- 1Department of Nuclear Medicine, Centre Hospitalier Universitaire de Bordeaux, Bordeaux, France
- 2University of Bordeaux, IMB, UMR CNRS 5251, INRIA Project Team Monc, Talence, France
- 3Department of Haematology, Centre Hospitalier Universitaire de Bordeaux, Bordeaux, France
- 4Department of Radiology, Centre Hospitalier Universitaire de Bordeaux, Bordeaux, France
- 5University of Bordeaux, INCIA UMR-CNRS 5287, Talence, France
- 6Institut Universitaire de France (IUF), Paris, France
During the last two decades, the imaging landscape of multiple myeloma (MM) has evolved with whole-body imaging techniques such as fluorodeoxyglucose positron emission tomography–computed tomography (18F-FDG PET/CT) and MRI replacing X-ray skeletal survey. Both imaging modalities have high diagnostic performance at the initial diagnosis of MM and are key players in the identification of patients needing treatment. Diffusion-weighted MRI has a high sensitivity for bone involvement, while 18F-FDG PET/CT baseline parameters carry a strong prognostic value. The advent of more efficient therapeutics, such as immunomodulatory drugs and proteasome inhibitors, has called for the use of sensitive imaging techniques for monitoring response to treatment. Diffusion-weighted MRI could improve the specificity of MRI for tumor response evaluation, but questions remain regarding its role as a prognostic factor. Performed at key time points of treatment in newly diagnosed MM patients, 18F-FDG PET/CT showed a strong association with relapse risk and survival. The deployment of minimal residual disease detection at the cellular or the molecular level may raise questions on the role of these imaging techniques, which will be addressed. This review summarizes and outlines the specificities and respective roles of MRI and 18F-FDG PET/CT in the management of MM.
Introduction
Historically, multiple myeloma (MM) bone extent was assessed by a skeletal survey. This technique was part of the Durie–Salmon classification (1). Whole-body X-ray is, however, limited by its lack of sensitivity, partly because MM osteolytic lesions start being visible when more than 30% of the trabecular bone is involved (2).
Functional imaging with the use of fluorodeoxyglucose positron emission tomography–computed tomography (18F-FDG PET/CT) and MRI has progressively replaced skeletal survey. These whole-body imaging techniques had first been incorporated in the Durie and Salmon Plus classification (3). Both allow a more precise evaluation of tumor burden in bone/bone marrow, which has been recognized as an important prognostic factor in MM. Nowadays, bone involvement is defined by 18F-FDG PET/CT or MRI following the International Myeloma Working Group (IMWG) recommendations for MM (4). Both imaging modalities are therefore key players in the identification of myeloma patients needing treatment.
During the last decade, several studies have been published on the respective contribution of 18F-FDG PET/CT and MRI in the management of MM. Newer MRI techniques with the use of diffusion imaging have also emerged. The goal of this review was to summarize and outline the specificities and respective roles of both imaging modalities in MM.
Role of Whole-Body Low-Dose Computed Tomography
Advances in technology now allow performing CT with a low radiation dose while preserving image quality. In 2005, Horger et al. introduced this technique for MM imaging, showing an excellent inter-observer agreement of 95% (5). Whole-body low-dose CT (WBLDCT) is a faster imaging procedure and is useful in evaluating the risk of spine fracture instability. Overall, when compared with skeletal survey, WBLDCT detects additional lesions in about 20% of patients, especially in the spine and pelvis (6–9). Performing WBLDCT at baseline can also lead to a change in the clinical management of 18–20% of patients (6–8). It is, however, limited on some points: the detection of extra-medullary lesions and the assessment of diffuse bone marrow infiltration (10).
WBLDCT is one of the recommended imaging procedures for baseline imaging of MM following the guidelines of IMWG, the European Society for Medical Oncology (ESMO), and the European Myeloma Network (11–13). The detection of ≥1 osteolytic focal lesion (FL) is a sufficient criterion for treatment initiation. Only bone lesions ≥5 mm should be looked at to avoid false-positive findings, considering the high frequency of osteoporosis in this population (10). Of note is that WBLDCT images can replace standard CT in PET/CT procedures without any noticeable degradation in the attenuation-corrected PET scan (14).
Comparison of 18F-FDG PET/CT and MRI at Baseline Evaluation of MM
Fundamental Concepts of Imaging Signal
18F-FDG is a biochemical analog of glucose. It is imported through the cell by GLUT-1 and GLUT-3 transporters and phosphorylated by a hexokinase, then becoming 18F-FDG-6P. Under this form, 18F-FDG-6P cannot be further metabolized through the Krebs cycle for the purpose of aerobic glycolysis. This metabolism dead-end contributes to the accumulation of 18F-FDG-6P in the cell, reaching a state of equilibrium within 60 min after 18-FDG administration. MM plasma cells generally have an overexpression of hexokinase-2 helping them reach a high glycolytic activity. Therefore, in MM, 18-FDG focal uptake on PET/CT is a reflection of packed MM cells with higher glycolytic activity than normal surrounding cells.
In the bone marrow, the signal intensity of MRI depends on the proportions of hematopoietic red marrow, fatty yellow marrow, and, to a lesser extent, mineralized bone matrix. T1-weighted images reflect bone marrow fatty content, as the protons contained in the heavy molecular hydrophobic complex have a very efficient spin–lattice relaxation, resulting in a short T1 relaxation time, i.e., a high T1-weighted signal. Short tau inversion recovery (STIR) T2-weighted (T2w-STIR) sequences perform a homogenous suppression of fat signals by exploiting the difference in the relaxation times between water and fat. Multiple Myeloma lesions have high cellularity and water content, therefore appearing as hypointense on T1- and hyperintense on T2w-STIR images.
Diffusion-weighted imaging studies the Brownian stochastic movement of water molecules within extracellular, intracellular, and intravascular spaces, allowing the study of the tissue microarchitecture. Water diffusion in biological tissues is restrained by cellular membranes, organites, and macromolecules. Therefore, diffusion can be decreased in tissues with high cellular density, intact cellular membranes, or reduced extracellular space. The apparent diffusion coefficient (ADC) is a quantitative biomarker of diffusion. In normal bone marrow, the diffusion signal is decreased in fatty marrow due to large adipocytes restraining the extracellular space and is increased in red marrow due to the higher vascularization and water content. Bone marrow infiltration by MM cells creates a high diffusion signal due to the replacement of yellow marrow adipocytes and to an increased cellular density.
Disease Presentation and Imaging Patterns
Different imaging patterns of MM bone involvement have been described. Patients can present only with FLs, which are defined on 18F-FDG PET/CT as a focal uptake above bone marrow background with an underlying osteolytic lesion on companion CT (4). In numerous relevant studies, the definition of FL has been extended to focal bone uptake on two consecutive PET slices without evident changes on CT (15–17). On MRI, FL is defined as a lesion with a low T1-weighted signal and a high T2w-STIR signal (18). Two other patterns have been described: diffuse bone marrow infiltration (diffuse disease) only and the combination of FL and diffuse disease. Diffuse disease is typically described on MRI as a diffuse bone marrow hypointense signal on T1-weighted images associated with a diffuse bone marrow high signal on T2w-STIR images (19). The severity degree of diffuse disease can be further characterized into mild to moderate or severe depending on the intensity of signal modification on T1-weighted sequences by comparing it to the intervertebral disk signal (19). However, diffuse disease can sometimes be difficult to diagnose on MRI due to varying imaging features depending on the degree of infiltration. Diffusion-weighted MRI (DW-MRI) may help in that regard. On 18F-FDG PET/CT, diffuse disease diagnosis can be missed, especially in cases of mild and moderate infiltration due to low plasma cell density, unless the cell avidity for FDG is high. Diffuse bone marrow infiltration has been described as a diffuse bone marrow uptake superior to liver uptake (17, 20). An additional variegated pattern has been described on MRI and corresponds to multiple micronodular bone marrow lesions. Most authors consider this entity as a low-grade diffuse infiltration (21). The IMWG recommendations state that the presence of ≥1 osteolytic FL on 18F-FDG PET/CT or ≥2 FLs on MRI is a sufficient criterion to define bone involvement and start patient treatment (4).
Extramedullary disease (EMD) can be depicted by 18F-FDG PET/CT or whole-body MRI (22–24). It corresponds to disease sites located outside the bone structure and can be located in any organ or soft tissue (25). It should be differentiated from paramedullary disease (PMD), which corresponds to breakout bone lesions that invade the surrounding soft tissues. The two entities are different as EMD is composed of immature plasmablastic cells and PMD is made up of plasma cells (26). Approximatively 2–14% of patients have primary EMD at the initial diagnosis, and about 8–18% will develop EMD during the disease course throughout therapeutic sequences (26, 27). The most frequent sites involved are the pleura, liver, lymph nodes, spleen, subcutaneous tissue, and paravertebral areas (23, 28).
18F-FDG PET/CT readings can be standardized with the Italian Myeloma Criteria for PET Use (IMPETUS) (29, 30). The IMPETUS criteria have shown high inter-observer reproducibility (30). The main characteristics of both imaging modalities at baseline are summarized in Table 1.
18F-FDG PET/CT Compared With Conventional MRI for Baseline Evaluation
Earlier studies compared the detection rates of 18F-FDG PET/CT and axial skeleton (AS) MRI (spine/pelvis) in newly diagnosed MM (NDMM) patients (22, 31, 32). Overall, the results showed that 18F-FDG PET/CT and MRI have similar FL detection rates. It should be stressed that most of the additional lesions detected by 18F-FDG PET/CT were outside the field of view of AS-MRI. These studies also showed that MRI is superior to 18F-FDG PET/CT for the diagnosis of diffuse disease (22, 32, 33). However, a larger prospective study of 134 patients found no statistically significant difference in the concordance of AS-MRI and 18F-FDG PET/CT for the diagnosis of myeloma bone involvement (34). Compared to AS-MRI, whole-body MRI detects additional FLs located outside the axial skeleton (211 lesions in a study of 100 patients) (35). When compared with whole-body MRI, 18F-FDG PET/CT had a slightly lower sensitivity (59 vs. 68%) in a study of 24 patients (36). A recent study has shown that, although whole-body MRI had higher sensitivity than 18F-FDG PET/CT in 40 NDMM, 18F-FDG PET/CT had a higher impact on clinical decisions (37). Currently, 18F-FDG PET/CT or low-dose whole-body CT is recommended for patients with suspected myeloma as first-line imaging. MRI should be performed in the case of negative or inconclusive findings (11). Local availability and expertise may influence the choice of imaging modality.
18F-FDG PET/CT Compared With Diffusion-Weighted MRI for Baseline Evaluation
DW-MRI is a newer technique that complements standard MRI sequences. Diffusion imaging studies the diffusion of water molecules within extracellular, intracellular, and intravascular spaces (38). Diffusion is restrained in tissues with high cellular density, reduced extracellular space, and if cellular membranes are intact (39). It can further increase diagnostic confidence of FL and diffuse disease with the help of the ADC measurement (40, 41). A few studies compared the diagnostic performance of DW-MRI with 18F-FDG PET/CT in a pairwise fashion. A large study of 227 NDMM found that, in about 10% of patients, DW-MRI was positive, but with no apparent disease on 18F-FDG PET (42). In that study, data from companion CT of PET were not used to correct the false-negative PET cases. These patients likely have a lower expression of hexokinase-2, therefore hampering 18F-FDG PET evaluation (42). Similar results were found by two other recent studies (43, 44). It was also shown that CT helps improve the diagnosis of bone involvement in patients with a low 18F-FDG uptake (44, 45). Comparison of the detectability of FLs showed that, overall, DW-MRI detects more FLs than does 18F-FDG PET/CT (44–46). Reports agree that 18F-FDG PET/CT appears to be superior for the detection of upper limb FLs, while DW-MRI seems better at analyzing the skull, the spine, and the pelvis (44, 46). A prospective study found that, although DW-MRI detected more FLs compared to 18F-FDG PET/CT, there was no difference regarding bone disease diagnosis on a per patient basis, with an agreement of 1.0 (44). This goes against a retrospective study that reported an overall sensitivity for detecting bone disease on a per patient basis of 69.6% for 18F-FDG PET/CT vs. 91.3% for DW-MRI using an independent clinical reference standard (45). However, the authors reported that performing DW-MRI in addition to 18F-FDG PET/CT did not significantly change the clinical decision on treatment (45).
Baseline Imaging Techniques as Prognostic Factors
The value of baseline 18F-FDG PET/CT as a prognostic factor has been demonstrated in historical cohorts, which consistently showed that FL maximum standardized uptake value (SUVmax) (cutoff >3.9 or >4.2), the number of FLs (>3FL), and EMD are independently associated with progression-free survival (PFS) and overall survival (OS) (15, 16, 47). In populations of patients treated with the current standard of care comprising immunomodulatory drugs, recent works have shown that EMD and >3FL maintain a predictive value for PFS and OS (48–53). The threshold of FL SUVmax appears, however, to be higher (>6.3) in order to maintain a significant association with OS in patients treated with newer drugs, possibly due to the more effective treatments (48). More advanced imaging markers are also under study. Whole-body metabolic tumor volume (MTV) and whole-body tumor lesion glycolysis (TLG) (i.e., MTVxSUVmean) are promising prognostic markers showing superiority in comparison with the baseline number of FLs or baseline SUVmax in a cohort of patients treated with chemotherapy comprising thalidomide, dexamethasone, cisplatin, cyclophosphamide, and etoposide, followed by intensification by melphalan, tandem autologous stem cell transplant (ASCT), and maintenance by thalidomide and dexamethasone (total therapy 3 protocol) (54). Similar results were found in a study of 185 NDMM patients treated with a proteasome inhibitor-based regimen (55).
In MM patients treated with the total therapy 3 protocol, a study of 611 patients showed that >7FL on AS-MRI had a negative impact on OS (56). Furthermore, the combination of >7FL on MRI with cytogenetic abnormalities defined a group with a dismal prognosis (56). This FL threshold was found to be higher (>25 FLs) in a large study of patients undergoing whole-body MRI with similar treatments (57). Regarding diffuse bone marrow disease on MRI, several reports have found it to be associated with higher plasma cell infiltration, higher lactate dehydrogenase (LDH), higher incidence of anemia, and worse survival (47, 58, 59). Limited data are available regarding the predictive value of baseline DW-MRI parameters for PFS or OS. In 404 transplant-eligible patients undergoing baseline DW-MRI, Rasche et al. found that the presence of more than 3 large FLs (>5 cm2) was a strong independent prognostic factor for PFS and OS (60). Additionally, the number of FLs on MRI lost its predictive value for outcomes when adjusting the size of FLs (60). This underlines the importance of tumor burden as a prognostic factor. It was also found that a high tumor burden was associated with a lack of spleen signal on DW-MRI (61). Another smaller prospective study found that baseline FL ADC was not a significant prognostic factor (49).
The predictive value for relapse and survival of the two imaging techniques at baseline is summarized in Table 1.
18F-FDG PET/CT and MRI for Treatment Response Evaluation
The value of 18F-FDG PET/CT and MRI as prognostic factors has been studied at different time points of MM treatment. The main characteristics of both modalities in the evaluation of treatment response are summarized in Table 2.
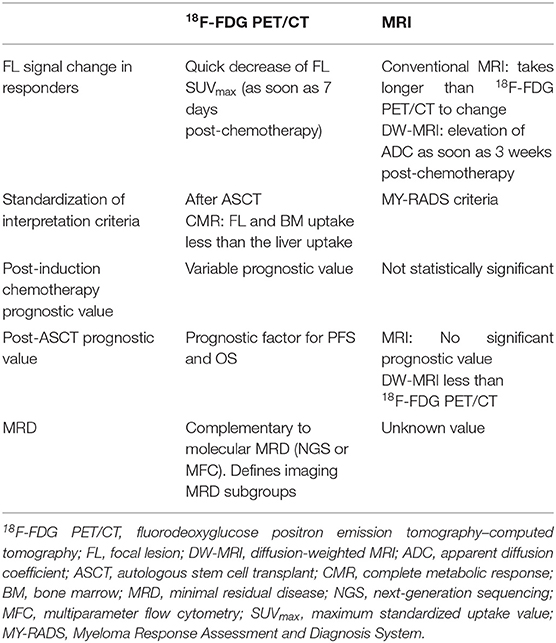
Table 2. Main characteristics of 18F-FDG PET/CT and MRI for multiple myeloma evaluation of response to treatment.
18F-FDG PET/CT Compared With Conventional MRI After Induction Chemotherapy
The team from the Bologna Center showed that SUVmax > 4.2 on 18F-FDG PET/CT performed after induction chemotherapy had a negative impact on the PFS of 85 patients treated with tandem transplant (16). These results slightly differed from those provided by the Little Rock (Arkansas) Center, which showed that the disappearance of FLs on pre-transplant 18F-FDG PET/CT had a positive impact on the PFS and OS of patients treated using the total therapy 3 protocol (51).
The multicenter IMAJEM Study considered post-induction 18F-FDG PET/CT as negative if the FL uptake was inferior or equal to the liver uptake in a mixed population of 134 patients treated either with a bortezomib-based chemotherapy (N = 71) followed by maintenance or with induction chemotherapy followed by ASCT, consolidation, and maintenance chemotherapy. The authors found no significant impact of the post-induction 18F-FDG PET/CT results on PFS and OS (17).
However, in a prospective study of 30 patients all treated with an immunomodulatory-based induction chemotherapy followed by ASCT and consolidation, post-induction 18F-FDG PET/CT showed a significant association with PFS with the same positivity threshold as that used in the IMAJEM Study (49).
Quantifying the variations in SUVmax is another interesting approach. In the 71 patients in the IMAJEM Study with a baseline uptake superior to that of the liver, a decrease of more than 25% of the SUVmax after induction chemotherapy was associated with a benefit in PFS, which was superior to that obtained with the biochemical evaluation of response (62).
The value of MRI after induction chemotherapy has mainly been studied in pairwise comparisons with 18F-FDG PET/CT. In a prospective study of 332 patients, only 64 patients had a negative MRI after induction chemotherapy, whereas 245 had a negative 18F-FDG PET/CT. Contrary to 18F-FDG PET/CT, post-induction MRI had no significant association with PFS or OS (47). The IMAJEM Study found that, in 134 patients, only 3% had a negative MRI after induction chemotherapy, whereas 32% had a negative 18F-FDG PET/CT (17). Similarly, there was no association of the MRI results with PFS or OS (17). These results illustrate the lack of specificity of the MRI signal after induction chemotherapy.
18F-FDG PET/CT Compared With Conventional MRI After ASCT
In patients treated with thalidomide and tandem ASCT, the Bologna team showed that a complete decrease of the FL SUVmax on post-transplant 18F-FDG PET/CT had a significant association with PFS and OS (16). The authors also found that all patients who had an SUVmax > 4.2 on post-ASCT 18F-FDG PET/CT relapsed during follow-up (63). Similarly, the team from Little Rock showed that a 100% decrease of FL uptake was associated with better PFS and OS, with prognosis similar to that of patients without FL at baseline (51).
The IMAJEM Study, using the liver SUVmax as the threshold for 18F-FDG PET/CT positivity, found that pre-maintenance 18F-FDG PET/CT had a strong association with PFS and OS as well, but only in the group of patients who underwent ASCT (17). Conversely, pre-maintenance MRI had no significant association with relapse or survival (17). The FL uptake of 18F-FDG PET/CT quickly changes after the start of MM treatment, whereas the FL signal on MRI will take longer to normalize after treatment (32, 64). In some cases, liquid transformation of focal lesions can occur, with an increase in the T2-weighted and DW imaging signals and in ADC, which will persist indefinitely.
A joint French–Italian cohort of transplant-eligible MM patients from the IFM/DFCI2009 and EMN02/HO95 studies confirmed the value of pre-maintenance 18F-FDG PET/CT as a prognostic factor for PFS and OS (65). This study also provided standardized criteria for post-ASCT response evaluation, further refining the IMPETUS criteria, with a complete metabolic response defined as a focal and/or diffuse bone marrow uptake lower than the liver background (30, 65).
Therefore, 18F-FDG PET/CT is recommended over MRI as it provides an earlier evaluation of the treatment response (66).
18F-FDG PET/CT Compared With Diffusion-Weighted MRI After ASCT
A few studies correlated the biochemical response of MM patients with DW-MRI ADC measurements (67–72). Different timings were used: either early (at 3 or 8 weeks post-chemotherapy) or later in the course of treatment (between 13 and 21 weeks post-chemotherapy). It is thought that an increase in the ADC would reflect tumor necrosis, microbleeding, tumor edema, and a decrease in cellular density (70). Conversely, a late evaluation would show a decrease in the ADC due to necrosis replacement by fatty bone marrow. Therefore, DW sequences could increase the specificity of MRI by providing a more dynamic evaluation of the FL response. Overall, studies agree that the ADC of FLs increases in patients with biochemical response, and thus at least until 21 weeks post-chemotherapy, whereas it is more uncertain in patients with diffuse bone marrow disease (68, 70–72). However, these studies suffer from a lack of harmonization regarding the time of MRI performance, the study population (newly diagnosed or treated MM), or the choice of b values for diffusion sequences. The Myeloma Response Assessment and Diagnosis System (MY-RADS) criteria have been recently published to address this need for harmonization by providing a classification of the probability of response or progression based on ADC measurements combined with morphological appreciation (73). These differed from the IMPETUS criteria, which provided a dichotomized classification of responses, individualizing high-risk patients.
Although the predictive value of 18F-FDG PET/CT on relapse and survival performed during treatment has been addressed, little is known about that of DW-MRI. A prospective study of 30 NDMM patients provided a head-to-head comparison of 18F-FDG PET/CT and DW-MRI performed after induction chemotherapy and after ASCT (49). 18F-FDG PET/CT was considered negative if the FL uptake was inferior to the liver uptake, and the DW-MRI response was addressed using the MY-RADS criteria (49). While 18F-FDG PET/CT showed a significant association with PFS at both times of treatment response evaluation, DW-MRI showed no value as a prognostic factor, within the limit of a small population (49). Similarly, another larger study comparing 18F-FDG PET/CT and DW-MRI without standardized reading criteria showed that the predictive value of 18F-FDG PET/CT on relapse was superior to that of DW-MRI (74). Larger standardized prospective studies are, however, warranted to confirm these results. Additionally, the complementary role of 18F-FDG PET/CT and DW-MRI should be further addressed, for example through studies performed on PET/MRI hybrid systems (75).
Functional Imaging Complementarity With Biological MRD
Biological minimal residual disease (MRD) evaluation refers to highly sensitive techniques such as multiparameter flow cytometry (MFC) or next-generation sequencing (NGS), with a ratio of detection of 1 clonal cell in 105–106 normal cells on bone marrow biopsy (67–70). MRD evaluation is standardized by IMWG recommendations, which defined a new category of response depending on the results of MRD among patients with biochemical complete remission (CR) (76). In the IFM 2009 study, patients with biochemical CR and positive MRD by NGS had a 3-year PFS of 42 vs. 87% in patients with CR and negative MRD (77).
These highly sensitive molecular techniques can raise the question of the utility of imaging procedures. To address this matter, the IMAJEM Study showed that the concordance of pre-maintenance 18F-FDG PET/CT with the MRD results was low. In a group of 86 patients, 18F-FDG PET/CT and MRD double-negative patients had a 3-year PFS of 86.8% compared to 52.9% in patients with either 18F-FDG PET/CT and/or positive MRD (17). The CASSIOPET Study also found in 176 NDMM patients eligible for transplant that the concordance between MRD and post-consolidation 18F-FDG PET/CT was low (78). This illustrates the complementarity of both approaches. Indeed, MRD may be negative in patients with tumor clones outside the field of bone marrow sample either in the case of FLs outside the pelvic area or in case of EMD. Still, the frequent relapses of MRD-negative patients with CR support the idea of MRD persisting outside the pelvis (79). Evaluation of the role of potentially more sensitive and specific PET tracers in the detection of MRD and for patient prognostication is also awaited. Pilot observational studies using 18F-choline, 11C-methionine, 11C-acetate, or immuno-PET tracers targeting CD38 have provided the first steps to promising results (80–83). Studies evaluating the concordance of DW-MRI and biological MRD are also warranted.
Conclusion
Whole-body functional imaging performed with 18F-FDG PET/CT or DW-MRI is a key player in the identification of NDMM patients needing treatment. The sensitivity of DW-MRI is superior to that of 18F-FDG PET/CT for the detection of bone involvement, and both modalities are crucial to detecting EMD and PMD (Table 1). Imaging modalities and reading are now standardized for both of these techniques, potentially adding to their clinical robustness. During treatment, the value of DW-MRI as a prognostic factor appears to be lower than that of 18F-FDG PET/CT, but larger prospective studies are warranted on this matter. 18F-FDG PET/CT remains the imaging modality of choice for monitoring treatment response, especially in patients undergoing ASCT, defining imaging-MRD that complements molecular/cellular MRD measurements (Table 2). The strengths of 18F-FDG PET/CT lie in its relative simplicity of interpretation and quantification, while the complexity of MRI leaves room for variability in its interpretation and quantification, therefore requiring longer training and strict harmonization. Future studies will have to further address the complementarity of DW-MRI and 18F-FDG PET/CT during the treatment of MM patients. Trials integrating imaging MRD measurements with 18F-FDG PET/CT for treatment decisions will be needed to further assess its prognostic value. This review mainly focused on the initial management of NDMM patients. Fewer studies considering their respective roles in the first and subsequent relapses are available. Again, more studies are needed to address the role of novel therapies involving antibodies, antibody–drug conjugates, bispecific antibodies, and chimeric antigen receptor (CAR) T cells. The possible roles of new promising PET tracers in the improvement of MRD assessment will also have to be addressed.
Author Contributions
CM and EH wrote the manuscript. All authors contributed to the article and approved the submitted version.
Conflict of Interest
The authors declare that the research was conducted in the absence of any commercial or financial relationships that could be construed as a potential conflict of interest.
Publisher's Note
All claims expressed in this article are solely those of the authors and do not necessarily represent those of their affiliated organizations, or those of the publisher, the editors and the reviewers. Any product that may be evaluated in this article, or claim that may be made by its manufacturer, is not guaranteed or endorsed by the publisher.
References
1. Durie BG, Salmon SE. A clinical staging system for multiple myeloma. Correlation of measured myeloma cell mass with presenting clinical features, response to treatment, and survival. Cancer. (1975) 36:842–54. doi: 10.1002/1097-0142(197509)36:3<842::AID-CNCR2820360303>3.0.CO;2-U
2. Edelstyn GA, Gillespie PJ, Grebbell FS. The radiological demonstration of osseous metastases. Experimental observations. Clin Radiol. (1967) 18:158–62. doi: 10.1016/S0009-9260(67)80010-2
3. Durie BG. The role of anatomic and functional staging in myeloma: description of Durie/Salmon plus staging system. Eur J Cancer. (2006) 42:1539–43. doi: 10.1016/j.ejca.2005.11.037
4. Rajkumar SV, Dimopoulos MA, Palumbo A, Blade J, Merlini G, Mateos MV, et al. International Myeloma Working Group updated criteria for the diagnosis of multiple myeloma. The lancet oncology. (2014) 15:e538–48. doi: 10.1016/S1470-2045(14)70442-5
5. Horger M, Claussen CD, Bross-Bach U, Vonthein R, Trabold T, Heuschmid M, et al. Whole-body low-dose multidetector row-CT in the diagnosis of multiple myeloma: an alternative to conventional radiography. Eur J Radiol. (2005) 54:289–97. doi: 10.1016/j.ejrad.2004.04.015
6. Kropil P, Fenk R, Fritz LB, Blondin D, Kobbe G, Modder U, et al. Comparison of whole-body 64-slice multidetector computed tomography and conventional radiography in staging of multiple myeloma. Eur Radiol. (2008) 18:51–8. doi: 10.1007/s00330-007-0738-3
7. Gleeson TG, Moriarty J, Shortt CP, Gleeson JP, Fitzpatrick P, Byrne B, et al. Accuracy of whole-body low-dose multidetector CT (WBLDCT) versus skeletal survey in the detection of myelomatous lesions, and correlation of disease distribution with whole-body MRI (WBMRI). Skeletal Radiol. (2009) 38:225–36. doi: 10.1007/s00256-008-0607-4
8. Wolf MB, Murray F, Kilk K, Hillengass J, Delorme S, Heiss C, et al. Sensitivity of whole-body CT and MRI versus projection radiography in the detection of osteolyses in patients with monoclonal plasma cell disease. Eur J Radiol. (2014) 83:1222–30. doi: 10.1016/j.ejrad.2014.02.008
9. Hillengass J, Moulopoulos LA, Delorme S, Koutoulidis V, Mosebach J, Hielscher T, et al. Whole-body computed tomography versus conventional skeletal survey in patients with multiple myeloma: a study of the International Myeloma Working Group. Blood Cancer J. (2017) 7:e599. doi: 10.1038/bcj.2017.78
10. Moulopoulos LA, Koutoulidis V, Hillengass J, Zamagni E, Aquerreta JD, Roche CL, et al. Recommendations for acquisition, interpretation and reporting of whole body low dose CT in patients with multiple myeloma and other plasma cell disorders: a report of the IMWG Bone Working Group. Blood Cancer J. (2018) 8:95. doi: 10.1038/s41408-018-0124-1
11. Hillengass J, Usmani S, Rajkumar SV, Durie BGM, Mateos MV, Lonial S, et al. International myeloma working group consensus recommendations on imaging in monoclonal plasma cell disorders. Lancet Oncol. (2019) 20:e302–e12. doi: 10.1016/S1470-2045(19)30309-2
12. Moreau P, San Miguel J, Sonneveld P, Mateos MV, Zamagni E, Avet-Loiseau H, et al. Multiple myeloma: ESMO clinical practice guidelines for diagnosis, treatment and follow-up. Ann Oncol. (2017) 28:iv52–61. doi: 10.1093/annonc/mdx096
13. Caers J, Garderet L, Kortum KM, O'Dwyer ME, van de Donk N, Binder M, et al. European Myeloma Network recommendations on tools for the diagnosis and monitoring of multiple myeloma: what to use and when. Haematologica. (2018) 103:1772–84. doi: 10.3324/haematol.2018.189159
14. Prieto E, Garcia-Velloso MJ, Aquerreta JD, Rosales JJ, Bastidas JF, Soriano I, et al. Ultra-low dose whole-body CT for attenuation correction in a dual tracer PET/CT protocol for multiple myeloma. Phys Med. (2021) 84:1–9. doi: 10.1016/j.ejmp.2021.03.019
15. Bartel TB, Haessler J, Brown TL, Shaughnessy JD Jr, van Rhee F, et al. F18-fluorodeoxyglucose positron emission tomography in the context of other imaging techniques and prognostic factors in multiple myeloma. Blood. (2009) 114:2068–76. doi: 10.1182/blood-2009-03-213280
16. Zamagni E, Patriarca F, Nanni C, Zannetti B, Englaro E, Pezzi A, et al. Prognostic relevance of 18-F FDG PET/CT in newly diagnosed multiple myeloma patients treated with up-front autologous transplantation. Blood. (2011) 118:5989–95. doi: 10.1182/blood-2011-06-361386
17. Moreau P, Attal M, Caillot D, Macro M, Karlin L, Garderet L, et al. Prospective evaluation of magnetic resonance imaging and [(18)F]Fluorodeoxyglucose positron emission tomography-computed tomography at diagnosis and before maintenance therapy in symptomatic patients with multiple myeloma included in the IFM/DFCI 2009 Trial: results of the IMAJEM study. J Clin Oncol. (2017) 35:2911–8. doi: 10.1200/JCO.2017.72.2975
18. Baur A, Stabler A, Nagel D, Lamerz R, Bartl R, Hiller E, et al. Magnetic resonance imaging as a supplement for the clinical staging system of Durie and Salmon? Cancer. (2002) 95:1334–45. doi: 10.1002/cncr.10818
19. Kloth JK, Hillengass J, Listl K, Kilk K, Hielscher T, Landgren O, et al. Appearance of monoclonal plasma cell diseases in whole-body magnetic resonance imaging and correlation with parameters of disease activity. Int J Cancer. (2014) 135:2380–6. doi: 10.1002/ijc.28877
20. Mesguich C, Fardanesh R, Tanenbaum L, Chari A, Jagannath S, Kostakoglu L. State of the art imaging of multiple myeloma: comparative review of FDG PET/CT imaging in various clinical settings. Eur J Radiol. (2014) 83:2203–23. doi: 10.1016/j.ejrad.2014.09.012
21. Stabler A, Baur A, Bartl R, Munker R, Lamerz R, Reiser MF. Contrast enhancement and quantitative signal analysis in MR imaging of multiple myeloma: assessment of focal and diffuse growth patterns in marrow correlated with biopsies and survival rates. AJR Am J Roentgenol. (1996) 167:1029–36. doi: 10.2214/ajr.167.4.8819407
22. Zamagni E, Nanni C, Patriarca F, Englaro E, Castellucci P, Geatti O, et al. A prospective comparison of 18F-fluorodeoxyglucose positron emission tomography-computed tomography, magnetic resonance imaging and whole-body planar radiographs in the assessment of bone disease in newly diagnosed multiple myeloma. Haematologica. (2007) 92:50–5. doi: 10.3324/haematol.10554
23. Usmani SZ, Heuck C, Mitchell A, Szymonifka J, Nair B, Hoering A, et al. Extramedullary disease portends poor prognosis in multiple myeloma and is over-represented in high-risk disease even in the era of novel agents. Haematologica. (2012) 97:1761–7. doi: 10.3324/haematol.2012.065698
24. Sachpekidis C, Hillengass J, Goldschmidt H, Mosebach J, Pan L, Schlemmer HP, et al. Comparison of (18)F-FDG PET/CT and PET/MRI in patients with multiple myeloma. Am J Nucl Med Mol Imaging. (2015) 5:469–78.
25. Sevcikova S, Minarik J, Stork M, Jelinek T, Pour L, Hajek R. Extramedullary disease in multiple myeloma - controversies and future directions. Blood Rev. (2019) 36:32–9. doi: 10.1016/j.blre.2019.04.002
26. Weinstock M, Ghobrial IM. Extramedullary multiple myeloma. Leukemia Lymphoma. (2013) 54:1135–41. doi: 10.3109/10428194.2012.740562
27. Gagelmann N, Eikema DJ, Iacobelli S, Koster L, Nahi H, Stoppa AM, et al. Impact of extramedullary disease in patients with newly diagnosed multiple myeloma undergoing autologous stem cell transplantation: a study from the Chronic Malignancies Working Party of the EBMT. Haematologica. (2018) 103:890–7. doi: 10.3324/haematol.2017.178434
28. Deng S, Xu Y, An G, Sui W, Zou D, Zhao Y, et al. Features of extramedullary disease of multiple myeloma: high frequency of p53 deletion and poor survival: a retrospective single-center study of 834 cases. Clin Lymphoma Myeloma Leuk. (2015) 15:286–91. doi: 10.1016/j.clml.2014.12.013
29. Nanni C, Zamagni E, Versari A, Chauvie S, Bianchi A, Rensi M, et al. Image interpretation criteria for FDG PET/CT in multiple myeloma: a new proposal from an Italian expert panel. IMPeTUs (Italian Myeloma criteria for PET USe). Eur J Nucl Med Mol Imaging. (2015) 43:414–21. doi: 10.1007/s00259-015-3200-9
30. Nanni C, Versari A, Chauvie S, Bertone E, Bianchi A, Rensi M, et al. Interpretation criteria for FDG PET/CT in multiple myeloma (IMPeTUs): final results. IMPeTUs (Italian myeloma criteria for PET USe). Eur J Nucl Med Mol Imaging. (2018) 45:712–9. doi: 10.1007/s00259-017-3909-8
31. Fonti R, Salvatore B, Quarantelli M, Sirignano C, Segreto S, Petruzziello F, et al. 18F-FDG PET/CT, 99mTc-MIBI, and MRI in evaluation of patients with multiple myeloma. J Nucl Med. (2008) 49:195–200. doi: 10.2967/jnumed.107.045641
32. Spinnato P, Bazzocchi A, Brioli A, Nanni C, Zamagni E, Albisinni U, et al. Contrast enhanced MRI and (1)(8)F-FDG PET-CT in the assessment of multiple myeloma: a comparison of results in different phases of the disease. Eur J Radiol. (2012) 81:4013–8. doi: 10.1016/j.ejrad.2012.06.028
33. Nanni C, Zamagni E, Farsad M, Castellucci P, Tosi P, Cangini D, et al. Role of 18F-FDG PET/CT in the assessment of bone involvement in newly diagnosed multiple myeloma: preliminary results. Eur J Nucl Med Mol Imaging. (2006) 33:525–31. doi: 10.1007/s00259-005-0004-3
34. Moreau P, Attal M, Karlin L, Garderet L, Facon T, Macro M, et al. Prospective evaluation of MRI and PET-CT at diagnosis and before maintenance therapy in symptomatic patients with multiple myeloma included in the IFM/DFCI 2009 Trial. Blood. (2015) 126:395–. doi: 10.1182/blood.V126.23.395.395
35. Bauerle T, Hillengass J, Fechtner K, Zechmann CM, Grenacher L, Moehler TM, et al. Multiple myeloma and monoclonal gammopathy of undetermined significance: importance of whole-body versus spinal MR imaging. Radiology. (2009) 252:477–85. doi: 10.1148/radiol.2522081756
36. Shortt CP, Gleeson TG, Breen KA, McHugh J, O'Connell MJ, O'Gorman PJ, et al. Whole-Body MRI versus PET in assessment of multiple myeloma disease activity. AJR Am J Roentgenol. (2009) 192:980–6. doi: 10.2214/AJR.08.1633
37. Lecouvet FE, Boyadzhiev D, Collette L, Berckmans M, Michoux N, Triqueneaux P, et al. MRI versus (18)F-FDG-PET/CT for detecting bone marrow involvement in multiple myeloma: diagnostic performance and clinical relevance. Eur Radiol. (2020) 30:1927–37. doi: 10.1007/s00330-019-06469-1
38. Koh DM, Collins DJ. Diffusion-weighted MRI in the body: applications and challenges in oncology. AJR Am J Roentgenol. (2007) 188:1622–35. doi: 10.2214/AJR.06.1403
39. Khoo MM, Tyler PA, Saifuddin A, Padhani AR. Diffusion-weighted imaging (DWI) in musculoskeletal MRI: a critical review. Skeletal Radiol. (2011) 40:665–81. doi: 10.1007/s00256-011-1106-6
40. Messiou C, Collins DJ, Morgan VA, Desouza NM. Optimising diffusion weighted MRI for imaging metastatic and myeloma bone disease and assessing reproducibility. Eur Radiol. (2011) 21:1713–8. doi: 10.1007/s00330-011-2116-4
41. Koutoulidis V, Fontara S, Terpos E, Zagouri F, Matsaridis D, Christoulas D, et al. Quantitative diffusion-weighted imaging of the bone marrow: an adjunct tool for the diagnosis of a diffuse MR imaging pattern in patients with multiple myeloma. Radiology. (2017) 282:484–93. doi: 10.1148/radiol.2016160363
42. Rasche L, Angtuaco E, McDonald JE, Buros A, Stein C, Pawlyn C, et al. Low expression of hexokinase-2 is associated with false-negative FDG-positron emission tomography in multiple myeloma. Blood. (2017) 130:30–4. doi: 10.1182/blood-2017-03-774422
43. Abe Y, Ikeda S, Kitadate A, Narita K, Kobayashi H, Miura D, et al. Low hexokinase-2 expression-associated false-negative (18)F-FDG PET/CT as a potential prognostic predictor in patients with multiple myeloma. Eur J Nucl Med Mol Imaging. (2019) 46:1345–50. doi: 10.1007/s00259-019-04312-9
44. Mesguich C, Hulin C, Latrabe V, Lascaux A, Bordenave L, Hindie E, et al. Prospective comparison of 18-FDG PET/CT and whole-body diffusion-weighted MRI in the assessment of multiple myeloma. Ann Hematol. (2020) 99:2869–80. doi: 10.1007/s00277-020-04265-2
45. Westerland O, Amlani A, Kelly-Morland C, Fraczek M, Bailey K, Gleeson M, et al. Comparison of the diagnostic performance and impact on management of 18F-FDG PET/CT and whole-body MRI in multiple myeloma. Eur J Nucl Med Mol Imaging. (2021) 48:2558–65. doi: 10.1007/s00259-020-05182-2
46. Messiou C, Porta N, Sharma B, Levine D, Koh DM, Boyd K, et al. Prospective evaluation of whole-body MRI versus FDG PET/CT for lesion detection in participants with myeloma. Radiol Imaging Cancer. (2021) 3:e210048. doi: 10.1148/rycan.2021210048
47. Usmani SZ, Mitchell A, Waheed S, Crowley J, Hoering A, Petty N, et al. Prognostic implications of serial 18-fluoro-deoxyglucose emission tomography in multiple myeloma treated with total therapy 3. Blood. (2013) 121:1819–23. doi: 10.1182/blood-2012-08-451690
48. Michaud-Robert AV, Zamagni E, Carlier T, Bailly C, Jamet B, Touzeau C, et al. Glucose metabolism quantified by SUVmax on baseline FDG-PET/CT predicts survival in newly diagnosed multiple myeloma patients: combined harmonized analysis of two prospective phase III trials. Cancers (Basel). (2020) 12:2532. doi: 10.3390/cancers12092532
49. Mesguich C, Latrabe V, Hulin C, Lascaux A, Bordenave L, Hindie E, et al. Prospective comparison of 18-FDG PET/CT and whole-body MRI with diffusion-weighted imaging in the evaluation of treatment response of multiple myeloma patients eligible for autologous stem cell transplant. Cancers (Basel). (2021) 13:1938. doi: 10.3390/cancers13081938
50. Park S, Lee SJ, Chang WJ, Maeng CH, Hong JY, Choi MK, et al. Positive correlation between baseline PET or PET/CT findings and clinical parameters in multiple myeloma patients. Acta Haematol. (2013) 131:193–9. doi: 10.1159/000354839
51. Davies FE, Rosenthal A, Rasche L, Petty NM, McDonald JE, Ntambi JA, et al. Treatment to suppression of focal lesions on positron emission tomography-computed tomography is a therapeutic goal in newly diagnosed multiple myeloma. Haematologica. (2018) 103:1047–53. doi: 10.3324/haematol.2017.177139
52. Jung SH, Kwon SY, Min JJ, Bom HS, Ahn SY, Jung SY, et al. (18)F-FDG PET/CT is useful for determining survival outcomes of patients with multiple myeloma classified as stage II and III with the Revised International Staging System. Eur J Nucl Med Mol Imaging. (2019) 46:107–15. doi: 10.1007/s00259-018-4114-0
53. Abe Y, Narita K, Kobayashi H, Kitadate A, Takeuchi M, O'Uchi T, et al. Medullary abnormalities in appendicular skeletons detected with (18)F-FDG PET/CT predict an unfavorable prognosis in newly diagnosed multiple myeloma patients with high-risk factors. AJR Am J Roentgenol. (2019) 213:918–24. doi: 10.2214/AJR.19.21283
54. McDonald JE, Kessler MM, Gardner MW, Buros AF, Ntambi JA, Waheed S, et al. Assessment of total lesion glycolysis by (18)F FDG PET/CT significantly improves prognostic value of GEP and ISS in myeloma. Clin Cancer Res. (2017) 23:1981–7. doi: 10.1158/1078-0432.CCR-16-0235
55. Terao T, Machida Y, Tsushima T, Miura D, Narita K, Kitadate A, et al. Pre-treatment metabolic tumour volume and total lesion glycolysis are superior to conventional positron-emission tomography/computed tomography variables for outcome prediction in patients with newly diagnosed multiple myeloma in clinical practice. Br J Haematol. (2020) 191:223–30. doi: 10.1111/bjh.16633
56. Walker R, Barlogie B, Haessler J, Tricot G, Anaissie E, Shaughnessy JD, et al. Magnetic resonance imaging in multiple myeloma: diagnostic and clinical implications. J Clin Oncol. (2007) 25:1121–8. doi: 10.1200/JCO.2006.08.5803
57. Mai EK, Hielscher T, Kloth JK, Merz M, Shah S, Raab MS, et al. A magnetic resonance imaging-based prognostic scoring system to predict outcome in transplant-eligible patients with multiple myeloma. Haematologica. (2015) 100:818–25. doi: 10.3324/haematol.2015.124115
58. Moulopoulos LA, Dimopoulos MA, Kastritis E, Christoulas D, Gkotzamanidou M, Roussou M, et al. Diffuse pattern of bone marrow involvement on magnetic resonance imaging is associated with high risk cytogenetics and poor outcome in newly diagnosed, symptomatic patients with multiple myeloma: a single center experience on 228 patients. Am J Hematol. (2012) 87:861–4. doi: 10.1002/ajh.23258
59. Mai EK, Hielscher T, Kloth JK, Merz M, Shah S, Hillengass M, et al. Association between magnetic resonance imaging patterns and baseline disease features in multiple myeloma: analyzing surrogates of tumour mass and biology. Eur Radiol. (2016) 26:3939–48. doi: 10.1007/s00330-015-4195-0
60. Rasche L, Angtuaco EJ, Alpe TL, Gershner GH, McDonald JE, Samant RS, et al. The presence of large focal lesions is a strong independent prognostic factor in multiple myeloma. Blood. (2018) 132:59–66. doi: 10.1182/blood-2018-04-842880
61. Rasche L, Kumar M, Gershner G, Samant R, Van Hemert R, Heidemeier A, et al. Lack of spleen signal on diffusion weighted MRI is associated with high tumor burden and poor prognosis in multiple myeloma: a link to extramedullary hematopoiesis? Theranostics. (2019) 9:4756–63. doi: 10.7150/thno.33289
62. Bailly C, Carlier T, Jamet B, Eugene T, Touzeau C, Attal M, et al. Interim PET analysis in first-line therapy of multiple myeloma: prognostic value of DeltaSUVmax in the FDG-avid patients of the IMAJEM study. Clin Cancer Res. (2018) 24:5219–24. doi: 10.1158/1078-0432.CCR-18-0741
63. Nanni C, Zamagni E, Celli M, Caroli P, Ambrosini V, Tacchetti P, et al. The value of 18F-FDG PET/CT after autologous stem cell transplantation (ASCT) in patients affected by multiple myeloma (MM): experience with 77 patients. Clin Nucl Med. (2013) 38:e74–9. doi: 10.1097/RLU.0b013e318266cee2
64. Derlin T, Peldschus K, Munster S, Bannas P, Herrmann J, Stubig T, et al. Comparative diagnostic performance of (1)(8)F-FDG PET/CT versus whole-body MRI for determination of remission status in multiple myeloma after stem cell transplantation. Eur Radiol. (2013) 23:570–8. doi: 10.1007/s00330-012-2600-5
65. Zamagni E, Nanni C, Dozza L, Carlier T, Bailly C, Tacchetti P, et al. Standardization of (18)F-FDG-PET/CT according to deauville criteria for metabolic complete response definition in newly diagnosed multiple myeloma. J Clin Oncol. (2021) 39:116–25. doi: 10.1200/JCO.20.00386
66. Cavo M, Terpos E, Nanni C, Moreau P, Lentzsch S, Zweegman S, et al. Role of (18)F-FDG PET/CT in the diagnosis and management of multiple myeloma and other plasma cell disorders: a consensus statement by the International Myeloma Working Group. Lancet Oncol. (2017) 18:e206–e17. doi: 10.1016/S1470-2045(17)30189-4
67. Horger M, Weisel K, Horger W, Mroue A, Fenchel M, Lichy M. Whole-body diffusion-weighted MRI with apparent diffusion coefficient mapping for early response monitoring in multiple myeloma: preliminary results. AJR Am J Roentgenol. (2011) 196:W790–5. doi: 10.2214/AJR.10.5979
68. Messiou C, Giles S, Collins DJ, West S, Davies FE, Morgan GJ, et al. Assessing response of myeloma bone disease with diffusion-weighted MRI. Br J Radiol. (2012) 85:e1198–203. doi: 10.1259/bjr/52759767
69. Latifoltojar A, Hall-Craggs M, Bainbridge A, Rabin N, Popat R, Rismani A, et al. Whole-body MRI quantitative biomarkers are associated significantly with treatment response in patients with newly diagnosed symptomatic multiple myeloma following bortezomib induction. Eur Radiol. (2017) 27:5325–36. doi: 10.1007/s00330-017-4907-8
70. Giles SL, Messiou C, Collins DJ, Morgan VA, Simpkin CJ, West S, et al. Whole-body diffusion-weighted MR imaging for assessment of treatment response in myeloma. Radiology. (2014) 271:785–94. doi: 10.1148/radiol.13131529
71. Lacognata C, Crimi F, Guolo A, Varin C, De March E, Vio S, et al. Diffusion-weighted whole-body MRI for evaluation of early response in multiple myeloma. Clin Radiol. (2017) 72:850–7. doi: 10.1016/j.crad.2017.05.004
72. Zhang Y, Xiong X, Fu Z, Dai H, Yao F, Liu D, et al. Whole-body diffusion-weighted MRI for evaluation of response in multiple myeloma patients following bortezomib-based therapy: a large single-center cohort study. Eur J Radiol. (2019) 120:108695. doi: 10.1016/j.ejrad.2019.108695
73. Messiou C, Hillengass J, Delorme S, Lecouvet FE, Moulopoulos LA, Collins DJ, et al. Guidelines for acquisition, interpretation, and reporting of whole-body MRI in myeloma: myeloma response assessment and diagnosis system (MY-RADS). Radiology. (2019) 291:5–13. doi: 10.1148/radiol.2019181949
74. Rasche L, Alapat D, Kumar M, Gershner G, McDonald J, Wardell CP, et al. Combination of flow cytometry and functional imaging for monitoring of residual disease in myeloma. Leukemia. (2019) 33:1713–22. doi: 10.1038/s41375-018-0329-0
75. Mule S, Reizine E, Blanc-Durand P, Baranes L, Zerbib P, Burns R, et al. Whole-body functional MRI and PET/MRI in multiple myeloma. Cancers (Basel). (2020) 12:3155. doi: 10.3390/cancers12113155
76. Kumar S, Paiva B, Anderson KC, Durie B, Landgren O, Moreau P, et al. International Myeloma Working Group consensus criteria for response and minimal residual disease assessment in multiple myeloma. Lancet Oncol. (2016) 17:e328–e46. doi: 10.1016/S1470-2045(16)30206-6
77. Avet-Loiseau H, Corre J, Lauwers-Cances V, Chretien M-L, Robillard N, Leleu X, et al. Evaluation of | (MRD) by next generation sequencing (NGS) Is Highly Predictive Of Progression Free Survival In The IFM/DFCI 2009 trial. Blood. (2015) 126:191–. doi: 10.1182/blood.V126.23.191.191
78. Moreau P, Zweegman S, Perrot A, Hulin C, Caillot D, Facon T, et al. Evaluation of the prognostic value of positron emission tomography-computed tomography (PET-CT) at diagnosis and follow-up in transplant-eligible newly diagnosed multiple myeloma (TE NDMM) patients treated in the phase 3 cassiopeia study: results of the cassiopet companion study. Blood. (2019) 134:692. doi: 10.1182/blood-2019-123143
79. Rasche L, Kortum KM, Raab MS, Weinhold N. The impact of tumor heterogeneity on diagnostics and novel therapeutic strategies in multiple myeloma. Int J Mol Sci. (2019) 20:1248. doi: 10.3390/ijms20051248
80. Cassou-Mounat T, Balogova S, Nataf V, Calzada M, Huchet V, Kerrou K, et al. 18F-fluorocholine versus 18F-fluorodeoxyglucose for PET/CT imaging in patients with suspected relapsing or progressive multiple myeloma: a pilot study. Eur J Nucl Med Mol Imaging. (2016) 43:1995–2004. doi: 10.1007/s00259-016-3392-7
81. Lapa C, Garcia-Velloso MJ, Luckerath K, Samnick S, Schreder M, Otero PR, et al. (11)C-methionine-PET in multiple myeloma: a combined study from two different institutions. Theranostics. (2017) 7:2956–64. doi: 10.7150/thno.20491
82. Fontana F, Ge X, Su X, Hathi D, Xiang J, Cenci S, et al. Evaluating acetate metabolism for imaging and targeting in multiple myeloma. Clin Cancer Res. (2017) 23:416–29. doi: 10.1158/1078-0432.CCR-15-2134
Keywords: FDG-PET/CT, MRI, diffusion-weighted (DW) MRI, multiple myeloma, minimal residual disease (MRD)
Citation: Mesguich C, Hulin C, Latrabe V, Lascaux A, Bordenave L and Hindié E (2022) 18F-FDG PET/CT and MRI in the Management of Multiple Myeloma: A Comparative Review. Front. Nucl. Med. 1:808627. doi: 10.3389/fnume.2021.808627
Received: 03 November 2021; Accepted: 27 December 2021;
Published: 22 February 2022.
Edited by:
Constantin Lapa, University of Augsburg, GermanyReviewed by:
Cristina Nanni, Sant'Orsola-Malpighi Polyclinic, ItalyStefan Delorme, German Cancer Research Center (DKFZ), Germany
Copyright © 2022 Mesguich, Hulin, Latrabe, Lascaux, Bordenave and Hindié. This is an open-access article distributed under the terms of the Creative Commons Attribution License (CC BY). The use, distribution or reproduction in other forums is permitted, provided the original author(s) and the copyright owner(s) are credited and that the original publication in this journal is cited, in accordance with accepted academic practice. No use, distribution or reproduction is permitted which does not comply with these terms.
*Correspondence: Charles Mesguich, Y2hhcmxlcy5tZXNndWljaEBjaHUtYm9yZGVhdXguZnI=