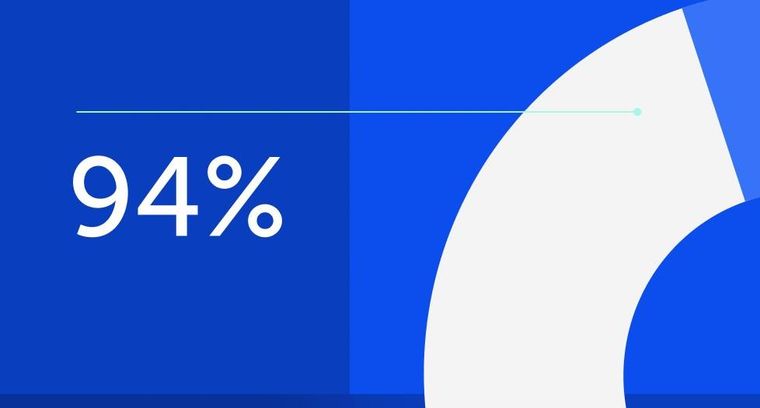
94% of researchers rate our articles as excellent or good
Learn more about the work of our research integrity team to safeguard the quality of each article we publish.
Find out more
REVIEW article
Front. Neurosci., 09 April 2025
Sec. Visual Neuroscience
Volume 19 - 2025 | https://doi.org/10.3389/fnins.2025.1560344
This article is part of the Research TopicRetinal Neuroprotection: Exploring Novel and Repurposed TherapeuticsView all articles
Glial cells play a crucial role in maintaining central nervous system (CNS) homeostasis and facilitating the repair of neural tissue following injury. The regulation of neuroglia may serve as a safe and effective strategy for modulating neuroinflammatory responses and restoring glial homeostasis and defense functions. Given that the glial network is composed of connexin (CX) proteins, its neuroprotective role is extensive. Therefore, connexins should be considered as functional “bridges” within this network. This review examines evidence for the active involvement of glial networks in neuroinflammation under both physiological and pathological conditions and summarizes the role of CXs in glaucoma. Finally, potential therapeutic strategies for glaucoma are explored.
Neuroglia, represented by astrocytes, oligodendrocytes, and microglia, are essential for maintaining the stability of the CNS (Tezel, 2022). They are crucial for preserving the structural integrity of the retina and serve as key regulators of retinal cell metabolism (Yoo et al., 2021). Pathological stimulation of the CNS, such as neuronal injury or various forms of damage, can activate glial cells. Reactive glial proliferation, observed in nearly all retinal diseases, supports retinal neuron survival but may also accelerate neuronal degeneration (Ou et al., 2022). These processes are associated with CXs on glial cells, which form highly interconnected networks through gap junctions (GJs) or hemichannels (HCs; Giaume et al., 2021). They maintain neuronal function under physiological conditions but accelerate disease progression under pathological conditions (Giaume et al., 2021; Huang et al., 2021). Substantial evidence has demonstrated the role of GJs and HCs in various CNS diseases, including but not limited to neurodegenerative disorders, with Cx43 in particular implicated in a wide range of neuropathologies (Charvériat et al., 2021). For instance, sites of enhanced Cx43 immunoreactivity have been identified in patients with Alzheimer's disease (AD) and in animal models of amyotrophic lateral sclerosis (ALS) and Parkinson's disease (PD; Provenzano et al., 2023; Maldonado et al., 2022; Seo et al., 2021). Inhibitors of glial cell CXs have been extensively studied over recent decades and have been found to differentially downregulate CXs expression in neurodegenerative diseases, chronic pain, epilepsy, and demyelinating disorders. These inhibitors regulate channel switching and suppress glial cell activation, thereby protecting neurons (Giaume et al., 2021; Charvériat et al., 2021).
In recent years, several studies have demonstrated that glial cell-mediated neuroinflammation influences the pathophysiology of glaucoma (Hu et al., 2021; Mélik Parsadaniantz et al., 2020). Glial cells are highly interconnected through CXs and function as an extensive cellular network (Boal et al., 2021). Therefore, this review explores the role of glial protein CXs in the neuroinflammatory response to glaucoma, extending beyond a focus on astrocytes as individual cellular units to provide insights for future research and therapeutic strategies.
Glaucoma, a primary cause of irreversible blindness worldwide, is characterized by progressive optic nerve damage and visual field loss (Quigley, 1999). Approximately 80 million people are affected by the disease, and this figure is projected to surpass 100 million by 2040 (Tham et al., 2014; Bourne et al., 2013). Clinically, glaucoma is categorized into open-angle glaucoma (OAG) and closed-angle glaucoma (ACG), based on the configuration of the anterior chamber angle. Current clinical treatments primarily focus on reducing intraocular pressure (IOP); however, despite effective IOP control, ~15%−25% of patients continue to experience progressive optic nerve damage (Soto and Howell, 2014; Tezel, 2022).
Mechanical and vascular factors are believed to contribute to the etiology of glaucoma (McMonnies, 2018). The pathogenic mechanisms of glaucoma are complex and not yet fully understood. Recent studies suggest that glaucomatous optic neuropathy is a neurodegenerative disorder sharing common neuroinflammatory mechanisms with classical neurodegenerative diseases (Mélik Parsadaniantz et al., 2020). The CNS, which includes the brain, retina, and optic nerve, is an immune-privileged tissue (Lampron et al., 2013). Neurodegenerative diseases exhibit specific mechanisms that induce inflammatory responses, such as the production of inflammatory inducers, the activation of innate immune cells in the CNS as sensors of neurological damage, and the amplification of key regulated transcription factors, leading to inflammatory responses, neurotoxicity, and neuronal death (Teleanu et al., 2022; Voet et al., 2019). In contrast to classical inflammation, glial-driven neuroinflammation exists in an intermediate state known as para-inflammation (Medzhitov, 2008). While this state may initially represent a beneficial response, it can subsequently progress into a neurodegenerative process (Bariş and Tezel, 2019).
Astrocytes and microglia are resident immune cells that perform innate immune functions within the retina and optic nerve (Salkar et al., 2024). Astrocytes play a crucial role in maintaining tissue homeostasis within the retina and optic nerve. Under physiological conditions, astrocytes form networks through CXs to sustain the homeostatic environment necessary for neuronal function (Lopez Ortiz and Eyo, 2024). However, studies have indicated that excessive activation of astrocytes can contribute to retinal ganglion cell (RGC) damage (Boal et al., 2021). Reactive astrocytes mediate extracellular matrix remodeling and pro-fibrotic processes, ultimately accumulating within the optic nerve head, thereby increasing glaucoma-related biomechanical and vascular pressures and promoting RGC apoptosis (Burgoyne, 2011).
Microglia are tissue-resident immune cells which are crucial for initiating inflammatory responses. These cells continuously monitor and maintain homeostasis through clearance and phagocytosis, while also providing neurotrophic support (Borst et al., 2021). They can switch between M1 (pro-inflammatory) and M2 (anti-inflammatory) phenotypes in response to environmental stimuli (Orihuela et al., 2016). They exhibit sensor and effector functions, as well as phagocytic activity, under both physiological and pathological conditions (Borst et al., 2021). Like macrophages, hyperactivated microglia can promote neuroinflammation. This process may be associated with the secretion of pro-inflammatory cytokines, such as interleukin-6 (IL-6) and tumor necrosis factor-alpha (TNFα), as well as reactive oxygen species (Woodburn et al., 2021). The proliferation of reactive microglia has also been observed in experimental glaucoma models (Fernandez-Albarral et al., 2022).
Microglia in the glaucomatous retina often demonstrate a mixed activation phenotype. M2 microglia are activated only in the early stages of elevated IOP, whereas M1 microglia remain continuously activated throughout sustained elevated IOP. In experimental models of glaucoma, elevated IOP results in the differentiation of most microglial cells into an M1 phenotype, while only a few M2 microglial cells are identified within RGCs (Wei et al., 2019). In the chronic ocular hypertension retina, ATP-induced microglial activation stimulates the release of pro-inflammatory factors (Xu et al., 2022). Additionally, ATP transiently increases the mRNA levels of anti-inflammatory factors in microglia. However, the protein concentrations of these anti-inflammatory factors remain relatively low, resulting in negligible neuroprotective effects of activated microglia (Hu et al., 2021). These findings suggest that while activated microglia may provide some neuroprotection in the early stages of glaucoma, their activation predominantly contributes to RGC damage by exacerbating neuroinflammation.
Glial cells play a crucial role in maintaining CNS homeostasis and facilitating repair following injury. Their neuroprotective function is extensive due to the presence of glial GJs, which are composed of CXs (Caruso et al., 2023; Denaro et al., 2025). CXs constitute a multigene family, with 21 members identified in humans (Danish et al., 2021). The expression patterns of Cx proteins in various types of glial cells are summarized in Table 1.
CXs exhibit unique physiological and pathological functions across different subtypes. Cx43, expressed in astrocytes, RGCs, and TM, regulates GJs, HCs, ATP release, and inflammatory responses, impacting neuroinflammation and ECM remodeling (Chen and Tang, 2022; Tellios et al., 2019; Sui et al., 2021). Cx30, in astrocytes and Müller cells, modulates synaptic plasticity, glutamate regulation, and neuroprotection (Giaume et al., 2021; Li et al., 2025). Cx32, in oligodendrocytes and Schwann cells, supports myelin maintenance and potassium buffering, with mutations causing X-linked Charcot-Marie-Tooth disease (Kleopa, 2011; Sargiannidou et al., 2009; Oguro et al., 2001). During the prenatal stage in mice, Cx26 has been reported to be expressed in neural progenitor cells, where it is primarily associated with neuronal migration and the development of neocortical circuits (Oguro et al., 2001). Cx36 is a neuron-specific Cx capable of forming functional channels. Cx45 has been observed to exhibit immunoreactivity in some MAP2 neurons and non-neuronal cells (Condorelli et al., 2003). Cx47, also in oligodendrocytes, facilitates oligodendrocyte-astrocyte communication, with dysfunction associated with leukodystrophies and multiple sclerosis (Nutma et al., 2020). These isoforms collectively underpin glial and neuronal function, with their dysregulation contributing to neurodegenerative and neuroinflammatory disorders.
HCs are transmembrane channels consisting of six Cx subunits. Two hemichannels can pair to create a complete GJ, enabling direct communication between adjacent cells. However, when hemichannels remain isolated within the cell membrane, they are referred to as “hemichannels” or “connexons,” facilitating molecular exchange between the intracellular and extracellular environments. HCs primarily facilitate the transfer of ions and small molecules between these compartments (Bennett et al., 2003; Lei et al., 2023; Bennett et al., 2012). A single GJs is composed of two HCs or connexons, each formed by six CXs subunits. Moreover, glial cell communication occurs either directly through GJs or over short distances via autocrine/paracrine signaling through HCs. GJs permit the intercellular exchange of various molecules, including ions (Ca2+, K+), essential nutrients such as glucose, signaling molecules such as ATP, cAMP, and IP3, as well as the neurotransmitter glutamate (Anderson and Swanson, 2000; Harris, 2007; Vinken, 2015; Figure 1).
Figure 1. Schematic illustration of the working model glial CX in neurologic disease (draw by Figdraw). Under normal physiological conditions, gap junctions (GJs) allow the exchange of molecules between cells. These permeable molecules include ions (Ca2+, K+), nutrients (e.g., glucose), signaling molecules (e.g., ATP), and the neurotransmitter glutamate. Under pathological conditions, astrocytes and microglia become activated. Microglia secrete proinflammatory cytokines, such as IL-1β and TNF-α, and subsequently activate hemichannels (HCs). Excessive ATP and glutamate release by astrocytes, in turn, act on microglia, initiating an inflammatory cascade.
Astrocytes play a crucial role in the neuroinflammatory response (Huang et al., 2021). The glial network requires further investigation due to its significance in this process. In general, GJs are believed to exert various neuroprotective functions. From both metabolic and buffering perspectives, GJs contribute to neuroprotection by redistributing nutrients to damaged regions and regulating the extracellular environment. However, the neuroprotective effect of GJs appears to be condition-dependent. GJs have been observed to close under extreme conditions, such as high intracellular Ca2+ concentrations, to prevent the propagation of death signals through the astrocytic network (De Vuyst et al., 2009). This response may be associated with the duration and severity of inflammatory damage. HCs play a pivotal role in inflammatory conditions, exhibiting contrasting pathophysiological roles compared to GJs. Often regarded as “pathological channels,” HCs exhibit pro-degenerative properties in pathological conditions. During neuroinflammation, activated microglia and astrocytes lead to the secretion of IL-1β and TNF-α pro-inflammatory cytokines by microglia, subsequently opening HCs. This exacerbates neuro-excitotoxicity through excessive ATP and glutamate release, perpetuating a cycle of chronic inflammation (Denaro et al., 2025). These findings suggest that Cx proteins play a pivotal role in neuroinflammation.
In the EAE (experimental autoimmune encephalomyelitis) animal model of multiple sclerosis, conditional knockout of Cx43 resulted in a reduction in the expression of pro-inflammatory cytokines IL-6, interferon-γ, and IL-10, leading to attenuated inflammatory responses and decreased demyelination. These findings suggest that knockout of Cx43 promotes astrocyte polarization toward an anti-inflammatory phenotype and ameliorates EAE pathogenesis by lowering pro-inflammatory cytokine and chemokine concentrations in the cerebrospinal fluid (Une et al., 2021). Additionally, studies on Cx30 knockout (KO) EAE mice revealed extensive microglial activation during the chronic phase of EAE. Furthermore, Cx30 deficiency facilitated astrocyte activation and promoted the differentiation of neuroprotective microglia, thereby mitigating chronic neuroinflammation in EAE (Fang et al., 2018).
Research on the role of microglia in neuroinflammation is also increasingly abundant. Studies have shown that the conditional knockout of Cx43 in EAE promotes the expression of M2. During the pre-immunization phase, Cx43 icKO spinal microglia demonstrate increased expression of anti-inflammatory genes while suppressing pro-inflammatory gene expression, thereby establishing an anti-inflammatory state prior to immunization. This, along with their synergistic interaction with astrocytes, contributes to the attenuation of EAE progression (Wang et al., 2024). Notably, in mouse models of glaucoma, the inhibition of GJs or ablation of Cx36 has been demonstrated to prevent dendritic remodeling and RGC death (Kumar et al., 2023). It is hypothesized that this phenomenon may be associated with the phenotypic switching of glial cells, which warrants further investigation. Collectively, these findings suggest that Cx proteins play a pivotal role in neuroinflammation.
In the CNS, glial cells, particularly astrocytes, predominantly express Cx43 (Giaume et al., 2021). Growing evidence supports the involvement of astrocyte Cx43 HCs in mediating neuroinflammatory processes (Retamal et al., 2007). Under inflammatory conditions, the activation of inflammasome pathways may coincide with increased Cx43 expression, thereby sustaining abnormal Cx43-mediated ATP release. As pro-inflammatory mediators, HCs facilitate the transfer of toxic metabolites during neuroinflammatory responses. Notably, Cx43 HCs significantly contribute to the amplification and persistence of inflammation by mediating the ATP autocrine feedback loop within the inflammatory cycle (Chen and Tang, 2022).
Collectively, these studies indicate that Cx43 HCs play a crucial role in neuroinflammatory processes. Therefore, strategies targeting glial Cx43 HC activity represent a promising therapeutic approach for neuroinflammatory diseases requiring novel treatment options, as suggested by findings on Cx43 deficiency and pharmacological blockade in neuroinflammation (Yin et al., 2018; Wang et al., 2021, 2020).
As a predominant connexin following glial activation, Cx43 is regulated by a variety of signaling pathways and, in turn, modulates downstream signals essential for neuronal activity, contributing to various central nervous system diseases, including glaucoma. Current gap junction modulators include global non-specific blockers, connexin isoform-specific antisense oligonucleotides, and connexin mimetic peptides (Becker et al., 2016).
Global non-specific blockers indiscriminately affect both GJs and HCs. Commonly used compounds include glycopyrrolate derivatives (Davidson et al., 1986; Takeuchi et al., 2011), as well as anesthetics (Mantz et al., 1993) and anti-inflammatory agents such as enflurane, isoflurane, flufenamic acid, niflumic acid, and meclofenamic acid (Harks et al., 2001; Srinivas and Spray, 2003). Antisense oligonucleotides enter the cell and bind to messenger RNA (mRNA), thereby inhibiting protein synthesis. Their advantages include high specificity and the ability to enable rapid restoration of function. Additionally, their long half-life allows for sustained delivery. Notably, experimental evidence suggests that antisense oligonucleotide-mediated attenuation of TGFβ signaling can restore neural function in adult non-human primates (Peters et al., 2021). Connexin mimetic peptides are short peptide sequences designed to mimic specific intra- and extracellular regions of connexin proteins. Gap26, Gap27, and Gap19 have been widely utilized to inhibit gap junctions primarily composed of Cx43 (Lucero et al., 2020; Li et al., 2015; Walrave et al., 2016). Gap26 attenuates LPS-induced apoptosis by inhibiting the PI3K/AKT pathway (Liu et al., 2021). The application of Peptide 5 effectively reduced Cx43 levels and the number of glial fibrillary acidic protein (GFAP)-positive astrocytes while mitigating neuronal loss in a concentration- and time-dependent manner. Interestingly, low concentrations of peptide 5 prevented HC opening but preserved GJ communication, whereas higher concentrations prevented HC opening but led to the uncoupling of existing GJs (O'Carroll et al., 2008). These findings suggest that connexin mimetic peptides can selectively inhibit HCs while maintaining GJ functionality in a dose- and time-dependent manner.
Therefore, given the critical role of gap junction channels (GJCs) and HCs in glial activity, CXs represent a promising therapeutic target for glaucoma treatment. CX43-mediated glial networks have been proposed as a potential therapeutic approach for glaucoma.
Studies have shown that connexins, particularly CX43, are closely associated with glaucoma. CX43 is widely expressed in the trabecular meshwork (TM) and is predominantly found in astrocytes, Müller cells, and microglial cells, with the highest prevalence in astrocytes (Boal et al., 2021; Tellios et al., 2019). Figure 2 illustrates the role of CX43 in glaucoma. Studies have shown that upregulation of CX43 serves as an initial trigger for the inflammatory cascade leading to RGC death following ischemic retinal injury. However, blocking CX43 HCs can preserve the integrity of the blood-retinal barrier, thereby reducing glial-related secondary tissue damage and exerting significant neuroprotective effects (Toychiev et al., 2021). A well-established causal link exists between vascular dysfunction and glial inflammation. The involvement of Cx43 HCs in RGC death in glaucoma cannot be ruled out. Strong evidence suggests that glaucoma is associated with changes in astrocytic Cx43 expression in both the retina and optic nerve.
Figure 2. The role of CX43 in glaucoma (draw by Figdraw). In glaucoma, astrocyte activation leads to the upregulation of Cx43, which disrupts gap junction intercellular communication (GJIC) and facilitates hemichannel (HC) opening, resulting in excessive ATP release. This ATP release promotes the transition of microglia to a pro-inflammatory phenotype, triggering the secretion of cytokines that further exacerbate astrocyte activation, ultimately resulting in retinal ganglion cell (RGC) apoptosis. Within trabecular meshwork (TM) cells, the upregulation of Cx43 and the subsequent ATP release disrupt purinergic signaling homeostasis through P2Y2 receptors, contributing to increased IOP.
Cx has been implicated in glaucoma progression through its modulation of neuroinflammation and alterations in gap junction intercellular communication (GJIC). Astrocytes, the predominant glial cells in the optic nerve head, form functional syncytia via Cx43-mediated gap junctions, facilitating communication and maintaining ionic and metabolic homeostasis in RGC axons. Elevated IOP in glaucoma has been associated with the loss of GJIC in astrocytes, disruption of RGC axonal homeostasis, acquisition of a reactive phenotype, and promotion of glaucomatous neurodegeneration (Malone et al., 2007). Upregulation of astrocytic Cx43 has been shown to enhance the release of pro-inflammatory factors, exacerbating neural damage. Notably, blocking GJs or ablating Cx36 has been demonstrated to prevent dendritic remodeling and RGC death in murine glaucoma models (Kumar et al., 2023). In glaucoma, increased CX43 immunoreactivity has been observed in the lamina cribrosa, peripapillary, and midperipheral retina, correlating with glial activation (Kerr et al., 2011). In human glaucoma optic nerve head astrocytes, Connexin43 gene expression was found to be elevated compared to controls. CX43 has been reported to mitigate neuroinflammation and alleviate neurodegenerative stress through metabolic resource redistribution in a mouse model of elevated IOP (Cooper et al., 2020). In an experimental glaucoma model with CX43 genetic ablation, astrocytic Cx43 deletion was found to be neuroprotective, enhancing RGC survival (Batsuuri et al., 2021). Astrocytic regulation of glaucomatous RGC survival has been linked to Cx43-mediated ATP release, which may involve modulation of the Rac1/PAK1/CX43 pathway and could represent a potential neuroprotective strategy in glaucoma (Zhao et al., 2021). Additionally, in animal models of unilateral optic nerve injury, retinal Cx43 protein levels were upregulated in both eyes, including the uninjured eye, in parallel with astrocyte activation. These findings suggest that the CX43 network may contribute to the propagation of glaucoma pathology (Chew et al., 2011).
Cx43 is widely expressed in GJs of the TM, where it regulates intercellular communication and extracellular matrix remodeling through both GJs and HCs, thereby influencing aqueous humor outflow and IOP regulation. The upregulation of Cx43 in damaged TM has been identified as a novel phenomenon, suggesting its involvement in the pathogenesis of glaucoma and its significant functional role in this process (Tellios et al., 2019). In a study utilizing a glaucoma mouse model to investigate the role of Cx43 in TM functional regeneration, knockdown of Cx43 expression was shown to attenuate the transplantation of induced pluripotent stem cell (iPSC)-derived TM cells, hinder TM function recovery, suppress endogenous TM cell division, and prevent the restoration of disrupted IOP homeostasis in glaucoma (Sui et al., 2021). In vitro studies have shown that mechanical stretch, simulating elevated IOP, induces upregulation of astrocytic Cx43 expression and promotes apoptosis (Tellios et al., 2019). This effect may be attributed to stress-induced Cx43 upregulation, which enhances intercellular communication and ATP efflux through these channels.
CXs have traditionally been recognized as transmembrane proteins that form gap junctions to facilitate intercellular communication. Recent studies have demonstrated that CXs influence tissue homeostasis through channel-independent mechanisms (Ha, 2009). The non-channel-dependent functions of CXs are integral to transcription, metabolism, cell/cell and cell/extracellular matrix (ECM) adhesion, and cell signaling (Iacobas et al., 2004; Li et al., 2023).
Cx43 has been localized to mitochondria in various cell types, including astrocytes (Kozoriz et al., 2010). Studies indicate that mitochondrial Cx43 (mtCx43) regulates ATP and ROS production, thereby modulating tissue injury, inflammation, and apoptosis (Li et al., 2023). Mitochondrial dysfunction and oxidative damage in RGCs are central mechanisms in glaucomatous neurodegeneration. Thus, mtCx43 dysfunction may exacerbate apoptosis in this condition. Another study demonstrated that Annular Cx43 HC-containing vesicles can form Cx43 GJs with recipient cells, serving as a mechanism for facilitating internalization that modulates inflammatory responses and degenerative processes (Varela-Eirín et al., 2022), potentially contributing to glaucoma progression.
In the TM, Cx43 interacts with the scaffolding protein ZO-1 and associates with integrins, forming multi-protein complexes that regulate ECM remodeling in response to mechanical stress (Li et al., 2020a). Another study identified Cx43 as a key regulator of phagocytic activity in human TM cells, thereby contributing to glaucoma pathogenesis (Li et al., 2020b). Moreover, Cx43 has been implicated in the regulation of epithelial-mesenchymal transition (EMT) and fibrotic processes. Although the specific mechanisms require further investigation, these findings highlight CXs as promising therapeutic targets for these disorders (Li et al., 2023).
These non-channel functions of CXs offer novel insights into glaucoma pathophysiology. Future research should utilize glaucoma models to elucidate regulatory mechanisms and explore therapeutic potential in neuroprotection and intraocular pressure regulation.
Therefore, targeting Cx43 may simultaneously improve aqueous humor dynamics and provide neuroprotection, potentially representing a novel approach for the comprehensive management of glaucoma. It is evident that the pathological mechanisms of HCs and GJs play a crucial role in disease progression. The gap junction network may exert neuroprotective effects during the early stages of the disease. Given that glaucoma is a chronic progressive condition, the roles of HCs and GJs in its pathogenesis warrant further investigation.
CX43 in glial cells ensures proper glial function and neuronal activity, which, in turn, regulates their survival and activity. This coordination is essential for maintaining functional integration throughout the CNS. GJs and HCs, formed by CXs, are both functionally synergistic and antagonistic. The role of HCs as pathological channels in glial activation is now well-established, as HCs become activated in pathological conditions, regulating various downstream signaling factors that accelerate neuroinflammation and promote disease progression. However, the role of GJs remains uncertain, as they may exert both beneficial and deleterious effects. Further clarification is required regarding the regulatory nodes that mediate these opposing effects. Additionally, the signaling pathways of connexins in neuroprotection warrant further investigation.
A better understanding of the complex role of astrocyte CX43 in glaucoma may facilitate significant therapeutic advancements. However, CX43 is widely expressed in multiple tissues throughout the body, posing a considerable challenge for the specific targeting of ocular CX43. Localized drug delivery and gene therapy techniques may enhance treatment specificity and safety. Studies have demonstrated that excessive opening of CX43 hemichannels is associated with ganglion cell damage in glaucoma. The use of specific inhibitors (such as Gap19 and TAT-Gap19) can mitigate neuroinflammation and apoptosis, highlighting their potential for clinical application. Regulating CX43 expression through gene-editing technologies (e.g., CRISPR/Cas9) or RNA interference (siRNA) may also provide innovative therapeutic avenues for glaucoma. Additionally, developing peptides that mimic CX43 function to modulate intercellular communication and protect retinal ganglion cells represents another promising strategy. Furthermore, utilizing carriers such as nanoparticles or liposomes to precisely deliver CX43-related drugs to ocular targets may serve as a novel therapeutic approach for glaucoma management. More refined clinical evaluation methods are required to accurately assess the efficacy of CX43-targeted therapies in glaucoma patients. Currently, most CX43-related therapeutic strategies are still in the preclinical phase, and future large-scale clinical trials are required to validate their safety and effectiveness. In summary, CX43 represents a promising therapeutic target for glaucoma, with multiple strategies under development. As research advances and technology progresses, CX43-based therapies are anticipated to emerge as a crucial component of future glaucoma treatment strategies.
QS: Conceptualization, Investigation, Writing – original draft, Writing – review & editing. XW: Conceptualization, Writing – original draft. JY: Funding acquisition, Writing – review & editing. SL: Investigation, Writing – review & editing. JD: Conceptualization, Methodology, Writing – review & editing.
The author(s) declare that financial support was received for the research and/or publication of this article. This work was supported by Sichuan Science and Technology Program (2023NSFSC1792) and China Postdoctoral Science Foundation (2023MD734105).
The authors declare that the research was conducted in the absence of any commercial or financial relationships that could be construed as a potential conflict of interest.
The author(s) declare that no Gen AI was used in the creation of this manuscript.
All claims expressed in this article are solely those of the authors and do not necessarily represent those of their affiliated organizations, or those of the publisher, the editors and the reviewers. Any product that may be evaluated in this article, or claim that may be made by its manufacturer, is not guaranteed or endorsed by the publisher.
Altevogt, B. M., and Paul, D. L. (2004). Four classes of intercellular channels between glial cells in the CNS. J. Neurosci. 24, 4313–4323. doi: 10.1523/JNEUROSCI.3303-03.2004
Anderson, C. M., and Swanson, R. A. (2000). Astrocyte glutamate transport: review of properties, regulation, and physiological functions. Glia 32, 1–14.3.3. doi: 10.1002/1098-1136(200010)32:1<1::AID-GLIA10>3.3.CO
Bariş, M., and Tezel, G. (2019). Immunomodulation as a neuroprotective strategy for glaucoma treatment. Curr. Ophthalmol. Rep. 7, 160–169. doi: 10.1007/s40135-019-00212-1
Batsuuri, K., Toychiev, A., and Srinivas, M. (2021). Genetic ablation of Cx43 in astrocytes is neuroprotective in experimental glaucoma and optic nerve injury. Invest. Ophthalmol. Visual Sci. 62:1691. Available online at: https://iovs.arvojournals.org/article.aspx?articleid=2775118
Becker, D. L., Phillips, A. R., Duft, B. J., Kim, Y., and Green, C. R. (2016). Translating connexin biology into therapeutics. Sem Cell Dev Biol. 50, 49–58. doi: 10.1016/j.semcdb.2015.12.009
Bennett, M. V., Garré, J. M., Orellana, J. A., Bukauskas, F. F., Nedergaard, M., Giaume, C., et al. (2012). Connexin and pannexin hemichannels in inflammatory responses of glia and neurons. Brain Res. 1487, 3–15. doi: 10.1016/j.brainres.2012.08.042
Bennett, M. V. L., Contreras, J. E., Bukauskas, F. F., and Sáez, J. C. (2003). New roles for astrocytes: gap junction hemichannels have something to communicate. Trends Neurosci. 26, 610–617. doi: 10.1016/j.tins.2003.09.008
Boal, A. M., Risner, M. L., Cooper, M. L., Wareham, L. K., and Calkins, D. J. (2021). Astrocyte networks as therapeutic targets in glaucomatous neurodegeneration. Cells 10:1368. doi: 10.3390/cells10061368
Borst, K., Dumas, A. A., and Prinz, M. (2021). Microglia: immune and non-immune functions. Immunity 54, 2194–2208. doi: 10.1016/j.immuni.2021.09.014
Bourne, R. R., Stevens, G. A., White, R. A., Smith, J. L., Flaxman, S. R., Price, H., et al. (2013). Causes of vision loss worldwide, 1990–2010: a systematic analysis. Lancet Glob. Health 1, e339–e349. doi: 10.1016/S2214-109X(13)70113-X
Burgoyne, C. F. A. (2011). Biomechanical paradigm for axonal insult within the optic nerve head in aging and glaucoma. Exp. Eye Res. 93, 120–132. doi: 10.1016/j.exer.2010.09.005
Caruso, G., Di Pietro, L., and Caraci, F. (2023). Gap junctions and connexins in microglia-related oxidative stress and neuroinflammation: perspectives for drug discovery. Biomolecules 13:505. doi: 10.3390/biom13030505
Charvériat, M., Mouthon, F., Rein, W., and Verkhratsky, A. (2021). Connexins as therapeutic targets in neurological and neuropsychiatric disorders. Biochim. Biophys. Acta Mol. Basis Dis. 1867:166098. doi: 10.1016/j.bbadis.2021.166098
Chen, Y., and Tang, L. (2022). The crosstalk between parenchymal cells and macrophages: a keeper of tissue homeostasis. Front. Immunol. 13:1050188. doi: 10.3389/fimmu.2022.1050188
Chew, S. S., Johnson, C. S., Green, C. R., and Danesh-Meyer, H. V. (2011). Response of retinal Connexin43 to optic nerve injury. Invest. Ophthalmol. Visual Sci. 52, 3620–3629. doi: 10.1167/iovs.10-6318
Condorelli, D. F., Trovato Salinaro, A., Mudò, G., Mirone, M. B., and Belluardo, N. (2003). Cellular expression of connexins in the rat brain: neuronal localization, effects of kainate-induced seizures and expression in apoptotic neuronal cells. Euro. J. Neurosci. 18, 1807–1827. doi: 10.1046/j.1460-9568.2003.02910.x
Cooper, M. L., Pasini, S., Lambert, W. S., D'Alessandro, K. B., Yao, V., Risner, M. L., et al. (2020). Redistribution of metabolic resources through astrocyte networks mitigates neurodegenerative stress. Proc. Natl. Acad. Sci. U.S.A. 117, 18810–18821. doi: 10.1073/pnas.2009425117
Danish, A., Gedschold, R., Hinz, S., Schiedel, A. C., Thimm, D., Bedner, P., et al. (2021). A cellular assay for the identification and characterization of connexin gap junction modulators. Int. J. Mol. Sci. 22:1417. doi: 10.3390/ijms22031417
Davidson, J. S., Baumgarten, I. M., and Harley, E. H. (1986). Reversible inhibition of intercellular junctional communication by glycyrrhetinic acid. Biochem. Biophys. Res. Commun. 134, 29–36. doi: 10.1016/0006-291X(86)90522-X
De Vuyst, E., Wang, N., Decrock, E., De Bock, M., Vinken, M., Van Moorhem, M., et al. (2009). Ca2+ regulation of connexin 43 hemichannels in C6 glioma and glial cells. Cell Calcium 46, 176–187. doi: 10.1016/j.ceca.2009.07.002
Denaro, S., D'Aprile, S., Vicario, N., and Parenti, R. (2025). Mechanistic insights into connexin-mediated neuroglia crosstalk in neurodegenerative diseases. Front. Cell. Neurosci. 19:1532960. doi: 10.3389/fncel.2025.1532960
Dermietzel, R., Farooq, M., Kessler, J. A., Althaus, H., Hertzberg, E. L., and Spray, D. C. (1997). Oligodendrocytes express gap junction proteins connexin32 and connexin45. Glia 20, 101–114. doi: 10.1002/(SICI)1098-1136(199706)20:2<101::AID-GLIA2>3.0.CO;2-C
Eugenín, E. A., Eckardt, D., Theis, M., Willecke, K., Bennett, M. V., and Saez, J. C. (2001). Microglia at brain stab wounds express connexin 43 and in vitro form functional gap junctions after treatment with interferon-γ and tumor necrosis factor-α. Proc. Natl. Acad. Sci. U.S.A. 98, 4190–4195. doi: 10.1073/pnas.051634298
Fang, M., Yamasaki, R., Li, G., Masaki, K., Yamaguchi, H., Fujita, A., et al. (2018). Connexin 30 deficiency attenuates chronic but not acute phases of experimental autoimmune encephalomyelitis through induction of neuroprotective microglia. Front. Immunol. 9:2588. doi: 10.3389/fimmu.2018.02588
Fernandez-Albarral, J. A., Ramírez, A. I., de Hoz, R., and Salazar, J. J. (2022). Retinal microglial activation in glaucoma: evolution over time in a unilateral ocular hypertension model. Neural Regener. Res. 17, 797–799. doi: 10.4103/1673-5374.322454
Garg, S., Syed, M. M., and Kielian, T. (2005). Staphylococcus aureus-derived peptidoglycan induces Cx43 expression and functional gap junction intercellular communication in microglia. J. Neurochem. 95, 475–483. doi: 10.1111/j.1471-4159.2005.03384.x
Giaume, C., Naus, C. C., Sáez, J. C., and Leybaert, L. (2021). Glial connexins and pannexins in the healthy and diseased brain. Physiol. Rev. 101, 93–145. doi: 10.1152/physrev.00043.2018
Ha, D. (2009). Connexins: a myriad of functions extending beyond assembly of gap junction channels. Cell Commun. Signal. 7, 1–17. doi: 10.1186/1478-811X-7-4
Harks, E. G., de Roos, A. D., Peters, P. H., de Haan, L. H., Brouwer, A., Ypey, D. L., et al. (2001). Fenamates: a novel class of reversible gap junction blockers. J. Pharmacol. Exp. Ther. 298, 1033–1041. doi: 10.1016/S0022-3565(24)29472-6
Harris, A. L. (2007). Connexin channel permeability to cytoplasmic molecules. Progress Biophys. Mol. Biol. 94, 120–143. doi: 10.1016/j.pbiomolbio.2007.03.011
Hu, X., Zhao, G. L., Xu, M. X., Zhou, H., Li, F., Miao, Y., et al. (2021). Interplay between Müller cells and microglia aggravates retinal inflammatory response in experimental glaucoma. J. Neuroinflamm. 18:303. doi: 10.1186/s12974-021-02366-x
Huang, X., Su, Y., Wang, N., Li, H., Li, Z., Yin, G., et al. (2021). Astroglial connexins in neurodegenerative diseases. Front. Mol. Neurosci. 14:657514. doi: 10.3389/fnmol.2021.657514
Iacobas, D. A., Scemes, E., and Spray, D. C. (2004). Gene expression alterations in connexin null mice extend beyond the gap junction. Neurochem. Int. 45, 243–250. doi: 10.1016/j.neuint.2003.12.008
Kerr, N. M., Johnson, C. S., de Souza, C. F., Chee, K.-S., Good, W. R., Green, C. R., et al. (2010). Immunolocalization of gap junction protein connexin43 (GJA1) in the human retina and optic nerve. Invest. Ophthalmol. Visual Sci. 51, 4028–4034. doi: 10.1167/iovs.09-4847
Kerr, N. M., Johnson, C. S., Green, C. R., and Danesh-Meyer, H. V. (2011). Gap junction protein connexin43 (GJA1) in the human glaucomatous optic nerve head and retina. J. Clin. Neurosci. 18, 102–108. doi: 10.1016/j.jocn.2010.06.002
Kleopa, K. A. (2011). The role of gap junctions in Charcot-Marie-Tooth disease. J. Neurosci. 31, 17753–17760. doi: 10.1523/JNEUROSCI.4824-11.2011
Kozoriz, M. G., Church, J., Ozog, M. A., Naus, C. C., and Krebs, C. (2010). Temporary sequestration of potassium by mitochondria in astrocytes. J. Biol. Chem. 285, 31107–31119. doi: 10.1074/jbc.M109.082073
Kumar, S., Akopian, A., and Bloomfield, S. A. (2023). Neuroprotection of retinal ganglion cells suppresses microglia activation in a mouse model of glaucoma. Invest. Ophthalmol. Visual Sci. 64:24. doi: 10.1167/iovs.64.7.24
Lampron, A., ElAli, A., and Rivest, S. (2013). Innate immunity in the CNS: redefining the relationship between the CNS and Its environment. Neuron 78, 214–232. doi: 10.1016/j.neuron.2013.04.005
Lei, L., Wang, Y.-T., Hu, D., Gai, C., and Zhang, Y. (2023). Astroglial connexin 43-mediated gap junctions and hemichannels: potential antidepressant mechanisms and the link to neuroinflammation. Cell. Mol. Neurobiol. 43, 4023–4040. doi: 10.1007/s10571-023-01426-5
Li, M., Nie, H., He, Q., Zhang, Z., Yu, S., Wang, T., et al. (2025). Astrocytic Gap Junctions protein Cx43/Cx30 modulate EAAT1 and glutamate to mediate cerebral ischemia–reperfusion injury. Brain Res. 1847:149306. doi: 10.1016/j.brainres.2024.149306
Li, X., Acott, T. S., Nagy, J. I., and Kelley, M. J. (2020a). ZO-1 associates with α3 integrin and connexin43 in trabecular meshwork and Schlemm's canal cells. Int. J. Physiol. Pathophysiol. Pharmacol. 12:1. Available online at: https://pmc.ncbi.nlm.nih.gov/articles/PMC7076326/
Li, X., Nagy, J. I., Li, D., Acott, T. S., and Kelley, M. J. (2020b). Gap junction connexin43 is a key element in mediating phagocytosis activity in human trabecular meshwork cells. Int. J. Physiol. Pathophysiol. Pharmacol. 12:25. Available online at: https://pmc.ncbi.nlm.nih.gov/articles/PMC7076328/
Li, X., Zhao, H., Tan, X., Kostrzewa, R. M., Du, G., Chen, Y., et al. (2015). Inhibition of connexin43 improves functional recovery after ischemic brain injury in neonatal rats. Glia 63, 1553–1567. doi: 10.1002/glia.22826
Li, Y., Acosta, F. M., and Jiang, J. X. (2023). Gap junctions or hemichannel-dependent and independent roles of connexins in fibrosis, epithelial–mesenchymal transitions, and wound healing. Biomolecules 13:1796. doi: 10.3390/biom13121796
Liu, L., Yan, M., Yang, R., Qin, X., Chen, L., Li, L., et al. (2021). Adiponectin attenuates lipopolysaccharide-induced apoptosis by regulating the Cx43/PI3K/AKT pathway. Front. Pharmacol. 12:644225. doi: 10.3389/fphar.2021.644225
Lopez Ortiz, A. O., and Eyo, U. B. (2024). Astrocytes and microglia in the coordination of CNS development and homeostasis. J. Neurochem. 168, 3599–3614. doi: 10.1111/jnc.16006
Lucero, C. M., Andrade, D. C., Toledo, C., Díaz, H. S., Pereyra, K. V., Diaz-Jara, E., et al. (2020). Cardiac remodeling and arrhythmogenesis are ameliorated by administration of Cx43 mimetic peptide Gap27 in heart failure rats. Sci. Rep. 10, 1–12. doi: 10.1038/s41598-020-63336-6
Maldonado, P. P., Guevara, C., Olesen, M. A., Orellana, J. A., Quintanilla, R. A., and Ortiz, F. C. (2022). Neurodegeneration in multiple sclerosis: the role of Nrf2-dependent pathways. Antioxidants 11:1146. doi: 10.3390/antiox11061146
Malone, P., Miao, H., Parker, A., Juarez, S., and Hernandez, M. R. (2007). Pressure induces loss of gap junction communication and redistribution of connexin 43 in astrocytes. Glia 55, 1085–1098. doi: 10.1002/glia.20527
Mantz, J., Cordier, J., and Giaume, C. (1993). Effects of general anesthetics on intercellular communications mediated by gap junctions between astrocytes in primary culture. J. Am. Soc. Anesthesiol. 78, 892–901. doi: 10.1097/00000542-199305000-00014
McMonnies, C. (2018). Reactive oxygen species, oxidative stress, glaucoma and hyperbaric oxygen therapy. J. Optom. 11, 3–9. doi: 10.1016/j.optom.2017.06.002
Medzhitov, R. (2008). Origin and physiological roles of inflammation. Nature 454, 428–435. doi: 10.1038/nature07201
Mélik Parsadaniantz, S., Réaux-le Goazigo, A., Sapienza, A., Habas, C., and Baudouin, C. (2020). Glaucoma: a degenerative optic neuropathy related to neuroinflammation? Cells 9:535. doi: 10.3390/cells9030535
Nutma, E., van Gent, D., Amor, S., and Peferoen, L. A. (2020). Astrocyte and oligodendrocyte cross-talk in the central nervous system. Cells 9:600. doi: 10.3390/cells9030600
O'Carroll, S. J., Alkadhi, M., Nicholson, L. F. B., and Green, C. R. (2008). Connexin43 mimetic peptides reduce swelling, astrogliosis, and neuronal cell death after spinal cord injury. Cell Commun. Adhesion 15, 27–42. doi: 10.1080/15419060802014164
Oguro, K., Jover, T., Tanaka, H., Lin, Y., Kojima, T., Oguro, N., et al. (2001). Global ischemia-induced increases in the gap junctional proteins connexin 32 (Cx32) and Cx36 in hippocampus and enhanced vulnerability of Cx32 knock-out mice. J. Neurosci. 21, 7534–7542. doi: 10.1523/JNEUROSCI.21-19-07534.2001
Orihuela, R., McPherson, C. A., and Harry, G. J. (2016). Microglial M1/M2 polarization and metabolic states. Br. J. Pharmacol. 173, 649–665. doi: 10.1111/bph.13139
Ou, K., Li, Y., Liu, L., Li, H., Cox, K., Wu, J., et al. (2022). Recent developments of neuroprotective agents for degenerative retinal disorders. Neural Regener. Res. 17, 1919–1928. doi: 10.4103/1673-5374.335140
Peters, S., Kuespert, S., Wirkert, E., Heydn, R., Jurek, B., Johannesen, S., et al. (2021). Reconditioning the neurogenic niche of adult non-human primates by antisense oligonucleotide-mediated attenuation of TGFβ signaling. Neurotherapeutics 18, 1963–1979. doi: 10.1007/s13311-021-01045-2
Provenzano, F., Torazza, C., Bonifacino, T., Bonanno, G., and Milanese, M. (2023). The key role of astrocytes in amyotrophic lateral sclerosis and their commitment to glutamate excitotoxicity. Int. J. Mol. Sci. 24:15430. doi: 10.3390/ijms242015430
Quigley, H. A. (1999). Neuronal death in glaucoma. Progress Retinal Eye Res. 18, 39–57. doi: 10.1016/S1350-9462(98)00014-7
Retamal, M. A., Froger, N., Palacios-Prado, N., Ezan, P., Sáez, P. J., Sáez, J. C., et al. (2007). Cx43 hemichannels and gap junction channels in astrocytes are regulated oppositely by proinflammatory cytokines released from activated microglia. J. Neurosci. 27, 13781–13792. doi: 10.1523/JNEUROSCI.2042-07.2007
Salkar, A., Vander Wall, R., Basavarajappa, D., Chitranshi, N., Parilla, G. E., Mirzaei, M., et al. (2024). Glial cell activation and immune responses in glaucoma: a systematic review of human postmortem studies of the retina and optic nerve. Aging Dis. 15:2069. doi: 10.14336/AD.2024.0103
Sargiannidou, I., Vavlitou, N., Aristodemou, S., Hadjisavvas, A., Kyriacou, K., Scherer, S. S., et al. (2009). Connexin32 mutations cause loss of function in Schwann cells and oligodendrocytes leading to PNS and CNS myelination defects. J. Neurosci. 29, 4736–4749. doi: 10.1523/JNEUROSCI.0325-09.2009
Seo, J. H., Dalal, M. S., and Contreras, J. E. (2021). Pannexin-1 channels as mediators of neuroinflammation. Int. J. Mol. Sci. 22:5189. doi: 10.3390/ijms22105189
Söhl, G., Güldenagel, M., Traub, O., and Willecke, K. (2000). Connexin expression in the retina. Brain Res. Rev. 32, 138–145. doi: 10.1016/S0165-0173(99)00074-0
Soto, I., and Howell, G. R. (2014). The complex role of neuroinflammation in glaucoma. Cold Spring Harbor Perspect. Med. 4:a017269. doi: 10.1101/cshperspect.a017269
Srinivas, M., and Spray, D. C. (2003). Closure of gap junction channels by arylaminobenzoates. Mol. Pharmacol. 63, 1389–1397. doi: 10.1124/mol.63.6.1389
Sui, S., Yu, H., Wang, X., Wang, W., Yang, X., Pan, X., et al. (2021). iPSC-derived trabecular meshwork cells stimulate endogenous TM cell division through gap junction in a mouse model of glaucoma. Invest. Ophthalmol. Visual Sci. 62:28. doi: 10.1167/iovs.62.10.28
Takeuchi, H., Mizoguchi, H., Doi, Y., Jin, S., Noda, M., Liang, J., et al. (2011). Blockade of gap junction hemichannel suppresses disease progression in mouse models of amyotrophic lateral sclerosis and Alzheimer's disease. PLoS ONE 6:e21108. doi: 10.1371/journal.pone.0021108
Teleanu, D. M., Niculescu, A. G., Lungu, I. I., Radu, C. I., Vladâcenco, O., Roza, E., et al. (2022). An overview of oxidative stress, neuroinflammation, and neurodegenerative diseases. Int. J. Mol. Sci. 23:5938. doi: 10.3390/ijms23115938
Tellios, N., Feng, M., Chen, N., Liu, H., Tellios, V., Wang, M., et al. (2019). Mechanical stretch upregulates connexin43 in human trabecular meshwork cells. Clin. Exp. Ophthalmol. 47, 787–794. doi: 10.1111/ceo.13492
Tezel, G. (2022). Molecular regulation of neuroinflammation in glaucoma: current knowledge and the ongoing search for new treatment targets. Progress Retinal Eye Res. 87:100998. doi: 10.1016/j.preteyeres.2021.100998
Tham, Y. C., Li, X., Wong, T. Y., Quigley, H. A., Aung, T., and Cheng, C. Y. (2014). Global prevalence of glaucoma and projections of glaucoma burden through 2040: a systematic review and meta-analysis. Ophthalmology 121, 2081–2090. doi: 10.1016/j.ophtha.2014.05.013
Toychiev, A. H., Batsuuri, K., and Srinivas, M. (2021). Gap junctional coupling between retinal astrocytes exacerbates neuronal damage in ischemia-reperfusion injury. Invest. Ophthalmol. Visual Sci. 62:27. doi: 10.1167/iovs.62.14.27
Une, H., Yamasaki, R., Nagata, S., Yamaguchi, H., Nakamuta, Y., Indiasari, U. C., et al. (2021). Brain gray matter astroglia-specific connexin 43 ablation attenuates spinal cord inflammatory demyelination. J. Neuroinflamm. 18:126. doi: 10.1186/s12974-021-02176-1
Varela-Eirín, M., Carpintero-Fernández, P., Guitián-Caamaño, A., Varela-Vázquez, A., García-Yuste, A., Sánchez-Temprano, A., et al. (2022). Extracellular vesicles enriched in connexin 43 promote a senescent phenotype in bone and synovial cells contributing to osteoarthritis progression. Cell Death Dis. 13:681. doi: 10.1038/s41419-022-05089-w
Vinken, M. (2015). Connexin hemichannels: novel mediators of toxicity. Arch. Toxicol. 89, 143–145. doi: 10.1007/s00204-014-1422-4
Voet, S., Srinivasan, S., Lamkanfi, M., and van Loo, G. (2019). Inflammasomes in neuroinflammatory and neurodegenerative diseases. EMBO Mol. Med. 11:e10248. doi: 10.15252/emmm.201810248
Voigt, J., Grosche, A., Vogler, S., Pannicke, T., Hollborn, M., Kohen, L., et al. (2015). Nonvesicular release of ATP from rat retinal glial (Müller) cells is differentially mediated in response to osmotic stress and glutamate. Neurochem. Res. 40, 651–660. doi: 10.1007/s11064-014-1511-z
Walrave, L., Vinken, M., Albertini, G., De Bundel, D., Leybaert, L., and Smolders, I. J. (2016). Inhibition of connexin43 hemichannels impairs spatial short-term memory without affecting spatial working memory. Front. Cell. Neurosci. 10:288. doi: 10.3389/fncel.2016.00288
Wang, H., Yang, Y., Yang, S., Ren, S., Feng, J., Liu, Y., et al. (2021). Ginsenoside Rg1 ameliorates neuroinflammation via suppression of connexin43 ubiquitination to attenuate depression. Front. Pharmacol. 12:709019. doi: 10.3389/fphar.2021.709019
Wang, Q., Dong, J., Du, M., Liu, X., Zhang, S., Zhang, D., et al. (2024). Chitosan-rapamycin carbon dots alleviate glaucomatous retinal injury by inducing autophagy to promote M2 microglial polarization. Int. J. Nanomed. 19, 2265–2284. doi: 10.2147/IJN.S440025
Wang, X., Feng, L., Xin, M., Hao, Y., Wang, X., Shang, P., et al. (2020). Mechanisms underlying astrocytic connexin-43 autophagy degradation during cerebral ischemia injury and the effect on neuroinflammation and cell apoptosis. Biomed. Pharmacother. 127:110125. doi: 10.1016/j.biopha.2020.110125
Wei, X., Cho, K.-S., Thee, E. F., Jager, M. J., and Chen, D. F. (2019). Neuroinflammation and microglia in glaucoma: time for a paradigm shift. J. Neurosci. Res. 97, 70–76. doi: 10.1002/jnr.24256
Woodburn, S. C., Bollinger, J. L., and Wohleb, E. S. (2021). The semantics of microglia activation: neuroinflammation, homeostasis, and stress. J. Neuroinflamm. 18, 1–16. doi: 10.1186/s12974-021-02309-6
Xu, M.-X., Zhao, G.-L., Hu, X., Zhou, H., Li, S.-Y., Li, F., et al. (2022). P2X7/P2X4 receptors mediate proliferation and migration of retinal microglia in experimental glaucoma in mice. Neurosci. Bull. 38, 901–915. doi: 10.1007/s12264-022-00833-w
Yin, X., Feng, L., Ma, D., Yin, P., Wang, X., Hou, S., et al. (2018). Roles of astrocytic connexin-43, hemichannels, and gap junctions in oxygen-glucose deprivation/reperfusion injury induced neuroinflammation and the possible regulatory mechanisms of salvianolic acid B and carbenoxolone. J. Neuroinflamm. 15, 1–24. doi: 10.1186/s12974-018-1127-3
Yoo, H., Shanmugalingam, U., and Smith, P. D. (2021). Harnessing astrocytes and müller glial cells in the retina for survival and regeneration of retinal ganglion cells. Cells 10:1339. doi: 10.3390/cells10061339
Keywords: glial connexins, glaucoma, neuroinflammation, Cx43, gap junction
Citation: Song Q, Wu X, Yang J, Li S and Duan J (2025) Glial connexins in glaucoma. Front. Neurosci. 19:1560344. doi: 10.3389/fnins.2025.1560344
Received: 14 January 2025; Accepted: 24 March 2025;
Published: 09 April 2025.
Edited by:
Lucia Gozzo, University of Catania, ItalyReviewed by:
Lidawani Lambuk, Universiti Sains Malaysia, MalaysiaCopyright © 2025 Song, Wu, Yang, Li and Duan. This is an open-access article distributed under the terms of the Creative Commons Attribution License (CC BY). The use, distribution or reproduction in other forums is permitted, provided the original author(s) and the copyright owner(s) are credited and that the original publication in this journal is cited, in accordance with accepted academic practice. No use, distribution or reproduction is permitted which does not comply with these terms.
*Correspondence: Junguo Duan, ZHVhbmpnQGNkdXRjbS5lZHUuY24=
Disclaimer: All claims expressed in this article are solely those of the authors and do not necessarily represent those of their affiliated organizations, or those of the publisher, the editors and the reviewers. Any product that may be evaluated in this article or claim that may be made by its manufacturer is not guaranteed or endorsed by the publisher.
Research integrity at Frontiers
Learn more about the work of our research integrity team to safeguard the quality of each article we publish.