- 1Karlsruhe Institute of Technology, Institute for Nuclear Waste Disposal, Karlsruhe, Germany
- 2Svensk Kärnbränslehantering AB, Solna, Sweden
The uptake of α-hydroxyisobutyric acid (HIBA), 3-hydroxybutyric acid (HBA) and glutaric acid (GTA) by hardened cement paste (HCP) in the degradation stage II was investigated at various ligand concentrations (10−7 M ≤ [L]tot ≤ 0.1 M) and solid-to-liquid ratios (0.2 g⋅dm−3 ≤ S:L ≤ 50 g⋅dm−3). These organic ligands were previously identified as representative of the main degradation products of UP2W, a polyacrylonitrile-based material used as filter aid in nuclear power plants, under repository conditions. Sorption experiments were conducted with inactive (HIBA, HBA, GTA) and active (14C-labelled GTA) organic ligands. Sorption experiments show a weak uptake of HIBA and HBA by HCP, with distribution coefficients determined as Rd (HIBA) = (2.2 ± 1.3)⋅10−3 m3⋅kg−1 and Rd (HBA) = (1.6 ± 0.8)⋅10−3 m3⋅kg−1. A stronger uptake is observed for GTA, i.e. Rd (GTA) = (1.3 ± 0.5)⋅10−2 m3⋅kg−1, likely reflecting the contribution from the ligand’s second carboxylate group. GTA follows a linear sorption behaviour within 10−7 M ≤ [GTA]tot ≤ 0.1 M, which was successfully modelled with a one-site Langmuir isotherm. The adsorption capacity determined for the uptake of GTA by HCP is slightly higher but in line with the capacity previously reported for isosaccharinic acid (ISA), whereas the affinity constant derived for GTA is significantly lower than values reported for stronger binding sites in HCP for the uptake of ISA. HIBA and HBA have a minor impact on the surface charge of HCP up to [L]tot ≈ 0.1 M. On the contrary, GTA induces a clear decrease in the surface charge above [GTA]tot ≈ 10−3 M resulting in an isoelectric point at [GTA]tot ≈ 6⋅10−2 M. Comparison of sorption data obtained in this work and reported in the literature for organic ligands containing the functional groups -COOH and -OH underlines the key role of multiple functionalities as a factor strengthening the interaction with the HCP surface. The participation of alcohol groups is particularly strong when present in their deprotonated state. These results provide information to quantitatively assess the uptake by HCP of organic ligands relevant in the context of nuclear waste disposal, and to understand their impact on the surface properties of cement.
1 Introduction
A large variety of organic materials are disposed in repositories for low and intermediate level radioactive waste (L/ILW). One of these materials is UP2W, a polyacrylonitrile-based polymer (PAN, see Figure 1) used in nuclear power plants as filter aid in condensate clean-up, which reduces clogging and thus improves filtration (Dario et al., 2004; Keith-Roach et al., 2021). Significant amounts of used UP2W are disposed of in SFR, the repository for short-lived L/ILW in Sweden (Dario et al., 2004; Duro et al., 2012; Keith-Roach et al., 2014; Keith-Roach et al., 2021).
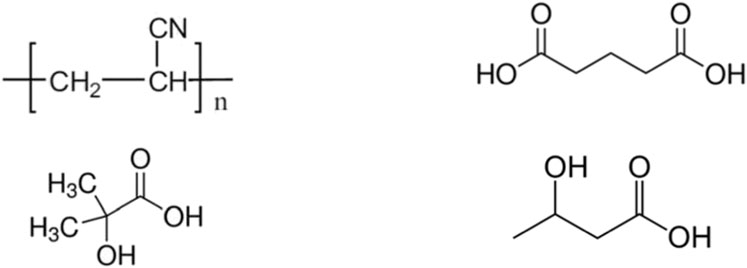
FIGURE 1. Chemical formula of polyacrylnitrile (PAN, upper left), glutaric acid (GTA, upper right), α-hydroxyisobutyric acid (HIBA, bottom left) and 3-hydroxybutyric acid (HBA, bottom right).
Cementitious materials are extensively used in L/ILW repositories for construction purposes and for the stabilization and conditioning of the waste. These materials have been described to strongly sorb radionuclides, primarily metal ions and cationic species, but also (to lesser extent) anions including organic ligands (Van Loon et al., 1997; Pointeau et al., 2008; Wieland, 2014; Ochs et al., 2016; Wieland et al., 2016; Garcia et al., 2020). Cementitious materials also buffer the porewater composition at hyperalkaline pH conditions (10 ≤ pH ≤ 13.3) with Ca concentrations of up to ∼0.02 M. These specific chemical boundary conditions strongly affect the solution chemistry of the radionuclides and inorganic or organic ligands eventually present in the repository.
The degradation of organic materials under the hyperalkaline pH conditions defined by cement may result in the formation of organic ligands able to form stable complexes with radionuclides, thus affecting the retention of the latter in the repository. Moreover, these organic ligands can sorb onto cement, accordingly modifying the surface properties of this material and potentially alter the affinity of radionuclides for such surfaces (Pointeau et al., 2006a; Pointeau et al., 2006b; Pointeau et al., 2008; Barzgar et al., 2020; Tasi et al., 2021a). The organic compounds glutaric acid (GTA), α-hydroxyisobutyric acid (HIBA) and 3-hydroxybutyric acid (HBA) were proposed as possible degradation products of PAN as main component of UP2W (see Figure 1) within the Work Package 3 CORI of the EURAD EU project (CORI-SOTA, 2021), in the context of an on-going collaboration between Karlsruhe Institute for Technology—Institute for Nuclear Waste Disposal (KIT-INE) and the Swedish Nuclear Fuel and Waste Management Co. (SKB). The definition of these proxy ligands was based on the characterization of the supernatant solution in degradation experiments under hyperalkaline conditions by means of 13C-NMR (Tasi et al., 2021b). In view of the original PAN structure, GTA can be considered as representative of the bulk chain of the PAN polymer fragments, whereas HIBA and HBA account for the effect of the end-groups resulting in the degradation process. This considers the conversion of nitrile groups to carboxylate groups, as suggested in the literature for the alkaline hydrolysis of PAN (Ermakov et al., 2000; Litmanovich and Plate, 2000).
The impact of the UP2W degradation products on the solubility and sorption of radionuclides in cementitious systems has been previously investigated in a number of experimental studies (Dario et al., 2004; Holgersson et al., 2011; Duro et al., 2012; Szabo et al., 2022). However, no information is available so far on the interaction of these degradation products with hardened cement paste (HCP), and their possible impact on the surface properties of the latter material. In this context, this study aims at investigating the uptake of HIBA, HBA and GTA (as proxy ligands for the degradation products of UP2W) by HCP. Sorption experiments are conducted using a combination of inactive (HIBA, HBA, GTA) and radioactive (14C-labelled GTA) organic ligands. Measurements of the electrophoretic mobility are performed to gain insight on the evolution of the surface charge of HCP with increasing ligand concentration. The results obtained in this work for the uptake of HIBA, HBA and GTA are compared with the scarce data available in the literature for other organic ligands containing carboxylic acid and alcoholic hydroxyl functional groups to assess general trends in the uptake process and highlight the main parameters affecting sorption.
2 Experimental
2.1 Chemicals
All experiments were conducted in gloveboxes at T = (22 ± 2)°C under Ar gas atmosphere and O2 < 2 ppm. Purified water (Millipore Milli-Q Advantage A10 (18.2 MΩ·cm at 22°C, 4 ppb TOC) with Millipore Millipak® 40 0.22 µm) was used for sample preparation after purging with Ar for > 1 h to remove traces of O2 and CO2. Glutaric acid (C5H8O4, 99%), α-hydroxyisobutyric acid (C4H8O3, 99%) and 3-hydroxybutyric acid (C4H8O3, 99%) were purchased from Sigma-Aldrich. NaOH Titrisol was obtained from Merck. 14C-labelled glutaric acid [1,5-14C- GTA](s) was obtained from American Radiolabeled Chemical. The specific activity of the tracer was 50 mCi/mmol–60 mCi/mmol, with a total activity of 50 μCi = 1.85 MBq.
2.2 Cement and cement porewater
The hardened cement paste used in this work was provided by SKB and was prepared with a mixture of Swedish structural Portland cement for civil engineering (CEM I 42, 5 N BV/SR/LA type) and deionized water at a water-to-cement weight ratio of 0.5. The HCP monolith was milled, sieved to a particle size of < 100 μm as described by Tasi and co-workers and stored under Ar atmosphere until use in the sorption experiments Tasi et al. (2021a). An extensive characterization of the HCP material by X-ray diffraction (XRD), differential thermal analysis (DTA)/thermogravimetric analysis (TG), X-ray photoelectron spectroscopy (XPS) and Brunauer-Emmett-Teller (BET) measurements is provided in Tasi et al. (2021a).
All sorption experiments were performed using a cement porewater representative of the degradation stage II, with pH ≈ 12.5, [Ca] ≈ 0.02 M and low content of Na and K (≤ 3⋅10−4 M). The cement porewater was prepared using the protocol described in Tasi et al. (2021a). The HCP was weighted in a 2 L Kautex flask and contacted with Milli-Q water (previously boiled and purged with Ar) to reach a solid-to-liquid ratio (S:L, with S corresponding to the mass of HCP) of 25 g·dm−3. After 7 days of contact time, the initial leachate was separated by decantation from the HCP, and the solid material was equilibrated again during 7 days with fresh Milli-Q water in order to obtain the final porewater solution representative of degradation stage II.
2.3 pH measurements
The total free proton concentration (pHc = −log [H+], with [H+] in molar (M) units) was determined using combination pH–electrodes (type Orion Ross, Thermo Scientific) freshly calibrated against standard pH buffers (pH = 9–13, Merck). In aqueous solutions of ionic strength I ≥ 0.1 M, the measured pH value (pHexp) is an operational value related to [H+] by pHc = pHexp + Ac, where Ac is an empirical parameter entailing the activity coefficient of H+ and the liquid junction potential of the combination electrode for a given background electrolyte, ionic strength and temperature. Values of Ac reported in (Altmaier et al., 2003) for NaCl systems were taken for the correction of pHexp in this work.
2.4 Sorption experiments with inactive and 14C-labelled ligands
All batch sorption samples were prepared using the HCP and cement porewater described in Section 2.2. Experiments were conducted using both inactive (HBA, HIBA, GTA) and 14C-labelled ligands (14C-GTA). Stock solutions of the inactive ligands were titrated with 1.0 M NaOH to pH ≈ 12.5 in order to avoid any impact on the pH of the cement porewater. Sorption experiments with inactive ligands were conducted at (i) constant S:L = 4 g·dm−3 and 10−6 M ≤ [L]tot ≤ 0.1 M, and (ii) constant [L]tot = 10−3 M and 0.2 g·dm−3 ≤ S:L ≤ 50 g·dm−3 (45 samples, 10 ml per sample). Inactive samples were equilibrated for up to 360 days. At selected contact times, an aliquot of the supernatant solution of each sample was centrifuged at 5,806 g for 10 min. Subsequently, a known volume of the resulting solution was diluted in 2% HNO3 solutions and the non-purgeable organic carbon content (NPOC) quantified using an Analytik Jena multi n/c 2100 S equipment. The pH was systematically monitored after each measurement. Note that the use of any organic-based filtration device (e.g. 10 kD filters) was disregarded to avoid enhanced NPOC values as a result of filter leaching.
Samples with 14C-GTA were prepared at (i) constant [14C-GTA]tot = 10−7 M (0.462 kBq⋅ml−1) and 0.2 g·dm−3 ≤ S:L ≤ 50 g·dm−3, as well as (ii) constant S:L = 20 g·dm−3, [14C-GTA]tot = 10−7 M (0.462 kBq⋅ml−1) and varying the concentration of inactive ligand 10−5 M ≤ [GTA]tot ≤ 0.1 M (20 samples, 10 ml per sample). Samples containing 14C-labelled GTA were equilibrated for up to 82 days. An aliquot of each sample was centrifuged at 1,210 g for 5 min, and the supernatant filtered with 10 kD filters (pore diameter size ≈ 2–3 nm, Nanosep®, Pall Life Sciences) by centrifugation at 1,210 g for 12 min. An aliquot of the filtered solution was diluted in 2% HNO3, and the mixture was spiked to 10 ml of liquid scintillation counting (LSC) cocktail (Ultima Gold XR, Perkin Elmer). Beta counting was carried out using a Tri-Carb 3110 TR (PerkinElmer) equipment, considering an energy window between 0 keV and 100 keV and a measuring time of 30 min per sample. Measurements considering an energy window between 0 keV and 2,000 keV resulted in analogous values. A count rate of ∼20 cpm was usually determined for the blanks.
After each sampling in both inactive and active systems, the total sample volume was restored with the corresponding volume of cement porewater containing the same initial ligand concentration. Table 1 summarizes the experimental conditions of all sorption systems investigated in this work.
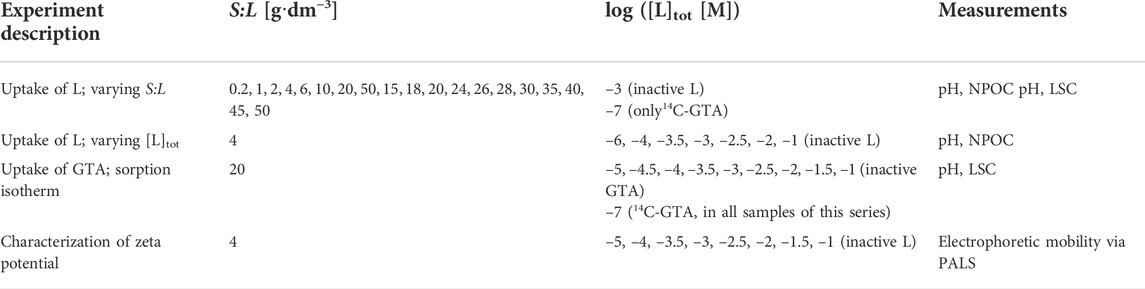
TABLE 1. Summary of the HCP-L systems and experimental conditions investigated in this work, with L = HIBA, HBA and GTA.
Sorption was evaluated following the evolution of the ligand concentration as a function of S:L, or as a sorption isotherm, i.e. log [L]solid vs. log [L]aq, where [L]aq was determined by either NPOC or LSC measurements. Distribution ratios, Rd (in m3∙kg−1), were calculated as the ratio of organic ligand concentration in the solid ([L]solid, in mol∙kg−1) and aqueous ([L]aq, in M) phases:
where [L]0 is the initial organic ligand concentration in the aqueous phase, V is the volume of sample (m3) and m is the mass of the solid used as sorbent (kg).
2.5 Measurements of the electrophoretic mobility
The impact of HIBA, HBA and GTA on the electrophoretic mobility of the suspended HCP colloidal particles was investigated in a series of independent batch experiments (Vtot = 10 cm3, 27 batches in total) with S:L = 4 g⋅dm−3 and 10−5 M ≤ [L]tot ≤ 0.1 M. After 14 days of contact time, aliquots of the HCP suspensions containing different ligand concentrations were transferred into plastic cuvettes and sealed under Ar atmosphere with parafilm. Measurements of the electrophoretic mobility were performed with a Nanobrook 90 plus PALS (Brookhaven Instruments Co.) equipment. Electrophoretic mobility was converted into zeta potential (ζ) using the Smoluchowsky equation, which is built-in the software of the equipment. Ten values of the ζ potential were acquired and averaged for each data point, whereas 15 points were collected for each sample in the course of one measurement. Electrophoretic mobility was measured in freshly agitated samples, as well as after 15 min to account for settling effects. The uncertainty of each measurement (calculated as 2 times the standard deviation of the 150 acquisitions) usually ranged between ± 1 mV and ± 3 mV, with the greatest uncertainty calculated as ± 4 mV for the HIBA system at [HIBA]tot ≈ 3⋅10−4 M. Experimental conditions considered in the measurements of the electrophoretic mobility are summarized in Table 1.
3 Results and discussion
3.1 Uptake of HIBA and HBA by HCP
Sorption experiments conducted with HIBA and HBA at S:L = 4 g⋅dm−3 and 10−6 M ≤ [L]0 ≤ 0.1 M show a negligible uptake of these proxy ligands by the HCP investigated in this work (see Figure 2). Figure 3 shows that in the experiments at [L]0 ≈ 10−3 M and 0.2 g·L−1 ≤ S:L ≤ 50 g·L−1, a decrease in the initial concentration of proxy ligand occurs only at the highest S:L, i.e. 50 g·L−1. The latter observations are consistent with the results obtained at S:L = 4 g⋅dm−3 and varying ligand concentration, and highlight the weak sorption of HIBA and HBA onto the surface of the HCP investigated in this work.
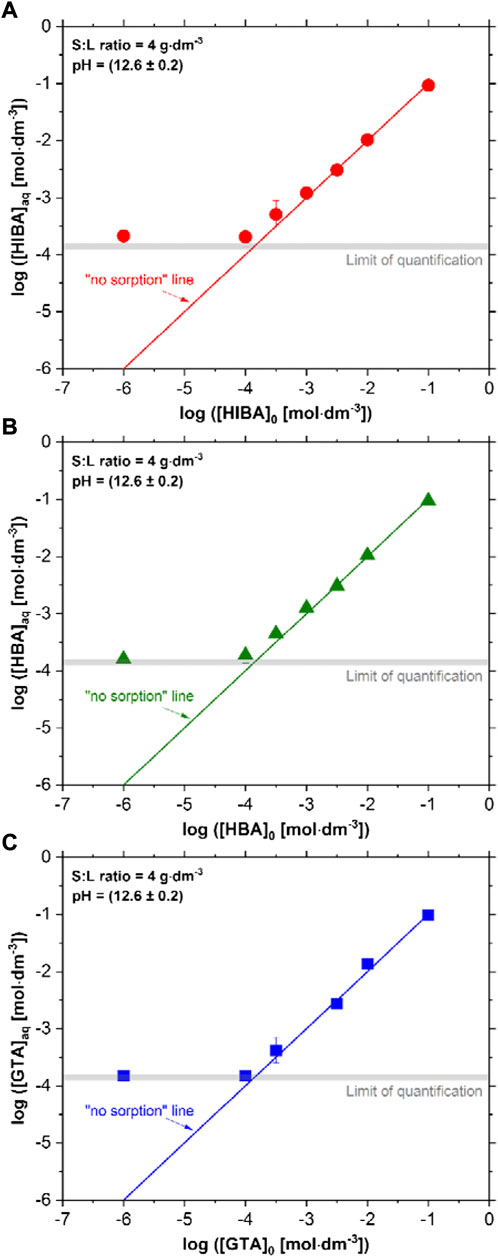
FIGURE 2. Uptake of (A) HIBA, (B) HBA and (C) GTA by HCP in the degradation stage II. Data represented as log [L]aq vs. log [L]0. All experiments performed at S:L = 4 g⋅dm−3. Shadowed grey areas correspond to the limit of quantification, which is imposed by the organic content leached from cement.
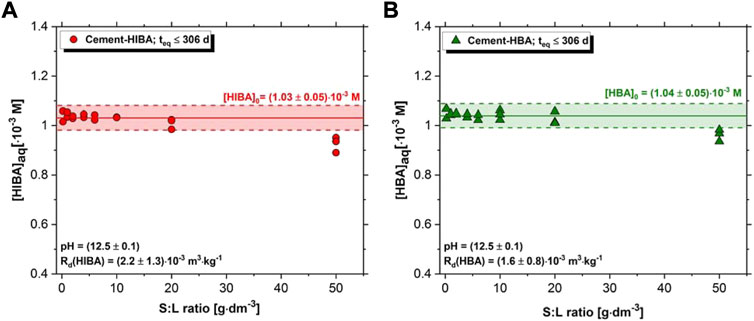
FIGURE 3. Uptake of (A) HIBA and (B) HBA by HCP in the degradation stage II. Data represented as [L]aq vs. S:L ratio. Shadowed red and green areas correspond to the experimentally measured initial concentrations of HIBA and HBA, respectively. The uncertainty of individual measurements is within the size of the symbols.
Rd values for the uptake of HIBA and HBA by HCP can be quantified on the basis of sorption data obtained at S:L = 50 g⋅dm−3, which result in Rd (HIBA) = (2.2 ± 1.3)⋅10−3 m3⋅kg−1 and Rd (HBA) = (1.6 ± 0.8)⋅10−3 m3⋅kg−1, with uncertainties being calculated as two times the standard deviation of the average value. These Rd values are higher than those reported by Wieland and co-workers for the uptake of formate and acetate by HCP, i.e. Rd (formate) ≈ 1⋅10−3 m3⋅kg−1 and Rd (acetate) ≈ 2–5⋅10−4 m3⋅kg−1 (Wieland et al., 2016). The latter authors conducted their sorption experiments at S:L = 333 g⋅dm−3 and pH = 13.3 (representative of the degradation stage I). We note that the less positive surface charge expected at pH ≈ 13.3 (Wieland et al., 2016) as compared to pH ≈ 12.5 [this work, see Section 3.3) can possibly explain the lower uptake reported for formate and acetate. An extended discussion exploring possible correlations between sorption phenomena and properties of the organic ligands (i.e. type and number of functional groups, acid-base properties (as log K°a) and strength of the complexation with Ca (in terms of log β°Ca-L)] is provided in Section 3.4.
3.2 Uptake of GTA by HCP
As in the case of HIBA and HBA, no significant sorption of GTA onto HCP is observed at S:L = 4 g⋅dm−3 and 10−6 M ≤ [GTA]0 ≤ 0.1 M (see Figure 3C). On the other hand, a substantial decrease of the initial GTA concentration is observed in the experiments performed at constant [GTA]0 and increasing S:L. For this system, consistent trends are obtained at [GTA]0 ≈ 10−3 M (as quantified by NPOC) and [14C-GTA]0 ≈ 10−7 M (as quantified by LSC) (see Figures 4A,B). Using experimental data at S:L ≥ 15 g⋅dm−3, both datasets result in similar distribution coefficients for the uptake of GTA, i.e. Rd (GTA) = (1.0 ± 0.5)⋅10−2 m3⋅kg−1 and Rd (14C-GTA) = (1.3 ± 0.5)⋅10−2 m3⋅kg−1 (with uncertainties calculated as two times the standard deviation of the average value). These Rd values are 4–8 times higher than those quantified for HIBA and HBA (see Section 3.1). The stronger uptake of GTA by HCP could potentially reflect either the higher stability of the Ca-GTA complex on the Ca surface sites of HCP or the formation of polynuclear complexes where GTA coordinates to two Ca atoms. An extended discussion on the influence of the different functional groups on the uptake of organic ligands by HCP is provided in Section 3.4.
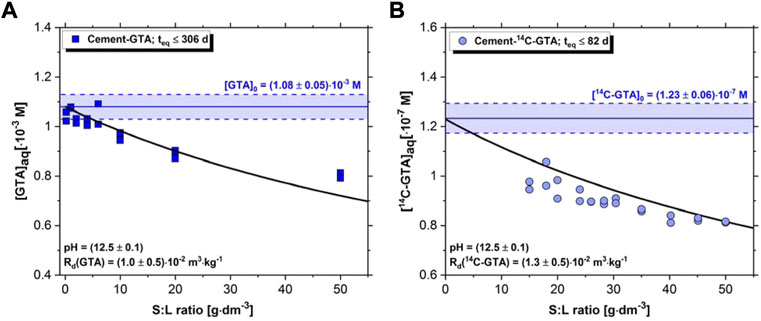
FIGURE 4. Uptake of (A) GTA and (B) 14C-GTA by HCP in the degradation stage II. Data represented as [GTA]aq or [14C-GTA]aq vs. S:L ratio. Shadowed blue region corresponds to the experimentally measured initial concentrations of GTA and 14C-GTA. Solid black lines correspond to the GTA concentration calculated using the one-site Langmuir isotherm derived in this work. The uncertainty of individual measurements is within the size of the symbols.
Figure 5 shows the results of the sorption experiments using inactive and radiolabelled GTA at S:L = 20 g⋅dm−3 and 10−7 M ≤ [GTA]0 ≤ 0.1 M, together with the sorption isotherm reported in the literature for the uptake of ISA by HCP (Van Loon et al., 1997). A combined fit of all available data points (see Figures 4A,B, 5) indicated that a one-site Langmuir isotherm is sufficient to describe the sorption process:
where q is the adsorption capacity of cement for GTA (mol⋅kg−1), K is the adsorption affinity constant (dm3⋅mol−1) and [GTA]aq is the equilibrium concentration of GTA (mol⋅dm−3), with:
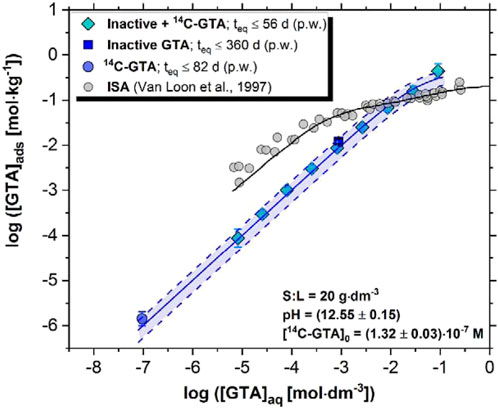
FIGURE 5. Sorption isotherm for the uptake of GTA by HCP in the degradation stage II. Experiments conducted with (i) inactive GTA at [GTA]0 = 10−3 M (), (ii) 14C-labelled GTA at [14C-GTA]0 = 10−7 M (
), and (iii) a combination of inactive and 14C-labelled GTA with 10−7 M ≤ [GTA]0 ≤ 0.1 M (with [GTA]0 = [inactiveGTA]0 + [14C-GTA]0) and [14C-GTA]0 = constant = 10−7 M (
). Sorption isotherm reported by Van Loon and co-workers for the uptake of ISA by HCP in the degradation stage I is included for comparison (
) (Van Loon et al., 1997). p.w. stands for present work.
The adsorption capacity q determined for the uptake of GTA by HCP is slightly higher but in line with the capacity previously reported for isosaccharinic acid (ISA) considering two sorption sites, i.e. qtot (ISA) = q1 + q2 = (0.35 ± 0.02)⋅mol⋅kg−1 (Tasi et al., 2021a) (for HCP in the degradation stage II) and qtot (ISA) = (0.27 ± 0.02)⋅mol⋅kg−1 (Van Loon et al., 1997) (for HCP in the degradation stage I). A higher adsorption capacity was reported by Glaus and co-workers for the uptake of gluconate by HCP (qtot (GLU) = (0.7 ± 0.3)⋅mol⋅kg−1) (Glaus et al., 2006), although the value reported by the latter authors overlaps with q (GTA) determined in this work when considering the corresponding uncertainties.
A relatively small adsorption affinity constant is determined in this work for the uptake of GTA, i.e. K (GTA) = (22.5 ± 6.1)⋅dm3⋅mol−1. This constant is 2–5 orders of magnitude lower than the values reported for the stronger binding site in HCP for the uptake of ISA and GLU, i.e., K1 (ISA) = (1730 ± 385)⋅dm3⋅mol−1 (Van Loon et al., 1997) (for HCP in the degradation stage I, pH = 13.3), K1(ISA) = (2,510 ± 500)⋅dm3⋅mol−1 (Tasi et al., 2021a) (for HCP in the degradation stage II, pH = 12.5), K1(ISA) = (714 ± 266)⋅dm3⋅mol−1 (Jo et al., 2022) (for HCP in the degradation stage I, pH = 13.5) or K1 (GLU) = (2 ± 1)⋅106⋅dm3⋅mol−1 (for HCP in the degradation stage I, pH = 13.3) (Glaus et al., 2006). However, the value of K (GTA) determined in this work is of the same order as the adsorption affinity constant reported for ISA in the weaker binding site in HCP, i.e., K2 (ISA) = (12 ± 4)⋅dm3⋅mol−1 (Van Loon et al., 1997) and K2(ISA) = (12 ± 2)⋅dm3⋅mol−1 (Tasi et al., 2021a). This comparison highlights again the weaker affinity of HCP towards GTA as compared to ISA and GLU.
3.3 Electrophoretic mobility of HCP in the presence of proxy ligands
Zeta potentials of suspended HCP particles (ζ, mV) measured through electrophoretic mobility analysis as a function of total concentration of proxy ligand are shown in Figure 6. For comparison purposes, Figure 6 shows also the values of zeta potential reported by Tasi and co-workers under analogous experimental conditions but in the presence of ISA (black squares in the figure) (Tasi et al., 2021a). The grey region in the figure indicates the range of zeta potentials representative of HCP (CEM I) in the degradation stage II and absence of organic ligands (Pointeau et al., 2006b; Tasi et al., 2021a).
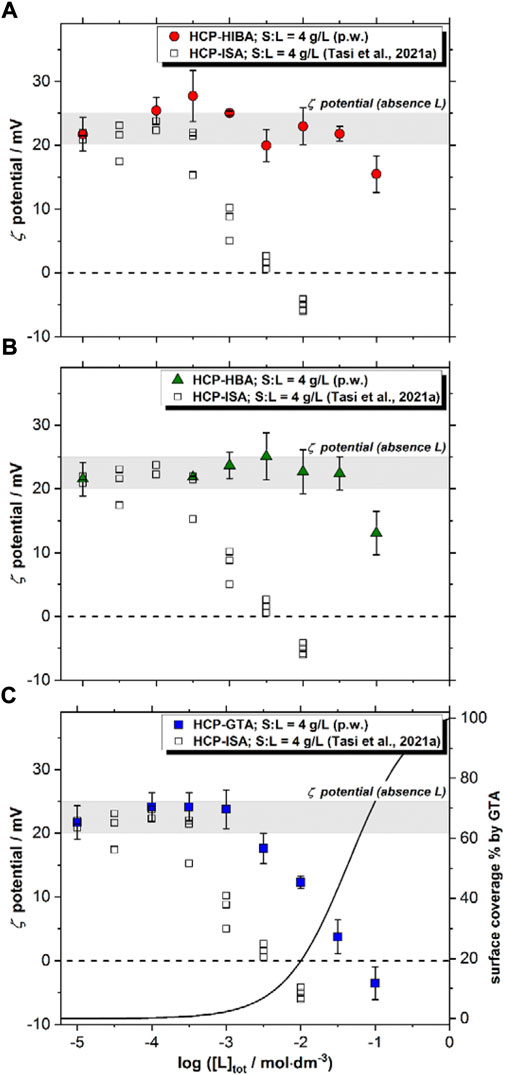
FIGURE 6. Zeta potentials of suspended HCP particles in the presence of proxy ligands at 10−5 M ≤ [L]tot ≤ 0.1: (A) HIBA, (B) HBA and (C) GTA. Zeta potentials reported in the literature for the HCP-ISA system are included for comparison (Tasi et al., 2021a). Grey zone in the figures correspond to the zeta potentials representative of HCP (CEM I) in the degradation stage II and absence of organic ligands (see text). Solid line in figure c corresponds to the surface coverage of HCP and is calculated with the one-term Langmuir isotherm derived in Section 3.2.
Figures 5A,B show that HIBA and HBA have a minor impact on the surface charge of HCP, and a slight decrease in the zeta potential values to ζ ≈ 15 mV is only observed at [L]tot ≈ 0.1 M. These results are consistent with the weak sorption quantified for both proxy ligands (see Section 3.2). In contrast to HIBA and HBA, a significant decrease in the zeta potentials is observed for GTA at ligand concentrations above ∼10−3 M with the isoelectric point being achieved at [GTA]tot ≈ 6⋅10−2 M. The evolution of the surface charge in the HCP-GTA system correlates well with the surface coverage of HCP by GTA (see solid line in Figure 4C), as calculated with the one-site Langmuir isotherm derived in Section 3.2.
These results can be explained considering the stronger uptake of GTA as compared to HIBA and HBA, but also accounting for the net charge of the ligand in the investigated hyperalkaline conditions, i.e. −2 for GTA and −1 for HIBA and HBA. Figure 6C shows also that ISA promotes an earlier decrease in the zeta potentials, resulting in an isoelectric point at [ISA]tot ≈ 3⋅10−3 M (Tasi et al., 2021a). The stronger impact of ISA on the surface charge of HCP is in line with the significantly higher affinity of this ligand towards the surface of cement, as compared to the proxy ligands for the degradation of UP2W investigated in this work.
3.4 Comparison with sorption data available in the literature
As recently discussed in the State of the Art Report on cement-organic-radionuclide interactions prepared in the context of the Work Package 3 CORI of the EURAD EU project (CORI-SOTA, 2021), little information is available in the literature on the interaction of organic ligands with HCP. Table 2 summarizes the Rd values determined in this work for the uptake of HIBA, HBA and GTA by HCP, together with sorption data previously reported for other organic compounds containing the functional groups –COOH and –OH, i.e. EDTA, ISA, GLU, formic acid, acetic acid, methanol and ethanol. Table 2 provides also the most relevant experimental conditions of each study, as well as the type and number of functional groups in each compound. The few studies reporting on the interaction with HCP of organic compounds containing other functionalities (e.g. sulfonate, –SO3M, see Glaus et al., 2006) have been disregarded in this exercise.
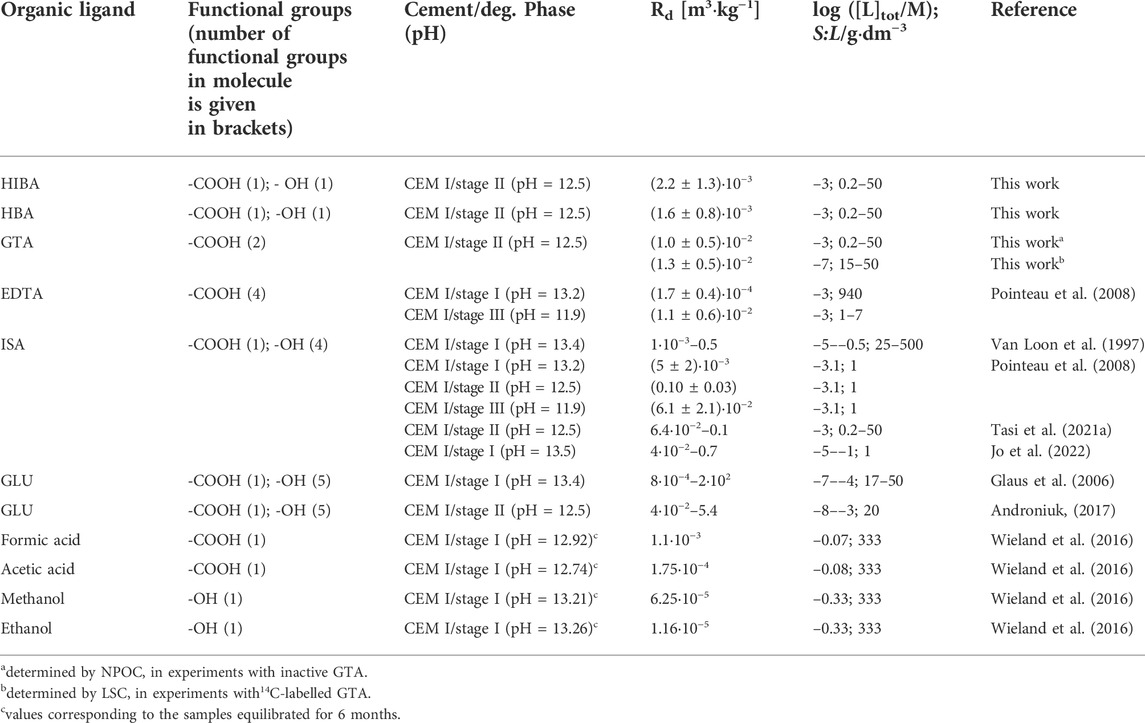
TABLE 2. Summary of Rd values determined in this work and reported in the literature as well as related experimental conditions for the uptake of different organic ligands with –COOH and –OH functionalities by HCP (CEM I) in the degradation stages I–III.
The comparison of all Rd values is less straightforward because sorption is affected by multiple variables, i.e. pH/cement degradation stage, [Ca], [L], S:L ratio or the specific surface area of the HCP material used. Moreover, a non-linear sorption involving strong/weak sorption sites was confirmed for both ISA and GLU (Van Loon et al., 1997; Glaus et al., 2006; Tasi et al., 2021a) (see for instance the sorption isotherm of ISA in Figure 5), which results in significantly higher Rd values at low [L] compared to high [L]. Evidence on linear sorption was obtained in this work for GTA over almost 6 orders of magnitude in terms of ligand concentration, whereas for the rest of the ligands experimental data are available only at one ligand concentration and thus insights on the linearity/non-linearity of the sorption remain unknown.
Table 2 allows a qualitative assessment of the strength of the cement–organic interaction as a function of the type and number of functional groups in the organic ligand. Accordingly, the different ligands and functionalities can be listed in the following general order (number of functional groups in molecule is given in brackets):
(weaker uptake) // -OH (1) < -COOH (1) < [-COOH (1) + -OH (1)] < -COOH (2) ≈ -COOH (4) << [-COOH (1) + -OH (4)] < [-COOH (1) + -OH (5)] // (stronger uptake)
Differences of up to 7 orders of magnitude are found between Rd values reported for the weakest and strongest uptake under similar experimental conditions, i.e. Rd (ethanol) = 1.16⋅10−5 m3⋅kg−1 (Wieland et al., 2016) and Rd (GLU) = 2⋅102 m3⋅kg−1 (Glaus et al., 2006), thus highlighting the key role of the functional groups in the strength of the uptake process of organic substances. Although the presence of alcohol groups alone results in a very weak uptake (methanol, ethanol), these functional groups play a key role in the sorption when several of them co-exist with a carboxylate group, i.e. in GLU or ISA, specifically in the carboxylate’s alpha position. In contrast to the latter ligands, the proxy ligands investigated in this work containing one carboxylate and one alcohol group (HIBA and HBA) show Rd values only slightly higher than those reported for monocarboxylates (formate, acetate), and clearly lower than a dicarboxylate ligand like GTA. In addition to the number of functional groups in the molecules, the denticity of the organic ligands (i.e. the number of atoms with which a ligand binds to a metal ion) is expected to have significant implications for the thermodynamic stability of surface complexes forming. Although ethanol and methanol are clearly monodentate, the denticity of the rest of the ligands in Table 2 is less evident. Moreover, denticity is expectedly affected by pH for those ligands containing alcohol groups susceptible to be deprotonated.
Wieland and co-workers proposed that Rd values for the uptake of low molecular weight organic compounds by HCP could tentatively be correlated with the acid-base properties of the organic molecule (Wieland et al., 2016). Moreover, a number of modelling and molecular dynamics studies provided evidence on the participation of Ca atoms in bridging organic ligands with the surface of HCP/C-S-H phases (Pointeau et al., 2008; Androniuk and Kalinichev, 2020; Jo et al., 2022), which may suggest that the strength of the sorption could be correlated with the stability of the Ca-L complexes forming in the aqueous phase. To explore both hypotheses, Table 3 summarizes the acid base and complexation constants with Ca for all the ligands considered in Table 2.
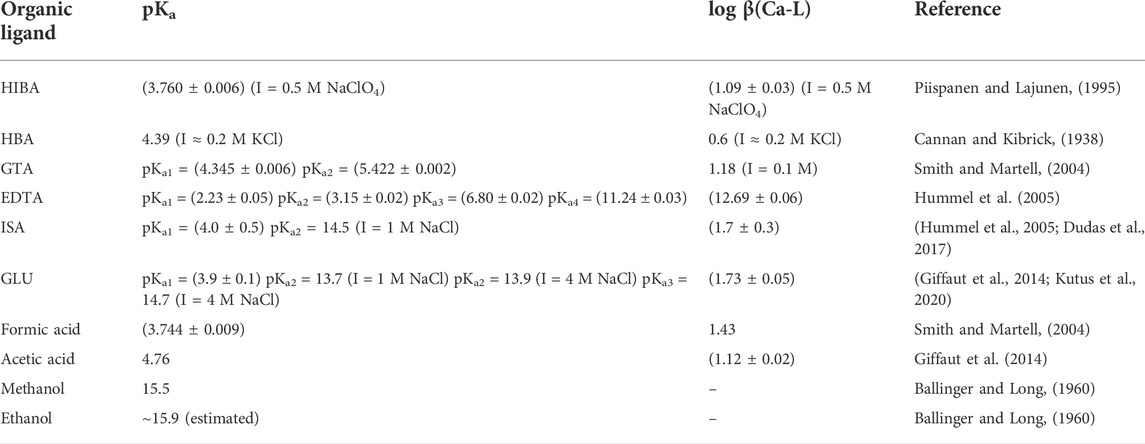
TABLE 3. Summary of pKa and log β(Ca-L) values reported in the literature for HIBA, HBA, GTA, EDTA, ISA, GLU, formic acid, acetic acid, methanol and ethanol. Equilibrium constants for the formation of Ca-L complexes corresponding to the chemical reaction Ca2+ + Lx– ⇌ CaL(2–x). All constants reported at I = 0 except otherwise indicated.
The correlation of Rd values summarized in Table 2 with the pKa’s reported in Table 3 for the same organic ligands shows a good qualitative inverse correlation for the four ligands containing only one functional group. The proxy ligands HIBA and HBA (both with 1 -COOH and 1 -OH groups) show higher Rd values than formic and acetic acids, in spite of the similar pKa of their carboxylate groups. Although the participation of the alcohol group in the stabilization of surface complexes with HCP might be envisaged for HIBA and HBA, differences in the Rd values reported in Table 2 can be possibly explained by the positive surface charge of HCP in degradation stage II (experiments with HIBA and HBA; this work), as opposed to the negatively charged surface expected in the degradation stage I (experiments with formic and acetic acid; Wieland et al., 2016).
Tables 2, 3 clearly show that the equilibrium constants for the formation of the binary complexes Ca-L are not a sufficient (sole) indicator of the tendency of a given ligand L to sorb onto the HCP surface. Hence, GTA (2 -COOH groups, log βCa-L = 1.18) and EDTA (4 -COOH groups and two amine functionalities, log βCa-L = 12.69) show similar Rd values, which suggests that only two carboxylate groups participate in the sorption of EDTA onto the HCP and thus that steric constraints need to be taken into account when assessing the interaction of organic ligands with the cement surface. ISA and GLU are characterized by the strongest interaction with HCP. Although they form weak binary complexes with Ca of the type CaL+ in acidic to weakly alkaline conditions, several studies available in the literature provide evidence on the formation of stable complexes of ISA/GLU with Ca in hyperalkaline pH conditions, which involve the deprotonation (besides the carboxylic group) of at least one of the alcohol groups in those ligands [e.g. CaLH–1 (aq) or Ca3L2H–4 (aq)] (Hummel et al., 2005; Pallagi et al., 2014; Dudas et al., 2017; Kutus et al., 2020). A similar effect was suggested by Jo and co-workers for C-S-H-ISA surface complexes based on molecular dynamics calculations (Jo et al., 2022). We note that the presence of only one alcohol group in HIBA and HBA is insufficient to promote the enhancement of the Rd values as observed in the case of ISA and GLU. No thermodynamic studies are available in the literature dealing with the complexation of HIBA and HBA with Ca in hyperalkaline pH conditions. However, our previous undersaturation solubility experiments with Ca(OH)2 (cr) in the presence of HIBA and HBA at 10−6 M ≤ [L]tot ≤ 0.1 M confirmed that CaL complexes involving the deprotonation of the alcohol group are either very weak or not forming at all (Szabo et al., 2022).
A number of experimental and theoretical studies have provided evidence on the participation of C-S-H phases in the retention of low molecular weight organic ligands (Pointeau et al., 2006a; Pointeau et al., 2008; Androniuk, 2017; Garcia et al., 2020; Jo et al., 2022). The possible participation of C-A-S-H phases in the uptake mechanism was also proposed based on the different sorption sites observed for strongly sorbing ligands like ISA (Van Loon and Glaus, 1998; Tasi et al., 2021a). In their recent study, Wieland and co-workers confirmed the uptake of formate and acetate by AFm and AFt phases, although with Rd values significantly lower than those reported for the C-S-H counterparts (Wieland et al., 2016). These authors proposed the exchange of SO42–/HCOO− in AFt-SO4 or CO32–/HCOO− in AFm-CO3 as plausible uptake mechanisms. Note that Guidone and co-workers reported also the uptake of larger ligands (citrate, gluconate) by AFt and AFm phases (Guidone et al., 2022). The linear trend observed in our study for the uptake of GTA (see Figure 5) strongly supports the participation of only one sorption site in the retention of this organic ligand. These observations underline the likelihood that different sorption sites and possibly cement phases are involved in the uptake of organic ligands with different functionalities, charge and/or size.
Although it is evident that additional experimental and theoretical efforts are required in this field, the comparison and discussion above provide the basis to qualitatively assess the sorption properties of other organic ligands or degradation products expected under repository conditions containing the functionalities –COOH and –OH. The key role of alpha-positioned alcohol groups in the interaction of organic ligands with the HCP surface is highlighted, under the remark that their contribution is mostly relevant when participating in their deprotonated state. Differences in the acidity of the α alcohol group of ISA and GLU can partially explain the differences in the sorption behaviour of both ligands. In the case of HIBA, the two -CH3 groups in α position possibly exert an electron-donating effect, which significantly increases the pKa value of the OH group and prevents deprotonation of the OH group even under strongly alkaline conditions. On-going studies in the context of the Work Package 3 CORI of the EURAD EU project are expected to fill-in some of the identified gaps.
4 Summary and conclusions
A detailed experimental sorption study was conducted aiming at the quantitative evaluation of the uptake of HIBA, HBA and GTA by HCP, and their impact on the surface properties of the investigated cement material. HIBA and HBA are monohydroxomonocarboxylate ligands, whereas GTA is characterized by two carboxylate groups. These low molecular weight organic ligands were proposed in the context of the Work Package 3 CORI of the EURAD EU project as possible degradation products of UP2W, a polyacrylonitrile-based filter aid. Experiments were conducted with a CEM I-based HCP in contact with a porewater solution representative of the degradation stage II of cement, i.e. with pH ≈ 12.5 M and [Ca] ≈ 0.02 M.
Sorption experiments confirm the weak uptake of HIBA and HBA, in line with previous studies investigating the sorption of other monocarboxylate ligands, e.g. formate and acetate. A stronger uptake was observed in the case of GTA, which is attributed to the presence of two carboxylate groups in the structure of the ligand. Studies combining inactive and 14C-labelled GTA confirmed the linear sorption over ca. 6 orders of magnitude in terms of [GTA]aq, which supports that primarily one sorption site/cement phase is responsible for the uptake of this ligand by HCP. This trend differs from the non-linear sorption behaviour reported for strongly sorbing ligands like ISA or gluconate, thus highlighting that different mechanisms (sorption sites, cement phases) likely participate in the uptake of ligands containing different type/number of functional groups.
Measurements of the electrophoretic mobility show that HIBA and HBA expectedly have only a minor impact on the surface charge of HCP. On the contrary, a clear decrease of the surface charge is observed for GTA at ligand concentrations above ∼10−3 M, which involves the inversion of the surface charge (i.e. isoelectric point) at [GTA] ≈ 6⋅10−2 M.
The comparison of Rd values determined in this work and reported in the literature for several organic ligands containing the functional groups -COOH and -OH provides qualitative insights on the main parameters generally governing the uptake of these organic ligands by HCP. Sorption is strongly affected by the degradation stage of cement, which defines the pH, [Ca] and surface charge of the system. The comparison of sorption data available for the same degradation stage supports the key role of multiple functionalities as a factor strengthening the interaction with the HCP surface. The presence of alcohol groups can importantly enhance sorption when available in their deprotonated state. This is triggered by the direct vicinity of other functional groups (-COOH and/or -OH), which induces a decrease of the pKa of the alcohol group and hence facilitates deprotonation.
These results provide relevant information to quantitatively assess the uptake of organic ligands by HCP relevant in the context of nuclear waste disposal, and further to understand their impact on the surface properties of these materials.
Data availability statement
The original contributions presented in the study are included in the article/Supplementary Material, further inquiries can be directed to the corresponding authors.
Author contributions
SH, AM, XG, MA, and HG defined the initial concept of the project. AT and XG contributed to conception and design of the experimental study. PS and AT performed the experiments. AT and PS conducted data evaluation. XG wrote the first draft of the manuscript. PS and AT wrote sections of the manuscript. All authors contributed to manuscript revision, read, and approved the submitted version.
Acknowledgments
The EURAD project leading to this application has received funding from the European Union’s Horizon 2020 research and innovation programe under grant agreement No 847593. The work was performed as part of the WP CORI and was partially funded by SKB. Jonas Rentmeister, Darlyn Rehhorn and Melanie Böttle (all KIT–INE) are gratefully acknowledged for the NPOC measurements and technical support. The manuscript was significantly improved by comments provided by two anonymous reviewers and Axel Liebscher. We acknowledge support by the KIT-Publication Fund of the Karlsruhe Institute of Technology.
Conflict of interest
The authors declare that the research was conducted in the absence of any commercial or financial relationships that could be construed as a potential conflict of interest.
Publisher’s note
All claims expressed in this article are solely those of the authors and do not necessarily represent those of their affiliated organizations, or those of the publisher, the editors and the reviewers. Any product that may be evaluated in this article, or claim that may be made by its manufacturer, is not guaranteed or endorsed by the publisher.
Supplementary material
The Supplementary Material for this article can be found online at: https://www.frontiersin.org/articles/10.3389/fnuen.2022.997398/full#supplementary-material.
References
Altmaier, M., Metz, V., Neck, V., Müller, R., and Fanghänel, T. (2003). Solid-liquid equilibria of Mg(OH)2(cr) and Mg2(OH)3Cl 4H2O(cr) in the system Mg-Na-H-OH-O-Cl-H2O at 25°C. Geochim. Cosmochim. Acta 67, 3595–3601. doi:10.1016/s0016-7037(03)00165-0
Androniuk, I. (2017). Effects of cement organic additives on the adsorption of uranyl ions on calcium silicate hydrate phases: Experimental determination and computational molecular modelling. PhD thesis. France: SUBATECH.
Androniuk, I., and Kalinichev, A. G. (2020). Molecular dynamics simulation of the interaction of uranium (VI) with the C-S-H phase of cement in the presence of gluconate. Appl. Geochem. 113, 104496. doi:10.1016/j.apgeochem.2019.104496
Ballinger, P., and Long, F. A. (1960). Acid ionization constants of alcohols. II. Acidities of some substituted methanols and related Compounds1, 2. J. Am. Chem. Soc. 82, 795–798. doi:10.1021/ja01489a008
Barzgar, S., Lothenbach, B., Tarik, M., Di Giacomo, A., and Ludwig, C. (2020). The effect of sodium hydroxide on Al uptake by calcium silicate hydrates (C-S-H). J. Colloid Interface Sci. 572, 246–256. doi:10.1016/j.jcis.2020.03.057
Cannan, R. K., and Kibrick, A. (1938). Complex formation between carboxylic acids and divalent metal cations. J. Am. Chem. Soc. 60, 2314–2320. doi:10.1021/ja01277a012
CORI-SOTA (2021). State of the art report on cement-organic-radionuclide interactions. Final version as of 19.05.2021 of deliverable D3.1 of the HORIZON 2020 project EURAD. EC Grant agreement no: 847593.
Dario, M., Molera, M., and Allard, B. (2004). Effect of organic ligands on the sorption of europium on TiO2 and cement at high pH. SKB TR-04-04. Stockholm: SKB.
Dudas, C., Kutus, B., Boszormenyi, E., Peintler, G., Kele, Z., Palinko, I., et al. (2017). Comparison of the Ca2+ complexing properties of isosaccharinate and gluconate - is gluconate a reliable structural and functional model of isosaccharinate? Dalton Trans. 46, 13888–13896. doi:10.1039/c7dt03120c
Duro, L., Grive, M., Gaona, X., Bruno, J., Andersson, T., Boren, H., et al. (2012). Study of the effect of the fibre mass UP2 degradation products on radionuclide mobilisation. SKB R-12-15. Stockholm: SKB.
Ermakov, I. V., Rebrov, A. I., Litmanovich, A. D., and Plate, N. A. (2000). Alkaline hydrolysis of polyacrylonitrile, 1 - structure of the reaction products. Macromol. Chem. Phys. 201, 1415–1418. doi:10.1002/1521-3935(20000801)201:13<1415::aid-macp1415>3.0.co;2-r
Garcia, D., Henocq, P., Riba, O., Lopez-Garcia, M., Made, B., and Robinet, J. C. (2020). Adsorption behaviour of isosaccharinic acid onto cementitious materials. Appl. Geochem. 118, 104625. doi:10.1016/j.apgeochem.2020.104625
Giffaut, E., Grive, M., Blanc, P., Vieillard, P., Colas, E., Gailhanou, H., et al. (2014). Andra thermodynamic database for performance assessment: ThermoChimie. Appl. Geochem. 49, 225–236. doi:10.1016/j.apgeochem.2014.05.007
Glaus, M. A., Laube, A., and Van Loon, L. R. (2006). Solid-liquid distribution of selected concrete admixtures in hardened cement pastes. Waste Manag. 26, 741–751. doi:10.1016/j.wasman.2006.01.019
Guidone, R. E., Lothenbach, B., Tasi, A., Gaona, X., Altmaier, M., and Geckeis, H. (2022). “Impact of formate, citrate and gluconate on the uptake of radionuclides by cement: Study of the binary and ternary systems cement-L and cement-RN-L,” in Proceedings NUWCEM Conference, Avignon, France, 2022, 4.
Holgersson, S., Dubois, I., and Börstell, L. (2011). Batch experiments of Cs, Co and Eu sorption onto cement with dissolved fibre mass UP2 in the liquid phase. SKB P-11-24. Stockholm: SKB.
Hummel, W., Anderegg, G., Rao, L., Puigdomènech, I., and Tochiyama, O. (2005). Chemical thermodynamics of compounds and complexes of U, Np, Pu, Am, Tc, Se, Ni and Zr with selected organic ligands, 9. North HollandAmsterdam: Elsevier.
Jo, Y., Androniuk, I., Cevirim-Papaioannou, N., de Blochouse, B., Altmaier, M., and Gaona, X. (2022). Uptake of chloride and iso-saccharinic acid by cement: Sorption and molecular dynamics studies on HCP (CEM I) and C-S-H phases. Cem. Concr. Res. 157, 106831. doi:10.1016/j.cemconres.2022.106831
Keith-Roach, M. J., Lindgren, M., and Källström, K. (2014). Assessment of complexing agent concentrations in SFR. SKB R-14-03. Stockholm: SKB.
Keith-Roach, M., Lindgren, M., and Källström, K. (2021). Assessment of complexing agent concentrations for the post-closure safety assessment in PSAR SFR. SKB R-20-04. Stockholm: SKB.
Kutus, B., Gaona, X., Pallagi, A., Palinko, I., Altmaier, M., and Sipos, P. (2020). Recent advances in the aqueous chemistry of the calcium(II)-gluconate system - equilibria, structure and composition of the complexes forming in neutral and in alkaline solutions. Coord. Chem. Rev. 417, 213337. doi:10.1016/j.ccr.2020.213337
Litmanovich, A. D., and Plate, N. A. (2000). Alkaline hydrolysis of polyacrylonitrile. On the reaction mechanism. Macromol. Chem. Phys. 201, 2176–2180. doi:10.1002/1521-3935(20001101)201:16<2176::aid-macp2176>3.0.co;2-5
Ochs, M., Mallants, D., and Wang, L. (2016). Radionuclide and metal sorption on cement and concrete, topics in safety, risk, reliability and quality. 1st ed. Cham: Springer International Publishing, 1.
Pallagi, A., Bajnoczi, E. G., Canton, S. E., Bolin, T., Peintler, G., Kutus, B., et al. (2014). Multinuclear complex formation between Ca(II) and gluconate ions in hyperalkaline solutions. Environ. Sci. Technol. 48, 6604–6611. doi:10.1021/es501067w
Piispanen, J., Lajunen, L. H. J., Mutikainen, I., Nasakkala, M., Robinson, W. T., Arnarp, J., et al. (1995). Complex formation equilibria of some aliphatic alpha-hydroxycarboxylic acids. 1. The determination of protonation constants and the study of calcium(II) and magnesium(II) complexes. Acta Chem. Scand. 49, 235–240. doi:10.3891/acta.chem.scand.49-0235
Pointeau, I., Coreau, N., and Reiller, P. (2008). Uptake of anionic radionuclides onto degraded cement pastes and competing effect of organic ligands. Radiochim. Acta 96, 367–374. doi:10.1524/ract.2008.1503
Pointeau, I., Hainos, D., Coreau, N., and Reiller, P. (2006a). Effect of organics on selenite uptake by cementitious materials. Waste Manag. 26, 733–740. doi:10.1016/j.wasman.2006.01.026
Pointeau, I., Reiller, P., Mace, N., Landesman, C., and Coreau, N. (2006b). Measurement and modeling of the surface potential evolution of hydrated cement pastes as a function of degradation. J. Colloid Interface Sci. 300, 33–44. doi:10.1016/j.jcis.2006.03.018
Smith, R. M., and Martell, A. E. (2004). NIST critically selected stability constants of metal complexes database. NIST standard reference database 46.
Szabo, P., Tasi, A., Gaona, A., Polly, R., Maier, A., Hedström, S., et al. (2022). Solubility of Ca(II), Ni(II), Nd(III) and Pu(IV) in the presence of proxy ligands for the degradation of polyacrylonitrile in cementitious systems. Dalton Trans. 51, 9432–9444. doi:10.1039/d2dt01409b
Tasi, A., Gaona, X., Rabung, T., Fellhauer, D., Rothe, J., Dardenne, K., et al. (2021a). Plutonium retention in the isosaccharinate - cement system. Appl. Geochem. 126, 104862. doi:10.1016/j.apgeochem.2020.104862
Tasi, A., Szabo, P., Gaona, X., Altmaier, M., and Geckeis, H. (2021b). “Contribution by KIT-INE to CORI Task 2 (pers. co.),” in Eurad European joint programme on radioactive waste management, milestone DMS97 – CORI technical Report -task 2 hydrolytic/radiolytic degradation of organics: Description of first results on hydrolytic and radiolytic organic degradation and identification of released species. Editors D. Ricard, and J. Vandenborre.
Van Loon, L. R., and Glaus, M. A. (1998). Experimental and theoretical studies on alkaline degradation of cellulose and its impact on the sorption of radionuclides. PSI Report 98-07. Villigen, Switzerland: PSI.
Van Loon, L. R., Glaus, M. A., Stallone, S., and Laube, A. (1997). Sorption of isosaccharinic acid, a cellulose degradation product on cement. Environ. Sci. Technol. 31, 1243–1245. doi:10.1021/es960505i
Wieland, E., Jakob, A., Tits, J., Lothenbach, B., and Kunz, D. (2016). Sorption and diffusion studies with low molecular weight organic compounds in cementitious systems. Appl. Geochem. 67, 101–117. doi:10.1016/j.apgeochem.2016.01.009
Keywords: sorption, UP2W, polyacrylonitrile, α-hydroxyisobutyric acid, 3-hydroxybutyric acid, glutaric acid, portland cement
Citation: Szabo PG, Tasi AG, Gaona X, Maier AC, Hedström S, Altmaier M and Geckeis H (2022) Uptake of selected organic ligands by hardened cement paste: Studies on proxy ligands for the degradation of polyacrylonitrile and general considerations on the role of different functionalities in the uptake process. Front. Nucl. Eng. 1:997398. doi: 10.3389/fnuen.2022.997398
Received: 18 July 2022; Accepted: 25 August 2022;
Published: 05 October 2022.
Edited by:
David García, Amphos 21, SpainReviewed by:
Markus Olin, VTT Technical Research Centre of Finland Ltd., FinlandAxel Liebscher, Federal Company for Radioactive Waste Disposal, Germany
Erich Wieland, Paul Scherrer Institut , Switzerland
Copyright © 2022 Szabo, Tasi, Gaona, Maier, Hedström, Altmaier and Geckeis. This is an open-access article distributed under the terms of the Creative Commons Attribution License (CC BY). The use, distribution or reproduction in other forums is permitted, provided the original author(s) and the copyright owner(s) are credited and that the original publication in this journal is cited, in accordance with accepted academic practice. No use, distribution or reproduction is permitted which does not comply with these terms.
*Correspondence: A. G. Tasi, agost.tasi@kit.edu; X. Gaona, xavier.gaona@kit.edu