- 1Department of Radiology/Nuclear Medicine, Northwell Health, New York, NY, United States
- 2Division of Arts and Science, Texas A&M University at Qatar, Doha, Qatar
- 3Department of Electronics and Information Systems, Medical Image and Signal Processing (MEDISIP), Ghent University, Ghent, Belgium
- 4Qatar Computing Research Institute, Hamad Bin Khalifa University, Doha, Qatar
- 5Siemens Medical Solutions, Malvern, PA, United States
- 6Department of Radiology & Radiological Sciences, Medical University of South Carolina, Charleston, SC, United States
- 7Radiography and Diagnostic Imaging, University College Dublin, Dublin, Ireland
Fibroblast activation protein (FAP) is a type-II membrane bound glycoprotein specifically expressed by activated fibroblasts almost exclusively in pathological conditions including arthritis, fibrosis and cancer. FAP is overexpressed in cancer-associated fibroblasts (CAFs) located in tumor stroma, and is known to be involved in a variety of tumor-promoting activities such as angiogenesis, proliferation, resistance to chemotherapy, extracellular matrix remodeling and immunosuppression. In most cancer types, higher FAP expression is associated with worse clinical outcomes, leading to the hypothesis that FAP activity is involved in cancer development, cancer cell migration, and cancer spread. Recently, various high selectivity FAP inhibitors (FAPIs) have been developed and subsequently used for positron emission tomography (PET) imaging of different pathologies. Considering the paucity of widely available and especially mainstream reliable radioligands in brain cancer PET imaging, and the poor survival rates of patients with certain types of brain cancer such as glioblastoma, FAPI-PET represents a major development in enabling the detection of small primary or metastatic lesions in the brain due to its biological characteristics and low background accumulation. In this work, we aim to summarize the potential avenues for use of FAPI-PET, from the basic biological processes to oncologic imaging and with a main focus on brain imaging.
1. Introduction
Brain tumors are frequently associated with poor outcomes, with a 5-year relative survival for all malignant brain tumors of around 36%, and 7% specifically for glioblastoma (1). Imaging plays a major role in guiding treatment and is indispensable in medical decision making. Magnetic resonance imaging (MRI) performs relatively well, however presents limitations in evaluation of tumor boundaries and post-treatment changes which can be challenging to estimate accurately (2). There are also limits in determining viable tumor from surrounding inflammatory and non-inflammatory changes (i.e., due to post treatment effects from therapeutic interventions: i.e., surgery, chemotherapy or radiation) (3). Contrast-enhanced MRI is mostly a function of blood-brain-barrier (BBB) integrity and tumor vascularity and thus is a non-specific form of tumor mass characterization prone to equivocal interpretation. Accurate assessment of various tumor characteristics and parameters is fundamental to improve management and outcomes.
Positron emission tomography (PET) has been used in the evaluation of brain tumors for many years (4), showing utility in several instances such as improving delineation of tumor boundaries for surgery and radiotherapy planning (5), differentiating between tumor progression and treatment-related changes (6), monitoring early/late therapy response (7), and identification of favorable biopsy sites (8). Historically, evaluation of glucose metabolic rate (18F-FDG) has been used in assessing brain tumors, with sensitivity being hindered by elevated normal background uptake of 18F-FDG, as well as increased glucose usage due to inflammatory responses potentially independent to the tumor response or growth.
As PET evolves, 18F-FDG is slowly becoming supplanted by amino-acid imaging (when available), with radioligands such as 11C-MET, 18F-FET, and 18F-DOPA (9). Along with the crucial advantage that amino acid tracers can easily permeate across the BBB (whether the BBB is compromised or not), this pathway is thus more specific in highlighting “live” proliferating cells rather than structural changes. On the other hand, FAPI ligands do not cross the BBB and hence may offer an additional complementary representation of tumor characteristics (10, 11). Other amino acid radioligands such as α-[11C]-methyl-l-tryptophan (AMT) and 18F-Fluciclovine (FACBC), as well as glutamine-based amino acids are under evaluation for their potential in tumor characterization, prognostication, viable volume delineation and detection of recurrence in brain tumors and metastases (12).
Other uptake pathways have been explored such as the thymidine nucleoside analogue using i.e., (18F-FLT), which reflects cellular proliferation (13). 18F-FLT is dependent on disruption of the BBB for successful tracer uptake, and thus is a limitation compared to amino acid-based tracers. Hypoxia imaging ligands (18F-FMISO, 18F-FAZA, 18F-HX4, 64Cu-ATSM) have also displayed limitations such as a low target-to-background ratio, poor clearance times, and none have made a successful translation to routine clinical practice (14). Considering neuroinflammation has been explored as a hallmark of several neurological diseases, it has also been postulated to be an important element of neuro-oncology assessments. Neuroinflammation investigations has included identification of elevated expression of translocator protein (TSPO) in glioblastoma (GBM), a primary brain tumor with a high level of aggressiveness, in part due to neuroinflammation and microglial activation hinting at a pivotal role of TSPO in tumorigenesis and progression (15). Radiotracers such as 11C-PK11195, 18F-DPA-714 and 18F-GE-180 show promising results (16–18), but lack large scale clinical trials to prove efficacy. Other uptake routes such as poly(ADP ribose) polymerase (PARP) enzymes which are critical for DNA repair, are under development. In comparison to amino acid-based tracers, PARP tracers have demonstrated good uptake in brain tumors with a lower background uptake in the cortex (19), and preclinical investigations of 3 different PARP radioligands (18F-PARPi, 18F-FTT and 18F-FPyPARP) investigated imaging pharmacokinetics (20), however still require small molecules to cross the BBB. Chemokine receptor-4 (CXCR4) have been shown to exhibit affinity for glioma cells, and tracers such as 68Ga-Pentixafor have demonstrated higher tumor uptake compared to amino acid tracers such as 18F-FET (21). Use of somatostatin receptor (SSTR) targeting for treating brain tumors has also been investigated (using 90Y-DOTATOC) (22), although remains investigational. Studies have also confirmed prostate specific membrane antigen (PSMA) expression in the tumoral vessels of high-grade gliomas (23). With the advent of low background uptake in the normal brain tissue and a high tumor-to-brain ratio of 68Ga-PSMA and a theranostic pair of 177Lu-PSMA, recent efforts have been made to exploit this mechanism for treatment, showing good imaging characteristics but a limited use of 177Lu-PSMA (24).
In lieu of the aforementioned, imaging the tumor microenvironment can be an essential tool in the evaluation of these patients as it gives a complete picture of the patient's disease status at diagnosis and throughout the patient care continuum. This has proven to be extremely useful with novel ligands such as fibroblast activated protein inhibitors (FAPI) PET (25).
FAPI-PET has been shown to be very useful in many non-oncological indications (26), including:
• Cardiovascular diseases (27–29): FAPI PET has been assessed in patients with myocardial infarction, myocardial fibrosis and metabolic syndrome (27, 30–33).
• Immune mediated diseases such as Crohn's disease or Rheumatoid Arthritis (34–36)
• FAPI PET is undoubtedly a promising future PET radiopharmaceutical for solid tumors in various cancers (37–47).
Taking into consideration the great potential of FAPI-PET and taking note of the fact that the tumor microenvironment has also been evaluated with FAPI-PET in brain tumors (48–50). We propose to review the benefits, limitations, and potential indications of FAPI PET in brain tumors.
2. Background: fibroblasts and imaging
2.1. The tumor microenvironment
Many tumor types are characterized by a strong desmoplastic reaction, i.e., they are subject to causing or forming adhesions or fibrous connective tissue within a tumor. Tumors can be considered to comprise two parts, the malignant cells and the stroma (containing components such as basement membrane, fibroblasts, extracellular matrix, immune cells, and vasculature), also known collectively as the tumor microenvironment. While tumor microenvironment may vary with different tumor types, a hallmark feature includes a tumor mass with up to 90% of cancer associated fibroblasts (CAFs—a cell that synthesizes extracellular matrix & collagen and produces the structural tissue framework) and extracellular fibrosis, leaving the original tumor cells in the minority (51–53).
2.2. Fibroblast activation protein-α (FAP) characteristics
Fibroblast activation protein-ɑ (FAP) is a type II transmembrane glycoprotein, specifically expressed on the surface of activated fibroblasts. It is a 760 amino acid serine protease, where the active site is localized in the extracellular part of the protein, and is catalytically active as a 170 kD homodimer. Normal fibroblasts have shown low FAP expression in normal human tissues, however in activated fibroblasts, expression of FAP is increased in the presence of tissue damage, remodeling, or inflammation (54). Furthermore, FAP expression is seen on activated CAFs of more than 90% of epithelial carcinomas (54). Through crosstalk with surrounding cells of the tumor microenvironment, CAFs play an essential role in tumor proliferation, metastasis, neoangiogenesis, extracellular matrix remodeling and immunosuppression (37).
2.3. Molecular imaging of FAP
Differential expression of FAP in normal tissue compared with tumors or inflammation allows FAP to become a candidate for molecular imaging, which can be performed by linking FAP inhibitors (FAPIs), peptides or antibodies to radionuces using chelators for use in PET/CT, PET/MR, SPECT/CT or SPECT/MR imaging. Through the development of FAPIs, the exploration of FAPI-PET imaging and FAPI-based radioligand therapy for different tumor types and some non-oncological diseases is gaining momentum (38, 39). Various derivatives of FAPI have been developed with the aim to improve affinity for FAP and demonstrate better pharmacokinetic properties. Examples include FAPI-02 (40), FAPI-04 (41), DATA5m.SA.FAPI (42), and DOTA-2P(FAPI)2 (43) which have demonstrated promising results in clinical studies, and other derivatives are at preclinical imaging stages, such as 18F-fluoroglycosylation-FAPI (44), QCP01, QCP02 (45), and AAZTA5.SA.FAPI (46). The most common radioisotopes used in clinical FAPI imaging development are 18F and 68Ga, with 99mTc ligands also being recently developed for situations where PET imaging is not available. 68Ga labeled FAPIs provide the more favorable imaging features in terms of high detection rate in a variety of tumors, even in cases considered to be challenging for 18F-FDG PET (47).
3. FAPI PET in oncology
A recent review summarized many of the most common FAPIs used in clinical oncological research studies (55) and some examples of clinical studies are detailed in Table 1. Much research is focused on chemical modification of inhibitors to obtain derivatives with higher affinity for FAP, in turn improving imaging characteristics and pharmacokinetics, with a recent paper evaluating 11 derivatives in animal models (56).
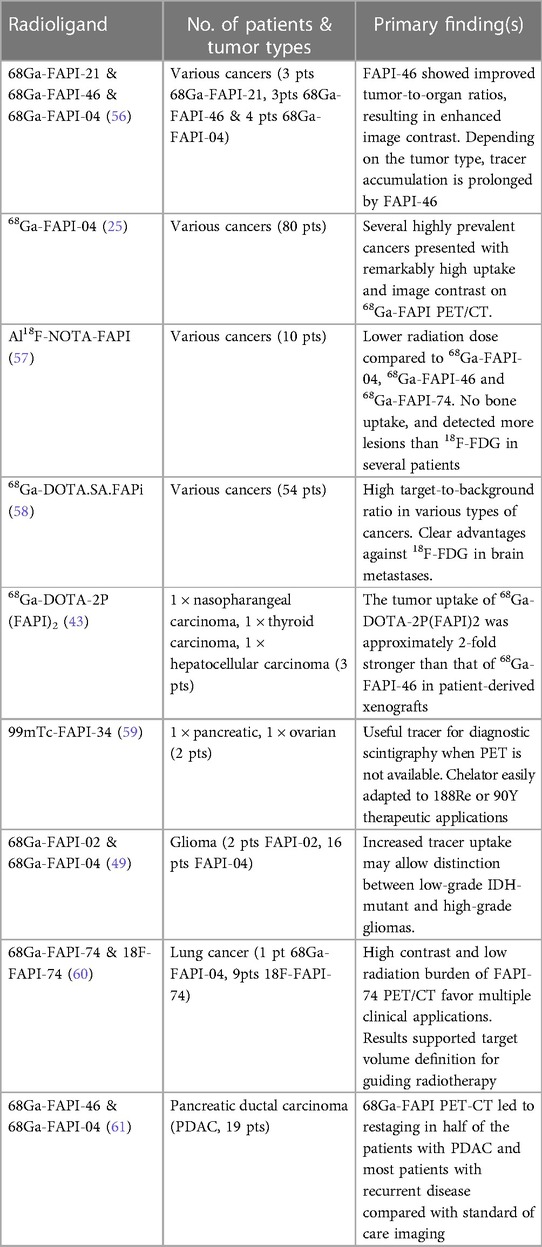
Table 1. Summary of some FAP inhibitors used in a selection of clinical oncology research studies. It should be noted that FAPI-PET has so far been explored primarily in single-center studies, in heterogeneous and small cohorts of oncology patients.
It serves to summarize some of the results from Table 1. A retrospective analysis of 80 patients (28 different cancers) demonstrated high uptake of 68Ga-FAPI-04 with low background uptake and determined that tumor-to-background contrast ratios were between 3-fold and 6-fold higher depending on a low, medium and high uptake group (25). Related work showed that FAPI-PET in pancreatic carcinomas leads to significant changes in tumor staging compared to routine contrast enhanced CT (CECT) (61), with 7 of the 19 patient cohort having changes in treatment management (i.e., alteration of treatment type, treatment intent or changes within a treatment regime).
3.1. Radiation dosimetry and uptake time
Regarding radiation dosimetry and imaging times in FAPI-PET, a recent study of 10 lung cancer patients, longitudinal PET scans of 18F-FAPI-74 demonstrated an ideal imaging time-point of 1 h post-injection and a good radiation dosimetry profile (effective dose = 1.4 + −0.2 mSv) (60). A related biodistribution study showed good efficacy with 18F-NOTA-FAPI, demonstrating low physiological bone uptake, and was able to detect more lesions than 18F-FDG in several patients also with 1 h uptake (57). Another study directly compared 18F-FDG to 68Ga-DOTA.SA.FAPi imaging in 54 patients (14 types of cancers), also showing a good radiation dosimetry profile (effective dose = 0.011 mSv/MBq), and demonstrated a higher contrast to noise in comparison to the 18F-FDG images for certain cancers and a similar uptake time (58). Imaging of 68Ga-DOTA-2P(FAPI) in 3 patients also demonstrated an effective dose = 0.019 mSv/MBq. In preclinical studies, tumor uptake of 68Ga-DOTA-2P(FAPI) was approximately 2-fold higher than that of 68Ga-FAPI-46 in patient-derived xenografts. Results in patients demonstrated a significantly higher tumor uptake of 68Ga-DOTA-2P(FAPI)2 than of 68Ga-FAPI46 in all tumor lesions (SUVmax, 8.1–39.0 vs. 1.7–24.0, respectively) at similar post-injection imaging times (57). Although research studies utilize a 1 h uptake time for the majority of FAPI-PET agents, serial timepoint imaging in brain FAPI-PET is still commonly performed in order to evaluate an ideal imaging timepoint (63).
4. FAPI-PET in neuro-oncology
4.1. Rationale
Although brain tumor PET can also be performed using radioligands exploring a host of other uptake routes such as amino acids, somatostatin receptors, TSPO, CXCR4, PSMA and hypoxia, so far there are no specific clinical studies involving a direct comparison of FAPI-PET to non-FDG radioligands in the brain. A range of studies have shown a significantly lower FAPI background distribution in the brain relative to other radiotracers evaluating brain tumors, especially 18F-FDG (62–64). This makes FAPI a promising radiotracer in the detection and evaluation of tumors and lesions naturally exhibiting low to moderate uptake of 18F-FDG including primary and secondary/metastatic brain lesions as well as post-treatment changes. Favorable early clinical trial results demonstrate the potential for FAPI to outperform or even complement other radiotracers.
4.2. Protocol
In FAPI-PET for neuro-oncology applications, the protocol consists of various aspects: patient preparation, radiopharmaceutical preparation, performing imaging and subsequent image analysis & reporting findings.
a. No specific patient preparation is required other than adequate hydration and voiding in order to decrease the radiation exposure to the patient.
b. FAPI radiopharmaceutical is radio-synthesized, whereby FAPI is coupled to a radionuclide of choice (most commonly 68Ga or 18F) via a chelator to produce the radiopharmaceutical. A range of experimental radiotracers exist, the use of which are institution dependent until regulatory approval of specific FAPI-PET agents (see Table 1). Labeling with 18F has also been performed, solving logistical issues with the short half-life of 68Ga, improving transport availability/distribution, automated radiochemistry, and also improving the image spatial resolution due to a shorter positron pathlength.
c. Patient is administered the radiopharmaceutical intravenously. Uptake phase varies from 15 to 60 min, with images frequently acquired around 30–60 min post-administration of the tracer. Highest contrast was achieved in primary tumors, lymph nodes, and distant metastases at 1 h after injection with many FAPI radioligands, but should be optimized to the specific scanner used and ideally chosen based on published data specifically to the radioligand of interest (50).
d. Literature demonstrates that administered activities for clinical cohorts are within 200–300 MBq for all 18F and 68Ga FAPI radioligands, both with favorable radiation dosimetry and good image quality. Image reconstruction varies widely depending on the PET scanner used, and routine clinical parameters for either 18F or 68Ga radioligands currently should be used as a first iteration.
e. Interpretative criteria include traditional qualitative visual assessments with positivity defined as uptake above background. Semi-quantitative methods using standardized uptake values (SUV) measurements and corrections compared to contralateral cerebral uptake have been described.
4.3. Diagnosis
In a recent first-in-man trial comparing 18F-FDG to 68Ga-DOTA.SA.FAPi imaging in a cohort of patients with secondary brain metastases, quantification of tracer uptake demonstrated higher image contrast with better SULpeak (peak lean body mass corrected SUV) and SULavg (average lean body mass corrected SUV) and higher brain metastases-to normal brain parenchyma ratios observed on 68Ga-DOTA.SA.FAPi compared to 18F-FDG (SULpeak: FAPI 34.2–249.3 vs. FDG 1–2.3 and SULavg: FAPi: 33.5–130.8 vs. FDG: 1–2.3) (58). Furthermore, they demonstrated in 2 patients that 68Ga-DOTA.SA.FAPi identified additional lesions in the brain that could not be detected on 18F-FDG PET/CT, likely due to the lower normal brain parenchyma uptake of 68GaDOTA.SA.FAPi. Figure 1 demonstrates a comparison of 68Ga-DOTA.SA.FAPi to 18F-FDG in the same patient. A recent paper using 68Ga-FAPI-04 in a single patient with lung cancer demonstrated the ability to observe 2 brain metastases unobserved in CT images, thereby changing the patients staging and subsequent management, clearly showing the potential power of its diagnostic ability (65).
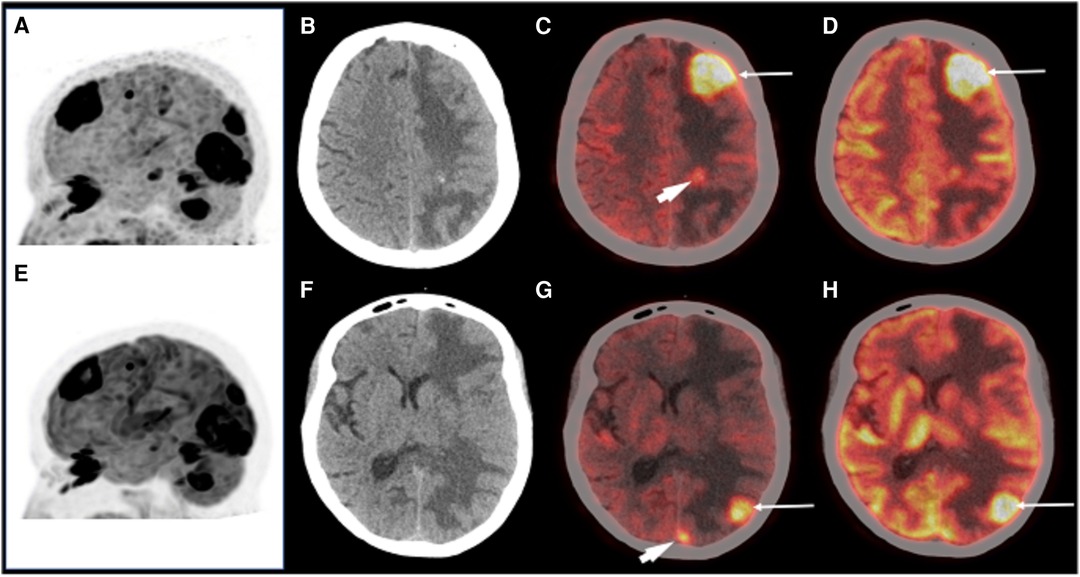
Figure 1. A patient with brain metastases imaged with 68Ga-DOTA.SA.FAPi (A,C,G) and 18F-FDG (E,D,H) for restaging. MIP images (A,E) showing multiple foci of intense tracer uptake in both. Axial CT revealed ill-defined isodense lesions in the left frontal, parietal, and occipital lobes with perilesional edema (B,F). Images showing increased 18F-FDG uptake in these lesions (C,D,G,H arrows). 68Ga-DOTA.SA.FAPi also revealed small occult lesions in the left parietal and left occipital lobe (C,G arrowhead). Reproduced with permission from (58).
4.4. Tumor volume delineation and radiation treatment planning
Another useful clinical application for FAPI-PET may be to improve gross tumor volume (GTV) delineation in external-beam radiotherapy (EBRT) planning. 18F-FDG and hypoxia imaging ligands have traditionally been the radiotracers of most interest to allow EBRT dose boosts to metabolically active or hypoxic areas (66–70). With selective targeting of FAP, and better imaging characteristics of FAPI-PET, a more accurate delineation of additional areas of interest (i.e., the tumor microenvironment) may be of clinical benefit, such as in head and neck cancer with 68Ga-FAPI, where differentiation between residual/recurrent disease and post-chemoradiation fibrosis may be a diagnostic challenge for 18F-FDG (71).
Recent work compared automated delineation of seven 68Ga-FAPI-04 pancreatic cancer cases against manual segmentation of CECT (72), demonstrating that although a clinical standard, CECT led to high interoperator variability of up to 100% in gross target volume (GTV) definition, with a Dice score coefficient of 0.55–0.65 (amongst 6 oncologists). Improved imaging performance of 68Ga-FAPI-04 compared to CECT determined that automated delineation using 2 times the background SUV compared well to manual segmentations on review, and may allow for GTV standardization in certain clinical conditions.
In brain tumors, recent research has described the usefulness and added value of using FAPI-PET ligands in tumor volume delineation in radiation treatment planning in a cohort of 13 glioblastoma patients (50), showing a difference in measured GTV compared to MRI (73). Considering the tumor microenvironment is a significant portion of the tumoral mass, gaining complementary information may prove helpful in delineating the best volumes to be treated with radiation therapy. Of note also is that dose painting and dose escalation are common in radiotherapy regimes involving PET imaging (50), adding a FAPI-specific metabolic GTV may be useful for dose escalation on areas with a strong uptake and also allowing a reduction of dose to healthy tissue by changing the PTV accordingly.
4.5. Tumor resection margins
There is a growing utilization of intraoperative MRI to assess for tumor margins and completeness of resection. FAPI-PET radioligands may play an important role in delineating the best tumor resection boundaries preoperatively and then be used intraoperatively with the aid of advanced semi-automated navigation systems. Utilization can also be intraoperative use after tumor resection in order to assess any residual tumor while still in the operating room assisting surgeons similar to a frozen section. This may be helpful especially in difficult cases with tumors near eloquent areas.
4.5. Biopsy guidance
FAPI-PET may also prove useful in biopsy planning given the heterogeneous uptake in the tumor and the importance of FAP for invasive growth, in turn suggestive of the presence of active tumor cells in areas with high tracer uptake (50). Choosing the right biopsy site can prove difficult with the current standard of care (MRI). Considering tumor heterogeneity and associated peritumoral changes seen on anatomical imaging the biopsy specimen may not be the most representative of tumor aggressiveness. FAPI-PET has been reported to be associated with tumor invasiveness and hence may be suitable to map the tumor pre-biopsy (74). It may also be interesting to have a complete tumor phenotype with a complementary role of hypoxia, glucose and amino acid metabolism radiotracers when used together. With newer PET scanning technology such as digital PET and large field of view imaging (75, 76), the use of lower radiopharmaceutical doses is possible and radiation exposure would be minimal with the main limiting factors being cost and availability of FAPI-PET radioligands.
4.6. Recurrence
Brain tumor recurrence is, at the moment, inevitable in a large number of cases. Differentiating progression from pseudoprogression as well as subtle or early true recurrence vs. post-treatment changes can be challenging with MRI. PET techniques especially with aminoacid imaging offer a proven advantage. FAPI-PET offers an additional tool in the armamentarium of the oncologist or neurosurgeon. Important work is in progress.
4.7. Therapy and theranostics
FAP has been touted as an attractive treatment target for oncology in brain tumors and other cancers. Since FAPI is showing significant strides one may envision a role for FAPI theranostics in the treatment of brain tumor patients. While the therapeutic application of FAPI tracers is still limited thus far, some studies have been carried out. A trial in 26 patients came about to make use of high FAP expression as a therapeutic target, for primarily metastatic colorectal cancer using a sibrotuzumab antibody (77). Testing of antibodies developed against FAP and labeled with 177Lu was also carried out in preclinical models (78, 79). Promising efficacy results were observed. FAP-targeting immunotherapy agents (80), vaccines (81) and FAP-specific nanoparticles (82) are also under active research.
A recent clinical investigation has investigated theranostic pairing of experimental 90Y-FAPI-46 coupled with 68Ga-FAPI-04 in a patient with metastasized breast and colorectal cancer with peritoneal metastases. Imaging with 68Ga-FAPI-04 revealed high tracer uptake in metastases and a reduction in pain symptoms after therapy with a 3-cycle regime (7.4 GBq per cycle) of experimental 90Y-FAPI-46. The patient responded with stable disease related to breast cancer, and remission of peritoneal lesions, before eventually progressing some months later. As well as 90Y-FAPI-46 as a therapeutic option, development is also ongoing for 188Re FAPI (59). Although still very much in its infancy, this case study illustrates the theranostic potential of FAPI radioligands and it is expected that more efforts will be directed towards brain cancers (83).
5. Benefits
In summary, FAPI Brain PET may be helpful in:
• Primary brain tumor assessment/prognostication and brain metastasis detection
• Tumor volume delineation and radiation treatment planning
• Tumor surgical resection margins and completeness
• Biopsy guidance
• Suspicious recurrence assessment
• Therapy and Theranostics
In vivo visualization of the tumor microenvironment is made possible by FAPI-PET, resulting in better understanding of tumor heterogeneity and a crucial piece of tumor aggressiveness and invasiveness. FAPI-PET may overcome limitations of other radiotracers including tumors with low FDG-avidity and result in some cases of upstaging through the detection of unknown distant metastases. It may also improve target volume delineation for radiotherapy planning in conjunction with other radiotracers and MRI. FAPI-PET may also complement other PET probes by offering a comprehensive phenotypic assessment of primary brain tumors in adjunct to hypoxia and amino acid metabolism tumor mapping. Multitracer evaluations may be in the future an important piece of brain tumor evaluation and characterization.
6. Limitations
As other radioligands, FAPI may not be perfect and have some minimal limitations. Considering FAP is overexpressed mainly in CAFs and not in tumor cells, FAPI-PET may not always reflect the actual tumor microenvironment. A clinical study using 68Ga-FAPI-04 and 68Ga-FAPI-46 reported non-specific uptake at sites where patients had scarring or degenerative lesions, which lead to false-positive tumor detection (84), although expert reading may alleviate this issue. Similarly, in another study, for one patient with pancreatic cancer who had developed chronic peritumoral inflammation as a result of radiotherapy, the FAPI tracer was unable to accurately identify the target volume for radiotherapy (49).
Additionally, at present only data from single-center studies of small cohorts of patients is available. As a result, in studies where findings are unexpected, larger sample studies are needed to confirm these results. An example of this is a study where sites with low or moderate FAP expression (i.e., the uterus and breast) showed higher FAPI tracer uptake (84). Additionally, ongoing discussions continue on the controversies associated with using FAPI-PET for the diagnosis of bone and lymph node metastases (85–87). Pharmacokinetics with fast clearance from blood and short retention in tumors may be problematic for radionuclide therapy applications or be an advantage allowing closer follow-up cycles with lower toxicity. Hence, structural modification of FAPI optimizing tumor uptake and tumor retention time for peptide radionuclide therapy (PRRT) is another key research direction.
7. Conclusions
FAPI-PET is a promising tool for the assessment of primary and metastatic brain tumors and offers several clinical advantages including added sensitivity, management guidance as well a more complete phenotypic evaluation of tumors. Promising research shows the potential for translation of FAPI imaging agents to the development of therapy agents in the realm of theranostic nuclear medicine. Although data is currently sparse, new studies demonstrate FAPI as a viable target for brain PET imaging either as a single probe or within a multi-tracer tumor mapping paradigm, however large prospective trials are needed.
Author contributions
RA, MA & OB performed the literature review. JD & MD devised the concept for review and filtered review material. All authors contributed to the article and approved the submitted version.
Funding
RA and OB were supported by the Dean's impact initiative at TAMUQ.
Conflict of interest
JD is an employee of Siemens Medical Solutions who did not financially sponsor this work.
The author(s) OB declared that they were an editorial board member of Frontiers, at the time of submission. This had no impact on the peer review process and the final decision.
The remaining authors declare that the research was conducted in the absence of any commercial or financial relationships that could be construed as a potential conflict of interest.
Publisher's note
All claims expressed in this article are solely those of the authors and do not necessarily represent those of their affiliated organizations, or those of the publisher, the editors and the reviewers. Any product that may be evaluated in this article, or claim that may be made by its manufacturer, is not guaranteed or endorsed by the publisher.
References
1. Miller KD, Ostrom QT, Kruchko C, Patil N, Tihan T, Cioffi G, et al. Brain and other central nervous system tumor statistics. CA Cancer J Clin. (2021) 71:381–406. doi: 10.3322/caac.21693
2. Ellingson BM, Wen PY, van den Bent MJ, Cloughesy TF. Pros and cons of current brain tumor imaging. Neuro Oncol. (2014) 16(S7):vii2–11. doi: 10.1093/neuonc/nou224
3. Upadhyay N, Waldman AD. Conventional MRI evaluation of gliomas. Br J Radiol. (2011) 84(Spec Iss 2):S107–11. doi: 10.1259/bjr/65711810
4. Verger A, Kas A, Darcourt J, Guedj E. PET Imaging in neuro-oncology: an update and overview of a rapidly growing area. Cancers (Basel). (2022) 14(5):1103. doi: 10.3390/cancers14051103
5. Tanaka H, Yamaguchi T, Hachiya K, Miwa K, Shinoda J, Hayashi M, et al. 11C-methionine positron emission tomography for target delineation of recurrent glioblastoma in re-irradiation planning. Rep Pract Oncol Radiother. (2018) 23(3):215–9. doi: 10.1016/j.rpor.2018.04.003
6. Ma Y, Wang Q, Dong Q, Zhan L, Zhang J. How to differentiate pseudoprogression from true progression in cancer patients treated with immunotherapy. Am J Cancer Res. (2019) 9(8):1546–53.31497342
7. Costa LB, Queiroz MA, Barbosa FG, Nunes RF, Zaniboni EC, Ruiz MM, et al. Reassessing patterns of response to immunotherapy with PET: from morphology to metabolism. Radiographics. (2021) 41(1):120–43. doi: 10.1148/rg.2021200093
8. Hicks RJ. The role of PET in monitoring therapy. Cancer Imaging. (2005) 5(1):51–7. doi: 10.1102/1470-7330.2005.0006
9. Law I, Albert NL, Arbizu J, Boellaard R, Drzezga A, Galldiks N, et al. Joint EANM/EANO/RANO practice guidelines/SNMMI procedure standards for imaging of gliomas using PET with radiolabelled amino acids and [18F]FDG: version 1.0. Eur J Nucl Med Mol Imaging. (2019) 46(3):540–57. doi: 10.1007/s00259-018-4207-9
10. Gündoğan C, Güzel Y, Can C, Kaplan İ, Kömek H. FAPI-04 uptake in healthy tissues of cancer patients in 68Ga-FAPI-04 PET/CT imaging. Contrast Media Mol Imaging. (2021) 2021:9750080. doi: 10.1155/2021/9750080
11. Mu X, Huang X, Li M, Sun W, Fu W. Comparison of physiological uptake of normal tissues in patients with cancer using 18F-FAPI-04 and 18F-FAPI-42 PET/CT. Front Nucl Med. (2022) 2. doi: 10.3389/fnume.2022.927843
12. Galldiks N, Law I, Pope WB, Arbizu J, Langen KJ. The use of amino acid PET and conventional MRI for monitoring of brain tumor therapy. Neuroimage Clin. (2016) 13:386–94. doi: 10.1016/j.nicl.2016.12.020
13. Alwadani B, Dall'Angelo S, Fleming IN. Clinical value of 3′-deoxy-3′-[18F]fluorothymidine-positron emission tomography for diagnosis, staging and assessing therapy response in lung cancer. Insights Imaging. (2021) 12(1):90. doi: 10.1186/s13244-021-01026-1
14. Gouel P, Decazes P, Vera P, Gardin I, Thureau S, Bohn P. Advances in PET and MRI imaging of tumor hypoxia. Front Med (Lausanne). (2023) 10:1055062. doi: 10.3389/fmed.2023.1055062
15. Ammer LM, Vollmann-Zwerenz A, Ruf V, Wetzel CH, Riemenschneider MJ, Albert NL, et al. The role of translocator protein TSPO in hallmarks of glioblastoma. Cancers (Basel). (2020) 12(10):2973. doi: 10.3390/cancers12102973
16. Su Z, Herholz K, Gerhard A, Roncaroli F, Du Plessiss D, Jackson A, et al. [¹¹C]-(R)PK11195 tracer kinetics in the brain of glioma patients and a comparison of two referencing approaches. Eur J Nucl Med Mol Imaging. (2013) 40(9):1406–19. doi: 10.1007/s00259-013-2447-2
17. Winkeler A, Boisgard R, Awde AR, Dubois A, Theze B, Zheng J, et al. The translocator protein ligand [¹⁸F]DPA-714 images glioma and activated microglia in vivo. Eur J Nucl Med Mol Imaging. (2012) 39(5):811–23. doi: 10.1007/s00259-011-2041-4
18. Holzgreve A, Pötter D, Brendel M, Orth M, Weidner L, Gold L, et al. Longitudinal [18F]GE-180 PET imaging facilitates in vivo monitoring of TSPO expression in the GL261 glioblastoma mouse model. Biomedicines. (2022) 10(4):738. doi: 10.3390/biomedicines10040738
19. Kossatz S, Carney B, Schweitzer M, Carlucci G, Miloushev VZ, Maachani UB, et al. Biomarker-based PET imaging of diffuse intrinsic pontine glioma in mouse models. Cancer Res. (2017) 77(8):2112–23. doi: 10.1158/0008-5472.CAN-16-2850
20. Stotz S, Kinzler J, Nies AT, Schwab M, Maurer A. Two experts and a newbie: [18F]PARPi vs [18F]FTT vs [18F]FPyPARP-a comparison of PARP imaging agents. Eur J Nucl Med Mol Imaging. (2022) 49(3):834–46. doi: 10.1007/s00259-021-05436-7
21. Lapa C, Lückerath K, Kleinlein I, Monoranu CM, Linsenmann T, Kessler AF, et al. (68)Ga-pentixafor-PET/CT for imaging of chemokine receptor 4 expression in glioblastoma. Theranostics. (2016) 6(3):428–34. doi: 10.7150/thno.13986
22. Heute D, Kostron H, von Guggenberg E, Ingorokva S, Gabriel M, Dobrozemsky G, et al. Response of recurrent high-grade glioma to treatment with (90)Y-DOTATOC. J Nucl Med. (2010) 51(3):397–400. doi: 10.2967/jnumed.109.072819
23. Holzgreve A, Biczok A, Ruf VC, Liessche-Starnecker F, Steiger K, Kirchner MA, et al. PSMA expression in glioblastoma as a basis for theranostic approaches: a retrospective, correlational panel study including immunohistochemistry, clinical parameters and PET imaging. Front Oncol. (2021) 11:646387. doi: 10.3389/fonc.2021.646387
24. Kunikowska J, Kuliński R, Muylle K, Koziara H, Królicki L. 68Ga-prostate-specific membrane antigen-11 PET/CT: a new imaging option for recurrent glioblastoma multiforme? Clin Nucl Med. (2020) 45(1):11–8. doi: 10.1097/RLU.0000000000002806
25. Kratochwil C, Flechsig P, Lindner T, Abderrahim L, Altmann A, Mier W, et al. 68Ga-FAPI PET/CT: tracer uptake in 28 different kinds of cancer. J Nucl Med. (2019) 60(6):801–5. doi: 10.2967/jnumed.119.227967
26. Dendl K, Koerber SA, Kratochwil C, Cardinale J, Finck R, Dabir M, et al. FAP and FAPI-PET/CT in malignant and non-malignant diseases: a perfect symbiosis? Cancers (Basel). (2021) 13(19):4946. doi: 10.3390/cancers13194946
27. Heckmann MB, Reinhardt F, Finke D, Katus HA, Haberkorn U, Leuschner F, et al. Relationship between cardiac fibroblast activation protein activity by positron emission tomography and cardiovascular disease. Circ Cardiovasc Imaging. (2020) 13:e010628. doi: 10.1161/CIRCIMAGING.120.010628
28. Wang L, Wang Y, Wang J, Xiao M, Xi XY, Chen BX, et al. Myocardial activity at 18F-FAPI PET/CT and risk for sudden cardiac death in hypertrophic cardiomyopathy. Radiology. (2023) 306(2):e221052. doi: 10.1148/radiol.221052
29. Diekmann J, Koenig T, Thackeray JT, Derlin T, Czerner C, Neuser J, et al. Cardiac fibroblast activation in patients early after acute myocardial infarction: integration with MR tissue characterization and subsequent functional outcome. J Nucl Med. (2022) 63(9):1415–23. doi: 10.2967/jnumed.121.263555
30. Varasteh Z, Mohanta S, Robu S, Braeuer M, Li Y, Omidvari N, et al. Molecular imaging of fibroblast activity after myocardial infarction using a 68Ga-labeled fibroblast activation protein inhibitor, FAPI-04. J Nucl Med. (2019) 60(12):1743–9. doi: 10.2967/jnumed.119.226993
31. Aghajanian H, Kimura T, Rurik JG, Hancock AS, Leibowitz MS, Scholler J, et al. Targeting cardiac fibrosis with engineered T cells. Nature. (2019) 573(7774):430–3. Erratum in: Nature. 2019;576(7785). doi: 10.1038/s41586-019-1546-z
32. Song W, Zhang X, He S, Gai Y, Qin C, Hu F, et al. 68Ga-FAPI PET visualize heart failure: from mechanism to clinic. Eur J Nucl Med Mol Imaging. (2023) 50(2):475–85. doi: 10.1007/s00259-022-05994-4
33. Siebermair J, Köhler MI, Kupusovic J, Nekolla SG, Kessler L, Ferdinandus J, et al. Cardiac fibroblast activation detected by ga-68 FAPI PET imaging as a potential novel biomarker of cardiac injury/remodeling. J Nucl Cardiol. (2021) 28(3):812–21. doi: 10.1007/s12350-020-02307-w
34. Luo Y, Pan Q, Zhang W. IgG4-related disease revealed by 68Ga-FAPI and 18F-FDG PET/CT. Eur J Nucl Med Mol Imaging. (2019) 46(12):2625–6. doi: 10.1007/s00259-019-04478-2
35. Pan Q, Luo Y, Zhang W. Recurrent immunoglobulin G4-related disease shown on 18F-FDG and 68Ga-FAPI PET/CT. Clin Nucl Med. (2020) 45(4):312–3. doi: 10.1097/RLU.0000000000002919
36. Demmert TT, Maric I, Pomykala KL, Lueckerath K, Siveke J, Schaarschmidt BM, et al. Novel 68Ga-FAPI PET/CT offers oncologic staging without COVID-19 vaccine-related pitfalls. J Nucl Med. (2023) 64(3):368–71. doi: 10.2967/jnumed.122.264872
37. Gaggioli C, Hooper S, Hidalgo-Carcedo C, et al. Fibroblast-led collective invasion of carcinoma cells with differing roles for RhoGTPases in leading and following cells. Nat Cell Biol. (2007) 9(12):1392–400. doi: 10.1038/ncb1658
38. Giesel FL, Kratochwil C, Lindner T, Marschalek MM, Loktev A, Lehnert W, et al. 68Ga-FAPI PET/CT: biodistribution and preliminary dosimetry estimate of 2 DOTA-containing FAP-targeting agents in patients with various cancers. J Nucl Med. (2019) 60(3):386–92. doi: 10.2967/jnumed.118.215913
39. Lindner T, Loktev A, Altmann A, Giesel F, Kratochwil C, Debus J, et al. Development of quinoline-based theranostic ligands for the targeting of fibroblast activation protein. J Nucl Med. (2018) 59:1415–22. doi: 10.2967/jnumed.118.210443.40
40. Loktev A, Lindner T, Mier W, Debus J, Altmann A, Jager D, et al. A tumour-imaging method targeting cancer-associated fibroblasts. J Nucl Med. (2018) 59(9):1423–9. doi: 10.2967/jnumed.118.210435.41
41. Lindner T, Loktev A, Altmann A, Giesel F, Kratochwil C, Debus J, et al. Development of quinoline-based theranostic ligands for the targeting of fibroblast activation protein. J Nucl Med. (2018) 59(9):1415–22. doi: 10.2967/jnumed.118.210443.42
42. Moon ES, Elvas F, Vliegen G, De Lombaerde S, Vangestel C, De Bruycker S, et al. Targeting fibroblast activation protein (FAP): next generation PET radiotracers using squaramide coupled bifunctional DOTA and DATA5m chelators. EJNMMI Radiopharm Chem. (2020) 5(1):19. doi: 10.1186/s41181-020-00102-z.43
43. Zhao L, Niu B, Fang J, Pang Y, Li S, Xie C, et al. Synthesis, preclinical evaluation, and a pilot clinical PET imaging study of (68)Ga-labeled FAPI dimer. J Nucl Med. (2022) 63(6):862–8. doi: 10.2967/jnumed.121.263016
44. Toms J, Kogler J, Maschauer S, Daniel C, Schmidkonz C, Kuwert T, et al. Targeting fibroblast activation protein: radiosynthesis and preclinical evaluation of an (18)F-labeled FAP inhibitor. J Nucl Med. (2020) 61(12):1806–13. doi: 10.2967/jnumed.120.242958.45
45. Slania SL, Das D, Lisok A, Du Y, Jiang Z, Mease RC, et al. Imaging of fibroblast activation protein in cancer Xenografts using novel (4-quinolinoyl)-glycyl-2-cyanopyrrolidine-based small molecules. J Medicinal Chem. (2021) 64(7):4059–70. doi: 10.1021/acs.jmedchem.0c02171.46
46. Moon ES, Van Rymenant Y, Battan S, De Loose J, Bracke A, Van der Veken, et al. In vitro evaluation of the squaramide-conjugated fibroblast activation protein inhibitor-based agents AAZTA(5).SA.FAPi and DOTA.SA.FAPi. Molecules. (2021) 26(12):3482. doi: 10.3390/molecules26123482.47
47. Mori Y, Dendl K, Cardinale J, Kratochwil C, Giesel FL, Haberkorn U. FAPI PET: fibroblast activation protein inhibitor use in oncologic and nononcologic disease. Radiology. (2023) 306(2):e220749. doi: 10.1148/radiol.220749
48. Matrasova I, Busek P, Balaziova E, Sedo A. Heterogeneity of molecular forms of dipeptidyl peptidase-IV and fibroblast activation protein in human glioblastomas. Biomed Pap Med Fac Univ Palacky Olomouc Czech Repub. (2017) 161(3):252–60. doi: 10.5507/bp.2017.010
49. Röhrich M, Loktev A, Wefers AK, et al. IDH-wildtype glioblastomas and grade III/IV IDH-mutant gliomas show elevated tracer uptake in fibroblast activation protein–specific PET/CT. Eur J Nucl Med Mol Imaging. (2019) 46(12):2569–80. doi: 10.1007/s00259-019-04444-y
50. Windisch P, Röhrich M, Regnery S, Tonndorf-Martini E, Held T, Lang K, et al. Fibroblast activation protein (FAP) specific PET for advanced target volume delineation in glioblastoma. Radiother Oncol. (2020) 150:159–63. doi: 10.1016/j.radonc.2020.06.040
51. Sahai E, Astsaturov I, Cukierman E, DeNardo DG, Egeblad M, Evans RM, et al. A framework for advancing our understanding of cancer-associated fibroblasts. Nat Rev Cancer. (2020) 20(3):174–86. doi: 10.1038/sss41568-019-0238-1
52. Martin M, Wei H, Lu T. Targeting microenvironment in cancer therapeutics. Oncotarget. (2016) 7(32):52575–83. doi: 10.18632/oncotarget.9824
53. Santi A, Kugeratski FG, Zanivan S. Cancer associated fibroblasts: the architects of stroma remodeling. Proteomics. (2018) 18:e1700167. doi: 10.1002/pmic.201700167
54. Gilardi L, Airò Farulla LS, Demirci E, Clerici I, Omodeo Salè E, Ceci F. Imaging cancer-associated fibroblasts (CAFs) with FAPi PET. Biomedicines. (2022) 10(3):523. doi: 10.3390/biomedicines10030523
55. Huang R, Pu Y, Huang S, et al. FAPI-PET/CT in cancer imaging: a potential novel molecule of the century. Front Oncol. (2022) 12:854658. doi: 10.3389/fonc.2022.854658
56. Loktev A, Lindner T, Burger EM, Annette A, Giesel F, Kratochwil C, et al. Development of fibroblast activation protein-targeted radiotracers with improved tumor retention. J Nucl Med. (2019) 60(10):1421–9. doi: 10.2967/jnumed.118.224469
57. Wang S, Zhou X, Xu X, Ding J, Liu S, Hou X, et al. Clinical translational evaluation of Al18F-NOTA-FAPI for fibroblast activation protein-targeted tumour imaging. Eur J Nucl Med Mol Imaging. (2021) 48(13):4259–71. doi: 10.1007/s00259-021-05470-5
58. Ballal S, Yadav MP, Moon ES, Kramer VS, Roesch F, Kumari S, et al. Biodistribution, pharmacokinetics, dosimetry of [68Ga]ga-DOTA.SA.FAPi, and the head-to-head comparison with [18F]F-FDG PET/CT in patients with various cancers. Eur J Nucl Med Mol Imaging. (2021) 48(6):1915–31. doi: 10.1007/s00259-020-05132-y
59. Lindner T, Altmann A, Krämer S, Kleist C, Loktev A, Kratochwil C, et al. Design and development of 99mTc-labeled FAPI tracers for SPECT imaging and 188Re therapy. J Nucl Med. (2020) 61(10):1507–13. doi: 10.2967/jnumed.119.239731
60. Giesel FL, Adeberg S, Syed M, Lindner T, Jimenez-Franco LD, Mavriopoulou E, et al. FAPI-74 PET/CT using either 18F-AlF or cold-kit 68Ga labeling: biodistribution, radiation dosimetry, and tumor delineation in lung cancer patients. J Nucl Med. (2021) 62(2):201–7. doi: 10.2967/jnumed.120.245084
61. Röhrich M, Naumann P, Giesel FL, Choyke PL, Staudinger F, Wefers A, et al. Impact of 68Ga-FAPI PET/CT imaging on the therapeutic management of primary and recurrent pancreatic ductal adenocarcinomas. J Nucl Med. (2021) 62(6):779–86. doi: 10.2967/jnumed.120.253062
62. Giesel FL, Kratochwil C, Schlittenhardt J, Dendl K, Eiber M, Staudinger F, et al. Head-to-head intra-individual comparison of biodistribution and tumor uptake of 68Ga-FAPI and 18F-FDG PET/CT in cancer patients. Eur J Nucl Med Mol Imaging. (2021) 48(13):4377–85. doi: 10.1007/s00259-021-05307-1
63. Hicks RJ, Roselt PJ, Kallur KG, Tothill RW, Mileshkin L. FAPI PET/CT: will it end the hegemony of 18F-FDG in oncology? J Nucl Med. (2021) 62(3):296–302. doi: 10.2967/jnumed.120.256271
64. Treglia G, Muoio B, Roustaei H, Kiamanesh Z, Aryana K, Sadeghi R. Head-to-head comparison of fibroblast activation protein inhibitors (FAPI) radiotracers versus [18F]F-FDG in oncology: a systematic review. Int J Mol Sci. (2021) 22(20):11192. doi: 10.3390/ijms222011192
65. Giesel FL, Heussel CP, Lindner T, Rohrich M, Rathke H, Kauczor HU, et al. FAPI-PET/CT improves staging in a lung cancer patient with cerebral metastasis. Eur J Nucl Med Mol Imaging. (2019) 46(8):1754–5. doi: 10.1007/s00259-019-04346-z
66. Thureau S, Dubray B, Modzelewski R, Bohn P, Hapdey S, Vincent S, et al. FDG and FMISO PET-guided dose escalation with intensity-modulated radiotherapy in lung cancer. Radiat Oncol. (2018) 13(1):208. doi: 10.1186/s13014-018-1147-2
67. van den Bosch S, Dijkema T, Kunze-Busch MC, et al. Uniform FDG-PET guided GRAdient dose prEscription to reduce late radiation toxicity (UPGRADE-RT): study protocol for a randomized clinical trial with dose reduction to the elective neck in head and neck squamous cell carcinoma. BMC Cancer. (2017) 17(1):208. doi: 10.1186/s12885-017-3195-7
68. Fan B, Li C, Mu F, et al. Dose escalation guided by 18F-FDG PET/CT for esophageal cancer. Radiation Medicine and Protection. (2021) 2(2):55–60. doi: 10.1016/j.radmp.2021.04.002
69. Liu T, Karlsen M, Karlberg AM, Redalen KR. Hypoxia imaging and theranostic potential of [64Cu][Cu(ATSM)] and ionic Cu(II) salts: a review of current evidence and discussion of the retention mechanisms. EJNMMI Res. (2020) 10(1):33. doi: 10.1186/s13550-020-00621-5
70. Peeters SG, Zegers CM, Lieuwes NG, van Elmpt W, Eriksson J, van Dongen GA, et al. A comparative study of the hypoxia PET tracers [¹⁸F]HX4, [¹⁸F]FAZA, and [¹⁸F]FMISO in a preclinical tumor model. Int J Radiat Oncol Biol Phys. (2015) 91(2):351–9. doi: 10.1016/j.ijrobp.2014.09.045
71. Lai V, Khong PL. Updates on MR imaging and ¹⁸F-FDG PET/CT imaging in nasopharyngeal carcinoma. Oral Oncol. (2014) 50(6):539–48. doi: 10.1016/j.oraloncology.2013.05.005
72. Liermann J, Syed M, Ben-Josef E, Schubert K, Schlampp I, Sprengel SD, et al. Impact of FAPI-PET/CT on target volume definition in radiation therapy of locally recurrent pancreatic cancer. Cancers (Basel). (2021) 13(4):796. doi: 10.3390/cancers13040796
73. Niyazi M, Geisler J, Siefert A, Schwarz SB, Ganswindt Garny S, et al. FET-PET for malignant glioma treatment planning. Radiother Oncol. (2011) 99(1):44–8. doi: 10.1016/j.radonc.2011.03.001
74. Mentlein R, Hattermann K, Hemion C, Jungbluth AA, Held-Feindt J. Expression and role of the cell surface protease seprase/fibroblast activation protein-α (FAP-α) in astroglial tumors. Biol Chem. (2011) 392(3):199–207. doi: 10.1515/BC.2010.119
75. Alberts I, Sachpekidis C, Prenosil G, Viscione M, Bohn KP, Mingels C, et al. Digital PET/CT allows for shorter acquisition protocols or reduced radiopharmaceutical dose in [18F]-FDG PET/CT. Ann Nucl Med. (2021) 35(4):485–92. doi: 10.1007/s12149-021-01588-6
76. Slart RHJA, Tsoumpas C, Glaudemans AWJM, et al. Long axial field of view PET scanners: a road map to implementation and new possibilities. Eur J Nucl Med Mol Imaging. (2021) 48(13):4236–45. doi: 10.1007/s00259-021-05461-6
77. Scott AM, Wiseman G, Welt S, Adjei A, Lee FT, Hopkins W, et al. A phase I dose-escalation study of sibrotuzumab in patients with advanced or metastatic fibroblast activation protein-positive cancer. Clin Cancer Res. (2003) 9(5):1639–47.12738716
78. Fischer E, Chaitanya K, Wüest T, Wadle A, Scott AM, van den Broek M, et al. Radioimmunotherapy of fibroblast activation protein positive tumors by rapidly internalizing antibodies. Clin Cancer Res. (2012) 18(22):6208–18. doi: 10.1158/1078-0432.CCR-12-0644
79. Puré E, Blomberg R. Pro-tumorigenic roles of fibroblast activation protein in cancer: back to the basics. Oncogene. (2018) 37(32):4343–57. doi: 10.1038/s41388-018-0275-3
80. Bughda R, Dimou P, D'Souza RR, Klampatsa A. Fibroblast activation protein (FAP)-targeted CAR-T cells: launching an attack on tumor stroma. Immunotargets Ther. (2021) 10:313–23. doi: 10.2147/ITT.S291767
81. Zhang X, Cui H, Zhang W, Li Z, Gao J. Engineered tumor cell-derived vaccines against cancer: the art of combating poison with poison. Bioact Mater. (2022) 22:491–517. doi: 10.1016/j.bioactmat.2022.10.016
82. Zhou S, Zhen Z, Paschall AV, Xue L, Yang X, Bebin-Blackwell AG, et al. FAP-targeted photodynamic therapy mediated by ferritin nanoparticles elicits an immune response against cancer cells and cancer associated fibroblasts. Adv Funct Mater. (2021) 31(7):2007017. doi: 10.1002/adfm.202007017
83. Rathke H, Fuxius S, Giesel FL, Lindner T, Debus J, Haberkorn U, et al. Two tumors, one target: preliminary experience with 90Y-FAPI therapy in a patient with metastasized breast and colorectal cancer. Clin Nucl Med. (2021) 46(10):842–4. doi: 10.1097/RLU.0000000000003842
84. Kessler L, Ferdinandus J, Hirmas N, et al. Pitfalls and common findings in 68Ga-FAPI PET: a pictorial analysis. J Nucl Med. (2022) 63(6):890–6. doi: 10.2967/jnumed.121.262808
85. Gu B, Xu X, Zhang J, et al. The added value of 68Ga-FAPI PET/CT in patients with head and neck cancer of unknown primary with 18F-FDG-negative findings. J Nucl Med. (2022) 63(6):875–81. doi: 10.2967/jnumed.121.262790
86. Wu J, Wang Y, Liao T, et al. Comparison of the relative diagnostic performance of [68Ga]ga-DOTA-FAPI-04 and [18F]FDG PET/CT for the detection of bone metastasis in patients with different cancers. Front Oncol. (2021) 11:737827. doi: 10.3389/fonc.2021.737827
Keywords: PET, FAPI imaging, neuro-oncology, nuclear medicine, radiotracer
Citation: Djekidel M, Alsadi R, Abi Akl M, Bouhali O and O’Doherty J (2023) Tumor microenvironment and fibroblast activation protein inhibitor (FAPI) PET: developments toward brain imaging. Front. Nucl. Med. 3:1183471. doi: 10.3389/fnume.2023.1183471
Received: 10 March 2023; Accepted: 3 July 2023;
Published: 18 July 2023.
Edited by:
Mathias Kranz, University Hospital of North Norway, NorwayReviewed by:
Inigo Martinez, UiT The Arctic University of Norway, NorwayManuel Röhrich, Heidelberg University, Germany
© 2023 Djekidel, Alsadi, Abi Akl, Bouhali and O' Doherty. This is an open-access article distributed under the terms of the Creative Commons Attribution License (CC BY). The use, distribution or reproduction in other forums is permitted, provided the original author(s) and the copyright owner(s) are credited and that the original publication in this journal is cited, in accordance with accepted academic practice. No use, distribution or reproduction is permitted which does not comply with these terms.
*Correspondence: Jim O’Doherty amFtZXMub2RvaGVydHlAc2llbWVucy1oZWFsdGhpbmVlcnMuY29t