- Department of Nuclear Chemistry, Faculty of Nuclear Sciences and Physical Engineering, Czech Technical University in Prague, Prague, Czechia Republic
Commercial cementitious material, hydrated cement pastes of type CEM I and CEM III, and a synthetic cementitious phase CSH (calcium-silicate-hydrates) with a Ca/Si ratio equal to 1.0, 1.2, and 1.4 were used in the study of lead sorption. Kinetic and equilibrium experiments were performed under different conditions, namely liquid to solid phase ratio and the presence of organic compounds (EDTA, phthalate). The duration of equilibrium experiments was determined based on kinetic experiments, 1 week for calcium-silicate-hydrates and 3 weeks for hydrated cement paste. Equilibrium experiments were evaluated using the distribution ratio Rd and sorption isotherms. For both hydrated cement pastes, Rd’s in the range 102–103 L kg−1 were found while for calcium-silicate-hydrates the distribution ratio values were an order of magnitude higher under comparable conditions. The difference in the studied cementitious materials was also manifested in the shape of the sorption isotherm, while the behavior of calcium-silicate-hydrates can be fitted linearly and therefore by the Kd-model, for hydrated cement paste as more complex cement material it is necessary to use a non-linear isotherm description. The effect of organic matter, represented here by EDTA and phthalate, described by the sorption reduction factor SRF was more apparent for calcium-silicate-hydrates, where its value was always higher than one and reached up to 500. On the contrary, for hydrated cement paste CEM I and CEM III, all determined SRFs were less than 1.
1 Introduction
Cement materials serve as an engineering barrier for the storage of low- and intermediate-level radioactive waste (L/ILW). In the Czech Republic, cementation is used for institutional L/ILW, while low- and intermediate-level waste generated during the operation of nuclear power plants is solidified mainly with bitumen and stored in the Dukovany surface repository. Cemented radioactive waste has been stored in near-surface repositories. The Richard near-surface repository near Litoměřice, intended for L/ILW waste, is in operation, its capacity was currently increased, but no further expansion is planned. After filling this repository, radioactive waste, both institutional and also waste generated during the operation and decommissioning of nuclear power plants, should be stored in the planned deep geological repository (DGR). The waste will probably be stored in barrels, which will be placed four at a time in concrete containers; the concrete would be used as a filling protective material in the space between the barrels (cement possibly also as a material for solidifying the waste). It is a similar concept to storing waste in the Richard repository, where cemented waste is deposited in one-hundred-liter drums (using the cement of CEM II type), which are then placed in external drums with a volume of 200 L. The space between these two barrels is filled with concrete (made of cement of CEM I type), which creates another protective layer. After filling, the entire chamber with barrels is poured with concrete containing cement of CEM III type. Moreover, in DGR cement used for construction purposes cannot be neglected either.
The study of the migration behavior of radionuclides stored in the cement matrix is therefore a significant contribution to the safety assessment of existing surface repositories and future deep repositories. Hydrolysis in the environment of cementitious materials takes place at a very high alkaline pH, the value of which is about 13.5 for fresh cement, around 12.5 for cement in the second stage of aging and decreases with time to values below pH ≈ 10 (Ochs et al., 2016).
A highly alkaline pH also significantly affects the behavior of radionuclides including lead, which isotopes occur in decay series. Particularly important is isotope 210Pb present in the uranium-radium series that, due to its half-life (22 years), forms a so-called long-term radioactive deposit. At high pH (very alkaline environment, pH ≥ 12.5) corresponding to the leachate from hydrated cement paste (HCP), the lead oxidation state II dominates in the solution, mainly in the form of hydroxides and oxides (Berner, 2003; Ochs et al., 2016). (Berner, 2003) also describes the possible solubility limits (maximum concentration) of lead in the DGR in the case that the concentration of HS− is neglected in the calculations (otherwise sulfide precipitation to PbS occurs). The Pb concentration at pH = 12.55 is determined by the solubility (2.8∙10–3 mol L−1) of solid Pb(OH)2, which undergoes hydrolysis leading to 98% Pb(OH)3- and 2% Pb(OH)2. At pH = 13.44, the solubility increases to 2.2·10–2 mol L−1, which can be considered as the upper limit of the concentration. The stated concentrations are in accordance with (Moulin et al., 1999) where in the alkaline environment of cement materials, lead maximum concentration determined by the precipitation of PbO equals 3∙10–3 mol L−1. There are therefore no such limitations regarding solubility of lead as with other studied radionuclides, e.g. U (Brownsword et al., 1989).
Besides pH and the related solubility limitation, the initial lead concentration is the other factor that greatly influences lead sorption in the DGR (Ochs et al., 2016). As the initial concentration of lead or pH increases, sorption on cementitious materials decreases. Another parameter is the surface size of the solid phase. The likely mechanism of lead uptake on cementitious materials is the isomorphic substitution of Pb2+ for Ca2+ (Evans, 2008) in which lead as a divalent cation is probably sorbed on cement materials [specifically on the CSH, which is the most important phase for the immobilization of a whole range of radionuclides that interact with cement materials through cation exchange (Nonat, 2004)] similarly to Ra, Sr and Ba to form the bond Si - O- Pb (Rose et al., 2000; Coleman et al., 2005).
(Ochs et al., 2016) summarized the values of the distribution ratio between the liquid and solid phase (Rd) for lead during individual stages of HCP aging in the range of pH = 10–13.5. From the point of view of the possible interaction of radionuclides with the cement material in the repository, the aging stage III that is attributed to the occurrence of CSH (pH of the system depends on the Ca/Si ratio, Ca/Si 0.8–1.5 corresponds to pH = 10–12.4) appears to be the most significant. The Rd value for Pb for this stage is estimated to be 3·104 L kg−1. The distribution ratios for other aging stages of HCP are lower. For stage I (sorption at 12.5 < pH < 13.5) Rd = 3·102 L kg−1 is proposed, for stage II (sorption to Ca(OH)2 at pH = 12.5) Rd = 3·103 L kg−1 and for stage IV (sorption to calcite at pH < 10) Rd = 8·102 L kg−1. In general, degradation of cementitious materials leads to a decrease of both the Ca/Si ratio in CSH and the concentration of ions (especially calcium) competitive for sorption. These factors can cause the increase in sorption of radionuclides onto the cement material, but only as long as CSH, i.e. the main sorbing material, is still present in it (Ochs et al., 2006).
Sorption of lead on CSH for various Ca/Si ratios (.7–1.65) and leached sulfate-resistant Portland cement (SRPC) was studied (Ochs et al., 2003). For CSH, Rd depends on the Ca/Si ratio (as the value of Ca/Si decreases, Rd increases) and the values of the distribution ratio were in the range of 103–105 L kg−1. For leached SRPC, Rd ranges from 103–104 L kg−1. The initial concentration of lead in the lead nitrate solution was between 10−4–10–6 mol L−1 and the equilibrium state for SRPC occurred after 2,600 h (approx. 108 days). Based on the data obtained equations were created to estimate the distribution ratio based e.g. on pH or lead concentration (Eq. 1)
where the unit of Rd is (L g−1) (Ochs et al., 2003).
(Bayliss et al., 1988) studied the sorption of 210Pb on fresh HCP of SRPC and OPC/BFS (Ordinary Portland cement blended with blast furnace slag) carried out under a N2 atmosphere and with L/S = 40 L kg−1. The concentration of lead in the solution was in the range of 10−3–10–9 mol L−1. The distribution ratios for SRPC were Rd = 500 L kg−1 (for a lead concentration 10–3 mol L−1) and Rd = 1,300 L kg−1 (10–5 mol L−1 Pb), while the distribution ratio for OPC/BFS came out as Rd = 2,000 L kg−1 (Pb 10–3 mol L−1; for 10–5 mol L−1 Pb the results were not evaluated). For lead concentrations bellow 10–8 mol L−1 Rd’s were obtained in the order of 105 L kg−1. Again, it was confirmed that the distribution ratio decreases with increasing initial lead concentration.
The interaction of Pb2+ ions with CCF (crushed concrete fraction, containing approx. 50% HCP CEM I, with a water/cement ratio of .4) was investigated (Coleman et al., 2005). CCF (fraction 1–2 mm) was added to Pb(NO3)2 solution of 5·10–3 mol L−1 at a phase ratio L/S = 40 L kg−1. At pH = 11.9, the equilibrium state of Pb2+ ion sorption from the aqueous solution occurred already after 48 h, when approximately 90% of Pb2+ was excluded from the solution, of which 79% was bound to CCF, the rest was precipitated. The results show that the removal of Pb2+ by CCF took place by diffusion into the cement matrix.
Sorption properties of calcite (as a material of final stage of HCP aging) towards lead at pH = 8.2, on air for 4 days were studied (Rouff et al., 2002). Increasing initial concentration of lead resulted in a decrease of Rd (2,100 L kg−1 at a lead concentration of 10–8 mol L−1 and 1,000 L kg−1 at 10–6 mol L−1). ((Ochs et al., 2016) indicates Rd = 800 L kg−1).
Evaluation of lead sorption on cement phases hydrated with portlandite water (with the formation of CSH) using sorption isotherms was done in (Moulin et al., 2000). Experiments with an initial lead concentration of 5·10–4 mol L−1 were carried out in an N2 atmosphere for 21 days at L/S 10 L kg−1 and pH 12.8. The sorption isotherm of lead sorption on CSH has a linear increasing character. The distribution ratio estimated from the given isotherm for the range of equilibrium concentration of lead in the solution 10−6–10–4 mol L−1 is in the order of 103 L kg−1, slightly increasing with decreasing concentration. Evaluation of the sorption behavior of lead on cementitious materials using isotherms were done also for CAC (calcium aluminate cement) that began to be used as an alternative building material to OPC (Navarro-Blasco et al., 2013). Description using the Langmuir isotherm determined the maximum value of the sorption capacity at qmax = 28.35 mg g−1 (corresponding to a value of .1368 mol kg−1) and the Langmuir constant as KL = 9·103 L kg−1, the value of the Freundlich constant was determined as KF = 6.135 mg1−p dm3p g−1, where p = .195.
1.1 Effect of presence of organic substances
In addition to the above mentioned influences, the sorption of radionuclides in cement systems can be affected by the presence of organic substances especially in L/ILW repositories (Park et al., 2012; Wieland et al., 2020). Organic substances can occur separately in a deposited waste in a number of forms, e.g. gloves, papers, ion exchange resins, ampoules, etc., or directly in the cement matrix, as organic compounds form the basis of some additives to concrete, especially plasticizers (influence of these materials is shown in García et al., 2018; Wieland, 2014).
Ligands of organics (most often carboxylic and hydroxycarboxylic acids) can form complexes with radionuclides and thereby affect their sorption. The most important complex-forming organic ligands include, e.g. isosaccharinic acid (ISA) as a cellulose degradation product, ethylenediaminetetraacetic acid (EDTA), nitrilotriacetic acid (NTA), gluconic acid and carboxylic acid anions (e.g. citrate, oxalate, phthalate), or other compounds such as cyanide and ammonia. Under the hyperalkaline conditions of the cement environment, the molecules of these organic acids usually have a high solubility and carry a high negative charge. In addition to the formation of complexes, sorption of organic substances to the sorption sites of cement materials and therefore competition with radionuclides can occur (Wieland, 2014; Ochs et al., 2022).
A sorption reduction factor (SRF) is used to quantify the decrease in sorption of radionuclides by complexation with organic ligands, which is useful for systems with constant conditions (constant pH) and is specific for a given set of conditions. SRF describes the decrease in radionuclide sorption depending on the concentration of the organic ligand. As summarized e.g. in (Ochs et al., 2022), SRF values for given organic ligands can be obtained directly from experiments, can be estimated based on analogy with measured solubility, or based on thermodynamic calculations. The influence of organic substances is further differentiated according to their concentration, because it has been shown that there is a threshold concentration NEC (no-effect concentration, Hummel, 1993) above which the organic substance present affects measurably the behavior of the radionuclide.
Due to difficulties in determining specific SRFs by direct methods, the available data are obtained rather by estimation based on calculations and for a whole range of experimental setups with various metals and organic ligands are summarized in (e.g. Hummel, 1993; Bradbury and Van Loon, 1998; Keith-Roach et al., 2021). For metals like Ra, Pb, Cs, Ni, Tc or Sn and actinoids from Th to Am and various organic compounds (EDTA, ISA or NTA), the assumed SRFs range from units up to several thousand. In general, the ability of organic substances to form complexes with radionuclides in the cement environment is strongly influenced by high pH = 11–13 and high Ca concentrations (10−3–10–1 mol L−1), the stability of the relevant Ca organic complexes and the concentration of the organic ligand, since complexation of radionuclide with an organic ligand usually occurs only when the concentration of the ligand is higher than the concentration of calcium in the pore water of the cement, otherwise a competitive reaction takes place with the formation of e.g. Ca-EDTA2-.
The SRF of lead is estimated to be in the order of 100–102 for various organic ligands. According to (Bradbury and Van Loon, 1998), SRFs for critical radionuclides (including Pb) occurring in a repository in the presence of cellulose degradation products, cement additives and EDTA are estimated to be in the order of units to hundreds. Specifically, EDTA significantly affects only some radionuclides and contributes to the SRF of others. Moreover, it also affects sorption only at a higher concentration (NEC >10–3 mol L−1). The SRF value for EDTA with a concentration of 4.6·10–2 mol L−1 is for Pb sorption assumed to be 5, for other affected elements (Co, Ni, Pd) approx. 50. For other organic ligands (ISA and NTA), SRF for Pb2+ sorption in contact with ISA with concentration >2·10–5 mol L−1 (expected concentration of ISA in cement water is 3·10–4 mol L−1) is equal to 100, NTA does not affect lead sorption (Keith-Roach et al., 2021).
When evaluating the safety of both existing L/ILW repositories and the planned deep geological repository, the influence of organic substances that may be part of the disposed waste as well as may be contained directly in the cementitious material must be obviously taken into account. Our research dedicated to EDTA and, to a lesser extent, phthalate is a contribution to broaden the discussion on this issue.
2 Materials and methods
Three types of different materials were chosen as representatives of cementitious matrices: newly prepared hydrated cement paste CEM I, test specimen of structural concrete containing the HCP of CEM III type from the Richard repository and pure CSH phase.
Hydrated cement paste referred to as “HCP CEM I” was prepared from Portland cement CEM I 42.5 R (CEMEX Prachovice) with water/cement ratio .4 and used as a fresh material after 14 days of hydration, i.e. in stage I of its ageing.
The concrete samples (referred here as HCP CEM III) originating from the Richard L/ILW repository were prepared during the reconstruction of the chambers in 2006. The samples were stored underground until sampling in 1/2020. According to the available information (source SÚRAO; recipe No. 377), the structural concrete is C 30/37 XA2 concrete (with a compressive strength of 30 MPa after 28 days) which contains approx. 17% of CEM III/B 32.5 blast furnace cement, 77% of aggregates, .2% of plasticizer (superplasticizer Stachement ML, Stachema) and 7% of water and was used as concrete for the final floors and walls of the chamber segments. After crushing the concrete, larger pieces of aggregate were removed for the purpose of sorption experiments.
The synthetic cement phase CSH (Ca/Si 1.0, 1.2, and 1.4) was prepared by mixing calcium oxide with high surface area silica in the presence of water in an inert atmosphere (Atkins et al., 1992; Lange et al., 2018). Specific surface areas were also determined, for CSH Ca/Si 1.0 it ranged from 181 ± 28 m2 g−1 (1 month old) to 213 ± 34 m2 g−1 (after 1.5 years), and for CSH Ca/Si 1.2 (1 year old material) 162 ± 5 m2 g−1.
As a liquid phase for the sorption experiments with HCP CEM I or concrete CEM III a simple solution was selected simulating leachate from cementitious materials due to its complexity and time variability: a saturated solution of Ca(OH)2 (portlandite or lime water) with a concentration approximately 2·10−2 mol L−1 and a pH of approximately 12.5. Portlandite water is only an approximation to the equilibrium solution. In our previous research it was observed that there is a slight decrease in the concentration of Ca in the solution of portlandite water after 4 days of contact with fresh HCP, compared to the value belonging to a saturated solution of Ca(OH)2 (cCa = 1.99·10–2 mol L−1), while the concentration of other cations (Na and mainly K) expectedly increased. The observed behavior is more pronounced for smaller L/S. The aqueous chemistry is not the same for all studied L/S, comparison of the results is only possible with some simplification. The liquid phase used for the CSH phase was its own leachate. A saturated solution of CSH Ca/Si 1.0 was analyzed by AAS, Ca and Si concentrations were determined to be cCa = 2.14·10–4 mol L−1 and cSi ≈ 2·10–4 mol L−1. Equilibrium CSH solution had pH = 10.7 (on a combined pH electrode with a range up to pH = 14).
The ratio of solid and liquid phase L/S in all sorption experiments described below was equal to 100, 250, 500 and 800 L kg−1. Moisture was determined for all materials by drying to constant weight for 3 days at 105°C. On average, HCP CEM I contained 5.3% of moisture, CEM III material 3.3% while CSH with Ca/Si 1.0 has 64% of water, Ca/Si 1.2 has 56% and Ca/Si 1.4 has 61%. For CSH, moisture depends almost entirely on the experimenter’s estimate of how dried up material is possible to work with. Hence, the moisture content of CSH used is not characteristic for the given material.
A radionuclide of interest, the 210Pb isotope is very difficult to determine, mainly due to its weak low-efficiency gamma radiation (46.536 keV, 4.25%) and weak beta emission (May et al., 2017). The initial lead concentration 5·10–4 mol L−1 allowed the use of inactive lead in combination with conventional analytical methods. In case of sorption experiments with HCP CEM I or CEM III lead was determined by the FAAS (Flame atomic absorption spectrometry) on the AA240FS device (Varian) on λPb = 283.3 nm and evaluated in the SpectraAA program. For the flame, an air/acetylene mixture was used. All samples were acidified with 1% v/v HNO3. ETA AAS (electrothermal atomization) method at the same wavelength was used for CSH experiments. To improve atomization, Pd was used as a modifier in combination with ascorbic acid. Measurements were carried out on the AA280 Z (Varian) instrument and evaluated in the SpectrAA program. Limit of detection for FAAS was on average 4.5·10–7 mol L−1, for ETA AAS 4·10−8 mol L−1.
From an extensive group of organic substances and their radiolytic or hydrolytic degradation products present in the radioactive waste, EDTA, in the form of Na2EDTA (Ethylene-diamine-tetra-acetic acid disodium salt dihydrate, C10H14N2Na2O8 · 2H2O, hereinafter referred to as EDTA (Lachema)), p. a. and phthalic acid (phthalate), manufactured by Sigma-Aldrich, with purity ≥99.5% were chosen. The organics working concentrations 5·10–5 mol L−1 and 5·10–3 mol L−1 were selected based on (Hummel, 1993). The influence of addition of organic compound can be characterized by NEC as one working concentration is below this limit and the other exceeds it. In the environment of cement leachate, the most probable form of selected organic compounds is their calcium salt.
The procedure of batch sorption experiments was following. First, the hydrated cement paste was ground into smaller pieces, until they passed through a .4 mm sieve. CSH was only ground. Furthermore, solutions of predetermined concentrations were prepared, containing either only Ca(OH)2 (or CSH leachate), or being mixed with one of the selected organic substances (EDTA and phthalate in the case of HCP, EDTA in the case of CSH). Each of these solutions also contained enough lead to match the concentration required in the experiment. The liquid phase in its final composition was prepared prior to its contact with cementitious material weighed to individual ampoules.
The amount of cement in the ampoules corresponded to the studied phase ratios (100, 250, 500, and 800 L kg−1) with a constant liquid volume of 9 mL for HCP and 6 mL for CSH, the ampoules were shaken on a shaker during the experiments (120 rpm). Finally, the ampoules were centrifuged at RCF 900 g for HCP and 2,500 g for CSH for 10 min on an MPW 350R centrifuge before sampling.
At the start of the kinetic experiment, ampoules were prepared containing a given liquid phase and a cementitious material. The samples were then removed after a total of 2 weeks at predetermined intervals. In the case of equilibrium experiments, lead samples were taken after 3 weeks from systems containing HCP (2 weeks were found not sufficient to achieve equilibrium) and after 1 week from systems with CSH. Each experimental set-up was performed in two parallel determinations.
The evaluation of equilibrium sorption experiments was performed using the distribution ratio Rd, the calculation of which can be found in the literature (e.g., Lange et al., 2018; Kittnerová et al., 2020). In the literature most often the term Kd is used (same relationship), but this term entails that the sorption on the solid has linear dependency on the concentration in the solution and is reversible (Maes et al., 2021). Sorption to the walls was taken into account.
For the description of isotherms following approaches were chosen. For linear isotherm the linear Kd-model (Eq. 2) is used:
where q (mol kg−1) is the equilibrium concentration in the solid phase, c (mol L−1) is equilibrium concentration in the liquid phase and Kd (L kg−1) is the distribution coefficient, constant over the given range of concentrations, dimensionally identical to the distribution ratio Rd.
For a non-linear isotherm, the description utilizing the Langmuir Eq. 3 was used
where KL (L kg−1) is the equilibrium constant of the addition reaction, c is the concentration in the liquid phase and qmax is the model maximum attainable concentration of the monitored component in the solid phase under the given conditions.
The Freundlich equation also describes a non-linear equilibrium isotherm (Eq. 4)
where KF (L kg−1) and p are empirical coefficients.
The given model is acceptable if “goodness of fit”, also referred to as WSOS/DF (or χ2/df, where χ2 is a weighted sum of squares and df is a number of degrees of freedom) lies within the range .1 < χ2/df < 20 (Herbelin and Westall, 1996).
The most reliable method for SRF determination is directly from the experiment using the share of the distribution ratio in the system without organics (Kd(-)) and with organics (Kd(org)) containing the required cement material, radionuclide and organic ligand according to Eq. 5.
3 Results and discussion
3.1 Lead sorption on hydrated cement paste CEM I
Lead was used in sorption experiments with HCP CEM I to observe the effect of the presence of EDTA (5·10–5 mol L−1 and 5·10–3 mol L−1) and phthalate (5·10–5 mol L−1). The lower concentration of EDTA is below the predicted NEC limit, the higher concentration is above the NEC limit hence the significant effect of this addition was expected. The average determination uncertainty was 5%.
First, a kinetic experiment (Figure 1) was performed with lead (5·10–4 mol L−1) at L/S 250 L kg−1 without/in the presence of EDTA of a lower concentration (it was assumed that the time required to reach equilibrium would be shorter at a higher concentration of EDTA). The chosen duration of 2 weeks was not fully sufficient to reach equilibrium state, and therefore for equilibrium experiments the duration was set to 3 weeks. The kinetic curves of both systems were almost identical meaning that EDTA at a low concentration does not affect the rate of equilibrium establishment. The interpolations of the experimental points were added for clarity.
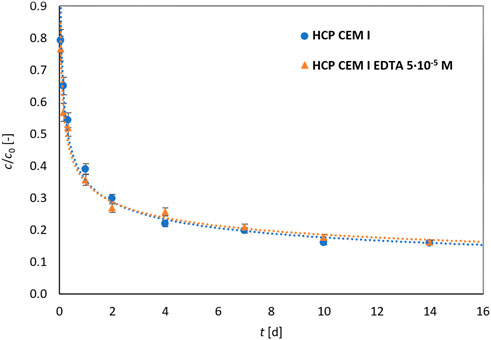
FIGURE 1. Kinetics course of lead sorption (5·10–4 mol L−1) on HCP CEM I for L/S = 250 L kg−1 with/without 5·10–5 mol L−1 EDTA.
Equilibrium experiments in the range of L/S 100–800 L kg−1 were extended by a set with the addition of EDTA of a higher concentration (except for L/S 800 L kg−1) and phthalate. The obtained dependencies of Rd on L/S are shown in Figure 2.
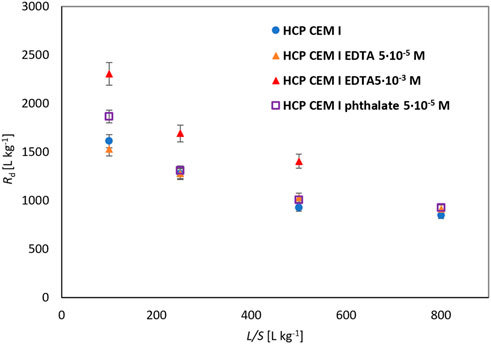
FIGURE 2. Comparison of Rd for Pb sorption on HCP CEM I for different L/S with/without organics (5·10–5 mol L−1 and 5·10–3 mol L−1).
On Figure 2 and in Table 1 a very small effect of EDTA with a lower concentration corresponding to SRF = 1.0 ± .1 is demonstrated. On the other hand, a significant increase in Rd in the system with EDTA of a higher concentration does not correspond to the expected behavior. An EDTA concentration of 5·10–3 mol L−1, which is likely above the NEC limit, has been hypothesized to cause complexation of lead in solution, thereby reducing sorption to the cementitious material (e.g. Bradbury and Van Loon, 1998). However, since SRF = .7 ± .1, effect on sorption is opposite than expected. This could be due to the entrapment of the Pb-EDTA complex or other, positively charged, complex containing Pb in the cementitious material, and possibly by other processes taking place in this unbalanced environment (Ca(OH)2 as a mere approximation to cement water) associated with the complexation of elements (e.g. Ca) with EDTA.
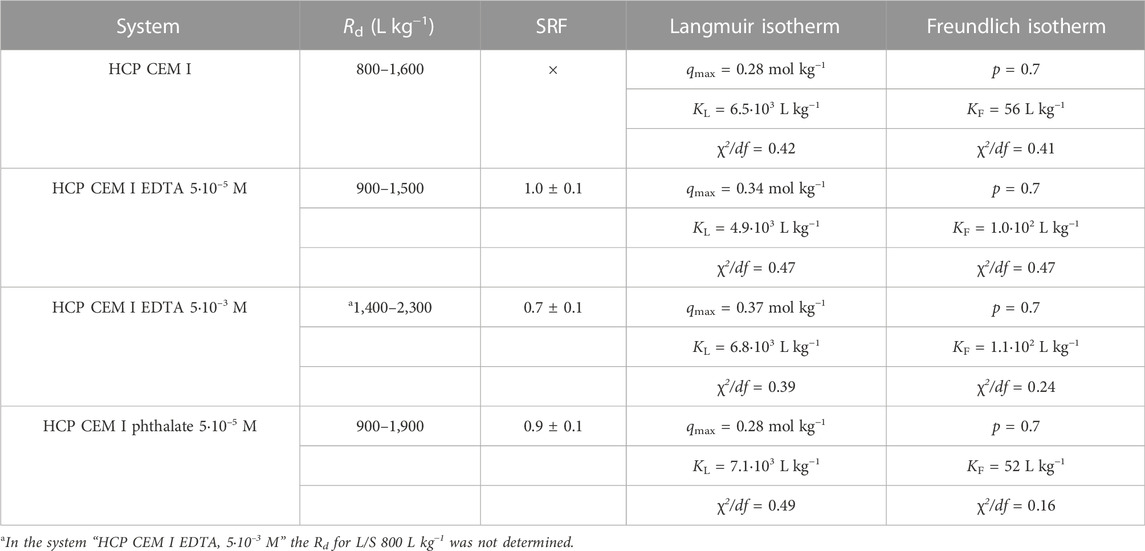
TABLE 1. The range of determined Rd values for Pb sorption on HCP CEM I with/without organics (5·10–5 mol L−1 and 5·10–3 mol L−1), the SRF corresponding to the addition of organics and the Langmuir and Freundlich isotherm parameters corresponding to the dependence of Rd values on the ratio L/S (average error of Rd determination is 5%).
As another possible explanation, the possibility of sorption of the Pb-EDTA complex on the walls of the ampoules was examined. By comparing the sorption of Pb on the walls of the ampoules in the system without EDTA and in the systems with two concentrations of EDTA, however, no significant difference was found in the rate of Pb sorption on the ampoules in the individual systems, as this value ranged from 2.4% to 4.9% and for the system with a higher concentration of EDTA, this sorption was the smallest (also applies to the system with HCP CEM III). The effect of phthalate shows a similar trend regarding SRF reduction, already at the concentration 5·10–5 mol L−1 (Table 1) which clearly appears in the system containing HCP CEM III (Table 2).
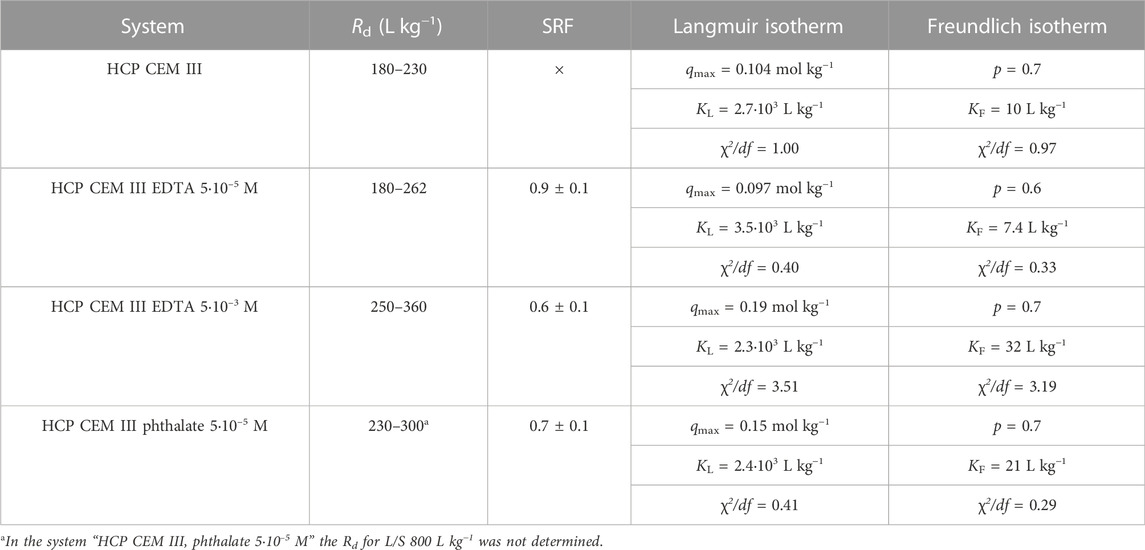
TABLE 2. The range of determined Rd values for Pb sorption on HCP CEM III with/without organics (5·10–5 mol L−1 and 5·10–3 mol L−1), the SRF corresponding to the addition of organics and the Langmuir and Freundlich isotherm parameters corresponding to the dependence of Rd values on the ratio L/S (average error of Rd determination is 8.4%).
The decrease of Rd with L/S corresponds to a concave isotherm with the parameters given in Table 1. Figure 3 shows isotherms for HCP CEM I systems without organics, with EDTA concentration of 5.10–5 mol L−1 and 5.10–3 mol L−1, and with phthalate of 5.10–5 mol L−1. For the given set of experiments, the values of the isotherm parameters are comparable (as the values of χ2/df are small). A concave-shaped sorption isotherm with a similar Langmuir parameter qmax = .14 mol kg−1 was obtained for Pb sorption on CAC (calcium aluminate cement) in the work (Navarro-Blasco et al., 2013).
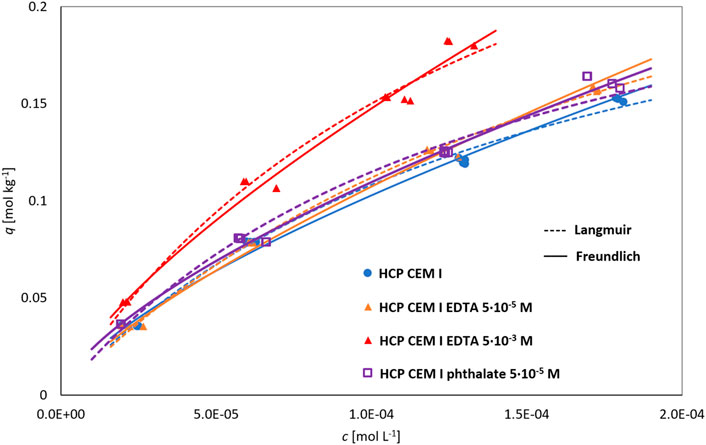
FIGURE 3. Fitting of equilibrium sorption experiments with HCP CEM I with different liquid phases by Langmuir and Freundlich isotherms (the experimental points are shown here from the parallel determinations).
The values of the distribution ratio in the order of 102–103 L kg−1 are similar to the results, for example, in (Bayliss et al., 1988). Using Eq. 1, the Rd for the initial concentration of Pb 5·10–4 mol L−1 equal to 2,029 L kg−1 was obtained, which is in good agreement with our experimental values.
3.2 Lead sorption on hydrated cement paste CEM III
The distribution ratio values for HCP CEM III are shown in Table 2 and in Figure 4. The Rd for lead sorption in the presence of phthalate (5·10–5 mol L−1) at L/S = 800 L kg−1 was not determined. For the distribution ratios of HCP CEM III for L/S 100, 250 and 500 L kg−1, a slight influence of the organic component in the liquid phase can be seen even at low concentrations. The value of SRF ∼1 for EDTA with a concentration of 5·10–5 mol L−1 corresponds to the assumptions about the behavior of Pb below the concentration corresponding to the NEC, which is believed to be for the Pb EDTA system <1·10–3 mol L−1 (Bradbury and Van Loon, 1998). In contrast, at a higher concentration of EDTA (5·10–3 mol L−1) the expected behavior (decrease in sorption resulting in SRF >1) was not confirmed. The effect of phthalate (5·10–5 mol L−1) was similar to that of EDTA at a higher concentration.
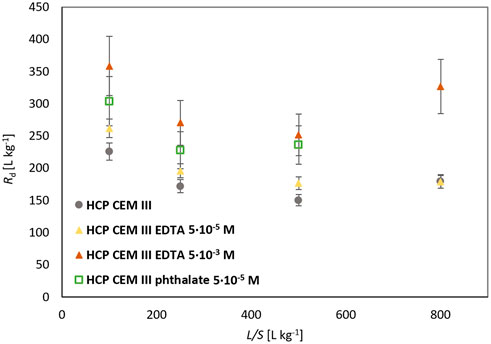
FIGURE 4. Comparison of Rd for Pb sorption on HCP CEM III for different L/S with/without organics (5·10–5 mol L−1 and 5·10–3 mol L−1).
A general trend of increasing Rd was observed in the order: organic-free < EDTA (5·10–5 mol L−1) < phthalate (5·10–5 mol L−1) < EDTA (5·10–3 mol L−1) that is more pronounced than for the systems containing HCP CEM I.
Values of Rd’s for HCP CEM III are difficult to compare regarding the sorption of lead on a similar material. (Bayliss et al., 1988) reported Rd = 2,000 L kg−1 for L/S = 40 L kg−1 at a lead concentration of 10–3 mol L−1 on fresh HCP of OPC/BFS. The experimentally determined value of the distribution ratio for HCP CEM III, L/S = 100 L kg−1, with a lead concentration of 5·10–4 mol L−1 is 225 L kg−1. The value is therefore an order of magnitude lower than in the mentioned study, which could be due to a different material, phase ratio and working concentrations.
From a comparison of Table 1 and 2 is evident that distribution ratios for HCP CEM I are approximately 6–times higher than for HCP CEM III. This result is slightly unexpected as on concrete slightly higher Rd’s are obtained than on HCP, which is consistent with the assumption of higher Rd values in concretes due to the lower Ca/Si ratio (e.g. Lange et al., 2018) caused by the addition of admixtures (Richardson, 2008).
According to the parameter KL > 0 of the Langmuir isotherm and the parameter p < 1 of the Freundlich isotherm, isotherms in Table 2 have also a concave shape, that corresponds to the natural course of the sorption isotherm. The values of the isotherm parameters are again comparable relating to the values of χ2/df.
3.3 Lead sorption on calcium-silicate-hydrates
Determination of several effects on CSH sorption, namely the presence of EDTA at two concentrations and the Ca/Si ratio of the CSH material, was performed with lead at a concentration of c = 5·10–4 mol L−1. The average determination uncertainty was 23%.
All the equilibrium dependencies of the distribution ratio Rd on the phase ratio L/S are considerably fluctuating for Pb CSH systems, which may be caused by the measurement uncertainty, since the measurement method (ETA AAS) is more sensitive to sample preparation errors and sample purity than radiometric methods used for e.g. Ra (Kittnerová et al., 2022). Another significant factor is the sorption to the walls, which in these systems reaches a value of 80 ± 15% for system without EDTA and with EDTA of lower concentration. However, sorption to the walls is significantly lower for a system with a solid phase. The correction procedure for sorption to the walls, based on the subtraction of the part of the concentration of the monitored element attached to the walls and the concentration available for sorption from the concentration captured on the solid phase in this case significantly affects the absolute concentration results for lead in liquid phase. After the comparison of Rd values obtained with and without the correction of the wall sorption, the corrected values were taken into account leading to a more conservative results of Rd. It is one of the phenomena that can introduce additional uncertainty into the composition of experimental solutions.
Despite the fluctuation of the obtained dependencies, they can be considered linear rather than clearly decreasing or increasing, which was also confirmed by the evaluation of non-linear isotherms (Langmuir and Freundlich), which in most cases did not yield an usable description of the given dependence, while the one evaluated by the linear isotherm (Kd-model) was more successful.
3.3.1 Effect of the presence of organic compounds on lead sorption
When comparing the kinetic curve of Pb sorption on CSH Ca/Si 1.0 with/without EDTA at two concentrations (5·10–5 mol L−1 and 5·10–3 mol L−1) with L/S 800 L kg−1, a clear effect is evident, since even in the presence of EDTA with a lower concentration, there was a slight increase in the Pb sorption, while with a higher concentration of EDTA sorption almost stopped (Figure 5). Values of non-adsorbed fraction of CSH Ca/Si 1.0 EDTA 5·10–3 mol L−1 series exceeding c/c0 = 1 are due to measurement uncertainty as shown by error bars.
In the equilibrium experiments with CSH Ca/Si 1.0 and 1.2, a clear effect of the addition of EDTA was observed, when already at a lower concentration of EDTA (5·10–5 mol L−1) the sorption is reduced approx. 4–times and when moving to a higher concentration EDTA (5·10–3 mol L−1) SRF reach values of several hundred (Table 3). The obtained results agree with general estimates from (Bradbury and Van Loon, 1998), where possible SRFs for Pb and various organic substances (especially cellulose degradation products) are described as in range of hundreds corresponding to the estimate of NEC for EDTA = 10–3 mol L−1. In our case the NEC value is lower, as for an EDTA concentration of 5·10–5 mol L−1 there is still a reduction in sorption quantified by SRF ≈ 5. This value for SRF is a specific estimate of the system with EDTA at a concentration of the order of 10–2 mol L−1 in (Bradbury and Van Loon, 1998), however, the estimate based on calculations, not an experiment.
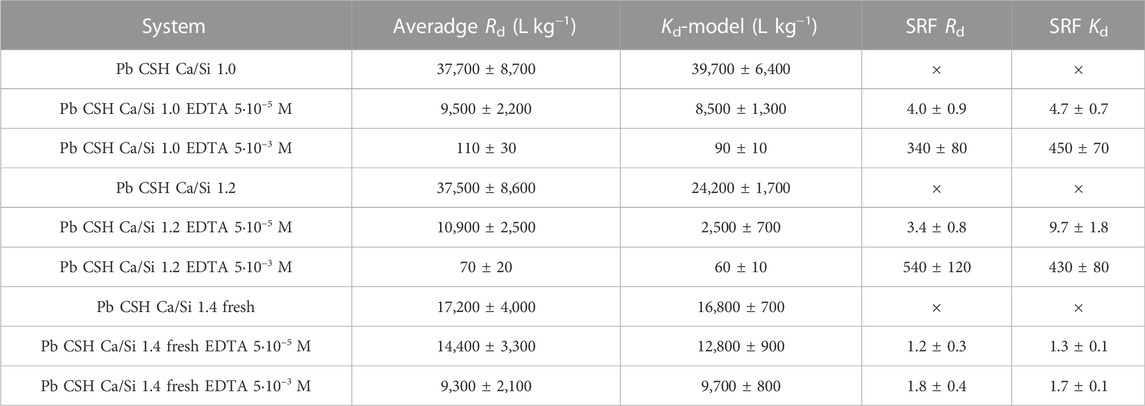
TABLE 3. Average Rd values of Pb sorption on CSH Ca/Si 1.0, 1.2 and 1.4 without/in the presence of EDTA (5·10–5 mol L−1 and 5·10–3 mol L−1) compared to the Kd values obtained from the Kd-model and SRF corresponding to EDTA addition.
Another significant effect of the addition of EDTA with a higher concentration is the almost complete suppression of lead sorption on the walls of the ampoules and a change in the shape of the dependence of Rd on L/S for CSH Ca/Si 1.0 and 1.2, which changes from a rather linear shape to a slightly decreasing one. However, a suitable isotherm was not found for these dependencies, and given that the decrease is only very slight, these data series were also evaluated using a linear isotherm (Table 3). The average Rd and Kd from the Kd-model obtained for these two Ca/Si ratios are in good agreement except for the case of system CSH Ca/Si 1.2 EDTA 5.10–5 M, the SRFs are also similar within the uncertainty.
In the equilibrium experiments with CSH Ca/Si 1.4 (Figure 6; Table 3), a good agreement was again achieved between the average Rd and Kd from the Kd-model, but a significant effect of the addition of the organic compound was not observed here, as the SRF reach values (SRFRd) of 1.2 ± .3 for a lower concentration of EDTA and 1.8 ± .4 for a higher concentration of EDTA (Table 3), which means only a slight suppression of sorption, especially compared to the previously mentioned Ca/Si 1.0 and 1.2. This behavior may be due to the general behavior of cations, which are widely sorbed less on CSH with higher Ca/Si (Table 3), which may affect the relative decrease in sorption with the addition of EDTA. The Ca/Si 1.4 material was also freshly prepared, which may change its properties and the variance or uncertainty of the determined results. Moreover, no change in the dependence of Rd on L/S was observed, which is rather linear for all cases. A fit of the experimental data for the CSH Ca/Si 1.4 material is shown in Figure 7.
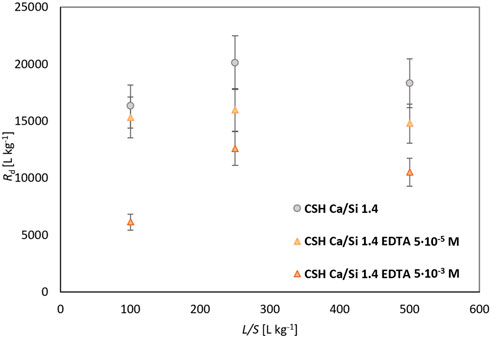
FIGURE 6. Comparison of Rd for Pb sorption on CSH Ca/Si 1.4 for different L/S with/without EDTA (5·10–5 mol L−1 and 5·10–3 mol L−1).
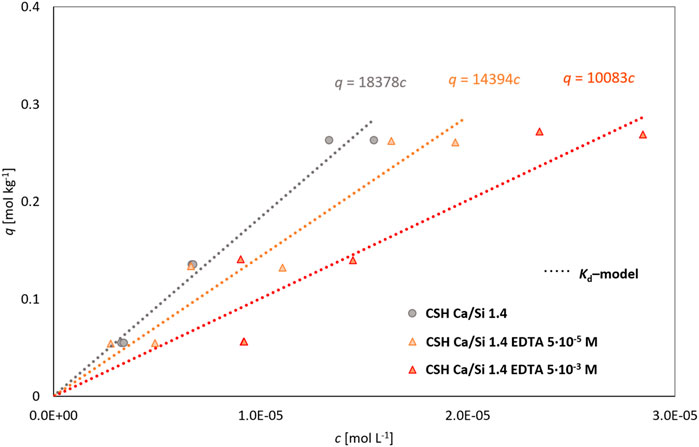
FIGURE 7. Fitting of experiments with CSH Ca/Si 1.4 with different liquid phase by Kd-model (the experimental points are shown here from the parallel determinations).
Regarding the obtained data, it is also necessary to note that for the Ca/Si ratio of 1.2 and 1.4, only 3 L/S ratios (100–500 L kg−1) were used for the determination.
Speciation calculations were performed in PhreeqC ver. 3.7.3 using the ThermoChimie database ver. 10d (Parkhurst and Appelo, 2013) for Pb with a concentration of 5·10–4 mol L−1 and CSH Ca/Si 1.2 solution (without CO2 access). The systems studied differ in rough correlation with Rd results. In the case of the organic-free system and the system with 5·10–5 mol L−1 EDTA, the Pb(OH)42- ion clearly dominates (75%), accompanied only by the species Pb(OH)3-. With the addition of EDTA at a higher concentration, there is a substantial change in the speciation diagram, in which the Pb(EDTA)2- ion is presented almost at 100%.
3.3.2 Effect of Ca/Si ratio on lead sorption
The effect of the Ca/Si ratio of CSH on Pb sorption can also be described based on the data in Table 3 by comparing the values without the presence of EDTA. The average Rd of the material with Ca/Si 1.0 and 1.2 can be considered rather comparable, however, both of these values are significantly higher than for Ca/Si 1.4. For the values obtained from the Kd-model, the predicted trend of Kd 1.0 > Kd 1.2 > Kd 1.4 was observed. For CSH Ca/Si 1.4 the assumption of the lowest sorption on CSH with the highest Ca/Si applied, it must be noted that this material was freshly prepared (unlike the materials with Ca/Si 1.0 and 1.2, which were already several months old at the time of the experiments), and with time there could be a slight increase in Rd values, as observed e.g. for Ra (Kittnerová et al., 2022).
4 Conclusion
The sorption behavior of lead was studied on five different cementitious materials, also in the presence of EDTA or phthalate.
The Rd values for lead sorption onto HCP CEM I in portlandite water ranged from 900–1,600 L kg−1 for L/S 100–800 L kg−1. For HCP CEM III, the distribution ratio was found to be approximately 6–times lower (180–230 L kg−1) under same conditions. In general, Rd increased with decreasing L/S ratio and simultaneously, a trend of increasing Rd was observed in the series: organic-free system < EDTA (5·10–5 mol L−1) < phthalate (5·10–5 mol L−1) < EDTA (5·10–3 mol L−1), more significantly for HCP CEM III. The effect of the presence of both organic substances, especially EDTA at a concentration of 5·10–3 mol L−1 considered higher than NEC, was opposite to expectations, i.e. with SRF ≤1. To describe the sorption behavior, an evaluation using non-linear Langmuir and Freundlich sorption isotherms, which showed a concave shape, was used.
Slightly contradictory results were obtained for pure cement phases, CSH. For at least 1 year old CSH with Ca/Si 1.0 and with Ca/Si 1.2, average Rd values of 38,000 L kg−1 were similarly found for the same L/S range as in the case of HCP. The studied systems could be described by the Kd-model. The achieved Rd was reduced by influences including the use of fresher CSH and in the presence of organics. The most significant effect shown EDTA with a concentration of 5·10–3 mol L−1 that almost eliminated lead sorption with SRF over 500.
Further research is ongoing.
Data availability statement
The raw data supporting the conclusions of this article will be made available by the authors, without undue reservation.
Author contributions
BD and JK led the experimental study. JK, KB and MB performed the experiments. All authors conducted data evaluation. LB contributed speciation. BD wrote the first draft of the manuscript. All authors contributed to manuscript revision, read, and approved the submitted version.
Funding
The research leading to these results has received funding from the European Union’s Horizon 2020 Innovation Programme under grant agreement n° 847593 (EURAD–CORI). The output was created with the financial participation of SÚRAO (Czech Radioactive Waste Repository Authority) (SO 2020-017). This contribution is also partially a result of European Regional Development Fund-Project “Centre for Advanced Applied Sciences” (Grant No. CZ.02.1.01/0.0/0.0/16_019/0000778) and the grant of the CTU Student Grant Scheme No. SGS22/187/OHK4/3T/14.
Conflict of interest
The authors declare that the research was conducted in the absence of any commercial or financial relationships that could be construed as a potential conflict of interest.
Publisher’s note
All claims expressed in this article are solely those of the authors and do not necessarily represent those of their affiliated organizations, or those of the publisher, the editors and the reviewers. Any product that may be evaluated in this article, or claim that may be made by its manufacturer, is not guaranteed or endorsed by the publisher.
References
Atkins, M., Glasser, F. P., and Kindness, A. (1992). Cement hydrate phase: Solubility at 25°C. Cem. Concr. Res. 22, 241–246. doi:10.1016/0008-8846(92)90062-Z
Bayliss, S., Ewart, F. T., Howse, R. M., Smith-Briggs, J. L., Thomason, H. P., and Willmott, H. A. (1988). The solubility and sorption of lead-210 and carbon-14 in a near-field environment. MRS Online Proc. Libr. 112, 33–42. doi:10.1557/proc-112-33
Berner, U. (2003). Project Opalinus Clay: Radionuclide concentration limits in the cementitious near-field of an ILW repository. PSI Ber. N. R. 02-26, 62.
Bradbury, M. H., and Van Loon, L. R. (1998). Cementitious near-field sorption data bases for performance assessment of a LIILW repository in a paifris marl host rock CEM-94: UPDATE I, june 1997. Switzerland: PSI Berich, 86. PSI Bericht Nr. 98-01.
Brownsword, M., Buchan, A. B., Ewart, F. T., McCrohon, R., Ormerod, G. J., Smith-Briggs, J. L., et al. (1989). The solubility and sorption of uranium(VI) in a cementitious repository. MRS Proc. 176, 577–582. doi:10.1557/proc-176-577
Coleman, N. J., Lee, W. E., and Slipper, I. J. (2005). Interactions of aqueous Cu2+, Zn2+ and Pb2+ ions with crushed concrete fines. J. Hazard. Mat. 121, 203–213. doi:10.1016/j.jhazmat.2005.02.009
Evans, N. D. M. (2008). Binding mechanisms of radionuclides to cement. Cem. Concr. Res. 38, 543–553. doi:10.1016/j.cemconres.2007.11.004
García, D., Grivé, M., Duro, L., Brassinnes, S., and de Pablo, J. (2018). The potential role of the degradation products of cement superplasticizers on the mobility of radionuclides. Appl. Geochem. 98, 1–9. doi:10.1016/j.apgeochem.2018.09.004
Herbelin, A. L., and Westall, J. C. (1996). FITEQL-A computer program for determination of chemical equilibrium constants from experimental data. Corvallis, Oregon: Department of Chemistry, Oregon State University. Report 96-01 Version 3.2.
Hummel, W. (1993). Organic complexation of radionuclides in cement pore water: A case study. PSI Intern. Rep., 33.
Keith-Roach, M., Lindgren, M., and Källström, K. (2021). Assessment of complexing agent concentrations for the post-closure safety assessment in PSAR SFR, 75. SKB Report R-20-04.
Kittnerová, J., Drtinová, B., Štamberg, K., Deissmann, G., Lange, S., and Evans, N. (2022). Study of radium behavior in contact with calcium-silicate-hydrates. ASME J Nucl. Rad Sci 9 (1), 011901. doi:10.1115/1.4055160
Kittnerová, J., Drtinová, B., Štamberg, K., Vopálka, D., Evans, N., Deissmann, G., et al. (2020). Comparative study of radium and strontium behaviour in contact with cementitious materials. Appl. Geochem. 122, 104713. doi:10.1016/j.apgeochem.2020.104713
Lange, S., Kowalski, P. M., Pšenička, M., Klinkenberg, M., Rohmen, S., Bosbach, D., et al. (2018). Uptake of 226Ra in cementitious systems: A complementary solution chemistry and atomistic simulation study. Appl. Geochem. 96, 204–216. doi:10.1016/j.apgeochem.2018.06.015
Maes, N., Glaus, M., Baeyens, B., Marques Fernandes, M., Churakov, S., Dähn, R., et al. (2021). State-of-the-Art report on the understanding of radionuclide retention and transport in clay and crystalline rocks. EC Grant agreement no, 847593. Final version as of 30.04.2021 of deliverable D5.1 of the HORIZON 2020 project EURAD.
May, D., Nelson, A. N., and Schultz, M. K. (2017). Quantitation of lead-210 (210Pb) using lead-203 (203Pb) as a “Massless” yield tracer. J. Environ. Radioact. 171, 93–98. doi:10.1016/j.jenvrad.2017.02.003
Moulin, I., Rose, J., Stone, W., Bottero, J. Y., Mosnier, F., and Haehnel, C. (2000). Lead, zinc and chromium (III) and (VI) speciation in hydrated cement phases. Waste Mat. Constr. 1, 269–280. doi:10.1016/S0713-2743(00)80039-2
Moulin, I., Stone, W. E. E., Sanz, J., Bottero, J. Y., Mosnier, F., and Haehnel, C. (1999). Lead and zinc retention during hydration of tri-calcium silicate: A study by sorption isotherms and 29Si nuclear magnetic resonance spectroscopy. Langmuir 15, 2829–2835. doi:10.1021/la981062u
Navarro-Blasco, I., Duran, A., Sirera, R., Fernández, J. M., and Alvarez, J. I. (2013). Solidification/stabilization of toxic metals in calcium aluminate cement matrices. J. Hazard. Mat. 260, 89–103. doi:10.1016/j.jhazmat.2013.04.048
Nonat, A. (2004). The structure and stoichiometry of C-S-H. Concr. Res. 34, 1521–1528. doi:10.1016/j.cemconres.2004.04.035
Ochs, M., Dolder, F., and Tachi, Y. (2022). Decrease of radionuclide sorption in hydrated cement systems by organic ligands: Comparative evaluation using experimental data and thermodynamic calculations for ISA/EDTA-actinide-cement systems. Appl. Geochem. 136, 105161. doi:10.1016/j.apgeochem.2021.105161
Ochs, M., Mallants, D., and Wang, L. (2016). Radionuclide and metal sorption on cement and concrete. Springer, 322. doi:10.1007/978-3-319-23651-3_9
Ochs, M., Pointeau, I., and Giffaut, E. (2006). Caesium sorption by hydrated cement as a function of degradation state: Experiments and modelling. Waste Manag. 26, 725–732. doi:10.1016/j.wasman.2006.01.033
Ochs, M., Talerico, C., Lothenbach, B., and Giffaut, E. (2003). Systematic trends and empirical modeling of lead uptake by cements and cement minerals. MRS Proc. 757, 101–106. doi:10.1557/PROC-757-II10.1
Park, J. B., Kim, C. W., Kim, S. H., and Kim, J. Y. (2012). Determination of a representative composition of dry active waste from Korean nuclear power plants. Ann. Nucl. Energy 42, 89–95. doi:10.1016/j.anucene.2011.12.004
Parkhurst, D. L., and Appelo, C. A. J. (2013). Description of input and examples for PHREEQC Version 3 – a computer program for speciation, batch-reaction, one-dimensional transport, and inverse geochemical calculations. U.S. Geol. Surv. Tech. Methods, book 6, 497. chapter A43. doi:10.3133/tm6A43
Richardson, I. G. (2008). The calcium silicate hydrates. Cem. Concr. Res. 38, 137–158. doi:10.1016/j.cemconres.2007.11.005
Rose, J., Moulin, I., Hazemann, J. L., Masion, A., Bertsch, P. M., Bottero, J. Y., et al. (2000). X-Ray absorption spectroscopy study of immobilization processes for heavy metals in calcium silicate hydrates: 1. Case of lead. Langmuir 16, 9900–9906. doi:10.1021/la0005208
Rouff, A. A., Reeder, R. J., and Fisher, N. S. (2002). Pb (II) sorption with calcite: A radiotracer study. Aquat. Geochem. 8, 203–228. doi:10.1023/B:AQUA.0000003729.05602.de
Wieland, E., Kosakowski, G., Lothenbach, B., and Kulik, D. A. (2020). Geochemical modelling of the effect of waste degradation processes on the long-term performance of waste forms. Appl. Geochem. 115, 104539. doi:10.1016/j.apgeochem.2020.104539
Keywords: cement, calcium-silicate-hydrates, lead, organics, deep geological repository, sorption
Citation: Drtinová B, Kittnerová J, Bergelová K, Burešová M and Baborová L (2023) Sorption of lead on cementitious materials in presence of organics. Front. Nucl. Eng. 1:1095233. doi: 10.3389/fnuen.2022.1095233
Received: 10 November 2022; Accepted: 16 December 2022;
Published: 06 January 2023.
Edited by:
Michael Ojovan, Imperial College London, United KingdomReviewed by:
Tiziana Missana, Medioambientales y Tecnológicas, SpainTaishi Kobayashi, Kyoto University, Japan
Copyright © 2023 Drtinová, Kittnerová, Bergelová, Burešová and Baborová. This is an open-access article distributed under the terms of the Creative Commons Attribution License (CC BY). The use, distribution or reproduction in other forums is permitted, provided the original author(s) and the copyright owner(s) are credited and that the original publication in this journal is cited, in accordance with accepted academic practice. No use, distribution or reproduction is permitted which does not comply with these terms.
*Correspondence: Barbora Drtinová, YmFyYm9yYS5kcnRpbm92YUBmamZpLmN2dXQuY3o=