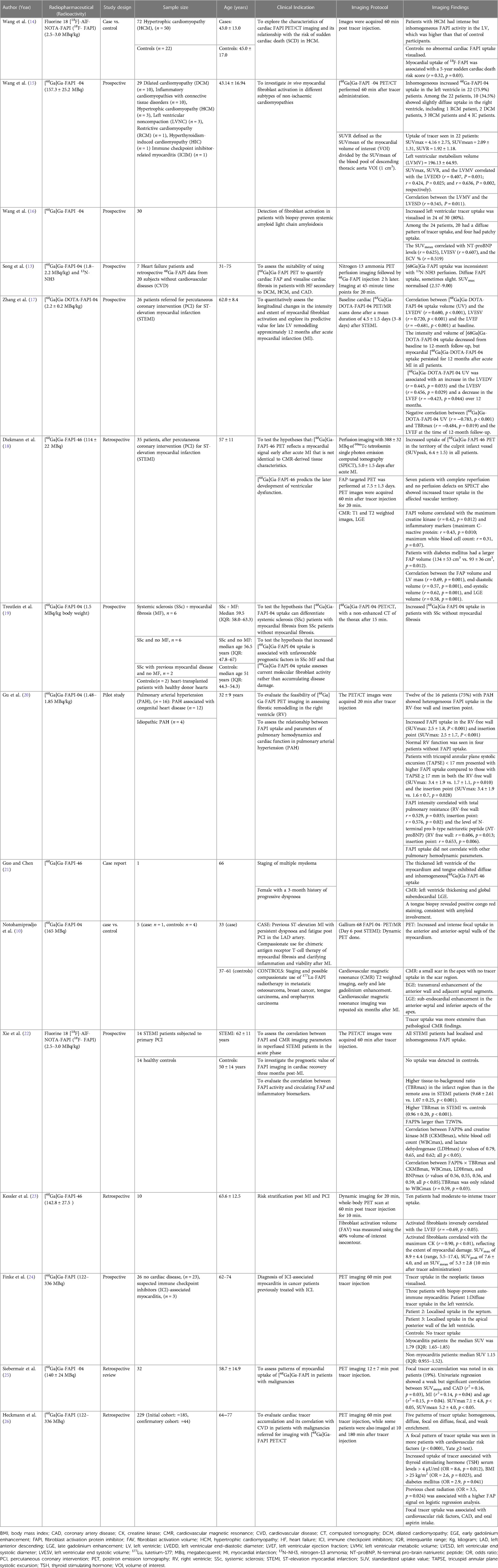
Table 1. Summary of original clinical research studies evaluating the uptake of fibrin-activating protein inhibitors in the myocardium of subjects with known or suspected cardiovascular disease.
4.1. Coronary artery disease
FAPI for ST-segment elevation myocardial infarction (STEMI) has been primarily used in patients with coronary artery disease. Zhang et al. studied 26 patients with STEMI after percutaneous coronary intervention referred for imaging with [68Ga]Ga-DOTA-FAPI Positron emission tomography/magnetic resonance imaging (PET/MRI) and found that both the volume and intensity of FAPI decreased over time when comparing the baseline and follow-up scan performed 12 months later. However, on the PET/MR images acquired 12 months after the acute myocardial infarction, FAPI uptake persisted in all patients studied (17). Similarly, in another study involving 35 patients with STEMI, [68Ga]Ga-DOTA-FAPI uptake was significantly elevated in the territory of the stenosed coronary artery (18).
Atherosclerotic coronary artery disease, presenting as chronic coronary or acute unstable syndromes, results from traditional risk factors such as hypertension and smoking, known to cause coronary vascular endothelial damage by inducing inflammation, as evidenced by elevated C-reactive protein plasma levels (27). In addition, atherosclerosis may occur as a secondary element of vascular inflammation, despite the absence of cardiovascular risk factors (28, 29). Acute coronary syndromes are precipitated by the thrombotic occlusion of an unstable, complicated atherosclerotic plaque. During myocardial ischaemia, the reduction in blood flow and subsequent delivery of oxygen to the heart muscle induces necrosis in the myocyte. Once the vessel is damaged, it attempts to “seal” the damaged area by depositing lipids and recruiting inflammatory cells (30). The diameter of the vessel where the atheroma is located narrows over time.
In response to myocardial ischaemia, a series of signalling mechanisms lead to the transformation of the structure of fibroblasts from the resting state to an activated proto-myofibroblast, which ultimately transforms into a myofibroblast (Figure 1).
Recurrent, small thrombotic non-occlusive ischaemic episodes and reperfusion or occlusive non-reperfused episodes are usually followed by the recruitment of macrophages and fibroblasts to the injured area of the myocardium or endocardium, a hallmark of myocardial fibrosis. As demonstrated by Zhang and colleagues, the persistent activation of fibroblasts may suggest the presence of underlying ventricular remodelling, in which the heart attempts to repair the infarcted area, or adverse ventricular remodelling, where the recruitment of fibroblasts will result in excessive deposition of collagen, which will eventually impair the contractility of the heart muscles.
Non-invasive fibroblast activation detection after acute myocardial infarction may identify areas of adverse ventricular remodelling depicted by persistent [68Ga]Ga-FAPI-04 uptake despite re-perfusion therapy. This persistent uptake has been demonstrated by Diekmann et al., who studied 35 patients with post acute STEMI. These patients were referred for imaging with single photon emission computed tomography (SPECT), PET, and CMR after percutaneous coronary intervention and dual-antiplatelet therapy (18). Despite receiving reperfusion therapy, [68Ga]Ga-FAPI-04 PET images showed uptake in the anterior and septal walls and, partially, in the apex (Figure 2)(18). The clinical significance of cardiac fibroblast activation after reperfusion therapy needs further exploration, as this finding may be indicative of adverse or expected ventricular remodelling after restoring myocardial perfusion.
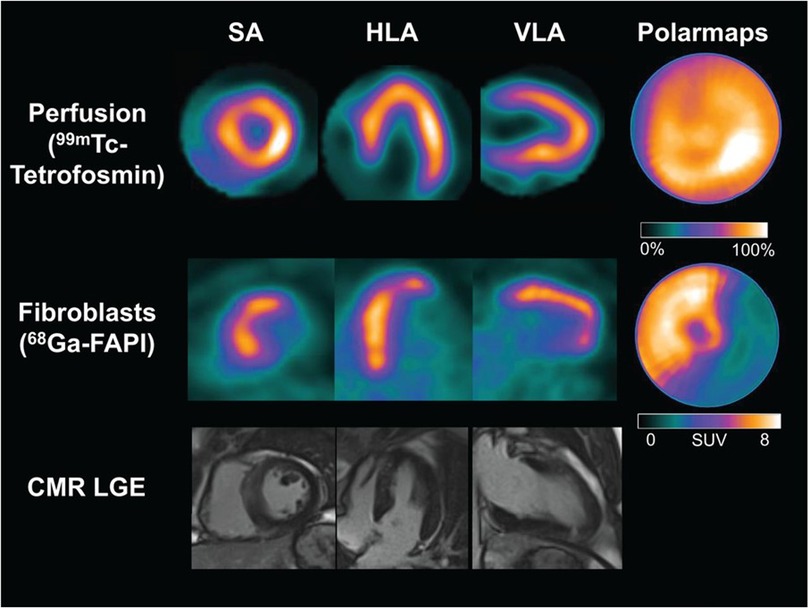
Figure 2. Cardiac images acquired after reperfusion therapy for acute myocardial infarction. The first row depicts single photon emission computed tomography (SPECT) myocardial perfusion images acquired at rest using [99mTc]Tc-tetrofosmin, followed by [68Ga]Ga-FAPI-04 PET images in the second row. The third row depicts cardiovascular magnetic resonance imaging. There are no perfusion defects visualised after reperfusion therapy in the SPECT resting perfusion images. However, a large area of cardiac fibroblast activation is visualised in the anterior and septal walls and partially in the apex. There is no evidence of late gadolinium enhancement in the corresponding myocardial images on CMR. HLA, horizontal long axis; SA, short axis; VLA, vertical long axis. This research was originally published in JNM. Johanna Diekmann et al. (18). © SNMMI.
4.2. Hypertrophic cardiomyopathy
Fibroblast activation has been studied in patients with dilated and hypertrophic cardiomyopathies (14, 15). In patients with hypertrophic cardiomyopathy (HCM), interstitial fibrosis is one of the typical histological features predisposing patients to arrhythmias and heart failure (31). Wang et al. performed PET imaging with Fluorine 18 [18F]-AlF-NOTA-FAPI (18F-FAPI) on 50 patients with HCM and 22 age and sex-matched healthy volunteers (14). They found intense and inhomogeneous tracer uptake in all patients with HCM, and the uptake was weakly correlated (r = 0.32) with a 5-year risk of sudden cardiac death (14). The potential role of FAPI-based tracers in HCM is for the selection of patients at high risk of sudden cardiac death (SCD), where an implantable cardioverter-defibrillator (ICD) may be implanted prophylactically to prevent lethal arrhythmias and heart failure in patients exhibiting FAPI uptake on imaging.
4.3. Amyloidosis
Amyloidosis is a systemic infiltrative disease characterised by the extracellular deposition of insoluble proteins in various organs, including the heart, and the abnormal accumulation of amyloid proteins in solid organs may lead to organ dysfunction (32). Non-invasive imaging modalities such as echocardiography and CMR imaging help to identify cardiac involvement in subjects with systemic amyloidosis (32). In a study involving 30 patients with biopsy-proven systemic light-chain amyloidosis, [68Ga]Ga-FAPI-04 PET/CT was used to assess cardiac fibroblast activation (16). Patchy and diffuse uptake patterns were found in 80% of patients, suggesting active cardiac remodelling (16). Gou and Chen explored the clinical utility of staging multiple myeloma with [68Ga]Ga-FAPI-04 PET/CT. Diffuse and inhomogeneous FAPI uptake was visualised in the left ventricle, suggesting cardiac amyloidosis (21). In patients with cardiac involvement, FAPI-based imaging may also play a role in identifying patients at risk for SCD requiring ICDs.
5. Assessment of myocardial fibrosis
Fibrosis in the heart indicates an area in the myocardium that cannot contract effectively, leading to myocardial contractile dysfunction, heart failure, a possible nidus for ventricular arrhythmias, and SCD (33–35). The endomyocardial biopsy allows for histological, immunohistochemical, and molecular evaluation of a specimen of cardiac tissue (36). Ideally, the biopsy should be performed under image guidance to increase the probability of sampling abnormal tissue. The major drawback of performing an endomyocardial biopsy is the limited access to cardiac catheterisation laboratories in most low-and middle-income countries.
Newer imaging techniques, such as the visualisation of late gadolinium enhancement and the quantification of the extracellular volume using CMR imaging, have proven to be helpful in identifying fibrotic tissue in patients with ischaemic and non-ischaemic dilated cardiomyopathy (37–39). In research settings, human cardiac tissue has been excised during coronary artery bypass graft (CABG) surgery, left ventricular assist device implantation, and cardiac transplant surgery to assess for micro- and macroscopic evidence of myocardial fibrosis (40). The characteristics of non-invasive imaging methods for evaluating myocardial fibrosis are summarised in Table 2.
6. Future Studies and Recommendations
Cardiac fibroblast activation has been studied in patients with various CVDs, mostly in patients with ischaemic heart disease. Whether the visualisation of FAPI uptake on the myocardium suggests the presence of an underlying normal response to myocardial injury or adverse remodelling remains to be elucidated. Future studies should attempt to perform non-invasive imaging at multiple time points and correlate imaging findings with inflammatory markers. The frequency of imaging could be extrapolated from animal models. In addition, subjects known to have coronary artery diseases should be subjected to coronary angiography and subsequently randomised to imaging with FAPI-based tracers and CMR imaging to evaluate the clinical impact of FAPI in defining clinically relevant outcomes such as cardiovascular death, all-cause mortality, and the rate of rehospitalisation. Furthermore, considering that the activation of fibroblasts is a momentary phase in the life cycle of fibroblasts, the window of opportunity for intervening should be clearly defined. Moreover, the utility of FAPI-based tracers could be further explored in patients with chronic coronary syndromes, potentially selecting candidates for coronary revascularisation.
7. Conclusions
Evidence supporting the application of FAPI-based radiopharmaceuticals in cardiac diseases is still in its infancy, comprising data collated from original research studies with small sample sizes, case reports, and retrospective studies on patients with oncological conditions. After a myocardial injury, the heart attempts to repair the damaged tissue and maintain its structural integrity by orchestrating a series of processes, including the deposition of extracellular matrix components and the activation of fibroblasts. Unresolved pertinent issues related to imaging activated fibroblasts include the appropriate timing for imaging and the need for a definitive management plan in patients with FAPI uptake in the myocardium.
Author contributions
Conceptualisation of the study: DM, MS, and NT. Literature review: DM, MS, SM, and JD. All authors contributed to the article and approved the submitted version. DM wrote the first draft of the manuscript. NT, MS, EK, SM, JD, BH, and MV edited the manuscript.
Acknowledgments
Figure 1 was created with Biorender software. The authors would like to thank the Society of Nuclear Medicine and Molecular Imaging for permission to reuse Figure 2 was originally published in the Journal of Nuclear Medicine by Johanna Diekmann et al. Cardiac Fibroblast Activation in Patients Early After Acute Myocardial Infarction: Integration with MR Tissue Characterization and Subsequent Functional Outcome. J Nucl Med 2022;63:1415-1423. © SNMMI
Conflict of Interest
The authors declare that the research was conducted in the absence of any commercial or financial relationships that could be construed as a potential conflict of interest.
The author MS declared that they were an editorial board member of Frontiers at the time of submission. This had no impact on the peer review process or the final decision.
Publisher's note
All claims expressed in this article are solely those of the authors and do not necessarily represent those of their affiliated organizations, or those of the publisher, the editors and the reviewers. Any product that may be evaluated in this article, or claim that may be made by its manufacturer, is not guaranteed or endorsed by the publisher.
References
1. Kalluri R. The biology and function of fibroblasts in cancer. Nat Rev Cancer. (2016) 16(9):582–98. doi: 10.1038/nrc.2016.73
2. Varasteh Z, Mohanta S, Robu S, Braeuer M, Li Y, Omidvari N, et al. Molecular imaging of fibroblast activity after myocardial infarction using a (68)Ga-labeled fibroblast activation protein inhibitor, FAPI-04. J Nucl Med. (2019) 60(12):1743–9. doi: 10.2967/jnumed.119.226993
3. Lindner T, Loktev A, Giesel F, Kratochwil C, Altmann A, Haberkorn U. Targeting of activated fibroblasts for imaging and therapy. EJNMMI Radiopharm Chem. (2019) 4(1):16. doi: 10.1186/s41181-019-0069-0
4. Sollini M, Kirienko M, Gelardi F, Fiz F, Gozzi N, Chiti A. State-of-the-art of FAPI-PET imaging: a systematic review and meta-analysis. Eur J Nucl Med Mol Imaging. (2021) 48(13):4396–414. doi: 10.1007/s00259-021-05475-0
5. Liermann J, Syed M, Ben-Josef E, Schubert K, Schlampp I, Sprengel SD, et al. Impact of FAPI-PET/CT on target volume definition in radiation therapy of locally recurrent pancreatic cancer. Cancers (Basel). (2021) 13(4):1–13. doi: 10.3390/cancers13040796
6. Lindner T, Loktev A, Altmann A, Giesel F, Kratochwil C, Debus J, et al. Development of quinoline-based theranostic ligands for the targeting of fibroblast activation protein. J Nucl Med. (2018) 59(9):1415–22. doi: 10.2967/jnumed.118.210443
7. Huang R, Pu Y, Huang S, Yang C, Yang F, Pu Y, et al. FAPI-PET/CT in cancer imaging: a potential novel molecule of the century. Front Oncol. (2022) 12:854658. doi: 10.3389/fonc.2022.854658
8. Alfteimi A, Lutzen U, Helm A, Juptner M, Marx M, Zhao Y, et al. Automated synthesis of [(68)Ga]Ga-FAPI-46 without pre-purification of the generator eluate on three common synthesis modules and two generator types. EJNMMI Radiopharm Chem. (2022) 7(1):20. doi: 10.1186/s41181-022-00172-1
9. Lyu Z, Han W, Zhao H, Jiao Y, Xu P, Wang Y, et al. A clinical study on relationship between visualization of cardiac fibroblast activation protein activity by Al(18)F-NOTA-FAPI-04 positron emission tomography and cardiovascular disease. Front Cardiovasc Med. (2022) 9:921724. doi: 10.3389/fcvm.2022.921724
10. Notohamiprodjo S, Nekolla SG, Robu S, Villagran Asiares A, Kupatt C, Ibrahim T, et al. Imaging of cardiac fibroblast activation in a patient after acute myocardial infarction using (68)Ga-FAPI-04. J Nucl Cardiol. (2022) 29(5):2254–61. doi: 10.1007/s12350-021-02603-z
11. Windisch P, Zwahlen DR, Giesel FL, Scholz E, Lugenbiel P, Debus J, et al. Clinical results of fibroblast activation protein (FAP) specific PET for non-malignant indications: systematic review. EJNMMI Res. (2021) 11(1):18. doi: 10.1186/s13550-021-00761-2
12. Qiao P, Wang Y, Zhu K, Zheng D, Song Y, Jiang D, et al. Noninvasive monitoring of reparative fibrosis after myocardial infarction in rats using (68)Ga-FAPI-04 PET/CT. Mol Pharm. (2022) 19(11):4171–8. doi: 10.1021/acs.molpharmaceut.2c00551
13. Song W, Zhang X, He S, Gai Y, Qin C, Hu F, et al. (68)Ga-FAPI PET visualize heart failure: from mechanism to clinic. Eur J Nucl Med Mol Imaging. (2022) 50:475–85. doi: 10.1007/s00259-022-05994-4
14. Wang L, Wang Y, Wang J, Xiao M, Xi XY, Chen BX, et al. Myocardial activity at (18)F-FAPI PET/CT and risk for sudden cardiac death in hypertrophic cardiomyopathy. Radiology. (2023) 306(2):e221052. doi: 10.1148/radiol.221052
15. Wang J, Huo L, Lin X, Fang L, Hacker M, Niu N, et al. Molecular imaging of fibroblast activation in multiple non-ischemic cardiomyopathies. EJNMMI Res. (2023) 13(1):39. doi: 10.1186/s13550-023-00986-3
16. Wang X, Guo Y, Gao Y, Ren C, Huang Z, Liu B, et al. Feasibility of (68)Ga-labeled fibroblast activation protein inhibitor PET/CT in light-chain cardiac amyloidosis. JACC Cardiovasc Imaging. (2022) 15(11):1960–70. doi: 10.1016/j.jcmg.2022.06.004
17. Zhang M, Quan W, Zhu T, Feng S, Huang X, Meng H, et al. [(68)Ga]ga-DOTA-FAPI-04 PET/MR in patients with acute myocardial infarction: potential role of predicting left ventricular remodeling. Eur J Nucl Med Mol Imaging. (2023) 50(3):839–48. doi: 10.1007/s00259-022-06015-0
18. Diekmann J, Koenig T, Thackeray JT, Derlin T, Czerner C, Neuser J, et al. Cardiac fibroblast activation in patients early after acute myocardial infarction: integration with MR tissue characterization and subsequent functional outcome. J Nucl Med. (2022) 63(9):1415–23. doi: 10.2967/jnumed.121.263555
19. Treutlein C, Distler JHW, Tascilar K, Fakhouri SC, Gyorfi AH, Atzinger A, et al. Assessment of myocardial fibrosis in patients with systemic sclerosis using [(68)Ga]ga-FAPI-04-PET-CT. Eur J Nucl Med Mol Imaging. (2023) 50(6):1629–35. doi: 10.1007/s00259-022-06081-4
20. Gu Y, Han K, Zhang Z, Zhao Z, Yan C, Wang L, et al. (68)Ga-FAPI PET/CT for molecular assessment of fibroblast activation in right heart in pulmonary arterial hypertension: a single-center, pilot study. J Nucl Cardiol. (2023) 30(2):495–503. doi: 10.1007/s12350-022-02952-3
21. Guo W, Chen H. (68)Ga FAPI PET/MRI in cardiac amyloidosis. Radiology. (2022) 303(1):51. doi: 10.1148/radiol.211951
22. Xie B, Wang J, Xi XY, Guo X, Chen BX, Li L, et al. Fibroblast activation protein imaging in reperfused ST-elevation myocardial infarction: comparison with cardiac magnetic resonance imaging. Eur J Nucl Med Mol Imaging. (2022) 49(8):2786–97. doi: 10.1007/s00259-021-05674-9
23. Kessler L, Kupusovic J, Ferdinandus J, Hirmas N, Umutlu L, Zarrad F, et al. Visualization of fibroblast activation after myocardial infarction using 68Ga-FAPI PET. Clin Nucl Med. (2021) 46(10):807–13. doi: 10.1097/RLU.0000000000003745
24. Finke D, Heckmann MB, Herpel E, Katus HA, Haberkorn U, Leuschner F, et al. Early detection of checkpoint inhibitor-associated myocarditis using (68)Ga-FAPI PET/CT. Front Cardiovasc Med. (2021) 8:614997. doi: 10.3389/fcvm.2021.614997
25. Siebermair J, Köhler MI, Kupusovic J, Nekolla SG, Kessler L, Ferdinandus J, et al. Cardiac fibroblast activation detected by ga-68 FAPI PET imaging as a potential novel biomarker of cardiac injury/remodeling. J Nucl Cardiol. (2021) 28(3):812–21. doi: 10.1007/s12350-020-02307-w
26. Heckmann MB, Reinhardt F, Finke D, Katus HA, Haberkorn U, Leuschner F, et al. Relationship between cardiac fibroblast activation protein activity by positron emission tomography and cardiovascular disease. Circ Cardiovasc Imaging. (2020) 13(9):e010628. doi: 10.1161/circimaging.120.010628
27. Sesso HD, Buring JE, Rifai N, Blake GJ, Gaziano JM, Ridker PM. C-reactive protein and the risk of developing hypertension. JAMA. (2003) 290(22):2945–51. doi: 10.1001/jama.290.22.2945
28. Avina-Zubieta JA, Choi HK, Sadatsafavi M, Etminan M, Esdaile JM, Lacaille D. Risk of cardiovascular mortality in patients with rheumatoid arthritis: a meta-analysis of observational studies. Arthritis Rheum. (2008) 59(12):1690–7. doi: 10.1002/art.24092
29. Tsabedze N, Seboka M, Mpanya D, Solomon A. Extensive triple vessel coronary artery disease in a young male with juvenile idiopathic arthritis. Oxf Med Case Rep. 2021;2021(11-12):omab119. doi: 10.1093/omcr/omab119
30. Falk E. Pathogenesis of atherosclerosis. J Am Coll Cardiol. 2006;47(8 Suppl):C7–12. doi: 10.1016/j.jacc.2005.09.068
31. Marian AJ, Braunwald E. Hypertrophic cardiomyopathy: genetics, pathogenesis, clinical manifestations, diagnosis, and therapy. Circ Res. (2017) 121(7):749–70. doi: 10.1161/CIRCRESAHA.117.311059
32. Muchtar E, Dispenzieri A, Magen H, Grogan M, Mauermann M, McPhail ED, et al. Systemic amyloidosis from A (AA) to T (ATTR): a review. J Intern Med. (2021) 289(3):268–92. doi: 10.1111/joim.13169
33. Disertori M, Rigoni M, Pace N, Casolo G, Mase M, Gonzini L, et al. Myocardial fibrosis assessment by LGE is a powerful predictor of ventricular tachyarrhythmias in ischemic and nonischemic LV dysfunction: a meta-analysis. JACC Cardiovasc Imaging. (2016) 9(9):1046–55. doi: 10.1016/j.jcmg.2016.01.033
34. Ekstrom K, Lehtonen J, Kandolin R, Raisanen-Sokolowski A, Salmenkivi K, Kupari M. Incidence, risk factors, and outcome of life-threatening ventricular arrhythmias in giant cell myocarditis. Circ Arrhythm Electrophysiol. (2016) 9(12):1–8. doi: 10.1161/CIRCEP.116.004559
35. Mc LA, Ellims AH, Prabhu S, Voskoboinik A, Iles LM, Hare JL, et al. Diffuse ventricular fibrosis on cardiac magnetic resonance imaging associates with ventricular tachycardia in patients with hypertrophic cardiomyopathy. J Cardiovasc Electrophysiol. (2016) 27(5):571–80. doi: 10.1111/jce.12948
36. Porcari A, Baggio C, Fabris E, Merlo M, Bussani R, Perkan A, et al. Endomyocardial biopsy in the clinical context: current indications and challenging scenarios. Heart Fail Rev. (2023) 28(1):123–35. doi: 10.1007/s10741-022-10247-5
37. Memon S, Ganga HV, Kluger J. Late gadolinium enhancement in patients with nonischemic dilated cardiomyopathy. Pacing Clin Electrophysiol. (2016) 39(7):731–47. doi: 10.1111/pace.12873
38. Kuruvilla S, Adenaw N, Katwal AB, Lipinski MJ, Kramer CM, Salerno M. Late gadolinium enhancement on cardiac magnetic resonance predicts adverse cardiovascular outcomes in nonischemic cardiomyopathy. Circ Cardiovasc Imaging. (2014) 7(2):250–8. doi: 10.1161/CIRCIMAGING.113.001144
39. Raman K S, Nucifora G, Leong DP, Marx C, Shah R, Woodman RJ, et al. Long term prognostic importance of late gadolinium enhancement in first-presentation non-ischaemic dilated cardiomyopathy. Int J Cardiol. (2019) 280:124–9. doi: 10.1016/j.ijcard.2019.01.018
40. Krul SP, Berger WR, Smit NW, van Amersfoorth SC, Driessen AH, van Boven WJ, et al. Atrial fibrosis and conduction slowing in the left atrial appendage of patients undergoing thoracoscopic surgical pulmonary vein isolation for atrial fibrillation. Circ Arrhythm Electrophysiol. (2015) 8(2):288–95. doi: 10.1161/CIRCEP.114.001752
41. Dendl K, Koerber SA, Kratochwil C, Cardinale J, Finck R, Dabir M, et al. FAP And FAPI-PET/CT in malignant and non-malignant diseases: a perfect symbiosis? Cancers (Basel). (2021) 13(19):1–17. doi: 10.3390/cancers13194946
42. Celiker-Guler E, Ruddy TD, Wells RG. Acquisition, processing, and interpretation of PET (18)F-FDG viability and inflammation studies. Curr Cardiol Rep. (2021) 23(9):124. doi: 10.1007/s11886-021-01555-7
43. Fathala A. Myocardial perfusion scintigraphy: techniques, interpretation, indications and reporting. Ann Saudi Med. (2011) 31(6):625–34. doi: 10.4103/0256-4947.87101
44. Gupta S, Ge Y, Singh A, Grani C, Kwong RY. Multimodality imaging assessment of myocardial fibrosis. JACC Cardiovasc Imaging. (2021) 14(12):2457–69. doi: 10.1016/j.jcmg.2021.01.027
Keywords: gallium-68, fibroblast activation protein, positron emission tomography, cardiovascular disease, myocardial injury, fibrosis
Citation: Mpanya D, Sathekge M, Klug E, Damelin J, More S, Hadebe B, Vorster M and Tsabedze N (2023) Gallium-68 fibroblast activation protein inhibitor positron emission tomography in cardiovascular disease. Front. Nucl. Med. 3:1224905. doi: 10.3389/fnume.2023.1224905
Received: 18 May 2023; Accepted: 29 June 2023;
Published: 27 July 2023.
Edited by:
Jasna Milos Mihailovic, University of Novi Sad, SerbiaReviewed by:
Raluca Mititelu, Central University Emergency Military Hospital Bucharest Romania, RomaniaPavel Ivanovich Krzhivitsky, N.N. Petrov National Medical Research Center of Oncology, Russia
© 2023 Mpanya, Sathekge, Klug, Damelin, More, Hadebe, Vorster and Tsabedze. This is an open-access article distributed under the terms of the Creative Commons Attribution License (CC BY). The use, distribution or reproduction in other forums is permitted, provided the original author(s) and the copyright owner(s) are credited and that the original publication in this journal is cited, in accordance with accepted academic practice. No use, distribution or reproduction is permitted which does not comply with these terms.
*Correspondence: Dineo Mpanya, ZGluZW8ubXBhbnlhQHdpdHMuYWMuemE=