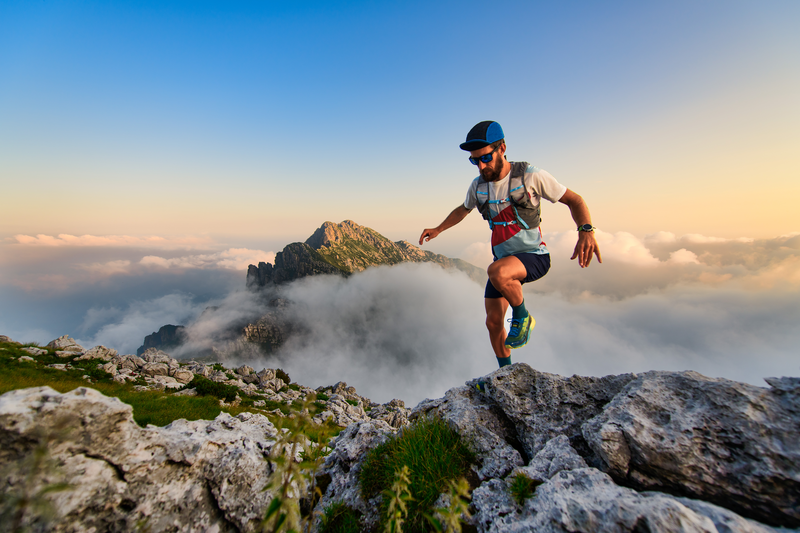
94% of researchers rate our articles as excellent or good
Learn more about the work of our research integrity team to safeguard the quality of each article we publish.
Find out more
ORIGINAL RESEARCH article
Front. Neurosci.
Sec. Perception Science
Volume 19 - 2025 | doi: 10.3389/fnins.2025.1536308
The final, formatted version of the article will be published soon.
You have multiple emails registered with Frontiers:
Please enter your email address:
If you already have an account, please login
You don't have a Frontiers account ? You can register here
The functional connectivity of the posterior parietal cortex-primary motor cortex (PPC-M1) is involved in goal-directed reaching actions and integrating visuomotor transformation. Human area V6A (hV6A), located in the medial PPC, is a critical node of the dorsomedial system that is involved in targeting during reaching movements. Here, we used Electroencephalography (EEG) to investigate functional connectivity and network efficiency during right-hand reaching tasks after inducing left hV6A activity with intermittent theta burst stimulation (iTBS).Methods: Based on individualized MRI neural navigation, 23 healthy subjects were randomly accepted into either real left hV6A or sham iTBS on two days. Resting-state and goal-directed reaching task EEG were recorded at baseline and immediately after iTBS to assess the effects of iTBS on functional connectivity. Alongside the reaching task, an additional Stroop test was conducted to assess each participant's degree of attention.Results: In the alpha band, medial posterior parietal cortical interhemispheric functional connectivity significantly increased during right-hand reaching tasks after hV6A iTBS (P=0.008) but not after sham iTBS (P=0.726). Alpha and beta band small-worldness of right-hand reaching tasks significantly increased (P=0.001 and 0.013, respectively) but not after sham iTBS (P=0.915 and 0.511, respectively).Discussions: Functional connectivity of the bilateral PPC and functional network efficiency increased after iTBS of the left hV6A during right-hand reaching tasks. These findings indicate that the left hV6A should be a potential target for iTBS modulation to improve the orienting movement function in space.
Keywords: Transcranial Magnetic Stimulation, Posterior parietal cortex, Functional Neuroimaging, reaching, V6A
Received: 28 Nov 2024; Accepted: 12 Mar 2025.
Copyright: © 2025 Chen, Xu, Ding, Li, Huang, Yao, Guo, Wang, Wei and Lan. This is an open-access article distributed under the terms of the Creative Commons Attribution License (CC BY). The use, distribution or reproduction in other forums is permitted, provided the original author(s) or licensor are credited and that the original publication in this journal is cited, in accordance with accepted academic practice. No use, distribution or reproduction is permitted which does not comply with these terms.
* Correspondence:
Guang-qing Xu, Department of Rehabilitation Medicine, Guangdong Provincial People's Hospital (Guangdong Academy of Medical Sciences), Southern Medical University, No. 106 Zhongshan Road II, Guangzhou, China
Disclaimer: All claims expressed in this article are solely those of the authors and do not necessarily represent those of their affiliated organizations, or those of the publisher, the editors and the reviewers. Any product that may be evaluated in this article or claim that may be made by its manufacturer is not guaranteed or endorsed by the publisher.
Research integrity at Frontiers
Learn more about the work of our research integrity team to safeguard the quality of each article we publish.